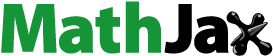
Abstract
The occlusal surface of a tooth is affected by the development of biofilm in pits and fissures as bacteria and food particles accumulate in its complex structure. In this study, miswak fibers containing cellulose and antimicrobial extract were incorporated in commercial pit and fissure sealants. The miswak powder was characterized by different analytical techniques. The powder was mixed in different ratios (0–5%) into a pit and fissure sealant to result in five sealants (Groups 0–5), and their mechanical properties i.e. flexural strength, compressive strength, and Vickers hardness were evaluated. The sealants were also evaluated against streptococcus mutans oral pathogenic bacteria. SEM analysis confirmed irregular shape and micron-size particles of miswak powder. The infrared spectral analysis and X-ray differential peaks showed characteristic peaks related to miswak fibers. The particle appearance increased in prepared pits and fissure sealants with higher loading of miswak powder in SEM analysis. The flexural strength, compressive strength, and Vickers hardness values were obtained in the range of 148–221 (±16.6: p-value < 0.001) MPa, 43.1–50.3 MPa (±1.7: p-value <0.001), and 15.2–21.26 VHN (±0.56: p-value <0.001) for control and prepared sealant specimens respectively. In the antibacterial study, the zone of inhibitions increased with increased content of miswak from 15.6 ± 0.45 mm (Group 1) to 20.3 ± 0.32 mm (Group 5). The MIC was calculated to be 0.039%. The prepared experimental sealant had acceptable mechanical and good antibacterial properties therefore it could be recommended as an efficient pit and fissure sealant.
1. Introduction
Dental caries is an opportunistic disease characterized by the continuous demineralization of tooth structure [Citation1]. Dental caries is a condition that results from complicated bio associations of fermentable carbohydrates, acid-forming bacteria, and host factors, such as teeth and saliva [Citation1]. It is the most frequently encountered disease globally and the major source of tooth loss in the population. The acidogenic bacterial species Streptococcus mutans is thought to be the primary cause of dental caries [Citation2]. Dental caries are more common in developing countries compared to economically developed countries affecting 60–90% of school-aged children and a huge quantity of elders too [Citation3]. For the past sixty years, pit and fissure sealants have been utilized in dentistry to prevent the development of such tooth cavities [Citation4]. Pit and fissure sealants are materials applied to the pits and fissures of teeth prone to caries [Citation5]. These materials provide a physical barrier to protect against bacteria and food stagnation by binding micromechanically or chemically with the tooth structure [Citation5]. However secondary caries are produced at the interface of materials and tooth due to either loss of materials or polymeric shrinkage induced microleakage [Citation6].
Various materials have been used as pit and fissure sealants including glass ionomer sealants, compomers, and resin-based sealants [Citation7]. Resin-based sealants can be classified based on viscosity, translucency, and polymerization [Citation8]. Based on viscosity, resin-based sealants are classified as filled and un-filled sealants [Citation8]. The unfilled sealants are resins without any fillers [Citation8]. The addition of fillers to the resins increases their viscosity [Citation9]. Based on translucency resin-based sealants are classified as opaque and transparent [Citation8]. Based on the method of polymerization resin-based sealants are classified as auto-polymerized and light polymerized [Citation10]. The auto-polymerized sealants, also known as self-cure, are two-component systems [Citation11]. These auto-polymerized sealants have more or less been replaced by light-polymerized sealants [Citation11].
Antibacterial agents have been added to sealants to inhibit bacterial growth that could result in dental decay [Citation12–14]. Miswak, botanically called ‘Salvadora persica’ is a species of the family of salvadoraceae. It serves as an anti-bacterial, anti-caries, and anti-peripatric disinfectant having antiplaque and antifungal properties [Citation15,Citation16]. It has also been used as an extract for the control of gingival bleeding and gingival inflammation in mouthwash [Citation17] and as an effective root canal irrigant in aqueous solution (10%) [Citation18]. Miswak sticks are commonly used for brushing teeth and for religious purposes in many countries of the Middle East. Miswak contains many functional ingredients like Salvadore, fluoride, chloride, trimethylamine, mustard oil, silica, vitamin C, sulfur, resins, saponins, and traces of sterols, tannins, and flavonoids [Citation19].
Miswak is mainly composed of cellulose, hemicelluloses, and lignin [Citation20] and contains other beneficial components like antibacterial agents, abrasives, cleansers, astringents, fluoride, and enzyme inhibitors [Citation20]. Natural fiber has recently been a field of active research for strengthening resin composite materials [Citation13]. Cellulose fibers are among the natural fibers and have drawn substantial interest due to their strong mechanical characteristics and excellent biocompatibility [Citation13]. Research has indicated that cellulose nanofiber composites have significantly improved their mechanical characteristics [Citation13].
Since the development of pit and fissure sealants, they have had shortcomings like inferior mechanical properties and limited antibacterial properties [Citation6,Citation13]. The addition of miswak fibers containing cellulosic constituents and antibacterial components might improve both the mechanical and antibacterial properties of such sealants. Thus, this study aimed to determine the effect of the addition of miswak fiber powder to a commercial pit and fissure sealant on various mechanical and anti-bacterial properties against streptococcus mutans. The null hypothesis was that miswak fiber addition to the sealant would not improve the mechanical properties irrespective of antibacterial properties.
2. Materials and methods
The stems of the olive plant (brand: Al Khair Naturals) were bought at the local market. The bark of the stems was peeled off and the stems were cut into small pieces. The small pieces were then added to a grinder (Silver crest) and turned into miswak fiber. The fibers were then milled using a vibrating miller (Fritsch). After milling, the powder was sieved and powder of 45 µm was collected.
2.1. Preparation of pit and fissure sealants
The experimental pit and fissure sealants were prepared by adding various percentages (0% to 5 wt%) of miswak powder into a commercial pit and fissure sealant (Conceal F, SDI) (). The required amount of Conceal F pit and fissure sealant was poured into dark bottles followed by miswak powder and stirred for 20 min using a magnetic stirrer. After stirring the bottles were properly labeled and stored for later use at room temperature in darkness.
Table 1. Experimental groups.
2.2. Characterization
2.2.1. Scanning electron microscopy
The morphology and surface properties of the miswak fibers were studied with the help of a Scanning Electron Microscope (JSM-IT100 InTouchScope™). The powder miswak fibers were gold coated in a gold sputter (QUORUM). For SEM analysis, images were taken in the range of 25X to 500X magnifications, and the voltage of the instrument was kept at 15kV.
2.2.2. Fourier transforms infrared spectroscopy
Fourier transforms infrared spectroscopy of miswak powder was done in Attenuated Total Reflectance (ATR) mode as an accessory of FTIR-spectrophotometer (IR Tracer 100 Shimadzu). A background scan was obtained before the test. Spectra were collected over the region 4000-400cm−1 at a resolution of 8cm−1 and averaged 256 scans.
2.2.3. X-rays diffraction
X-ray diffraction study of miswak powder was performed using an X-ray diffractometer (D8 Advance XRD Bruker) operated at 40 kV and 40 mA while employing Cu K radiation. The sensor was scanned across an angle range of 2 = 10°–70° with a step size of 0.02° and a period per step of 2 s.
2.3. Mechanical properties
2.3.1. Flexural strength
The flexural strength of the pit and fissure sealants was assessed using the procedure cited in ISO4049-2019 [Citation21]. Thirty bar-shaped specimens were made with the help of Teflon molds in the dimensions of 25mm in length, 2mm in width, and 2mm in height (n = 5). The mold was cleaned with ethanol to remove any contamination or debris. Sealant was introduced in the mold using a plastic instrument with great care. The mold was placed on a glass slab and the top and bottom surfaces were covered with a Mylar strip to prevent an oxygen inhibition layer. The curing of the specimens was done using a light-curing unit (Westcode, China) of light intensity: 1200–1500mW/cm on both sides for 40 s. The specimens were placed in deionized water at 37 °C in a drying oven (Memmert UNB-500) for 24 h. The specimens were then loaded in a universal testing machine (Walter + bai AG Switzerland) using a load cell of 10 KN and a crosshead speed of 1 mm/min. The maximum load (F) withstood by each specimen was recorded and compressive stress (S) was calculated using the following EquationEquation (1)(1)
(1) :
(1)
(1)
where S is flexural strength (MPa), F is the maximum fracture load, l is support distance in mm, b is the width of the specimen in mm, and h is the height of the specimen in mm22.
2.3.2. Compressive strength
The compressive strength of the sealants was assessed using the protocol mentioned in ISO 6874 [Citation22]. A total of 30 cylindrical specimens were made using Teflon molds in the dimensions of 6 mm in height and 4 mm in diameter (n = 5). The mold was cleaned with ethanol to remove any contamination or debris. Sealant was introduced in the mold using a plastic instrument with great care. The mold was placed on a glass slab and the top and bottom surfaces were covered with a Mylar strip to prevent an oxygen inhibition layer. A light-curing unit (Westcode, China) of light intensity: 1200–1500mW/cm was used to cure the specimens on both sides for 40 s. The specimens were placed in deionized water at 37 °C in a drying oven (Memmert UNB-500) for 24 h. The specimens were then a universal testing machine (Walter + bai AG Switzerland) with a load cell of 50 KN and a crosshead speed of 1 mm/min. The compressive load (P) of each specimen was recorded and compressive stress (σc) was calculated using the following equation:
(2)
(2)
where Ac is the cross-sectional area of cylinder [Citation22].
2.3.3. Hardness
For measuring Vickers hardness (VHN), a total of 30 cylindrical specimens of 8 mm in diameter and 2 mm in length were fabricated in brass molds (Two indentations per sample n = 5*2 = 10). Before each use the mold was cleaned with ethanol to remove any contamination or debris. The mold was then placed on a glass slab. Sealant was introduced into the mold and covered with Mylar strips. The curing of the specimens was done using a light-curing unit (Westcode China) of light intensity: 1200–500mW/cm for 40 s on both sides. The prepared specimens were placed in deionized water at 37 °C for 24 h in a drying oven (Memmert UNB-500). Vickers hardness was measured by applying a 0.49N load for 15 s according to ASTM E384-11e1. After applying the load (F), the values for diagonal indent lengths were measured. On each specimen, three indentations were produced; the predicted mean value of all three indentations was used to calculate VHN. The VHN values were calculated according to ASTM E384-11e1 by using the following formula
(3)
(3)
where HV denotes Vickers hardness number, F denotes indentation load, and do denote indentation diagonal.
3. Antibacterial properties
The bacterial culture was obtained from a known patient suffering from periodontitis who had been subjected to an obtained subculture of S mutants. S mutant’s subculture of 200 µL was swabbed on blood agar followed by well formation through a well borer of 8 mm. The specimens were kept in the well and 100 µl DMSO was added (to help in leaching of the material). After placing the specimens on inoculated plates, they were subjected to incubation for 48 h at 37 °C and under 5–10% CO2. After 48 h, the diameter of the zone of inhibition (ZOI) was measured in mm using a digital caliper. The samples were analyzed in a triplet.
3.1. Minimum inhibitory concentration (MIC)
In the MIC study, the amount of (5%) miswak fibers (showing effective antimicrobial properties when added to the sealant) was considered for analysis. 5% of the miswak was added into 1mL of DMSO. To make it a diluted solution, 500µl of 5%/1mL specimen was added into a 2nd tube followed by adding 500 µl of DMSO. For the next dilution, 500 µl of solution was taken from 2nd tube and added into the 3rd tube and diluted with 500 µl of DMSO. This procedure was followed for all 10 dilutions.
In the first well of the first row, a simple broth medium of 200 µl was added and considered as a negative control group. 200µl of 0.5 McFarland standard suspensions was added to the 12th well of the 1st row and was considered a positive control group. In the remaining 10 wells from the 2nd to the 11th, 200 µl of 0.5 McFarland suspensions was added. The 96 well plates were incubated at 37 °C for 24 h.
4. Statistical analysis
All data were entered in Microsoft Excel spreadsheet 2010 and were imported to R Package 4.1.2 for analysis. All outcome variables were compared using one-way ANOVA followed by Kruskal Wallis tests with a p-value <0.05. The Shapiro test was performed to detect the normality of data. For flexural strength p value was non-significant, data was normally distributed (parametric) and hence ANOVA was considered. For compressive strength and Vickers hardness, the data was not normally distributed (nonparametric) so Kruskal Wallis was applied instead of the ANOVA test.
5. Results
5.1. Scanning electron microscope (SEM)
SEM was performed at 100 and 500× magnifications as shown in . The miswak powder showed irregularly shaped particles of different sizes. Moreover, the particle showed a conglomerate texture. On average these fibers were less than 20 µm in one dimension.
5.2. Fourier transform infrared spectroscopy
The FTIR spectrum of miswak powder is given in . The peak around the region of 1050–870 shows C–O–C which represents compounds containing carbonyl compounds. In the region of 1275–1000, stretch represents C–H bending while the region around 1600–1430 represents C = C moieties [Citation23]. Both of these are typical for aromatic compounds. The region around 2900–2700 represents aldehyde C–H while the region around 3500–3300 represents the OH group of cellulose and phenol which is typical for compounds containing oxygen [Citation23].
5.3. X-ray diffraction (XRD)
The XRD pattern of miswak powder is given in . The XRD pattern of miswak fibers is similar to that observed for other lignocellulosic materials [Citation24]. The diffraction peaks at theta 14.1° (110), 22.4° (200), and 35° (400) are the major characteristic peaks of crystalline cellulose I [Citation25]. The other components like lignin, hemicellulose, and phenolic extract are amorphous and have not observed any characteristic peaks.
5.4. Mechanical testing
5.4.1. Flexural strength
The flexural strength results are shown in . The one-way ANOVA found the values to differ with statistical significance (p < 0.05). Higher flexural strengths were measured for groups 1 and 2 than for the control group and the experimental groups with the highest content of miswak fibers.
5.4.2. Compressive strength
The compressive strength results are shown in . The Kruskal Wallis tests found the values to differ with statistical significance (p < 0.01). Lower compressive strength was observed for sealants containing miswak fibers than for the control group, and the compressive strength decreased with increasing content of miswak fibers.
5.4.2. Vickers hardness
The Vickers hardness results are shown in . The Kruskal Wallis tests found the values to differ with statistical significance (p < 0.05). A higher Vickers hardness value was measured for the control group than for any of the groups containing miswak fibers. The sealant with the lowest fiber content (group 1) had significantly higher Vickers hardness than the three sealants with the highest miswak content.
5.5. Antibacterial properties
The results of the antibacterial studies are shown in . In the control group, no zones of bacterial inhibition against streptococcus mutants were found. Among the groups containing miswak fibers, the smallest zone of bacterial inhibition was found in group 1 and the largest in group 5.
Table 2. Results of agar well diffusion method of antibacterial property
The streaking method showed no visible colonies in the negative control group (), while in the positive control group, visible colonies of streptococcus mutans were seen.
As shown in , there was no growth up to 9 dilutions of 5% compounds. The growth was noticed on dilutions 10th and 11th. So it was concluded that the 9th dilution was the minimum inhibitory concentration of 5% compound against streptococcus mutans and the 0.039% is the MIC that inhibits the growth of S. mutans.
Table 3. Minimum inhibitory concentration of the specimens.
