Abstract
The present study emphasizes synthesis and bioprofiling of embelin, embelin-metal (EM) and embelin-azo-metal (EAM) complexes in detail. EM complexes were prepared using pure embelin and d-block transition elements, namely Mn, Fe, Co, Ni, Cu, and Zn. Similarly, EAM complexes were synthesized using phenyl azo-embelin with the said transition metals. Embelin, EM, and EAM complexes were subjected to ultra violet visible spectroscopy, Fourier transform infrared spectroscopy, nuclear magnetic resonance, electrospray ionization mass spectrometry, thermogravimetric analysis, carbon hydrogen nitrogen sulfur analysis. With regard to bioprofiling, the test complexes were studied for the antioxidant and antimicrobial activities. Results revealed that the prepared EM and EAM complexes form octahedral complexes with embelin with the yield in the range of 45–75%. All the instrumental analyses authenticate the interaction of metals with bidentate embelin through its enolic and quinonic oxygen atoms as [M(Emb)2(H2O)2]H2O and [M(Emb-Azo)2(H2O)2]. The antioxidant profile studies suggested that upon complexation with metals, the free radical scavenging activity of embelin reduced significantly. But, with regard to antimicrobial activity, cobalt and nickel embelin complexes displayed>80% growth inhibition in comparison with embelin alone. The hemolytic activity studies suggested that both embelin and the metal complexes are non-hemolytic. The reason for the reduction in antioxidant and an increase in antimicrobial activities were discussed in detail.
1. Introduction
Quinones are diverse class of phenolic compounds with wide range of biological activities [Citation1,Citation2]. Among them, naturally occurring naphthoquinones are widespread. Benzoquinones are also prevalent and extensive work has been done in the synthesis of their analogues. Embelin (2, 5-dihydroxy-3-undecyl-1, 4-benzoquinone) an orange colored pigment isolated from the berries of an Indian shrub Embelia ribes gained a great medicinal importance because of its antihelmintic [Citation3], analgesic [Citation4], antifertility [Citation5], anticancer [Citation6], wound healing [Citation7], ultraviolet B (UVB) radiation inhibition and antimicrobial properties [Citation8]. In addition, only a few reports revealed the antioxidant property of embelin [Citation9].
In general, the biological activity of embelin is attributed to the formation of semiquinone radical. The presence of quinone carbonyl and enolic hydroxyl groups in the same molecule is believed to enhance its antioxidant activity. Embelin, displayed high affinity toward transition metal ions, which, results in the formation of embelin-metal (EM) complexes and the existing reports available on EM complexes dealt only the structural elucidation with physical characterization [Citation10–15]. Though the biological properties of embelin had been widely studied, the unavailability of biological properties of metal complexes necessitates the present study. According to Chopra et al. [Citation16], complexation with metals reduces the inherent biological activity of the drug molecule. In the case of tetracycline, on coordination with metals, the antimicrobial activity reduced considerably. According to Lecomte et al. [Citation17] and Wallis et al. [Citation18], cations such as magnesium and aluminum when complexed with fluoroquinolones, results with low oral bioavailability and causes therapeutic failures. However, Bakola-Christianopoulou et al. [Citation19] reported that an increased antimicrobial activity by hydroxyquinone metal complexes and similarly Iqbal et al. [Citation20] also reported an increased biological activity (antimicrobial activity) of cephalexin copper (II) complex.
Recent research on transition metal complexes suggests that the aryl-azo complexes of transition metal displayed interesting structural as well as functional properties. Moreover, beta-diketones and azo-compounds are reported as good ligands and studies on metal complexes with azo-derivatives may demonstrate important biological functions. Metal complexes of Lawsone, a napthoquinone demonstrated antibacterial activity [Citation21] and Gokhale et al. [Citation22] reported that the phenyl azo-metal complexes of Lawsone displayed antitumor properties against MCF-7. Till date no reports are available on EAM complexes; hence, an attempt on synthesis and evaluation of biological profile of both EAM and EM complexes has been made in the present study.
