Abstract
Owing to the development of glass 45S5 (Bioglass®) comprising 45 mol% SiO2, 24.5 mol% Na2O, 24.5 mol% CaO, and 6 mol% P2O5, different compositions of silicate glasses have been developed. When these silicate glasses contact an aqueous environment, such as body fluids, they induce apatite layer formation on their surfaces owing to ion exchange. In addition to promoting hard tissue formation, researchers have sought to enhance the antibacterial properties of these glasses, thereby resulting in the development of metal-doped silicate glasses. The addition of antibacterial metals (silver, copper, zinc, and gallium) to silicate glass offers a promising avenue for combating oral pathogens. In recent years, there has been growing interest in metal-doped phosphate glasses. The release of metal ions can be regulated by modifying the dissolution rate of the phosphate glasses. This review summarizes the metal-doped silicate and phosphate glasses that confer antibacterial activity. Future strategies for the development of dental biomaterials that incorporate metal-doped glass and exhibit antibacterial effects are discussed.
1. Introduction
An ion-releasing glass is a type of glass that can release certain ions and stimulate desirable biological responses. In the 1960s, Hench et al. [Citation1, Citation2] developed 45S5 comprising 45 mol% SiO2, 24.5 mol% Na2O, 24.5 mol% CaO, and 6 mol% P2O5, which has been commercially available as Bioglass® since 1985 [Citation3]. 45S5 bonds with bone and is clinically applied as a bone-grafting material in orthopedic surgery. In dentistry, 45S5 has been used for several dental applications such as resin composites [Citation4–6], root canal sealers [Citation7, Citation8], scaffolds [Citation9], and toothpaste [Citation10] owing to its promotion of hard tissue formation and integration with surrounding tissues.
When 45S5 contacts an aqueous environment (body fluids), it induces apatite layer formation on its surface owing to ion exchange [Citation3]. The release of ions from 45S5, particularly sodium, calcium, and phosphate ions, increases the local pH of the surrounding environment. As regards 45S5, different compositions of silicate glasses in the system of SiO2-Na2O-CaO-P2O5 have been reported [Citation11, Citation12], such as S53P4 (53 wt% SiO2, 23 wt% Na2O, 20 wt% CaO, and 4 wt% P2O5) [Citation13], 58S (60 mol% SiO2, 36 mol% CaO, and 4 mol% P2O5) [Citation14], and 13-93 (53 wt% SiO2, 6 wt% Na2O, 12 wt% K2O, 5 wt% MgO, 20 wt% CaO, and 4 wt% P2O5) [Citation15]. These glasses were developed owing to their composition-dependent degradability, bone-forming ability, and anti-inflammatory properties.
An increase in pH owing to ions released from the aforementioned silicate glasses can reduce the number of bacteria sensitive to pH changes [Citation2]. However, silicate glass is insufficient to effectively inhibit bacteria living in oral biofilms or tooth substrates [Citation14,Citation16]. Hence, several attempts have been made to provide silicate glasses with antibacterial effects by replacing certain components (Ca or Na) with metals such as silver (Ag), copper (Cu), zinc (Zn), or gallium (Ga), thereby exhibiting strong antibacterial properties. These metal-doped silicate glasses maintain their apatite-forming ability by releasing sodium, calcium, silicate, and phosphate ions, whereas the added metals confer antibacterial effects.
In recent years, there has been a growing interest in phosphate glass as a carrier for metal ion release. Phosphate glass is based on P2O5 as the glass network former containing CaO and/or Na2O as the network modifiers. The chemical composition of phosphate glasses can be easily manipulated. Hence, the release of ions incorporated into the glass can be regulated by the dissolution rate of the glass [Citation17]. The duration, during which the glass exhibits antibacterial properties, can be adjusted by controlling the release of metal ions.
Dental caries has been recognized as an infectious disease induced by cariogenic bacteria. Developing restorative materials with antibacterial effects has become an important research topic in dental materials science. The control of bacteria around/beneath restorations could help eliminate the risks of further demineralization and cavitation, contributing to the prevention of secondary caries. Apical periodontitis is an infectious disease, and the treatment requires complete bacterial eradication inside filled root canals. However, it is challenging to achieve the complete elimination of bacterial infection in root canals using only mechanical instrumentation, irrigation, and medication. Therefore, endodontic filling materials capable of eliminating residual endodontic pathogens in root canals have been proposed as a possible solution to this problem. The application of metal-releasing glasses to dental materials has been attempted to address the growing need for antibacterial dental materials used for restorative/endodontic treatments. Further, a denture base with antimicrobial effects against fungi is also useful for the prevention of denture stomatitis.
In this review, metal-doped silicate and phosphate glasses with antibacterial activities are summarized. Furthermore, future strategies for the development of dental biomaterials that incorporate metal-doped glass and exhibit antibacterial effects are discussed.
2. Metal-doped glasses for conferring antibacterial activity
2.1. Silver-doped glass
Silver ions are widely recognized for their strong antibacterial properties and effectiveness against a wide range of bacteria. Silver ions and nanoparticles have demonstrated efficacy against different pathogenic bacteria, including Staphylococcus aureus, Escherichia coli, Pseudomonas aeruginosa, Streptococcus mutans, Porphyromonas gingivalis, Enterococcus faecalis, Lactobacillus casei, and Actinomyces naeslundii [Citation18–23]. When silver is incorporated into glass, it releases silver ions that contribute to its antibacterial effect. Silver ions can disrupt bacterial cell membranes, thereby resulting in an increased permeability and leakage of cellular contents [Citation24, Citation25]. They can also bind to sulfur-containing proteins and enzymes in bacteria, thereby interfering with their normal functions and inhibiting bacterial growth.
Over the past two decades, different Ag-doped silicate glasses have been developed [Citation26–34](). Bellantone et al. [Citation26] fabricated a silver-releasing glass comprising 76% silicon dioxide (SiO2), 19% calcium oxide (CaO), 2% phosphorus pentoxide (P2O5), and 3% silver oxide (Ag2O) in percentage of weight. The silver-doped glass in the range of 0.05 to 0.20 mg/mL of culture medium demonstrated bactericidal effects against E. coli, P. aeruginosa, and S. aureus. A complete killing effect was elicited within 24 h of incubation with 10 mg/mL silver-releasing glass. Phetnin et al. [Citation29] investigated the antibacterial activity of Ag-containing mesoporous silicate glass. The agar diffusion test revealed that mesoporous glasses containing 1, 3, or 5 mol% Ag2O produced inhibition zones against S. aureus and E. coli. Hence, these mesoporous glasses have potential as drug-loading carriers with antibacterial properties.
Table 1. Metal-doped silicate glasses and their application to dental biomaterials.
In dentistry, using silver-doped silicate glasses in pulp capping materials [Citation31, Citation32], resin composites [Citation33], and adhesives for orthodontic treatment [Citation34] has been reported. Zhu et al. [Citation31, Citation32] investigated the antibacterial activity of an injectable chitosan hydrogel pulp-capping material containing silver-doped silicate glass. When S. mutans and L. casei were incubated in the presence of the silver-doped glass/chitosan hydrogel at a 1:1 wt ratio, both bacteria were killed after incubation for 24 h. Lee et al. [Citation34] examined the antibacterial effect of silver-doped glass comprising 58 wt% SiO2, 32 wt% CaO, 9 wt% P2O5, and 1 wt% Ag2O in a bisphenol-A diglycidyl ether dimethacrylate (Bis-GMA)/triethylene glycol dimethacrylate (TEGDMA)-based adhesive resin. The cured resin containing 1 wt. % Ag-doped glass inhibited the growth of S. mutans after incubation for 24 h.
2.2. Copper-doped glass
Copper is a vital mineral that is essential for biological processes. Most Cu in the human body is bound to enzyme prosthetic groups or proteins. Copper is nontoxic to human tissues and exhibits strong antibacterial activity.
The antibacterial properties of copper-doped silicate and phosphate glasses have already been reported, particularly in the last ten years [Citation35–39](). Wu et al. [Citation35] investigated the antibacterial activity of Cu-doped silicate glass (molar ratio of Cu/Ca/P/Si = 5/10/5/80) against E. coli. The copper-doped glass was mixed with E. coli suspension (3.5–4.0 × 104 bacteria/mL), stored at 4 °C for 7 d, and incubated at 37 °C for 12 h. The copper ions released from the copper-doped glass demonstrated a killing effect against E. coli.
Choe et al. [Citation38] developed zinc phosphate cement incorporating copper-doped silicate glass nanoparticles comprising 5 wt% Cu, 10 wt% Ca, and 85 wt% Si. When E. faecalis (106 CFU/mL) was incubated in the presence of eluate from the zinc phosphate cements with/without copper-doped glass nanoparticles, the number of bacteria after incubation for 3 h was significantly decreased.
2.3. Zinc-doped glass
Silver- and copper-doped glasses have been extensively studied because of their ability to inhibit the growth of microorganisms. However, one of the major issues in incorporating metal-doped glasses into dental restorative materials is “discoloration”. When Ag or Cu ions are used at high concentrations or in certain formulations, they can cause noticeable discoloration of the material. This discoloration can be undesirable, particularly for restorative materials that must maintain their aesthetic appearance. To overcome this issue, researchers have explored the use of zinc, which may reduce discoloration (). The antibacterial action of Zn is still being studied, and its effects on bacteria vary depending on the specific species and strains involved. One potential mechanism by which zinc exhibits antibacterial activity against oral streptococci is through interference with bacterial glycolysis [Citation40]. Glycolysis is the metabolic pathway that converts glucose to pyruvate and generates energy for cells. Zinc ions have been shown to inhibit enzymes involved in glycolysis, thereby disrupting energy production and metabolic processes in bacteria.
Several attempts have been made to incorporate zinc oxide (ZnO) into silicate [Citation34, Citation42–44] or phosphate glasses [Citation41, Citation47–49] ( and ). The addition of ZnO to these glass systems resulted in zinc-ion-releasing properties and antibacterial activity against a wide range of bacteria. Zn-doped silicate glasses have also been incorporated into glass polyalkenoate cements [Citation43, Citation44]. Boyd et al. [Citation43] fabricated two kinds of cements containing glass A (42 mol% SiO2, 5 mol% CaO, and 53 mol% ZnO) and glass B (42 mol% SiO2, 5 mol% SrO, and 53 mol% ZnO). They found that the release of Zn ion from both glasses exhibited antibacterial activity against S. mutans and A. viscosus, with the inhibition of growth greatest shortly after cement preparation and little or no inhibition measurable after immersion in water for 30 d. Coughlan et al. [Citation45] reported that the addition of zinc and silver to silicate glass reduced bacterial viability when co-cultured with the elute from the glass for 2 h. Phosphate glasses are renowned for their substantial calcium and phosphate contents, which play a pivotal role in remineralization [Citation46]. The dissolution rate of phosphate glasses can be easily adjusted by changing their chemical composition [Citation17]. Lee et al. [Citation47, Citation48] and Kim et al. [Citation49] incorporated Zn-doped phosphate glasses into orthodontic acrylic resins/adhesives or resin composites and demonstrated their antimicrobial effects against oral microorganisms. However, these methods of incorporating Zn-doped silicate and phosphate glasses into restorative materials have only demonstrated short-term effects.
Table 2. Metal-doped phosphate glasses and their application to dental biomaterials.
2.4. Gallium-doped glass
Recently, extensive research has been conducted to examine the antimicrobial activity of Ga against different microorganisms [Citation50–53]. Several studies have reported that Ga exhibits antimicrobial effects, particularly against Gram-negative bacteria, which are known for their increased resistance to numerous antibiotics [Citation54, Citation55]. Gram-negative bacteria possess an outer membrane that acts as a protective barrier, thereby making it difficult for antimicrobial agents to penetrate and disrupt the cellular processes. The antimicrobial activity of Ga is owing to its ability to interfere with iron uptake by these organisms. Iron is essential for most bacteria, as it is required for enzymes that mediate numerous key processes such as DNA synthesis, electron transport, and oxidative stress defense. Gallium has a nearly identical ionic radius, coordination chemistry, and ionization potential to Fe(III) [Citation56]. Given that several bacterial uptake systems are unable to distinguish between gallium and iron, gallium can substitute for iron and disrupt iron-dependent processes in bacterial metabolism [Citation57]. By disrupting iron acquisition, Ga inhibits crucial processes within bacteria that rely on iron, such as the production of iron-containing enzymes and proteins necessary for growth and virulence. This disruption inhibits bacterial growth and increases the susceptibility of bacteria to the host immune system or other antimicrobial agents.
Several studies have reported the fabrication of gallium-doped silicate [Citation58, Citation59] and phosphate [Citation60, Citation61] glasses as carrier materials in terms of releasing gallium ions and have evaluated their antibacterial activities ( and ). Furthermore, gallium-doped glasses have been used as antibacterial materials against bone defects or skin incision infections [Citation62, Citation63]. Valappil et al. [Citation64] reported that gallium silver-doped phosphate glass inhibited the growth of P. gingivalis in biofilms in the constant-depth film fermentor model. Although the antibacterial effects against oral microorganisms have been previously investigated [Citation61, Citation64], research is ongoing on the potential applications of gallium-releasing glasses as antimicrobial dental materials.
3. Future strategy toward development of antibacterial glasses
Numerous investigations on metal-doped antibacterial glasses have been reported. However, the following points highlight the complexities associated with them:
Diminished antibacterial effect over time: Leaching of antibacterial metal ions from glass when exposed to saliva or other fluids can diminish the antibacterial effect over time. Continuous exposure to the oral environment may reduce the concentration of the released metal ions, thereby decreasing their long-term ability to combat bacteria.
Disruption of oral microbial homeostasis: The oral cavity harbors a complex ecosystem of microorganisms, including beneficial bacteria, that contribute to oral health. Continuous and potent delivery of antimicrobial components may disturb this delicate balance, thereby resulting in the disruption of microbial homeostasis. This could potentially result in unintended consequences such as promoting the growth of antibiotic-resistant strains or affecting beneficial bacteria that help to maintain oral health. Moreover, the continuous release of metals into the oral environment may have significant implications on biological safety.
To overcome these limitations, the on-demand release of antimicrobial components in response to environmental changes is a promising approach. This approach minimizes the risk of disrupting oral microbial homeostasis and influences biosafety. Furthermore, the controlled release in response to environmental changes can prolong the duration of the antibacterial activity. An example of an on-demand release approach is glass, which releases metal ions in response to environmental acidity. This is beneficial in terms of providing dental materials with a controlled release ability to effectively supply antimicrobial ions when the acidogenic bacteria in dental plaques produce acids. BioUnion filler is a glass particle developed by GC corp., comprising SiO2-ZnO-CaO-F that is capable of releasing Zn2+, Ca2+, and F- [Citation65]. Liu et al. [Citation66] reported that the release of Zn2+ from the BioUnion filler was accelerated under acidic conditions. This technology enables the on-demand release of antimicrobial components from dental materials. As a commercial material, a glass ionomer cement (GIC) containing BioUnion filler for root surface restoration (Caredyne® Restore, GC Corp., Japan) is on the market. Liu et al. [Citation67] reported that the acidity-induced release of Zn2+ from the GIC containing BioUnion filler effectively inhibited the growth and adherence of oral bacteria even after repeated exposure to acetic acid. Kohno et al. [Citation68] assembled an original bioreactor and reported that the biofilm, similar to those formed on dental materials in the oral cavity, could be formed using a bacterial suspension prepared from human saliva and adding 0.2% sucrose solution. Using the established in vitro culture system, it was demonstrated that the GIC containing BioUnion filler effectively hindered oral biofilm formation on its surface compared to fluoride-releasing GIC and resin composites [Citation68].
While the acidity-induced release of Zn2+ from BioUnion filler kills oral bacteria [Citation66], the concentration of Zn2+ from restorative cement incorporating BioUnion filler is not considerably high enough to exhibit bactericidal effects against microorganisms in oral biofilm. Deng et al. [Citation69] recently fabricated acidity-responsive silicate glasses containing high Zn concentrations (33–38 mol%; ). These glasses are modifications of the BioUnion filler that increase the Zn content and remove the Ca incorporated in the BioUnion filler. The glass particles with a greater Zn content demonstrated greater release concentrations of Zn2+ in acids. When S. mutans was anaerobically incubated in different pH media (5.1, 6.1, or 7.4), the number of viable S. mutans decreased in the presence of each particle with a decrease in the medium pH value. At pH 5.1, the number of viable cells in the presence of each particle was lower than the initial number of bacteria, thereby indicating that the glass particles exhibited bactericidal effects under acidic conditions. The glass particles that can release high concentrations of Zn2+ under acidic conditions would cause bactericidal effects in microorganisms in oral biofilms even when incorporated into the cements.
Figure 1. Acidity-responsive Zn-releasing glass particles.
(A) Scanning electron microscope (SEM) and energy dispersive X-ray spectroscopy (EDS) mapping images of silicate-based glass particles containing 33 mol% Zn.
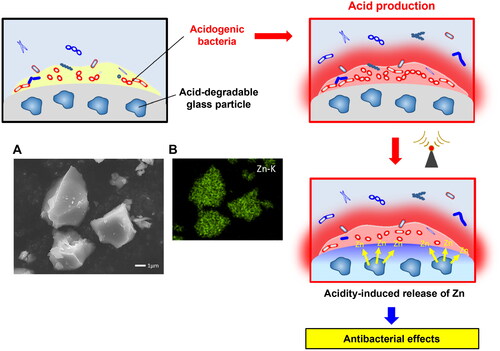
Dental caries is caused by acids from the glucose metabolism of specific bacteria in the oral cavity. One of the aims of conferring antibacterial activity to restorative or preventive materials is to suppress caries-related bacteria and inhibit the decrease in pH that occurs in dental plaques [Citation70]. The aforementioned glass particles were capable of releasing Zn ions when the environmental pH decreased. Such an acidity-responsive ion-releasing technology that produces antibacterial effects as a result of environmental (pH or microbiota) changes is of significant interest. The future design of dental materials with ion-releasing glasses must involve smart behaviors to maintain the oral environment and safeguard human health.
Another promising approach involves recharging metal ions into materials that incorporate glass. Kohno et al. [Citation71] investigated the release properties and recharging ability of GIC containing BioUnion filler using an in vitro saliva drop setting to simulate in vivo conditions of the oral cavity. The GIC incorporating the BioUnion filler was found to be rechargeable with Zn ions through the application of a tooth gel containing Zn. Following recharging, the effective concentration of Zn ions necessary to hinder the formation of a multispecies oral biofilm on the surface of the material was maintained. Such recharge properties are valuable for the long-term antimicrobial effects of dental materials as they can prevent the formation of multispecies oral biofilms on their surfaces.
Incorporating contact inhibition into dental materials is another smart approach to provide long-lasting antibacterial effects without releasing antimicrobial components [Citation70]. Several studies have reported that silver nanoparticles or silver-containing materials (zirconium phosphate and silica gel) exhibit antibacterial activities not owing to the release of silver ions but rather owing to the activation of oxygen based on the catalytic action of silver [Citation72, Citation73]. Silver generates reactive oxygen species (ROS) such as superoxide and hydroxyl radicals, which further contribute to bacterial cell damage [Citation74, Citation75]. Hence, if dental materials containing metal-doped glass exhibit contact inhibition, they must exhibit long-lasting inhibitory effects against oral microorganisms on their surfaces.
In summary, various compositions of metal-doped silicate and phosphate glasses have been shown to enhance the antibacterial properties of dental materials. Employing approaches such as the on-demand release of ions and contact inhibition in dental materials are promising strategies to exhibit long-lasting antimicrobial effects and minimize disruption in the oral microbial ecosystem. The rechargeability of ions in materials containing metal-doped glasses can extend the duration of ion release and antibacterial activity. These innovative approaches are expected to affect oral health, thereby preventing the formation of biofilms and maintaining the balance of the oral microbiome.
Acknowledgments
This work was supported in part by a Grant-in-Aid for Scientific Research (JP20H03871, JP23K09215, JP23KK0161) from the Japan Society for the Promotion of Science.
Declaration of interest
The authors declare no conflict of interest.
Data availability
Data sharing is not applicable to this article as no new data were created or analyzed in this study.
References
- Hench LL, Splinter RJ, Allen WC, et al. Bonding mechanisms at the interface of ceramic prosthetic materials. J Biomed Mater Res. 1971;5(6):1–10. doi: 10.1002/jbm.820050611.
- Hench LL, Jones JR. Bioactive glasses: Frontiers and challenges. Front Bioeng Biotechnol. 2015;3:194. doi: 10.3389/fbioe.2015.00194.
- Hench LL. The story of Bioglass. J Mater Sci Mater Med. 2006;17(11):967–78. doi: 10.1007/s10856-006-0432-z.
- Tezvergil-Mutluay A, Seseogullari-Dirihan R, Feitosa VP, et al. Effects of composites containing bioactive glasses on demineralized dentin. J Dent Res. 2017;96(9):999–1005. doi: 10.1177/0022034517709464.
- Par M, Gubler A, Attin T, et al. Ion release and hydroxyapatite precipitation of resin composites functionalized with two types of bioactive glass. J Dent. 2022;118:103950. doi: 10.1016/j.jdent.2022.103950.
- Yun J, Tsui KH, Fan Z, et al. A biomimetic approach to evaluate mineralization of bioactive glass-loaded resin composites. J Prosthodont Res. 2022;66(4):572–581. doi: 10.2186/jpr.JPR_D_21_00177.
- Hoikkala NJ, Siekkinen M, Hupa L, et al. Behavior of different bioactive glasses incorporated in polydimethylsiloxane endodontic sealer. Dent Mater. 2021;37(2):321–327. doi: 10.1016/j.dental.2020.11.013.
- Cardoso OS, Meier MM, Carvalho EM, et al. Synthesis and characterization of experimental endodontic sealers containing bioactive glasses particles of NbG or 45S5. J Mech Behav Biomed Mater. 2022;125:104971. doi: 10.1016/j.jmbbm.2021.104971.
- El-Gendy R, Kirkham J, Newby PJ, et al. Investigating the vascularization of tissue-engineered bone constructs using dental pulp cells and 45S5 Bioglass® scaffolds. Tissue Eng Part A. 2015;21(13–14):2034–43. doi: 10.1089/ten.tea.2014.0485.
- Zhong Y, Liu J, Li X, et al. Effect of a novel bioactive glass-ceramic on dentinal tubule occlusion: an in vitro study. Aust Dent J. 2015;60(1):96–103. doi: 10.1111/adj.12241.
- Shearer A, Montazerian M, Sly JJ, et al. Trends and perspectives on the commercialization of bioactive glasses. Acta Biomater. 2023;160:14–31. doi: 10.1016/j.actbio.2023.02.020.
- Shearer A, Montazerian M, Mauro JC. Modern definition of bioactive glasses and glass-ceramics. Journal of Non-Crystalline Solids. 2023;608:122228. doi: 10.1016/j.jnoncrysol.2023.122228.
- Barrak FN, Li S, Mohammed AA, Myant C, et al. Anti-inflammatory properties of S53P4 bioactive glass implant material. J Dent. 2022;127:104296. doi: 10.1016/j.jdent.2022.104296.
- Galarraga-Vinueza ME, Passoni B, Benfatti CAM, et al. Inhibition of multi-species oral biofilm by bromide doped bioactive glass. J Biomed Mater Res A. 2017;105(7):1994–2003. doi: 10.1002/jbm.a.36056.
- Bi L, Zobell B, Liu X, et al. Healing of critical-size segmental defects in rat femora using strong porous bioactive glass scaffolds. Mater Sci Eng C Mater Biol Appl. 2014;42:816–24. doi: 10.1016/j.msec.2014.06.022.
- Gubler M, Brunner TJ, Zehnder M, et al. Do bioactive glasses convey a disinfecting mechanism beyond a mere increase in pH? Int Endod J. 2008;41(8):670–8. doi: 10.1111/j.1365-2591.2008.01413.x.
- Pickup DM, Valappil SP, Moss RM, et al. Preparation, structural characterization, and antibacterial properties of Ga-doped sol–gel phosphate-based glass. J Mater Sci. 2009;44:1858–67. doi: 10.1007/s10853-008-3237-2.
- Hernández-Sierra JF, Ruiz F, Pena DC, et al. The antimicrobial sensitivity of Streptococcus mutans to nanoparticles of silver, zinc oxide, and gold. Nanomedicine. 2008;4(3):237–40. doi: 10.1016/j.nano.2008.04.005.
- Bruna T, Maldonado-Bravo F, Jara P, et al. Silver nanoparticles and their antibacterial applications. Int J Mol Sci. 2021;22(13):7202. doi: 10.3390/ijms22137202.
- Soma T, Iwasaki R, Sato Y, et al. An ionic silver coating prevents implant-associated infection by anaerobic bacteria in vitro and in vivo in mice. Sci Rep. 2022;12(1):18387. doi: 10.1038/s41598-022-23322-6.
- Lv S, Fan W, Fan B. Enhanced in vitro antibacterial effect against Enterococcus faecalis by using both low-dose cetylpyridinium chloride and silver ions. BMC Oral Health. 2023;23(1):299. doi: 10.1186/s12903-023-02972-6.
- Tian X, Jiang X, Welch C, et al. Bactericidal effects of silver nanoparticles on lactobacilli and the underlying mechanism. ACS Appl Mater Interfaces. 2018;10(10):8443–50. doi: 10.1021/acsami.7b17274.
- Tülü G, Kaya BÜ, Çetin ES, et al. Antibacterial effect of silver nanoparticles mixed with calcium hydroxide or chlorhexidine on multispecies biofilms. Odontology. 2021;109(4):802–11. doi: 10.1007/s10266-021-00601-8.
- Jung WK, Koo HC, Kim KW, et al. Antibacterial activity and mechanism of action of the silver ion in Staphylococcus aureus and Escherichia coli. Appl Environ Microbiol. 200;74(7):2171–8. doi: 10.1128/AEM.02001-07.
- Khan K, Javed S. Functionalization of inorganic nanoparticles to augment antimicrobial efficiency: A critical analysis. Curr Pharm Biotechnol. 2018;19(7):523–36. doi: 10.2174/1389201019666180731121401.
- Bellantone M, Coleman NJ, Hench LL. Bacteriostatic action of a novel four-component bioactive glass. J Biomed Mater Res. 2000;51(3):484–90. doi: 10.1002/1097-4636(20000905)51:3<484::AID-JBM24>3.0.CO;2-4.
- Blaker JJ, Nazhat SN, Boccaccini AR. Development and characterization of silver-doped bioactive glass-coated sutures for tissue engineering and wound healing applications. Biomaterials. 2004;25(7–8):1319–29. doi: 10.1016/j.biomaterials.2003.08.007.
- Jones JR, Ehrenfried LM, Saravanapavan P, et al. Controlling ion release from bioactive glass foam scaffolds with antibacterial properties. J Mater Sci Mater Med. 2006;17(11):989–96. doi: 10.1007/s10856-006-0434-x.
- Phetnin R, Rattanachan ST. Preparation and antibacterial property on silver incorporated mesoporous bioactive glass microspheres. Journal of Sol-Gel Science and Technology. 2015;75:279–90. doi: 10.1007/s10971-015-3697-1.
- Bellantone M, Williams HD, Hench LL. Broad-spectrum bactericidal activity of Ag2O-doped bioactive glass. Antimicrob Agents Chemother. 2002;46(6):1940–5. doi: 10.1128/AAC.46.6.1940-1945.2002.
- Zhu N, Chatzistavrou X, Ge L, et al. Biological properties of modified bioactive glass on dental pulp cells. J Dent. 2019;83:18–26. doi: 10.1016/j.jdent.2019.01.017.
- Zhu N, Chatzistavrou X, Papagerakis P, et al. Silver-doped bioactive glass/chitosan hydrogel with potential application in dental pulp repair. ACS Biomater Sci Eng. 2019;5(9):4624–33. doi: 10.1021/acsbiomaterials.9b00811.
- Kattan H, Chatzistavrou X, Boynton J, Dennison J, Yaman P, Papagerakis P. Physical properties of an Ag-doped bioactive flowable composite resin. Materials (Basel). 2015;8(8):4668–4678. doi: 10.3390/ma8084668.
- Lee SM, Kim IR, Park BS, et al. Remineralization property of an orthodontic primer containing a bioactive glass with silver and zinc. Materials (Basel). 2017;10(11):1253. doi: 10.3390/ma10111253.
- Wu C, Zhou Y, Xu M, et al. Copper-containing mesoporous bioactive glass scaffolds with multifunctional properties of angiogenesis capacity, osteostimulation, and antibacterial activity. Biomaterials. 2013;34(2):422–33. doi: 10.1016/j.biomaterials.2012.09.066.
- Li J, Zhai D, Lv F, et al. Preparation of copper-containing bioactive glass/eggshell membrane nanocomposites for improving angiogenesis, antibacterial activity, and wound healing. Acta Biomater. 2016;36:254–66. doi: 10.1016/j.actbio.2016.03.011.
- Zheng K, Kang J, Rutkowski B, et al. Toward highly dispersed mesoporous bioactive glass nanoparticles with high Cu concentration using Cu/ascorbic acid complex as precursor. Front Chem. 2019;7:497. doi: 10.3389/fchem.2019.00497.
