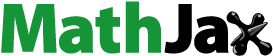
Abstract
From the last several decades, the origination of electrochemical sensors for neurotransmiters has dawned as a research desk of notable significance, that fascinated a massive attentiveness ascribed to its high selectivity, sensitivity and fast response. The current work was succeeded the advancement of electrochemical sensors for the detection of a neurotransmitter dopamine (DA) by the tavaborole drug modified carbon paste electrode (MCPE) by cyclic voltammetric (CV) and linear sweep voltammetric (LSV) techniques. Many different parameters was studied such as sweep rate, varied concentration, pH study, interference study and stability. The simultaneous investigation of DA in the existence of ascorbic acid (AA) and uric acid (UA) was studied. The limit of detection (LOD) and limit of quantification (LOQ) was calculated. This method was successfully applied for the investigation of DA in human blood serum and also in injection sample. Tavaborole drug modified electrode shows promising electrochemical sensing of DA.
1. Introduction
Neurotransmitters acts as a messengers of neurologic information. DA is a crucial biomolecule and catecholamine neurotransmitter, Aspect having great worth to study physiological function, diagnosis and treatment of mental sickness, toxicology screening and food hygiene. Thus, the accurate spotting of dopamine in biological fluids is very necessary (Ashoka, Swamy, and Jayadevappa Citation2017; Fredj et al. Citation2020; Fu, Sheng, and Zheng Citation2017; Pandey et al. Citation2020; Pradhan et al. Citation2014; Rajendrachari et al. Citation2022). Some common carbon based electrodes are used for the detection of DA in micromolar concentration. Carbon based electrodes show good electron transfer for neurotransmitters. DA is also called as 3,4-dihydroxy phenyl ethylamine. DA is a biological, pharmacological and physiological eminent neurotransmitter and the main function of this is to transfer message for human brain in the mammalian central nervous, renal and hormonal system (Baig, Kawde, and Ibrahim Citation2020; Cao, Puthongkham, and Jill Venton Citation2019; Chen et al. Citation2021; Li et al. Citation2019; Polepalli, Uttam, and Rao Citation2020; Yuan et al. Citation2022). The concentration of DA plays a highly valued role in human metabolism. Even the smallest variation leads to diseases like Parkinson’s disease, Alzheimer’s disease, Schizophrenia, Hentington’s disease (Liu et al. Citation2012; Liu, Zhua, and Yang Citation2014; Shashikumara and Swamy Citation2020; Zestos et al. Citation2015). Therefore it is very important to investigate DA and also cost effectual noticing methods are very much imperative. Many conventional procedure are there for the analysis of DA such as spectrophotometry, electrochemical methods etc. Among them electrochemical methods are used because of its cost effectiveness and efficiency. Furthermore, by electrochemical methods the quantification of eventually oxidisable phenolic hydroxyl groups present in dopamine was also achieved (Cervini, Mattioli, and Cavalheiro Citation2019; Guo et al. Citation2021; Liao et al. Citation2014; Martí et al., Citation2010; Rattanaumpa, Maensiri, and Ngamchuea Citation2022). Three different electrodes were used in cyclic voltammetry they are carbon paste electrode, glassy carbon paste and pencil graphite electrode. Compared to other electrodes carbon paste electrode was choosen because preparation and modification of the electrode was easy, more conducting facility, large potential window (Rajendrachari, Arslanoglu, et al. Citation2023; Rajendrachari, Basavegowda, et al. Citation2023). Here bare carbon paste electrode (BCPE) usually face a problem of interference in substances like AA and UA which have the same oxidation potential similar to DA which is contained in human serum and these are crucial biomolecules. In addition to this the decry of bare electrodes also deteriorates the sensing property, due to the secondary reactions after earlier electron transfer, which produce insulating species that shut off the surface of electrodes. As a result many researchers admire to develop sensors by modifying electrodes leads to solve this problem of interference, improves electron transfer rate and reactive sites and also improves selectivity and sensitivity of the detection substance (Bagherzadeh, Mozaffari, and Momeni Citation2015; He et al. Citation2022; Li et al. Citation2020; Sobahi et al. Citation2022; Xu et al. Citation2018; Zhang et al. Citation2011; Zhong et al. Citation2015).
Tavaborole drug is an antifungal agent used for the treatment of onychomycosis, it is a fungal infection of the nail. This drug is used as a modifier in this work.
In this study, we modified CPE by Tavaborole drug by electropolymerisation method for the analysis of DA in the presence of AA and UA using CV and LSV techniques. The tavaborole MCPE separates the oxidation peaks of DA, AA and UA and also shows the favourable intensification of the peak currents compared to BCPE and also this developed sensor exhibit a magnificent ability to find out DA in the existence of a high concentration of AA and UA. This MCPE helps to get a detailed electrochemical study for sensing of DA.
2. Experimental part
2.1. Chemical and apparatus
The chemicals used in this work are dopamine, uric acid, ascorbic acid, tavaborole, phosphate buffer(PBS) (NaH2PO4, Na2HPO4), silicon oil were bought from Sigma Aldrich. CH660c electrochemical workstation was used for the electrochemical studies. This consists of three-electrode system working, reference and auxiliary electrode.
2.2. Preparation of BCPE
By handmixing 70:30 of graphite powder and silicon oil for about 30–40 minutes to become a consistent paste. Then it was fill up to the hollow surface of a teflon tube about 3 mm inside diameter which was self prepared tube. The prepared tube was glazed on a butter paper to acquire a smoothened surface. Copper wire was connected at the terminate point of the tube which provides electrical support.
2.3. Preparation of MCPE
BCPE was prepared as discussed above. After that it was modified by tavaborole drug by electropolymerisation method. A cyclic voltammogram was obtained for 1 mM Tavaborole in 0.1 M NaOH at 20 multiple cycles with a scan rate of 0.05 Vs−1. A thin layer of tavaborole was absorbed on the surface of the electrode. The prepared electrode was known to be a Tavaborole modified carbon paste electrode.
3. Optimisation of the determination conditions
3.1. Electropolymerisation of tavaborole drug
According to the study, the MCPE was more stable and shows a good current response towards the redox species. Hence the fabrication of electrode is very much necessary. By electropolymerisation method, the BCPE was modified by the tavaborole drug. 1 mM tavaborole containing supporting electrolyte (0.1 M NaOH) swept between the potential range −0.4 V to 1.2 V for 20 successive cycles with the ʋ (scan rate) of 0.1 V/s (). During this process, with the increase in the potential cycling, the voltammogram decreases. This manifest the tavaborole film was settled on the electrode surface. To eliminate the physically absorbed material the modified electrode was immersed in a double distilled water. The tavaborole structure was shown in Scheme 1.
Figure 1. (A) Tentative CVs for the preparation of tavaborole/CPE. The electrolytic cell contains 1 mM tavaborole in 0.1 M NaOH at 20 multiple cycles with a scan rate of 0.05 Vs−1. (B) Relationship between anodic peak current and a total number of polymerisation cycles.
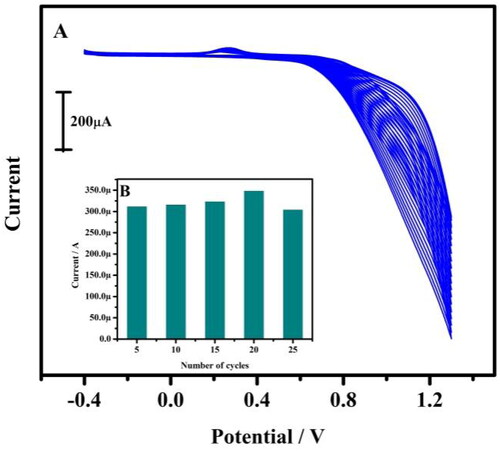
For the electrochemical response of TBMCPE, the girth of the film absorbed on the surface of the electrode enact an important role. More the thickness of the electroactive layer, it prevents the electron transfer process. Hence the number of polymerisation cycles was checked from 5 to 25 cycles. The plot of anodic peak current (Ipa) versus number of cycles was plotted (). The current gradually increased from 5 to 20 cycles and then at 25 cycles the current was decreased. According to this study, the maximum current response was shown at 20 successive cycles. Hence it was chosen for the electropolymerisation of tavaborole for the entire study in this work.
3.2. pH effect
The pH issue on TBMCPE was inquested from pH 5.8 to pH 7.8 for 10 μM DA in 0.1 M PBS. The pH of the supporting electrolyte has manifested a substantial impact on the current and the oxidation peak potential of DA. By increase in the pH, the peak current was also increased. At pH 7.4 maximal current was procured () and a Graph of anodic peak current (Ipa) versus pH of the solution for MCPE prepared with tavaborole was shown in (inset ). The pH was also shows a remarkable effect on the peak potential of the DA. The peak potential shifts from 0.1 V to −0.1 V as an increase in the pH from 5.8 to 7.8. With the increament in the pH the peak potential moved towards negative potential. By plotting graph of Epa versus pH, shows a very good linearity (). The slope obtained from this plot is 57 mV per pH unit. It was close to the theoretical value of 59 mV per pH unit (da Silva et al. Citation2021; Shashikumara et al. Citation2021; Xu et al. Citation2018). The slope informs that there is a equal protons and electrons are participating in the electrochemical reaction of DA on the TBMCPE surface (Scheme 2).
3.3. Electrochemical characterisation of TBMCPE using potassium ferrocyanide system
In the electrochemical cell, a newly primed 1 mM potassium ferrocyanide and 1 M potassium chloride was taken. The cyclic voltammogram was recorded for both BCPE and TBMCPE at a scan rate of 0.05 V/s. The current response was enhanced at TBMCPE. The peak current was more at TBMCPE than BCPE (). For the potassium ferrocyanide system the ʋ was increased from 0.05 V/s to 0.5 V/s to at TBMCPE to calculate the active surface area of the electrode. It was calculated by using the Randles-Sevick equation, EquationEquation (1)(1)
(1) (Arpitha, Kumara Swamy, and Shashikumara Citation2023; Chetankumar, Kumara Swamy, and Sharma Citation2019; Sharath Shankar et al. Citation2009; Shashikumara, Kumara Swamy, and Sharma Citation2020; Xia, Wang, and Wang Citation2020).
(1)
(1)
Figure 3. Cyclic voltammogram of 1 mM potassium ferrocyanide at BCPE (coloured black) and at tavaborole MCPE (coloured red) in 1 M KCl at a scan rate of 0.05 Vs−1.
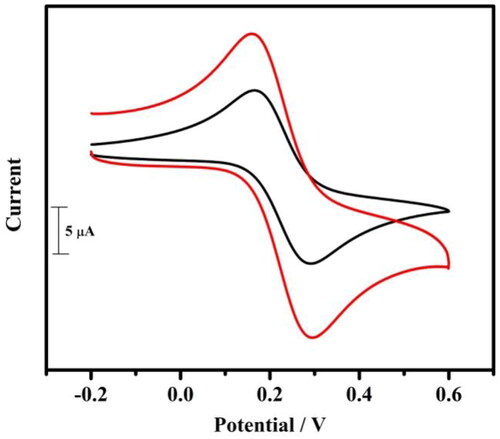
Where A is the area of electrode, Ipa is the anodic peak current, C0 is concentration, n is the number of electron changes, D0 is the diffusion coefficient, ʋ is the scan rate in mV/s.
The active surface area was accomplished to be 0.0339 cm2 (BCPE) and 0.0638 cm2 (TBMCPE).
3.4. Electrochemical response of DA at TBMCPE
The investigation of the electrochemical response of DA at BCPE and TBMCPE was recorded by cyclic voltammetric technique. The voltammogram was recorded for 10 μM DA at 0.2 M PBS of pH 7.4 with a scan rate of 0.05 V/s for both BCPE (dotted line) and TBMCPE (solid line) (). When compared to the TBMCPE the sensitivity of the voltammetric response was low at BCPE. For TBMCPE a well defined redox wave of DA was observed with Epa and Epc at 0.036 V and 0.019 V. Peak current was increased refinement in reversibility of electron transfer process was observed and also larger the surface area of TBMCPE. This result affirms that there is an efficient oxidation reaction of DA was achieved at TBMCPE.
3.5. Redox behaviour of dopamine
The electrode kinetics for DA was further analysed by the CV technique. The ʋ effect on DA was analysed by CV. The variation of ʋ has shown a remarkable effect on the peak current of the DA. The electro-oxidation of DA was examined by CV to survey the electrochemical behaviour of the modified electrode in PBS solution with DA in μM. The DA response was studied by varying its ʋ, conveyed that the redox peak current was proportional to the scan rate with negligible change in peak potential. The secured result showed that Ipa increases linearly with the increase in the ʋ from the range 10–100 mV/s (). The graph of Ipa versus square root of scan rate was plotted (). From the obtained graphs good linearity was shown with a correlation coefficient of R2 = 0.998, which stipulated that the electron transfer reaction of DA at the electrode surface is adsorption controlled process.
Figure 5. (A) Cyclic voltammogram of 10 µM DA in 0.2 M PBS of pH 7.4 at tavaborole MCPE with varied scan rate. (B) Graph of anodic peak current versus scan rate
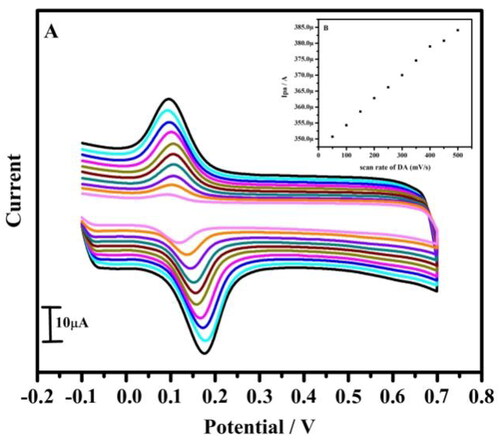
From the experimental peak potential difference (ΔEp) data, the k0 value was examined by using EquationEquation (2)(2)
(2) (Chetankumar, Kumara Swamy, and Sharma Citation2019; Sharath Shankar et al. Citation2009; Shashikumara, Kumara Swamy, and Sharma Citation2020; Xia, Wang, and Wang Citation2020), k0 value obtained from the scan rate 0.05–0.5 mV/s for the tavaborole MCPE exhibited by increasing scan rate the heterogeneous rate constant decreases (). This was used for such voltammograms whose ΔEp value are greater than 10 mV.
(2)
(2)
Table 1. Variation of the voltammetric parameters gathered from the plots shown in as a function of the potential sweep rate.
3.6. Effect of concentration
For the further investigation of the electrocatalytic performance of the TBMCPE, we further examined the linear concentration range and the limit of detection and quantification of the modified electrode for the detection of the least concentration of DA by cyclic voltammetric technique. By change in the concentration from 10 to 80 μM, it can be seen that the oxidation peak current of DA in 0.1 M PBS of pH 7.4 increases linearly (). The graph of Ipa versus concentration was plotted (), which also shows better linearity with with correlation coefficient of 0.997. This indicates that the peak current and concentration of the analyte are dependent to each other.
Figure 6. (A) Cyclic voltammogram of 10 µM DA in 0.2 M PBS of pH 7.4 at tavaborole MCPE with varied concentration at a scan rate of 0.05 Vs−1. (B) Graph of anodic peak current versus concentration.
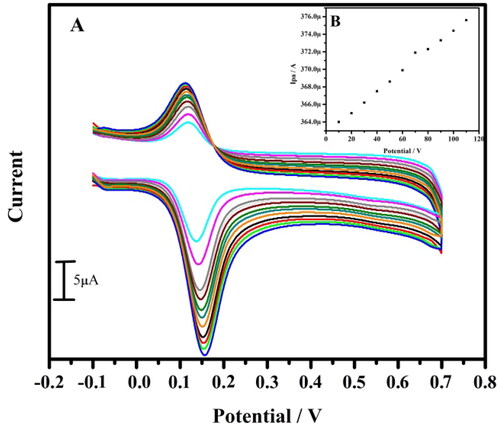
The LOD and LOQ was calculated by EquationEquation (3)(3)
(3) and Equation(4)
(4)
(4) .
(3)
(3)
(4)
(4)
The LOD was 5.28 μM and LOQ was 17.54 μM. The present work shows good performance which is tabulated in .
Table 2. Comparison of limit of detection with different modified electrodes and tavaborole-modified CPE.
3.7. Simultaneous study of DA in presence of AA and UA
The cyclic voltammogram of DA in the presence of AA and UA were recorded to study the electrochemical behaviour of DA at bare CPE and TBMCPE. It was investigated by Cyclic voltammetry and linear sweep voltammetric techniques. The voltammetric response of DA at BCPE and TBMCPE by CV and LSV was shown in and . The BCPE shows overlapped peaks and the separation of those analytes were difficult but in MCPE a well-distinct peak separation with an increase in the current response for the selective oxidation was shown. The oxidation peak potentials were detected at 0.033 V DA, 0.16 V AA, 0.10 V UA in 0.1 M PBS of pH 7.4 at TBMCPE (CV). In the same condition, the LSV also resolves the problem of overlapping peaks at TBMCPE. The solved LSV peaks of DA, AA, UA were located at 0.0039 V DA, 0.14 V AA, 0.12 V UA. This obtained results reveals that a resolved oxidation peak obtained for DA in the presence of AA and UA at TBMCPE.
3.8. Interference
For the examination of the detection achievement of the modified electrode in 0.1 M PBS of pH 7.4 mixed with DA, AA and UA was studied by LSV technique. We examined its anti-interference ability by adding DA continuously from 10 to 70 μM solution mixed with 10 μM AA and 10 μM UA. Even after the addition of DA the modified electrode still detect the DA at its higher concentration without interfering with AA and UA (). This result shows the oxidation peak of these analytes occurs independently and the issue of interference was demoralised by TBMCPE.
3.9. Stability
The standard electrodes commonly bear from low stability over long duaration. Hence the stability of the TBMCPE was checked for determining DA (10 μM) in 7.4 pH PBS at ʋ of 0.05 mV/s for 30 successive cycles. It was found that the redox peak currents were stable from the initial to the final cycle (). This shows the TBMCPE shows excellent stability and reproducibility towards electroactive species.
3.10. Application of the developed sensor
In the interest of determination of the applicableness of the propound method for the discovery of DA in human blood serum and in injection sample carried out by the standard addition method. The recovery percentage secured was shown in (). The DA concentration was varied from 10 to 30 μM in the real sample and TBMCPE was the retaliation of the oxidation peak current of DA. This evidence shows that the propounded method shows good recovery and the TBMCPE could be applied for the real sample analysis.
Table 3. Determination of DA in human blood serum and drug injection sample (n = 3).
4. Conclusion
Prepared tavaborole MCPE shows an effectual electrochemical sensing approaching the detection of DA in the presence of AA and UA by CV and LSV techniques. The TBMCPE shows a less heterogeneous constant at 50 mV/s towards the DA, this affirms that the modified electrode absorbed by DA. LOD and LOQ was found to be 5.28 μM and 17.54 μM. The investigation of pH studies reveals that an equal number of protons and electrons involved in the detection of DA. From the scan rate study the kinetic of the electrode process was proved to be adsorption controlled. The oxidation peak-to-peak separation of DA, AA, UA individually was good enough to detect electrochemically. The problem of interference was well resolved by Tavaborole MCPE. Therefore this modified electrode was used as an analytical tool for the tenacity of Dopamine in real samples. This studied parameter result was enough for the evolution of electrochemical sensors for Dopamine and the devoted neurotransmitters.
Disclosure statement
No potential conflict of interest was reported by the author(s).
References
- Arpitha, S. B., B. E. Kumara Swamy, and J. K. Shashikumara. 2023. “An Efficient Electrochemical Sensor Based on ZnO/Co3O4 Nanocomposite Modified Carbon Paste Electrode for the Sensitive Detection of Hydroquinone and Resorcinol.” Inorganic Chemistry Communications 152: 110656. https://doi.org/10.1016/j.inoche.2023.110656
- Ashoka, N. B., B. E. K. Swamy, and H. Jayadevappa. 2017. “Nanorod TiO2 Sensor for Dopamine: A Voltammetric Study.” New Journal of Chemistry 41 (20): 11817–11827. https://doi.org/10.1039/C7NJ02188G
- Bagherzadeh, M., S. A. Mozaffari, and M. Momeni. 2015. “Fabrication and Electrochemical Fabrication Characterization of Dopamine Sensing Electrode Based on Modified Grapheme Nanosheets.” Analytical Methods 7 (21): 9317–9323. https://doi.org/10.1039/C5AY02284C
- Baig, N., A.-N. Kawde, and M. Ibrahim. 2020. “Efficient Ionic Medium Supported Reduced Graphene Oxide-Based Sensor for Selective Sensing of Dopamine.” Materials Advances 1 (4): 783–793. https://doi.org/10.1039/D0MA00322K
- Cao, Q., P. Puthongkham, and B. Jill Venton. 2019. “Review: new Insights into Optimizing Chemical and 3D Surface Structures of Carbon Electrodes for Neurotransmitter Detection.” Analytical Methods 11 (3): 247–261. https://doi.org/10.1039/C8AY02472C
- Cervini, P., I. A. Mattioli, and E. T. G. Cavalheiro. 2019. “Developing a Screen-Printed Graphite Polyurethane Composite Electrode Modified with Gold Nanoparticles for the Voltammetric Determination of Dopamine.” RSC Advances 9 (72): 42306–42315. https://doi.org/10.1039/c9ra09046k
- Chen, X., N. Li, Y. Rong, Y. Hou, Y. Huang, and W. Liang. 2021. “Wenting Liang, b-Cyclodextrin Functionalized 3D Reduced Graphene Oxide Composite-Based Electrochemical Sensor for the Sensitive Detection of Dopamine.” RSC Advances 11 (45): 28052–28060. https://doi.org/10.1039/d1ra02313f
- Chetankumar, K., B. E. Kumara Swamy, and S. C. Sharma. 2019. “Poly (Benzoguanamine) Modified Sensor for Catechol in Presence of Hydroquinone: A Voltammetric Study.” Journal of Electroanalytical Chemistry 849: 113365. https://doi.org/10.1016/j.jelechem.2019.113365
- Corona-Avendaño, S., M. T. Ramírez-Silva, M. Palomar-Pardavé, L. Hernández-Martínez, M. Romero-Romo, and G. Alarcón-Ángeles. 2010. “Influence of CTAB on the Electrochemical Behavior of Dopamine and on Its Analytic Determination in the Presence of Ascorbic Acid.” Journal of Applied Electrochemistry 40 (2): 463–474. https://doi.org/10.1007/s10800-009-0017-x
- da Silva, L. V., N. D. dos Santos, A. K. A. de Almeida, D. Di E. R. dos Santos, A. C. F. Santos, M. C. França, D. J. P. Lima, P. R. Lima, and M. O. F. Goulart. 2021. “A New Electrochemical Sensor Based on Oxidized Capsaicin/Multi-Walled Carbon Nanotubes/Glassy Carbon Electrode for the Quantification of Dopamine, Epinephrine, and Xanthurenic, Ascorbic and Uric Acids.” Journal of Electroanalytical Chemistry 881: 114919. https://doi.org/10.1016/j.jelechem.2020.114919
- Fredj, Z., M. Ben Ali, M. N. Abbas, and E. Dempsey. 2020. “Simultaneous Determination of Ascorbic Acid, Uricacid and Dopamine Using Silver Nanoparticles and Copper Monoamino-Phthalocyanine Functionalised Acrylate Polymer, Journal of.” Analytical Methods 12 (31): 3883–3891. https://doi.org/10.1039/D0AY01183E
- Fu, Y., Q. Sheng, and J. Zheng. 2017. “The Novel Sulfonated Polyaniline-Decorated Carbon Nanosphere Nanocomposites for Electrochemical Sensing of Dopamine.” New Journal of Chemistry 41 (24): 15439–15446. https://doi.org/10.1039/C7NJ03086J
- Goyal, R. N., and S. P. Singh. 2008. “Simultaneous Voltammetric Determination of Dopamine and Adenosine Using a Single-Walled Carbon Nano Tube – MCPE.” Carbon 46 (12): 1556–1562. https://doi.org/10.1016/j.carbon.2008.06.051
- Guo, H., L. Sun, M. Yang, M. Wang, N. Wu, T. Zhang, J. Zhang, F. Yang, and W. Yang. 2021. “A Novel Electrochemical Sensor Based on TAPT-TFP-COF/COOH-MWCNT for Simultaneous Detection of Dopamine and Paracetamol, Journal of.” Analytical Methods 13 (42): 4994–5002. https://doi.org/10.1039/D1AY01537K
- He, C., M. Tao, C. Zhang, Y. He, W. Xu, Y. Liu, and W. Zhu. 2022. “Microelectrode-Based Electrochemical Sensing Technology for in Vivo Detectionof Dopamine: Recent Developments and Future Prospects.” Critical Reviews in Analytical Chemistry 52 (3): 544–554. https://doi.org/10.1080/10408347.2020.1811946
- Kamyabi, M. A., and F. Aghajanloo. 2009. “Electrocatalytic Response of Dopamine at a CPE Modifified with Ferrocene.” Roat Che Act 82: 599–606.
- Li, N., C. Nan, X. Mei, Y. Sun, H. Feng, and Y. Li. 2020. “Electrochemical Sensor Based on Dual-Template Molecularly Imprinted Polymer and Nanoporous Gold Leaf Modified Electrode for Simultaneous Determination of Dopamine and Uric Acid.” Mikrochimica Acta 187 (9): 496. https://doi.org/10.1007/s00604-020-04413-5
- Li, S., Y. Ma, Y. Liu, G. Xin, M. Wang, Z. Zhang, and Z. Liu. 2019. “Electrochemical Sensor Based on a Three Dimensional Nanostructured MoS2 nanospherePANI/Reduced Graphene Oxide Composite for Simultaneous Detection of Ascorbic Acid, Dopamine and Uric Acid.” RSC Advances 9 (6): 2997–3003. https://doi.org/10.1039/c8ra09511f
- Liao, C., M. Zhang, L. Niu, Z. Zheng, and F. Yan. 2014. “Organic Electrochemical Transistors with Graphene-Modified Gate Electrodes for Highly Sensitive and Selective Dopamine Sensors.” Journal of Materials Chemistry B 2: 191–200.
- Lin, K. C., T. H. Tsai, and S. M. Chen. 2010. “Performing Enzyme-Free H2O2 Biosensor and Simultaneous Determination for AA, DA and UA by MWCNT-PEDOT Film.” Biosensors & Bioelectronics 26 (2): 608–614. https://doi.org/10.1016/j.bios.2010.07.019
- Liu, G., J. Li, L. Wang, N. Zong, S. Yu, and F. Li. 2012. “Discrimination of Dopamine from Ascorbic Acid and Uric Acid on Thioglycolic Acid Modified Gold Electrode.” Analytical Methods 4 (3): 609. https://doi.org/10.1039/c2ay05838c
- Liu, X., H. Zhua, and X. Yang. 2014. “An Electrochemical Sensor for Dopamine Based on Poly(o-Phenylenediamine) Functionalized with Electrochemically Reduced Graphene Oxide.” RSC Advances. 4: 3706.
- Martí, M., G. Fabregat, F. Estrany, C. Alemán, and E. Armelin. 2010. “Nanostructured Conducting Polymer for Dopamine Detection.” Journal of Materials Chemistry 20 (47): 10652. https://doi.org/10.1039/c0jm01364a
- Pandey, P. K., K. Rawat, T. Prasad, and H. B. Bohidar. 2020. “Multifunctional, Fluorescent DNA-Derived Carbon Dots for Biomedical Applications: Bioimaging, Luminescent DNA Hydrogels, and Dopamine Detection, Journal of Materials Chemistry. B, 8 (6), 1277. https://doi.org/10.1039/c9tb01863h
- Polepalli, S., B. Uttam, and C. P. Rao. 2020. “Protein–Inorganic Nano Hybrid Sheets of Pd Embedded BSA as a Robust Catalyst in Water for Oxidase Mimic Activity and C–C Coupling Reactions, and as a Sustainable Material for Micromolar Sensing of Dopamine.” Materials Advances 1 (6): 2074–2083. https://doi.org/10.1039/D0MA00512F
- Pradhan, T., H. S. Jung, J. H. Jang, T. W. Kim, C. Kang, and J. S. Kim. 2014. “Chemical Sensing of Neurotransmitters.” Chemical Society Reviews 43 (13): 4684–4713. https://doi.org/10.1039/c3cs60477b
- Pruneanu, S., A. R. Biris, F. Pogacean, C. Socaci, M. Coros, M. C. Rosu, F. Watanabe, and A. S. Biris. 2015. “The Influence of Uric and Ascorbic Acid on the Electrochemical Detection of Dopamine Using Graphene-Modified Electrodes.” Electrochimica Acta 154: 197–204. https://doi.org/10.1016/j.electacta.2014.12.046
- Rajendrachari, S., G. Kudur Jayaprakash, A. Pandith, A. C. Karaoglanli, and O. Uzun. 2022. “Electrocatalytic Investigation by Improving the Charge Kinetics between Carbon Electrodes and Dopamine Using Bio-Synthesized CuO Nanoparticles.” Catalysts 12 (9): 994. https://doi.org/10.3390/catal12090994
- Rajendrachari, S., H. Arslanoglu, A. Yaras, and S. M. Golabhanvi. 2023. “Electrochemical Detection of Uric Acid Based on a Carbon Paste Electrode Modified with Ta2O5 Recovered from Ore by a Novel Method.” ACS Omega 8 (49): 46946–46954. https://doi.org/10.1021/acsomega.3c06749
- Rajendrachari, S., N. Basavegowda, R. Vinaykumar, D. Narsimhachary, P. Somu, and M. Lee. 2023. “Electrocatalytic Determination of Methyl Orange Dye Using Mechanically Alloyed Novel Metallic Glass Modified Carbon Paste Electrode by Cyclic Voltammetry.” Inorganic Chemistry Communications 155: 111010. https://doi.org/10.1016/j.inoche.2023.111010
- Rattanaumpa, T., S. Maensiri, and K. Ngamchuea. 2022. “Microporous Carbon in the Selective Electrooxidation of Molecular Biomarkers: uric Acid, Ascorbic Acid and Dopamine.” RSC Advances 12 (29): 18709–18721. https://doi.org/10.1039/d2ra03126d
- Sharath Shankar, S., B. E. Kumara Swamy, U. Chandra, G. Manjunatha, and B. S. Sherigara. 2009. “Simultaneous Determination of Dopamine, