Abstract
A new series of transition metal complexes of Co(II), Ni(II), Cu(II), Zn(II), Cd(II) and Hg(II), with a tridentate (oxygen nitrogen nitrogen) ligand 3,5-dimethyl-N′-[(1E)-1′-pyridin-2′-ylethylidene]-1H-indole-2-carbohydrazide (L) have been synthesized and characterized on the basis of analytical, magnetic susceptibility, infra red, nuclear magnetic resonance, fast atom bombardment-mass, electron paramagnetic resonance spectral studies, X-ray powder diffraction and UV-visible spectral data. Based on analytical and molar conductance data, the complexes may be formulated as [M(L)2]·(H2O)n, where M˭ Co, Cu and Ni and [M(L)Cl2].(H2O)n, where M=Zn, Cd and Hg. The spectral studies revealed that, an octahedral geometry for Co(II), Ni(II) and Cu(II) complexes, whereas square pyramidal geometry for Zn(II), Cd(II) and Hg(II) complexes. The ligand and its Co(II) complex have been characterized by X-ray powder diffraction studies. The ligand and its complexes were tested for their antibacterial activity against Escherichia coli & Staphyloccocus aureus and antifungal activity against Aspergillus niger & Candida albicans.
1. Introduction
Indole nucleus and its derivatives occupied a unique place in chemistry of nitrogen heterocyclic compounds, because of their varied biodynamic properties [Citation1]. The indole nucleus is present in a number of physiologically significant compounds like serotonin, tryptophan, indole-3-acetic acid, gramine, reserpine, lysergic acid diethylamide and also in important antibiotics like mitomycin and gliotoxin [Citation1]. The pyridine derivatives like, 2-acetylpyridine, 2-benzoylpyridine- and 2-formylpyridine thiosemicarbazones and their metal complexes have shown substantial in vitro activity against various human tumor lines [Citation2]. Their Cu(II) complexes possesses good activity against animal and human tumor strains [Citation3]. The Zn(II), Cd(II) and Hg(II) complexes of the ligands 2-acetylpyridine-4-N-ethylthiosemicarbazone and 2-acetylpyridine-N-oxide-4-N-ethyl thiosemicarbazone have been synthesized and tested for their antifungal activity against Aspergillus niger. Both the ligands and their Zn(II) complexes have exhibited good antifungal activity [Citation4]. The 2-acetylpyridine-4-N-methylthiosemicarbazone ligand and its Zn(II), Cd(II), Pd(II) and Pt(II) complexes were tested for their antifungal activity against pathogens A. niger and Paecilomyces variotii using the standard drug Nystatin [Citation5]. Elena et al. [Citation4] reported on the synthesis and varied biological studies of 2-acetylpyridine thiosemicarbazone ligands and their metal complexes. Published reports are sparse on the arylhydrazones of 2-acetylpyridine, containing heterocyclic moiety, which enhances the inhibitory action of the molecule.
As a part of our continuing efforts to synthesize and characterize transition metal chelates using Schiff base ligand [Citation6–9]. We now report the synthesis, characterization and biological activities of transition metal complexes containing Schiff base derived from 2-acetylpyridine and 3,5-dimethylindole-2-carbohydrazide. This ligand system coordinates with the metal ion in a tridentate manner through the carbonyl oxygen, azomethine nitrogen and pyridine ring nitrogen.
2. Experimental
All the chemicals are of analytical reagent grade. Solvents were dried and distilled before use according to standard methods [Citation10]. The precursor 3,5-dimethylindole-2-carbohydrazide was prepared by standard method [Citation11]. The metal chlorides were used in their hydrated form.
2.1 Synthesis of the ligand
An equimolar mixture of 3,5-dimethylindole-2- carbohydrazide (1.0 mmol) and 2-acetylpyridine (1.0 mmol) in ethanol (30 mL) were refluxed in the presence of catalytic amount of glacial acetic acid (1–2 drops) for about 6 h on a water bath. The reaction mixture was cooled to room temperature, the separated Schiff base was collected by filtration, washed with hot ethanol (10 mL) dried and recrystallized from 1,4 dioxan. Yield of the Ligand: 80% m.p: 258°C. Proposed molecular formula: C18H18N4O (FW=306): Calcd: C, 70.57%; H, 5.92%; N, 18.29 %. Found: C, 70.52%; H, 5.89%; N, 18.26%. The structure of the Schiff base (L) is given in .