6. Discussion
6.1. Scanning electron microscope (SEM)
In this study, SEM was performed to study the surface characteristics of miswak powder as well as the experimental pit and fissure sealants. The SEM images of miswak powder showed irregular particles distributed irregularly in the complex structure. Besides this, they were highly porous and had varied sizes. This structure is a typical structure of cellulose; it has been shown to have highly irregular porous fibers. The results were similar to those in the study conducted by Soner in which SEM of miswak powder was performed [Citation26].
6.2. Fourier transform infrared spectroscopy (FTIR)
FTIR spectroscopy, a non-invasive method was used to analyze the powder of miswak fibers as it contains complex molecules and structural components. The spectrum was evaluated in the range of 4000–650cm−1 and the characteristic infra-red peaks related to miswak fibers [Citation27]. The active substances present in miswak are indicated by various peaks present in the graph around the region of 1050–870, 2900–2700, and 3700–3500. The hydrophilic broad peaks (O–H group) related to α-cellulose were observed in the 3400–3100 cm−1 region. The bending vibration of the C–H stretch of the hemicellulose was observed at 2870 cm−1. The peak of CH3COO related to hemicellulose was observed at 1730 cm−1. A prominent peak related to lignin was observed at 1633 of C–O bending. The peaks ranging from 1500 to 1250 cm−1 related to structural moieties were identified. These results were similar to a study conducted by Jayanti et al in which they conducted FTIR of different specimens of miswak powder and extracts [Citation28].
6.3. X-ray diffraction (XRD)
The XRD results of miswak powder showed peaks at 14.2° at 110 planes, 22.4° at 200 planes, and 35° at 400. These peaks are similar to the results of the International Centre for Diffraction Data in which ground cellulose is used as cellulose reference [Citation29]. This result was also similar to results by Stubicar et al. in which XRD peaks in the same region were observed when XRD of microcrystalline cellulose powder was performed [Citation30]. French also observed peaks at the same point while analyzing the XRD of cellulose polymorphs [Citation31].
6.4. Mechanical properties
Microcrystalline Cellulose microfibers (MMC) or CNCs have been utilized to improve the mechanical and chemical qualities of a variety of dental composites [Citation32,Citation33]. In this study, the miswak fibers containing a major component of cellulose were incorporated in a resin-based pit and fissure sealants to improve mechanical properties like flexural strength, compressive strength, and Vickers hardness.
In this study, the control group containing no miswak fibers showed lower flexural strength than sealants containing 1 or 2 wt.%. Indeed, higher concentrations (3–5 wt.%) of miswak fibers did not have a beneficial effect on flexural strength. The fact that miswak fibers at concentrations of 1–2 wt.% improved flexural strength indicates a reinforcing effect of the fibers. The decrease in effect at higher concentrations may be the result of the miswak fibers decreasing the surface energy and adhesion with the matrix, consequently impairing the mechanical properties. The finding that lower loads of miswak fibers increased the flexural strength of the sealants while higher loads brought no improvement corroborates the results reported by Takako Nishimura et al for cellulose nanofibers addition in glass ionomer cement [Citation32]. In other studies, adding cellulose microfibers to glass ionomer cement has been shown not to affect its mechanical characteristics. Thus, while the inclusion of a tiny amount of CNCs (0.2–0.4 wt. %) enhanced the mechanical characteristics of the material, the improvement was marginal [Citation34].
The compressive strength results followed the reverse pattern; irrespective of miswak fiber load, the control showed the highest compressive strength. However, the values measured were in the range of those reported by Lee et al [Citation35] for T product/Kuraray 37–86 MPa.
The microhardness values were also found to decrease with the addition of miswak fibers. The microhardness values measured were in the same range as those reported by Thunyakitpisal et al for resin-based pit and fissure sealants [Citation36].
The decrease in mechanical properties is probably owed to the opaqueness of miswak fibers which did not allow light to pass through the transparent pit and fissure sealant, thus reducing the degree of conversion and consequently the mechanical properties. Moreover, the mechanical properties were influenced by the fiber-resin interface, the length of fibers, and the ability of fibers to transfer force. With the increase in the concentration of miswak fiber, their surface energy and adhesion with the matrix decreased, jeopardizing the mechanical properties [Citation26]. According to the literature, no previous studies were found in which natural fibers have been added to pits and sealants so the results of the present study cannot be confirmed.
6.5. Antibacterial properties
The agar well diffusion method showed no zone of inhibition for the control sealant, which reflects the fact that the sealant contains no anti-bacterial component. The zone of inhibition of experimental groups increased when the percentage of miswak was increased. This effect may be explained by the properties of miswak as it contains nitrogen and alkaloids in variable structural arrangements, including benzyl isothiocyanate, salvadourea, and salvadorine [Citation28]. In various clinical studies, the antimicrobial effects of miswak against different species present in the oral cavity have been demonstrated [Citation37]. The studies have shown that these effects are quite significant, especially against pathogens that cause caries and periodontal diseases [Citation38]. The role of miswak against the major bacteria i.e. Streptococcus mutans that causes dental caries has been recognized [Citation39].
6.6. Limitations of the study
The main limitation is that this study did not include a clinical evaluation of the prepared sealants. In addition, the miswak fibers obtained were of larger size which hindered the mixing process and hence their addition to the pit and fissure sealant might have reduced the mechanical properties. The miswak fibers are opaque and incorporated in pit and fissure sealants they would hinder the ultraviolet blue light penetration and cause a lower depth of penetration, which might affect the degree of conversion of the sealant.
7. Conclusion
The miswak fibers containing cellulose and antibacterial agents were successfully mixed with pit and fissure sealants. The FTIR and XRD analysis confirmed the characteristic peaks associated with the structure of miswak fibers. The SEM analysis showed irregular particles in micron sizes. Whereas low concentrations of miswak fiber improved flexural strength, compressive strength, and Vickers hardness decreased irrespective of concentration of miswak fibers. The addition of miswak fibers imparted an antibacterial effect to the pit and fissure sealants. Future studies should evaluate the miswak fibers in nano size and their surface treatment for better dispersion in the resin matrix. Other than pits and fissure sealants, miswak fiber could be recommended for the fabrication of other dental materials.
Disclosure statement
No potential conflict of interest was reported by the author(s).
Data availability statement
All data generated or analyzed during this study are included in this published article [and its supplementary information files].
Additional information
Funding
References
- Valm AM. The structure of dental plaque microbial communities in the transition from health to dental caries and periodontal disease. J Mol Biol. 2019;431(16):1–9. doi: 10.1016/j.jmb.2019.05.016.
- Veiga NJ, Aires D, Douglas F, et al. Dental caries: a review. J Dent Oral Health. 2016;2(5):1–3.
- World Health Organization. Oral health promotion: an essential element of a health-promoting school. WHO Headquarters, Geneva: World Health Organization; 2003.
- Ahovuo‐Saloranta A, Forss H, Walsh, T, et al. Pit and fissure sealants for preventing dental decay in permanent teeth. Coch Database Syst Rev. 2017;(7):CD001830. doi: 10.1002/14651858.CD001830.pub5.
- Yu F, Yu H, Lin P, et al. Effect of an antibacterial monomer on the antibacterial activity of a pit-and-fissure sealant. PLOS One. 2016;11(9):e0162281. doi: 10.1371/journal.pone.0162281.
- Barroso JM, Torres CP, Lessa FCR, et al. Shear bond strength of pit-and-fissure sealants to saliva-contaminated and noncontaminated enamel. J Dentist Child. 2005;72(3);95–99.
- Pardi V, Sinhoreti MAC, Pereira AC, et al. In vitro evaluation of microleakage of different materials used as pit-and-fissure sealants. Brazil Dental J. 2006;17:49–52. doi: 10.1590/s0103-64402006000100011.
- Simonsen RJ. Pit and fissure sealant: review of the literature. Pediat Dentist. 2002;24(5):393–414.
- Reddy VR, Chowdhary N, Mukunda K, et al.. Retention of resin-based filled and unfilled pit and fissure sealants: a comparative clinical study. Contemp Clin Dentist. 2015;6(Suppl 1):S18. doi: 10.4103/0976-237X.152932.
- Santini A, Gallegos, IT, Felix CM. Photoinitiators in dentistry: a review. Primary Dental J. 2013;2(4):30–33. doi: 10.1308/205016814809859563.
- Zakaria MA, Hossain M, Moral AA. Comparative efficacy of nanofilled and microfilled resin-modified glass ionomer as pits and fissure sealant in permanent molar teeth. Bangabandhu Sheikh Mujib Med Univ J. 2017;10(2):53–57. doi: 10.3329/bsmmuj.v10i2.31877.
- (a) Salas-López EK, Pierdant-Pérez M, Hernández-Sierra JF, et al. Effect of silver nanoparticle-added pit and fissure sealant in the prevention of dental caries in children. J Clin Pediat Dentist. 2017; 41(1):48–52. (b) Fei X, Li Y, Weir MD, et al. Novel pit and fissure sealant containing nano-CaF2 and dimethylaminohexadecyl methacrylate with double benefits of fluoride release and antibacterial function. Dental Mater. 2020;36(9):1241–1253. doi: 10.1016/j.dental.2020.05.010.
- Swetha DL, Vinay C, Uloopi K, et al. Antibacterial and mechanical properties of pit and fissure sealants containing zinc oxide and calcium fluoride nanoparticles. Contemp Clin Dentist. 2019;10(3):477. doi: 10.4103/ccd.ccd_805_18.
- Memarpour M, Shafiei F. The effect of antibacterial agents on fissure sealant microleakage: a 6-month in vitro study. Oral Health Prevent Dentist. 2014;12(2):149–155.
- Chaurasia A, Patil R, Nagar A. Miswak in oral cavity–an update. J Oral Biol Craniofac Res. 2013;3(2):98–101. doi: 10.1016/j.jobcr.2012.09.004.
- Mohammad, H. H. In vitro antibacterial activity of propolis, alum, miswak, green and black tea, cloves extracts against Porphyromonas gingivalis isolated from perio-dontitis patients in Hilla City, Iraq. Amer J Phytomed Clin Therap 2013, 1 (2), 140–148.
- Al-Bayaty FH, AI-Koubaisi AH, Ali NAW, et al. Effect of mouth wash extracted from Salvadora persica (Miswak) on dental plaque formation: a clinical trail. J Med Plants Res. 2013;4(14):1446–1458.
- Al Qarni FM, ElFasakhany FM, Kenawi LM, et al. Antimicrobial activity of Azadirachta indica (neem) and Salvadora persica (miswak) extracts as endodontic irrigants. Endo EPT. 2019;13:237–245.
- Nordin A, Saim AB, Ramli R, et al. Miswak and oral health: an evidence-based review. Saudi J Biol Sci. 2020;27(7):1801–1810. doi: 10.1016/j.sjbs.2020.05.020.
- Savaş S. Structural properties and mechanical performance of Salvadora persica L.(Miswak) reinforced polypropylene composites. Polym Composit. 2019:40 (S1);E663–E677. doi: 10.1002/pc.24939.
- Yang S-Y, Choi J-W, Kim K-M, et al . Prevention of secondary caries using resin-based pit and fissure sealants containing hydrated calcium silicate. 2020;12(5):1200. doi: 10.3390/polym12051200.
- Shahswar RS, Saleem SS. Compressive strength of three types of pit and fissure sealants: an in-vitro comparative study. ZANCO J Pure Appl Sci. 2018;30(6):107–117.
- Moawed EA, Abulkibash AB. Selective separation of light green and Safranin O from aqueous solution using Salvadora persica (Miswak) powder as a new biosorbent. J Saudi Chem Soc. 2016;20:S178–S185. doi: 10.1016/j.jscs.2012.10.011.
- Guimarães JL, Frollini E, da Silva CG, et al . Characterization of banana, sugarcane bagasse and sponge gourd fibers of Brazil. Indust Crops Prod. 2009;30(3):407–415. doi: 10.1016/j.indcrop.2009.07.013.
- Elanthikkal S, Unnikrishnan G, Varghese S, et al. Cellulose microfibers produced from banana plant wastes: isolation and characterisation. Carbohydr Polym. 2010;80:582–859.
- Savaş S. Structural properties and mechanical performance of Salvadora persica L. (Miswak) reinforced polypropylene composites. J Plast Profession. 2019;40(S1):E663–E677.
- Hou X, Lv S, Chen , et al. Applications of Fourier transform infrared spectroscopy technologies on asphalt materials. Measurement. 2018;121:304–316. doi: 10.1016/j.measurement.2018.03.001.
- Jayanti AT, Nasution A, Suyanto H, Bramantoro , et al. The content of active materials in miswak (Salvadora persica): an analytical study using Fourier-transform infrared spectroscopy and ultraviolet–visible spectrophotometer. J Int Oral Health. 2021;13(3):258.
- Fawcett T, Crowder C, Kabekkodu S, et al. Reference materials for the study of polymorphism and crystallinity in cellulosics. Powd Diff. 2013;28(1):18–31. doi: 10.1017/S0885715612000930.
- Stubičar N, Šmit I, Stubičar M, et al. An X-ray diffraction study of the crystalline to amorphous phase change in cellulose during high-energy dry ball milling. Holzforschung 1998;52:455–458.
- French AD. Idealized powder diffraction patterns for cellulose polymorphs. Cellulose. 2014;21(2):885–896. doi: 10.1007/s10570-013-0030-4.
- Nishimura T, Shinonaga Y, Nagaishi C, et al. Effects of powdery cellulose nanofiber addition on the properties of glass ionomer cement. Materials. 2019;12(19):3077.
- Sabir M, Ali A, Siddiqui U, et al. Synthesis and characterization of cellulose/hydroxyapatite based dental restorative composites. J Biomater Sci. 2020;31(14):1806–1819. doi: 10.1080/09205063.2020.1777827.
- (a) Silva RM, Pereira FV, Mota FA, et al. Dental glass ionomer cement reinforced by cellulose microfibers and cellulose nanocrystals. Mater Sci Eng. 2016;58:389–395. DOI: 10.1016/j.dental.2019.01.006FromNLM. (b) Menezes-Silva R, de Oliveira BMB, Fernandes PHM, et al. Effects of the reinforced cellulose nanocrystals on glass-ionomer cements. Dental Mater. 2019;35(4):564–573. doi: 10.1016/j.msec.2015.08.041.
- Lee E-K, Jeong M-K, Lee J-Y, et al. A comparison experiment on the compressive strength of the sealant and the micro-leakage according to the light source enlighting. Int J Clin Prevent Dentist. 2012;8(4):245–251.
- Kashima DP, Thunyakitpisal P, Jiemsirilers S, et al. Depth of cure, flexural strength, microhardness, and cytotoxicity of light activated pit and fissure resin-based sealant experimental prototypes. CU Dent J. 2016;39:43–52.
- Sukkarwalla A, Ali SM, Lundberg, et al. Efficacy of miswak on oral pathogens. Dent Res J. 2013;10(3):314.
- Naseem S, Hashmi K, Fasih F, et al. In vitro evaluation of antimicrobial effect of miswak against common oral pathogens. Pak J Med Sci. 2014;30(2):398.
- Al-Sohaibani S, Murugan K. Anti-biofilm activity of Salvadora persica on cariogenic isolates of Streptococcus mutans: in vitro and molecular docking studies. Biofouling. 2012;28(1):29–38. doi: 10.1080/08927014.2011.647308.