The present study includes the preparation, characterization and evaluation of major biological activities, namely antimicrobial, hemolytic, and antioxidant potential of embelin and its metal complexes, namely embelin–manganese, embelin–iron, embelin–cobalt, embelin– Nickel, embelin–copper and embelin–zinc. In addition, studies were further extended to EAM complexes for the above said metals for its biological activities.
2. Materials and methods
The transition metal salts were purchased from Sigma Aldrich (India). Analytical thin layer chromatography (TLC) was performed on precoated aluminum sheets of silica gel G/UV-254 of 0.2 mm thickness (Merck, Germany). Column chromatography was performed on silica gel (100–200 mesh, Merck, India). All the solvents employed were of analytical grade. The dried berries of E. ribes were collected in January 2012. Dr Anandan, Research Officer, Anna Siddha Hospital, Chennai authenticated the plant material and voucher specimen was deposited at Anna Hospital, Chennai.
2.1 Extraction of embelin
Dried berries (200 g) of E. ribes were shade dried, powdered, and extracted using a soxhlet apparatus with CHCl 3 for 48 h. The resulting extract was concentrated under reduced pressure to give a dark brown residue. The residue was purified by silica gel column chromatography using CHCl 3-methanol (9: 1 v/v) as solvent system and the resultant orange colored product was collected and stored in an airtight container at room temperature under desiccation. The structure of the solid obtained was confirmed by correlation of the spectral data as reported in the literature previously [Citation23].
2.2 Synthesis of EM complexes
The metal complexes were synthesized by stirring the methanolic solutions of embelin and metal salts in 2: 1 molar ratio for 3 h. The precipitated metal complexes were filtered washed with cold ethanol and dried in vacuum. The following schematic representation demonstrates the synthesis of embelin–cobalt complex as a representative scheme. Similar reactions were carried out for manganese, iron, cobalt, nickel, copper, and Zinc.
2.3 Synthesis of 2-(3′-methyl phenyl azo) embelin
m-Toluidine (0.185 mL, 1.7 mmol) was diazotized using sodium nitrite (0.117 g, 1.7 mmol) and 2 N HCl at 0–10°C as shown below. The diazonnium salt was then coupled with embelin (0.500 mg, 1.7 mmol) in ethanol in the presence of excess sodium acetate at 0–10°C. The precipitated orange red colored azo derivative was filtered, washed with cold water and dried under vacuum.
2.4 Synthesis of embelin-azo-metal complexes
To an ethanolic solution of azo derivative of embelin, added the metal salt solution at 2: 1 molar ratio. The pH of the mixture was maintained at 6.0 using sodium acetate. The solution was refluxed for 3 h and then cooled
overnight. The precipitate was filtered off and washed with ethanol. It was dried under vacuum to yield embelin-azo-metal (EAM) complex. The following schematic representation demonstrates the synthesis of embelin–cobalt complex as a representative scheme:
2.5 Instrumentation
Infra red spectra were obtained on a Perkin Elmer RXI HTIR spectrometer. 1HNMR and 13CNMR were recorded on a Jeol 500 nuclear magnetic resonance (NMR) spectrometer using CDCl 3 as solvent and tetramethylsilane (TMS) as an internal standard. Elemental analysis (C, H, and N) was carried out on a thermo flash EA 1112 CHN analyzer. ESI-MS (electronspray ionization mass spectrometry) was recorded on thermofinnigan LCQ advantage MAX 600 ESI. Thermogravimetric analysis was recorded on the thermogravimetric analysis (TGA) 2950 Thermogravimetric analyzer. DuPont, ultra violet visible spectroscopy (UV–visible) absorption spectra were performed using UV-2450 Shimadzu UV–visible spectrophotometer.
2.6 Biological activity
2.6.1 Antioxidant activity
The free radical scavenging activity of embelin, EM, and EAM complexes was assessed by allowing the complex solution to react with a stable free radical, 2, 2′-diphenyl-1-picrylhydrazyl radical (DPPH). The complexes were dissolved in dimethyl sulphoxide to the stock concentration of 1 g mL−1. About 1.8 mL of 0.3 mM DPPH was added to different dilutions and left for incubation at 25°C for 30–50 min. The scavenging activity was determined by measuring the absorbance at 517 nm, where DPPH alone serves as a negative control. The half-maximal inhibitory concentration (IC 50) of the antioxidant was calculated according to Amarowicz et al. [Citation24].
2.6.2 Antimicrobial activity
Two Gram-positive and Gram-negative strains were used to examine the antimicrobial activity of embelin, EM, and EAM complexes according to the standard protocol suggested by Clinical and Laboratory Standards Institute (CLSI) guidelines using the broth dilution method. Bacterial strains of Bacillus cereus (ATCC 10876), Staphylococcus aureus (ATCC 6538), Escherichia coli (ATCC 4157), and Shigella flexneri (ATCC 9199) were employed for this study. Stock cultures of the bacterial strains were maintained on nutrient agar and checked for purity and stored at 4°C. Sterile Mueller-Hinton broth media were prepared. The test compounds were dissolved in dimethyl sulfoxide (DMSO). Followed by inoculation, the required concentration of test compounds (0.25, 0.5, 0.75, 1.0, 1.25, 1.5, and 1.75 M) was added. Samples were incubated at 37°C and the turbidity of the broth was measured at 600 nm after 24 h. The percentage reduction in absorbance was calculated accordingly. Samples without test compound served as control.
2.6.3 Hemolytic assay
The hemolytic activity of metal complexes was determined using the suspension of erythrocyte cells (RBC) according to WHO guidelines (1998). Blood samples from healthy volunteers with informed consent were collected as per Institutional Ethical committee guidelines and approval (vide. No. 466/160/1999/CPCSEA), later the samples were centrifuged at 10,000 rpm for 20 min at 4°C, to remove the cell debris. Resultant pellet was washed (3–4 times) repeatedly with phosphate buffer saline (PBS; pH 7.4) to obtain RBC and was suspended in PBS containing test solutions at varying concentrations and incubated at room temperature for 10 min in the dark. At the end of incubation, tubes were centrifuged at 6000 RPM for 20 min at 4°C, in order to separate the intact cell and debris. The amount of releasing hemoglobin (Hb) in the supernatant was measured spectrophotometrically at 540 nm. The half-maximal effective concentration (ED 50) of hemolysis was then calculated.
3. Results and discussion
The extraction method followed in the present study yield concentration of embelin from E. ribes. (a) and 1(b) displays the structure of embelin and 2-(3′-methyl phenyl azo) embelin. and depicts the color, appearance, yield, percentage of constituents, spectral (UV–visible and Fourier transform infrared spectroscopy (FT-IR)), and ESI-MS results for the complexes (embelin, EM, and EAM) prepared in the present study.
Table 1. Physical and chemical characterizations of embelin and EM complexes.
Table 2. Physical and chemical characterizations of EAM complexes.
With regard to color, embelin is an orange colored solid substance, whereas the metal and azo-metal complexes are brown to gray colored solids when complexed with Mn, Fe, Co, Ni, and Cu. The Zn complexes are purple in color. The yield of the complexes showed significant variations between 45% and 75% irrespective of the metal or azo-metals. ESI-MS of the complexes is in the range of 760–773 m/z, and it was in the range of 960–1000 m/z for the azo-metal complexes.
3.1 UV–visible spectral details
(a) and 2(b) demonstrates the UV–visible spectrum of embelin, EM, and EAM complexes in methanol and showed an intense quinonoid absorption band at 289 nm. The metal complexes of embelin showed a similar absorption band between 270 and 285 nm and the observed hypsochromic shift was due to complexation. Another broad and weak band in the range of 348–405 nm observed was due to n→π* transition. The azo-derivatives of embelin showed an intense band at 251, 305, and 425 nm. Upon complexation, the bands undergo a bathochromic shift due to extended conjugation.
3.2 FT-IR spectral details
(a) and 3(b) depicts embelin with prominent peaks at 3309 cm−1 (-OH stretch), 2925 cm−1 (-CH stretch), 1612 cm−1 (C9O) and 1326 cm−1 (C–O stretch) in the IR spectrum. Upon complexation with the metal ions, in the case of [Zn (Emb)2(H2O)2] H2O, the carbonyl stretching frequency shifted to 1527 cm−1. The complex exhibit two bands at 1372 and 1229 cm−1 due to C–O stretch of enolic –OH groups at 2 and 5 positions, respectively, and suggested that embilinate anion acts as a bidentate ligand and involved in complexation with the divalent metal ion through its enolic and quinonic oxygen atoms located at positions 2 and 1. Similar observations were made with other metal complexes and azo-metal complexes.
Figure 3. (a) FT-IR spectrum of EM complex. [I. Embelin; II. Embelin–manganese; III. Embelin–iron; IV. Embelin–cobalt; V. Embelin–nickel; (VI) Embelin–copper; VII. Embelin–zinc]. (b) FT-IR spectrum of EAM complex. [(I) 2-(3′-methyl phenyl azo) Embelin; (II) Embelin-azo-manganese; (III) Embelin-azo-iron; (IV) Embelin-azo-cobalt; (V) Embelin-azo-nickel; (VI) Embelin-azo-copper; (VII) Embelin-azo-zinc].
![Figure 3. (a) FT-IR spectrum of EM complex. [I. Embelin; II. Embelin–manganese; III. Embelin–iron; IV. Embelin–cobalt; V. Embelin–nickel; (VI) Embelin–copper; VII. Embelin–zinc]. (b) FT-IR spectrum of EAM complex. [(I) 2-(3′-methyl phenyl azo) Embelin; (II) Embelin-azo-manganese; (III) Embelin-azo-iron; (IV) Embelin-azo-cobalt; (V) Embelin-azo-nickel; (VI) Embelin-azo-copper; (VII) Embelin-azo-zinc].](/cms/asset/995a64b3-0c8b-4e4d-93e8-a127fd0d7194/tcme_a_886963_f0003_b.gif)
The IR spectra of 2-(3′-methyl phenyl azo) embelin showed peaks at 3447 cm−1(−OH stretch), 1735 cm−1, 1622 cm−1 (C9O stretch), 1522 cm−1 (N9N stretch), and 1366 cm−1 (C–N stretch), respectively. The IR spectrum of embelin-azo-manganese complex showed the same absorption bands as the ligand. The bands due to C9O stretch are retained indicating that the carbonyl groups are not involved in chelation. The N9N stretch shifted to 1507 cm−1 (Δ 15 cm−1) and C–O stretch shifted to 1110 cm−1 (Δ 45 cm−1) in metal complexes. It has been observed that embelin-azo derivative chelated to the Mn (II) ion via the α nitrogen of C–N linkage and 3-OH.
3.3 Thermogravimetric analysis
Thermogravimetric analysis of the synthesized compounds ((a) and 4(b)) in addition to the parent compound, embelin was performed under nitrogen atmosphere with a heating rate of 10°C min−1. TGA of [Zn (Emb)2(H2O)2] showed three steps of decomposition. The first weight loss of 2.06% at the temperature range of 95–98°C (calcd 2.14%) accounted for the loss of one lattice water molecule. The second weight loss of 4.36% (calcd 4.37%) occurs at 162–186°C corresponds to the release of two coordinated water molecules. The complex starts decomposing at 313°C and ends at 415°C accounting for 65.17% weight loss. The residual weight corresponds to ZnO (obsd 9.63%, calcd 9.45%). On the basis of the above experimental results the most probable structure of EM complex may be assigned as [M(Emb)2(H2O)2]H2O, where M refers to the metal ions studied.
Figure 4. Thermogravimetric analysis of (a) embelin–zinc complex and (b) embelin-azo-manganese complex.