2.2 Synthesis of the complexes
2.2.1 Synthesis of cobalt(II), nickel(II) and copper(II) complexes
The metal complexes were prepared by adding stoichiometric quantities to a hot methanolic solution (30 mL) containing 1.0 mmol of ligand and methanolic solution (20 mL) of a metal chlorides (hydrated) (1.0 mmol). The mixtures were refluxed on a water bath for 6 h. The resulting solid complexes were collected by filtration and washed with 4–5 mL of hot methanol and dried in a vacuum over anhydrous calcium chloride in a desiccator (yield 65–80%).
2.2.2 Synthesis of zinc(II), cadmium(II) and mercury(II) complexes
The metal complexes were prepared by adding stoichiometric quantities to a hot methanolic solution (30 mL) containing 1.0 mmol of ligand and methanolic solution (20 mL) of a metal chlorides (hydrated) (1.0 mmol). The mixtures were refluxed on a water bath for 8 h. The resulting solid complexes were collected by filtration and washed with 4–5 mL of hot methanol and dried in a vacuum over anhydrous calcium chloride in a desiccator (yield 65–80%).
2.3 Physical measurements
Infra red (IR) spectra of the synthesized compounds were recorded as KBr pellets on PERKIN-ELMER Spectrum One FT-IR spectrometer. 1H nuclear magnetic resonance (NMR) spectra were recorded on a Bruker Avance 400 MHz spectrometer. UV-VIS spectra of the complexes were recorded on Elico-SL 164 double beam spectrometer in the range 200–1200 nm in dimethylformamide (DMF) solution ( M). Fast atom bombardment (FAB)-mass spectrum of the ligand was obtained on JEOL SX 102/DA-6000 mass spectrometer using Argon/Xenon as the gas. The accelerating voltage was 10 kV and the spectrum was recorded at room temperature using meta-dinitrobenzyl alcohol as a matrix. Elemental analyses were obtained from HERAEUS C, H, N‒ O rapid analyzer and metal analyses were carried out by following the standard methods. Electron paramagnetic resonance (EPR) measurements were carried out on a Bruker Biospin Gmbh spectrometer working at a microwave frequency of 9.4 GHz using DPPH as a reference with the field set at 3200 Gauss.
2.4 Antimicrobial activity
The antibacterial and antifungal activities were performed by the cup-plate method [Citation7]. In a typical procedure, molten agar kept at 45°C was then poured into Petri dishes and allowed to solidify. Holes of 8 mm diameter were punched using a sterile cork borer and these were completely filled with test solutions (1 mgmL−1 in DMF). The plates were incubated for 24 h at 37°C. The diameters of the zones of inhibition for all test compounds were measured and the results compared with streptomycin of the same concentration as that of the test compound under identical conditions.
Antifungal activity of the compounds was evaluated against the A. niger and Candida albicans by the cup-plate method, cultured on potato-dextrose agar medium adapting similar procedure as described above. The plates were incubated at 37°C for 48 h. The diameters of the zone of inhibition for all the test compounds were measured and the results compared with the standard drug griseofulvin of the same concentration under identical conditions.
3. Results and discussion
The Schiff base is soluble in DMF, dimethyl sulfoxide (DMSO) and insoluble in common organic solvents. The purity of the ligand was checked by elemental analysis, FT-IR, 1HNMR and FAB-mass. The physical characteristics of the Schiff base and its metal complexes are given in . The metal complexes are soluble in DMF and DMSO and insoluble in acetone, chloroform, ethanol, methanol and water. The elemental analysis data of the Schiff base and its metal complexes are consistent with the calculated results from the empirical formula ( and ). The observed molar conductance of the complexes in DMF for 10−3 M solutions at room temperature is consistent with the non-electrolytic nature of the complexes [Citation12].
Figure 1. Antimicrobial screening data of the ligand HL (ligand containing one dissociable hydrogen) and its complexes.
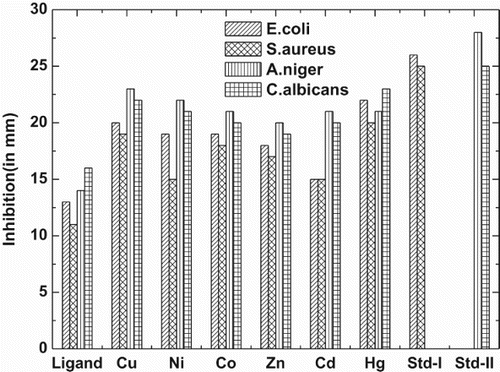
Figure 2. Suggested geometries of the complexes, where M=Co (II) Ni(II) and Cu(II) and n=1 for Co(II), Ni(II) and Cu(II).
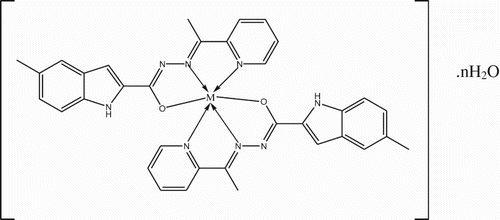
Table 1. Elemental analysis, color, yield, and molar conductance of the Schiff base and its metal complexes.
3.1 IR spectra
The important IR frequencies exhibited by the ligand and their Co(II), Ni(II), Cu(II), Zn(II), Cd(II) and Hg(II) complexes are given in along with their assignments. The IR spectrum of ligand showed a strong intensity band at 3265 and 3083 cm−1 attributable to indole ring νNH, and νNH of amide group, respectively. In the case of IR spectra of Cu(II), Co(II) and Ni(II) complexes, the band due to amide NH has disappeared, absence of this band due to νNH, indicates enolization of the amide carbonyl function during complexation and subsequent coordination of the amide carbonyl oxygen via deprotonation [Citation13]. The band due the νNH of indole ring [Citation14,Citation15] observed in the region 3330–3210 cm−1 in the above complexes, indicating its non-involvement in the complexation. The band appeared at 1649 cm−1 in the ligand due to has been disappeared in all complexes except Zn(II), Cd(II) and Hg(II) complexes, confirm enolization of amide function during complexation which is evident by the appearance of a new band in the region 1602–1597 cm−1 in these complexes due to the formation of azine moiety, >C˭ N‒ N˭ C< further confirms the enolization of amide carbonyl function during complexation [Citation16–18]. The band due to
which was observed at 1577 cm−1 in ligand has shown a negative shift of 36–26 cm−1 in of all the complexes and appeared in the region 1551–1541 cm−1 indicating the involvement of azomethine nitrogen in the complexation with metal ions [Citation19]. The appearance of a new bands in the region 3445–3409 cm−1 for Cu(II), Ni(II), Co(II), Zn(II), Cd(II) and Hg(II) complexes, indicate the presence of coordinated or lattice water in these complexes [Citation20]. Pyridine ring vibrations which were observed at 1430, 1048, 620 cm−1 in the case of ligand have shifted to higher wave number side in all complexes and appeared in the region 1493–1464, 1070–1065 and 674–635 cm−1 indicating the involvement of pyridine ring nitrogen in the coordination [Citation21]. Appearance of a new set of bands in all the complexes, due to
and
vibrations is the direct evidence for complexation. The bands observed in the region 440–426 and 531–478 cm−1 are assigned to
and νM−N, respectively. The bands in the region 321–318 cm−1 have been assigned to νM− Cl bands in the case of Zn(II), Cd(II) and Hg(II) complexes [Citation22]. The presence of chlorine atoms in these complexes is further confirmed by quantitative chloride estimation.
Table 2. Characteristics IR bands of the Schiff Base and its complexes (in cm−1).
3.2 Electronic spectra
The electronic spectra of Co(II), Ni(II) and Cu(II) complexes were recorded in DMF solution at 10−3 molar concentration. The electronic spectrum of the Co(II) complex exhibited absorption bands 10,582, 15,625 and 20,230 cm−1 due to the ,
and
transitions, respectively [Citation23–25]. The Ni(II) complex displayed an electronic spectrum with transitions at 10,526, 16,129 and 24,390 cm−1, respectively. These bands may be assigned to the transitions
,
and
, respectively [Citation23–25]. The Cu(II) complex displayed bands at 13,157 and 18,348 cm−1, which may be assigned to the transitions
, ν1),
and
. The third band at 34,482 cm−1 may be due to charge transfer [Citation16,Citation26] ().