- Choe YE, Kim YJ, Jeon SJ, et al. Investigating the mechanophysical and biological characteristics of therapeutic dental cement incorporating copper doped bioglass nanoparticles. Dent Mater. 2022;38(2):363–375. doi: 10.1016/j.dental.2021.12.019.
- Shetty S, Sekar P, Shetty RM, et al. Antibacterial and antibiofilm efficacy of copper-doped phosphate glass on pathogenic bacteria. Molecules. 2023;28(7):3179. doi: 10.3390/molecules28073179.
- Phan TN, Buckner T, Sheng J, et al. Physiologic actions of zinc related to inhibition of acid and alkali production by oral streptococci in suspensions and biofilms. Oral Microbiol Immunol. 2004;19(1):31–8. doi: 10.1046/j.0902-0055.2003.00109.x.
- Raja FNS, Worthington T, de Souza LPL, et al. Synergistic antimicrobial metal oxide-doped phosphate glasses; a potential strategy to reduce antimicrobial resistance and host cell toxicity. ACS Biomater Sci Eng. 2022;8(3):1193–9. doi: 10.1021/acsbiomaterials.1c00876.
- Atkinson I, Anghel EM, Predoana L, et al. Influence of ZnO addition on the structural, in vitro behavior and antimicrobial activity of sol–gel derived CaO–P2O5–SiO2 bioactive glasses. Ceram Int 2016;42(2):3033–45. doi: 10.1016/j.ceramint.2015.10.090.
- Boyd D, Li H, Tanner DA, et al. The antibacterial effects of zinc ion migration from zinc-based glass polyalkenoate cements. J Mater Sci Mater Med. 2006;17(6):489–94. doi: 10.1007/s10856-006-8930-6.
- Clarkin O, Wren A, Thornton R, Cooney J, Towler M. Antibacterial analysis of a zinc-based glass polyalkenoate cement. J Biomater Appl. 2011;26(3):277–92. doi: 10.1177/0885328210364430.
- Coughlan A, Scanlon K, Mahon BP, et al. Zinc and silver glass polyalkenoate cements: an evaluation of their antibacterial nature. Biomed Mater Eng. 2010;20(2):99–106. doi: 10.3233/BME-2010-0620.
- Ramadoss R, Padmanaban R, Subramanian B. Role of bioglass in enamel remineralization: Existing strategies and future prospects-A narrative review. J Biomed Mater Res B Appl Biomater. 2022;110(1):45–66. doi: 10.1002/jbm.b.34904.
- Lee MJ, Kim MJ, Mangal U, et al. Zinc-modified phosphate-based glass micro-filler improves Candida albicans resistance of auto-polymerized acrylic resin without altering mechanical performance. Sci Rep. 2022;12(1):19456. doi: 10.1038/s41598-022-24172-y.
- Lee MJ, Seo YB, Seo JY, et al. Development of a bioactive flowable resin composite containing a zinc-doped phosphate-based glass. Nanomaterials (Basel). 2020;10(11):2311. doi: 10.3390/nano10112311.
- Kim MJ, Seo JY, Jung IJ, et al. A novel orthodontic adhesive containing zinc-doped phosphate-based glass for preventing white spot lesions. J Dent. 2023;137:104689. doi: 10.1016/j.jdent.2023.104689.
- Kelson AB, Carnevali M, Truong-Le V. Gallium-based anti-infectives: Targeting microbial iron-uptake mechanisms. Curr Opin Pharmacol. 2013;13(5):707–16. doi: 10.1016/j.coph.2013.07.001.
- Zemke AC, Madison CJ, Kasturiarachi N, et al. Antimicrobial synergism toward Pseudomonas aeruginosa by Gallium(III) and inorganic nitrite. Front Microbiol. 2020;11:2113. doi: 10.3389/fmicb.2020.02113.
- Kircheva N, Dudev T. Competition between abiogenic and biogenic metal cations in biological systems: mechanisms of gallium’s anticancer and antibacterial effect. J Inorg Biochem. 2021;214:111309. doi: 10.1016/j.jinorgbio.2020.111309.
- Zeng J, Wu L, Liu Z, et al. Gain-of-function mutations in acid stress response (evgS) protect Escherichia coli from killing by gallium nitrate, an antimicrobial candidate. Antimicrob Agents Chemother. 2021;65(3):e01595–20. doi: 10.1128/AAC.01595-20.
- Goss CH, Kaneko Y, Khuu L, et al. Gallium disrupts bacterial iron metabolism and has therapeutic effects in mice and humans with lung infections. Sci Transl Med. 2018;10(460):eaat7520. doi: 10.1126/scitranslmed.aat7520.
- Li F, Liu F, Huang K, et al. Advancement of gallium and gallium-based compounds as antimicrobial agents. Front Bioeng Biotechnol. 2022;10:827960. doi: 10.3389/fbioe.2022.827960.
- Minandri F, Bonchi C, Frangipani E, et al. Promises and failures of gallium as an antibacterial agent. Future Microbiol. 2014;9(3):379–97. doi: 10.2217/fmb.14.3.
- Crunkhorn S. Antibacterial agents: Gallium fights infection in phase I trial. Nat Rev Drug Discov. 2018;17(11):786. doi: 10.1038/nrd.2018.186.
- Franchini M, Lusvardi G, Malavasi G, et al. Gallium-containing phospho-silicate glasses: synthesis and in vitro bioactivity. Mater Sci Eng C Mater Biol Appl. 2012;32(6):1401–6. doi: 10.1016/j.msec.2012.04.016.
- Pourshahrestani S, Zeimaran E, Adib Kadri N, Gargiulo N, Samuel S, Naveen SV, Kamarul T, Towler MR. Gallium-containing mesoporous bioactive glass with potent hemostatic activity and antibacterial efficacy. J Mater Chem B. 2016;4(1):71–86. doi: 10.1039/c5tb02062j.
- Łapa A, Cresswell M, Campbell I, et al. Gallium- and cerium-doped phosphate glasses with antibacterial properties for medical applications. Adv Eng Mater. 2020;22(9):1901577. doi: 10.1002/adem.201901577.
- Sahdev R, Ansari TI, Higham SM, et al. Potential use of gallium-doped phosphate-based glass material for periodontitis treatment. J Biomater Appl. 2015;30(1):85–92. doi: 10.1177/0885328215571952.
- Wang M, Yang Y, Chi G, et al. A 3D printed Ga containing scaffold with both anti-infection and bone homeostasis-regulating properties for the treatment of infected bone defects. J Mater Chem B. 2021;9(23):4735–45. doi: 10.1039/d1tb00387a.
- Łapa A, Cresswell M, Campbell I, et al. Ga and Ce ion-doped phosphate glass fibers with antibacterial properties and their composite for wound healing applications. J Mater Chem B. 2019;7(45):7246. doi: 10.1039/c9tb90158b.
- Valappil SP, Coombes M, Wright L, et al. Role of gallium and silver from phosphate-based glasses on in vitro dual species oral biofilm models of Porphyromonas gingivalis and Streptococcus gordonii. Acta Biomater. 2012;8(5):1957–65. doi: 10.1016/j.actbio.2012.01.017.
- Imazato S, Kohno T, Tsuboi R, Thongthai P, Xu HH, Kitagawa H. Cutting-edge filler technologies to release bio-active components for restorative and preventive dentistry. Dent Mater J. 2020;39(1):69–79. doi: 10.4012/dmj.2019-350.
- Liu Y, Kohno T, Tsuboi R, Kitagawa H, Imazato S. Acidity-induced release of zinc ion from BioUnionTM filler and its inhibitory effects against Streptococcus mutans. Dent Mater J. 2020;39(4):547–553. doi: 10.4012/dmj.2019-061.
- Liu Y, Kohno T, Tsuboi R, Thongthai P, Fan D, Sakai H, Kitagawa H, Imazato S. Antibacterial effects and physical properties of a glass ionomer cement containing BioUnion filler with acidity-induced ability to release zinc ion. Dent Mater J. 2021;40(6):1418–1427. doi: 10.4012/dmj.2021-052.
- Kohno T, Kitagawa H, Tsuboi R, et al. Establishment of novel in vitro culture system with the ability to reproduce oral biofilm formation on dental materials. Sci Rep. 2021;11(1):21188. doi: 10.1038/s41598-021-00803-8.
- Deng F, Sakai H, Kitagawa H, et al. Fabrication of pH-Responsive Zn2+-releasing glass particles for smart antibacterial restoratives. Molecules. 2022;27(21):7202. doi: 10.3390/molecules27217202.
- Imazato S, Kitagawa H. Oral Biofilms and Modern Dental Materials. Advances Toward Bioactivity. Dental resin-based materials with antibacterial properties: contact inhibition and controlled release. (Ionescu AC, Hahnel S, ed.), Springer, Switzerland, pp. 127–140, 2021.
- Kohno T, Liu Y, Tsuboi R, Kitagawa H, Imazato S. Evaluation of ion release and the recharge ability of glass-ionomer cement containing BioUnion filler using an in vitro saliva-drop setting assembly. Dent Mater. 2021;37(5):882–893. doi: 10.1016/j.dental.2021.02.022.
- Fan X, Yahia L, Sacher E. Antimicrobial properties of the Ag, Cu nanoparticle system. Biology (Basel). 2021;10(2):137. doi: 10.3390/biology10020137.
- Imazato S. Antibacterial properties of resin composites and dentin bonding systems. Dent Mater. 2003;19(6):449–57. doi: 10.1016/s0109-5641(02)00102-1.
- Padmavathy N, Vijayaraghavan R. Enhanced bioactivity of ZnO nanoparticles-an antimicrobial study. Sci Technol Adv Mater. 2008;9:035004. doi: 10.1088/1468-6996/9/3/035004.
- Wang L, He H, Yu Y, et al. Morphology-dependent bactericidal activities of Ag/CeO2 catalysts against Escherichia coli. J Inorg Biochem. 2014;100:45–53. doi: 10.1016/j.jinorgbio.2014.02.016.