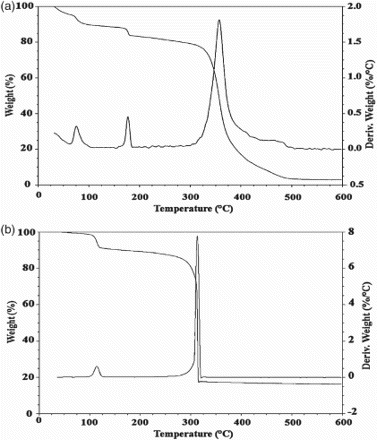
TGA of embelin-azo-manganese complex showed two-step decomposition. The first step decomposition (obsd 3.57%, calcd 3.75%) occurs at the temperature range of 78–124°C corresponds to the loss of two coordinated water molecules. A sharp decomposition of the organic component starts from 188°C to 402°C. The remaining residue corresponds to the formation of MnO (obsd 7.99%, calcd 7.39%). Hence, the proposed general formula for EAM complexes as [M(Emb-Azo)2(H2O)2], where M refers to the metal ions. Thus, considering all the above structural analysis data, the predicted structure of EM and EAM complexes is illustrated in (a) and 5(b).
3.4 Biological activities
3.4.1 Antioxidant activity
In general, DPPH radical scavenging activity is a commonly employed assay in antioxidant studies of extracts across a short time scale and has the advantage of being unaffected by certain side reactions, such as metal ion chelation and enzyme inhibition unlike other hydroxyl radical and super oxide anion [Citation25]. Furthermore, DPPH assay is known to give reliable information concerning the antioxidant ability of the tested compounds. To neutralize a free radical, an electron donating reducing agent can able to donate an electron to a free radical and the reduced species acquires proton from solution. In the present study, the antioxidant profile of embelin, EM, and EAM complexes were assessed using DPPH scavenging activity.
illustrates the antioxidant profile of embelin, EM, and EAM complexes. Embelin alone displayed IC 50 of 27±1 μ g mL−1 with reference to DPPH. The maximum antioxidant activity exhibited by embelin alone could be attributed to the presence of quinone and hydroxyl moiety [Citation26]. Upon complexation with transition metals such as Mn, Fe, Co, Ni, Cu, and Zn, a decrease in scavenging behavior was observed. But, within the metal complexes, the maximum scavenging activity was observed in the order of ((a)). This could be reasoned to the change in oxidation states in most of the transition metal ions, leading to instability in the d 10 configuration, which makes the metal to scavenge the free radicals. Furthermore, the reduction in scavenging activity with embelin-transition metal complexes could be attributed to the absence of hydroxyl and quinone moieties because of chelation with metals [Citation27].
Figure 7. (a–f) A comparative analysis of biological profile (antioxidant, antimicrobial, hemolytic) of embelin, EM and EAM complexes. (a) Antioxidant profile of embelin and EM (b) antioxidant profile of embelin and EAM; (c) antibacterial profile of embelin and EM; (d) antibacterial of embelin and EAM; (e) heamolytic profile of embelin and EM; (f) heamolytic profile of embelin and EAM.
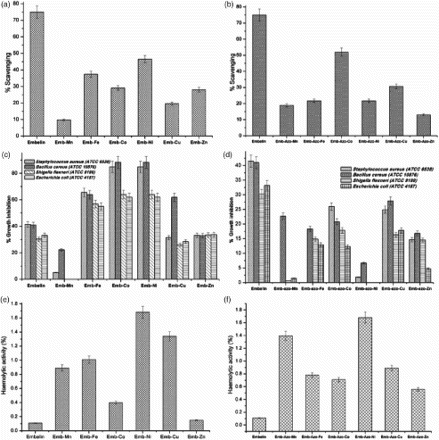
Table 3. Antioxidant profile of embelin, EM, EAM complexes in comparison with standard antioxidant ascorbic acid measured in terms of IC50 (μ g mL−1).
Compared with EM complexes, a significant increase in antioxidant activity was observed with EAM complexes especially for the metals Mn, Co, and Cu and could be reasoned to the involvement of azo-group in chelation ((b)), by which the benzene ring comes close to one of the ketone group of the embelin and forms an enol, thus facilitating the scavenging activity. The scavenging activity of EAM follows the order of as shown in (b).
3.4.2 Antimicrobial activity
(a) and 6(b) demonstrates antimicrobial profile of the chosen compounds embelin, EM, and EAM complexes. Embelin alone displayed 45% inhibition over Gram-positive bacterium and 34% growth inhibition over the Gram-negative bacterium. Similar to antioxidant profile, the antimicrobial activity displayed by embelin could also be reasoned to the presence of quinone moiety. Chitra et al. [Citation29] reported antibacterial activity of embelin alone and suggested embelin showed bacteriostatic activity toward Gram-positive organisms and bactericidal toward Gram-negative organisms. Similar observations made by Radhakrishnan et al. [Citation30]. Stern et al. [Citation27] reported, quinone moieties complexes irreversibly with nucleophilic amino acids and inactivate the proteins. The authors also suggested that the probable targets in the microbial cell are adhesions, cell wall polypeptides and membrane bound enzymes.
When embelin complexes with chosen transition metals, the metal complexes displayed a pronounced increase in percentage of growth inhibition, say inhibition in growth was observed with embelin–cobalt complexes followed by embelin–nickel and embelin–iron and the least activity shown by embelin–manganese complexes. Furthermore, within the group of organisms selected, the pronounced growth inhibition was with Gram-positive organisms compared with Gram-negative organisms ((c)). In general, the lipid bilayer is the major permeability barrier for all the molecules that pass in and out of the cell wall [Citation31]. The cell wall of Gram-negative bacteria contains lipopolysaccharide (LPS) as the outer leaflet of the outer membrane bilayer. The LPS containing asymmetric bilayer a highly ordered quasi crystalline structure with tight packing of saturated fatty acids in the inner lipid bilayer which reduces the fluidity [Citation32], and serves as an efficient barrier against rapid penetration by these lipophilic antibiotics and chemotherapeutic [Citation33]. Lambert [Citation34] proved that the Gram-positive bacteria though surrounded by a thick peptidoglycan layer, but not restrict the penetration of small molecules such as antimicrobials, which explains the elevated activity of the metal complex over Gram-positive bacteria than Gram-negative bacterial species.
A significant increase in antimicrobial activity with metal complexes can also be explained on the basis of Overtone's concept and chelation theory as reported by Hunoor et al. [Citation35]. According to Overtone's concept of cell permeability, lipid solubility is an important factor that controls antimicrobial activity, where the lipid membrane that surrounds the cell favors the passage of only lipid soluble materials. On chelation, the polarity of metal ion is reduced to a greater extent due to the overlap of the ligand orbital and partial sharing of the positive charge of the metal ion with donor groups. Furthermore, it increases the delocalization of π -electrons over the whole chelate ring and enhances the lipophilicity of the complex [Citation36]. The increased lipophilicities of complexes permit easy penetration into lipid membranes of organisms and facilitates as blockage of metal binding sites in enzymes [Citation37].
With regard to EAM complexes, we observed only a minimum percentage of growth inhibition irrespective of the organism's nature compared with embelin and EM complexes and the maximum 30% inhibition in growth by embelin-azo-cobalt and embelin-azo-copper complexes ((d)). The reduction in antibacterial activity observed with EAM complexes may be due to the poor solubility of the complexes and the reduction of functional hydroxyl groups upon chelating with metal ion, as well as the unavailability of the keto group because of keto–enol tautomerism [Citation38]. Similar observations with napthoquinone were reported by Bogdanov et al. [Citation39], where the authors observed a decrease in antibacterial activity of the complex isoazolyl naphthoquinones due to the change in position of the keto group.
Furthermore, antimicrobial activity of metal salts used for the preparation of the complexes was also studied for the said concentrations used for preparation of the complexes [Citation40].
3.4.3 Hemolytic activity
reveals the hemolytic behavior of embelin, EM, and EAM complexes assessed using human RBCs. It has been observed that no hemolytic activity was with the test compounds. In general, the cytotoxicity of quinones and transition metals (at certain concentrations) are well known [Citation41]. Embelin, being a member of that family, however, displayed non-hemolytic activity. The percentage hemolysis studied for EM and EAM was observed at concentrations >150μ g mL−1 and follows the order of ((e)) and for the EAM complexes it was in the order of
((f)). It has been expected that, upon complexation with metals, the hemolytic behavior of embelin may get changed. But results reveal that no hemolytic activity has been realized at the concentrations of >150 μ g mL−1 for both EM and EAM complexes and it was insignificant.
Table 4. Hemolytic profile of embelin, EM, and EAM observed and expressed as ED 50 (μg mL−1) in comparison with SDS.
4. Conclusions
In the present study, an attempt was made on to synthesize, characterize, and assesses the biological profile of the metal complexes of embelin, a natural benzoquionone. Only six transition metals were chosen for the study and the corresponding metal complexes were synthesized. In addition, the synthesis of embelin-azo complex was made. All the said complexes were characterized using instrumental techniques and confirmed the complexation and the presence of metals. Furthermore, these complexes were subjected to antioxidant, antimicrobial, and hemolytic activities for biological profiling. The antioxidant activity of embelin reduced significantly when it complexes with other metals or azo-metals. However, few metal complexes displayed a significant increase in antimicrobial activity compared with embelin alone. Hemolytic activity of embelin and its complexes were observed as meager. The present study concludes that upon complexation the antimicrobial activity of embelin increased significantly.
REFERENCES
- P.R. Dandawate, A.C. Vyas, S.B. Padhye, M.W. Singh, J.B. Baruah. Mini Rev Med Chem., 10, 436 (2010). doi: 10.2174/138955710791330909
- M.J.A. Martínez, P. Bermejo Benito. Stud. Nat. Prod. Chem., 30, 303 (2005). doi: 10.1016/S1572-5995(05)80036-5
- O.P. Gupta, K.K. Anand, B.J. Ghatak, C.K. Atal, M. Ali. Indian J. Exp. Biol., 14, 356 (1976).
- M. Chitra, E. Sukumar, V. Suja, C.S.S. Devi. Chemotherapy, 40, 109 (1994). doi: 10.1159/000239181
- N. Radhakrishnan, M. Alam. Indian J. Exp. Biol., 13, 70 (1975).
- M.D. Siegelin, T. Gaiser, Y. Siegelin. Neurochem. Int., 55, 423 (2009). doi: 10.1016/j.neuint.2009.04.011
- P.T. Deshmukh, V.B. Gupta. J. Asian Nat. Prod. Res., 15, 158 (2013). doi: 10.1080/10286020.2012.758634
- N. Radhakrishnan, A. Gnanamani, N.R. Prasad, A.B. Mandal. Int. J. Radiat. Biol., 88, 575 (2011). doi: 10.3109/09553002.2012.697644
- R. Joshi, J.P. Kamat, T. Mukherjee. Chem. Biol. Interact., 167, 125 (2007). doi: 10.1016/j.cbi.2007.02.004
- M.L. Dhar, O. Singh. Inorg. Chim. Acta., 117, 187 (1986). doi: 10.1016/S0020-1693(00)82198-2
- K.K. Abdul Rashid, J. Chacko, P.N.K. Nambisan. Inorg. Chim. Acta., 151, 1 (1988). doi: 10.1016/S0020-1693(00)83472-6
- R. Abraham, K.K.M. Yusuff. J. Mol. Catal. A Chem., 198, 175 (2003). doi: 10.1016/S1381-1169(02)00687-8
- J.K. Cherutoi, L.L. Cheruiyot. B. Chem. Soc. Ethiopia. 19, 295 (2005).
- V.U. Rani, G. Jyothi, G.N. Rao, B.B.V. Sailaja. Acta. Chem. Slov., 57, 916 (2010).
- V.U. Rani, M.S.P. Rao, D.V. Rao, B.B.V. Sailaja. Pharm. Chem., 2, 89 (2010).
- I. Chopra, P.M. Hawkey, M. Hinton, J. Antimicrob. Chemother., 29, 245 (1992).
- S. Lecomte, M.H. Baron, M.T. Chenon, C. Coupry, N.J. Moreau. Antimicrob. Agents Chemother., 38, 2810 (1994). doi: 10.1128/AAC.38.12.2810
- S.C. Wallis, B.G. Charles, L.R. Gahan, L.J. Filippich, M.G. Bredhauer, P.A. Duckworth. J. Pharm. Sci., 85, 803 (1996). doi: 10.1021/js960087f
- M.N. Bakola-Christianopoulou, L.B. Ecateriniadou, K.J. Sarris. Eur. J. Med. Chem., 21, 385 (1986).
- M.S. Iqbal, A.R. Ahmad, M. Sabir, S.M. Asad. J. Pharm. Pharmacol., 51, 371 (1999). doi: 10.1211/0022357991772556
- N.P. Amanda, C.C. Barbosa, S.J. Greco, M.D. Vargas, L.C. Visentin, C.B. Pinheiro, A.S. Mangrich, J.P. Barbosae, G.L. da Costae. J. Braz. Chem. Soc., 20, 712 (2009). doi: 10.1590/S0103-50532009000400015
- N. Gokhale, S. Padhye, C. Newton, R. Pritchard. Met.-Based Drugs, 7, 121 (2000). doi: 10.1155/MBD.2000.121
- C.B. Rao, V. Venkateswarlu. Tetrahedron, 20, 155 (1964). doi: 10.1016/S0040-4020(01)98407-8
- R. Amarowicz, R.B. Pegg, P. Rahimi-Moghaddam, B. Barl, J.A. Weil. Food Chem., 84, 551 (2004). doi: 10.1016/S0308-8146(03)00278-4
- K. Thaipong, U. Boonprakob, K. Crosby, L. Cisneros-Zevallos, D. Hawkins Byrne. J. Food. Compos. Anal., 19, 669 (2006). doi: 10.1016/j.jfca.2006.01.003
- I.F.F. Benzie, J.J. Strain. Methods in Enzymol., 299, 15 (1999). doi: 10.1016/S0076-6879(99)99005-5
- J.L. Stern, A.E. Hagerman, P.D. Steinberg, P.K. Mason. J. Chem. Ecol., 22, 1877 (1996). doi: 10.1007/BF02028510
- S. Mahendran, S. Badami, S. Ravi, B.S. Thippeswamy, V.P. Veerapur. Chem. Pharm. Bull., 59, 913 (2011). doi: 10.1248/cpb.59.913
- M. Chitra, S. Devi, E. Sukumar. Fitoterapia, 74, 401 (2003). doi: 10.1016/S0367-326X(03)00066-2
- N. Radhakrishnan, A. Gnanamani, A.B. Mandal. Biol. Med., 3, 1 (2011).
- P. Draper. Front. Bio. Sci., 3, D1253 (1998).
- M. Vaara, M. Nurminen. Antimicrob. Agents Chemother., 43, 1459 (1999).
- S.A. Chartrand, K.J. Thompson, C.C. Sanders. Semin. Pediatr. Infect. Dis., 7, 187 (1996). doi: 10.1016/S1045-1870(96)80007-0
- P.A. Lambert. J. App. Microbiol., 92, 46S (2002). doi: 10.1046/j.1365-2672.92.5s1.7.x
- R.S. Hunoor, B.R. Patil, D.S. Badiger, R.S. Vadavi, K.B. Gudasi. Pharm. Chem., 2, 116 (2010).
- P.G. Sushama, A.M. Dorothy, M. Alaudeen. Asian J. Chem., 19, 3403 (2007).
- Z.N. Coleska, L. Xu, Z. Hu, Y. Tomita, P. Li, P.P. Roller, R. Wang. J. Med. Chem., 47, 2430 (2004). doi: 10.1021/jm030420+
- A.T. Dinkova-Kostova, P. Talalay. Carcinogenesis, 20, 911 (1999). doi: 10.1093/carcin/20.5.911
- P.M. Bogdanov, I. Albesa, N.R. Sperandeo, B.M. Martanez. Rev. Argent. Microbiol., 25, 119 (1993).
- D. Petra, Z. Tatjana, P. Boris, J. Roman, K. Uwe, P. Andreja, T. Iztok. J. Inorg. Biochem., 99, 432 (2005). doi: 10.1016/j.jinorgbio.2004.10.018
- J.L. Bolton, M.A. Trush, T.M. Penning, G. Dryhurst, T.J. Monks. Chem. Res. Toxicol., 13, 135 (2000). doi: 10.1021/tx9902082