3.3 1H NMR spectral studies
The 1H NMR spectral data of the ligand and its complex were recorded in DMSO-d6. These 1H NMR spectrum of the ligand and complex were compared, it was observed that the signals of the protons of different functionalities of the ligand have been shifted toward the downfield region indicating the coordination of the ligand to the metal ion. The 1H NMR spectrum of the ligand showed a fine singlet at δ 2.50 s (‒ N˭ C‒ CH3) due to three protons of ‒ CH3 group on the azomethine carbon. Another two singlets appeared at δ 2.45 s (CH3) and δ 2.40 s (CH3) are due to protons of methyl groups at 3, 5-position of indole nucleus, respectively. Proton of the amide NH has resonated as a singlet at δ 11.40 s (1H), whereas proton on indole nitrogen appeared at δ 10.45 s (1H) as a distinct singlet. Three aromatic protons of the indole ring and four protons of pyridine ring have resonated as a multiplet in the region δ 7.07–8.65 m (Ar-H, 7H). In the 1H NMR spectrum of Zn(II) complex, the two singlets appeared at δ 2.50 s (CH3) and δ 2.45 s (CH3) are due to protons of methyl groups at 3, 5-position of indole nucleus. Another singlet at δ 2.59 s (CH3) is due to the protons of the methyl group on the carbon atom of azomethine function of the ligand. NH proton of the amide function and indole NH has resonated as a distinct singlet at δ 11.65 s (1H) and δ 10.60 s (1H), respectively. The seven aromatic protons have resonated in the region δ 7.46–8.75 m (Ar-H, 7H) as a multiplet ().
Table 3. 1HNMR spectral data of the Schiff base and its Zn(II) complex.
3.4 FAB-mass spectral analysis
Mass spectrum of ligand showed a molecular ion peak M+ at m/z 306 (13%), which is equivalent to its molecular weight. The molecular ion M+ by the expulsion of C7H8N3 species gave fragment ion A1 at m/z 172 (M+-C7H, 100%), which is also a base peak. The fragment ion A1 by the loss of carbon monoxide molecule gave fragment ion A2 at m/z 144
, 10%). Fragment ion A2 underwent further fragmentation by the loss methyl radical and gave fragment ion A 3 at m/z 129
. Fragment ion A3 by the loss of C2H species gave another fragment ion A4 at m/z 104
().
3.5 Magnetic susceptibilities
Magnetic susceptibilities of the complexes calculated at ambient temperature and the data presented in . The magnetic moments are in good agreement with the expected high spin configurations. The Co(II), Ni(II) and Cu(II) complexes showed magnetic moments of 4.89, 2.99 and 1.99 μB, corresponding to 3, 2 and 1 unpaired electrons respectively [Citation23,Citation27,Citation28]. The Zn(II), Cd(II) and Hg(II) complexes show a diamagnetic behavior having a d10 system which is as per expectation.
3.6 EPR spectrum
The EPR spectrum of the Cu(II) complex was recorded at 300 K as a polycrystalline sample and in acetonitrile solution, on the X-band at the frequency of 9.4 GHz under a magnetic field strength 3200 G. The polycrystalline spectrum showed a well-resolved anisotropically broad signal. The analysis of the spectrum gives g‖ 2.25 and g⊥ 2.06 (). The trend observed for the complex under study indicates that the unpaired electron is localized in the
orbital of the Cu(II) ion. The observed geometric parameter calculated by the expression
is greater than 4
evidenced the exchange interaction is negligible between metal ion in the solid complex [Citation29–31]. Based on the spectral studies, the following geometries may be suggested for the complexes ().
Table 4. Electronic spectral bands (in cm−1) of Co(II), Ni(II) and Cu(II) complexes and EPR data of Cu(II) complex.
3.7 X-ray diffraction studies
Powder X-ray diffraction was done for ligand and its cobalt complex and spectra is given in and . X-ray diffraction analysis of the ligand and its cobalt(II) complex confirm the cubic crystal system for these compounds. Ligand has unit cell dimensions a=b=c=16.59, and
has unit cell dimensions a=b=c=16.19,
[Citation32,Citation33] ( and ).
Table 5. Powder X-ray diffraction data of ligand HL.
3.8 Antimicrobial activity
A cup plate method was employed for the in vitro study of antibacterial and antifungal effect against, Staphylococcus aureus, Escherichia coli, C. albicans and A. niger. The inhibitory effect of ligand and its complexes against these organisms are given in . The antimicrobial screening data show that the metal chelates exhibit a higher inhibitory effect than the free ligand. The increased activity of the metal chelates can be explained based on the chelation theory [Citation7,Citation34–36] ().
Table 7. Antibacterial and antifungal activity of HL and its complexes.
4. Conclusions
The present study revealed octahedral geometry for the Co(II), Ni(II), Cu(II) and square pyramidal geometry for Zn(II), Cd(II) and Hg(II) complexes, respectively. The ligand acts in a tridentate manner coordinating through carbonyl oxygen, azomethine-N and pyridine ring-N groups in an oxygen nitrogen nitrogen fashion. Moreover, the microbial data revealed that the complexes were superior to the free ligand in the inhibition of the tested bacteria and fungi. It is proposed that concentration plays a vital role in increasing the degree of inhibition the activity increased with increasing concentration of the complexes ( and ).
Supplementary Material
Download MS Word (1.6 MB)The authors are thankful to the Chairman, Department of Chemistry, Gulbarga University, Gulbarga for providing laboratory facilities. They also thank IISc Bangalore, STIC Kochi and CDRI Lucknow for 1HNMR, ESR, Mass, FAB-Mass, XRD and elemental data.
REFERENCES
- A. Bayer. Chem. Ber., 13, 2254 (1880). doi: 10.1002/cber.188001302243
- E. Liberta, D.X. West. Biometals, 5, 121 (1992). doi: 10.1007/BF01062223
- (a) M.C. Millar, C.N. Stineman, J.R. Vance, D.X. West, I.H. Hall. Anticancer Res., 18, 4131 (1998); (b) M.C. Millar, C.N. Stineman, J.R. Vance, D.X. West, I.H. Hall. Appl. Organomet. Chem., 13, 9 (1999).
- B. Elena, C. Rosa, C. Alfonso, D. Ricarso, M.C. Maichle, S. Joachin, X.W. Douglas. Polyhedron, 18, 3695–3702 (1999). doi: 10.1016/S0277-5387(99)00309-5
- B. Elena, C. Rosa, C. Alfonso, D. Ricarso, L.E. Authorny, M.-M. Cacilia, M.S. Michelle, X.W. Douglas. Eur. J. Inorg. Chem., 1999, 965–973 (1999).
- B.H.M. Mruthyunjayaswamy, Y. Jadegoud, B.I. Omkar, G.P. somanath, M.K. Shreeshaila. Trans. Met. Chem., 30, 234–242 (2005). doi: 10.1007/s11243-004-2997-z
- B.H.M. Mruthyunjayaswamy, B.I. Omkar, Y. Jadegoud. J. Braz. Chem. Soc., 16, 783–789 (2005). doi: 10.1590/S0103-50532005000500016
- F. Rahaman, B. Hiremath, S.M. Basavarajaiah, B.H.M. Jayakumarswamy, B.H.M. Mruthyunjayaswamy. J. Indian. Chem. Soc., 85, 381–386 (2008).
- F. Rahaman, O.B. Ijare, Y. Jadegoud, B.H.M. Mruthyunjayaswamy. JCC, 62, 1457–1467 (2009).
- A.I. Vogel. A Text Book of Quantitative Organic Analysis, 3rd Edn, ELBS Longmans Green and Co. Ltd., London (1962).
- S.P. Hiremath, P.S. Badami, M.G. Purohit. Indian J. Chem., 24B, 1115 (1985).
- W.J. Geary. Coord. Chem. Rev., 7, 81 (1971). doi: 10.1016/S0010-8545(00)80009-0
- N.K. Singh, S.B. Singh. Indian J. Chem., 40A, 1071–1075 (2001).
- P.H. Shivayogi, B.H.M. Mruthyunjayaswamy, G.P. Muralidhar. Indian J. Chem., 16B, 789–792 (1978).
- J.S. Biradar, B. Sharanbasappa. Green Chem. Lett. Rev., 2, 237–241 (2009). doi: 10.1080/17518250903393890
- A.V. Lakshmi, N.R. Sangeetha, S. Pal. Indian J. Chem., 36, 844–848 (1997).
- E.R. Blout, M. Fields, R. Karplus. J. Am. Chem. Soc., 70, 194 (1948). doi: 10.1021/ja01181a056
- R.S. Baligar, V.K. Revankar. J. Serb. Chem. Soc., 71, 1301–1310, JSCS–352 (2006). doi: 10.2298/JSC0612301B
- A. Koji, M. Kanako, O. Masaki, O. Hishashi. Inorg. Chem., 41, 4461 (2002). doi: 10.1021/ic020002f
- Rajesh Malhotra, Sudhir Kumar, Jyoti, Hari Ram Singal, Kuldip Singh Dhindsa. Indian J. Chem., 39A, 421 (2000).
- W.E. Marsh, D. Eggleston, W.E. Hall Field, D.J. Hodgson. Inorg. Chem. Acta, 70(2), 137 (1983). doi: 10.1016/S0020-1693(00)82792-9
- B.B. Mohapatra, S.K. Saraf. J. Indian Chem. Soc., 80, 696 (2003).
- F.A. Cotton, G. Wilkinson. Advanced Inorganic Chemistry. 3rd Edn, Wiley Eastern Pvt. Ltd., New Delhi (1972).
- (a) I.M. Procter, R.J. Hathaway, P. Nicholls, J. Chem. Soc. A, 1678–1684 (1968); (b) K.G. Kocwin, W. Wajciechowski, Trans. Met. Chem., 21, 312 (1996).
- (a) K.C. Satpathy, B.B. Jal, R. Mishra, Trans. Met. Chem., 9, 8 (1984); (b) A.C. Fabretti, G. Peyrond, G.C. Franchini. Inorg. Chem. Acta, 35, 49 (1979).
- A.B.P. Lever. Inorganic Electronic Spectroscopy. 2nd Edn, pp. 487, Elsevier, Amsterdam (1984).
- (a) S. Chandra, K. Gupta. Indian J. Chem., 40A, 775 (2001); (b) S. Chandra, A. Gautam, M. Tyagi. Trans. Met. Chem. 32, 1079 (2007).
- R.K. Agarwal, Kisher Arora, Prashant Dutt. Polyhedron, 13, 957 (1994). doi: 10.1016/S0277-5387(00)83016-8
- L.K. Mishra, Y. Jha, B.K. Sinha, R. Kant, Rajeswar Singh. J. Indian. Chem. Soc., 76, 65 (1999).
- (a) H. Hathaway, D.E. Billing. Coord. Chem. Rev., 5, 143–207 (1970); (b) R.J. Dudley, B.J. Hathway. J. Chem. Soc., Sec. A, 1725 (1970); (c) S. Chandra, K. Gupta. Trans. Metal Chem., 27, 196–197 (2002); (d) K. Burger, Coordination Chemistry Experimental Methods, 1st Edn, Butterworth and Co. Ltd., London. (1973).
- M.F. Fouda, M.M. Abd-Elzaher, M.M. Shadofa, F.A. El Saied, M.I. Ayad, A.S. El Tabl. Trans. Metal Chem., 33, 219–228 (2008). doi: 10.1007/s11243-007-9024-0
- B.D. Cullity. Elements of X-Ray Diffraction, Addison-Wesley Pub. Co., Notre dame, IN (1978).
- (a) N.F.M. Henry, H. Lipson, W.A. Wooster. Interpretation of X-Ray Diffraction Photography, p. 179, Macmillon, London (1959); (b) M.J. Burger. X-Ray Crystallography, p. 100, Willey, New York (1953); (c) M.M. Woolfson. An Introduction to X-Ray Crystallography, p. 125, Cambridge University Press, Cambridge (1980).
- A.L. Barry. The Antimicrobial Susceptibility Test, Principles and Practices, 4th Edn, ELBS, London, 180–193 (1976).
- S.K. Sengupta, O.P. Pandey, B.K. Srivatsava, V.K. Sharma. Trans. Metal Chem., V-23, 349 (1998). doi: 10.1023/A:1006986131435
- S. Chandra, M. Tyagi. J. Serb. Soc., V-73, 727 (2008). doi: 10.2298/JSC0807727C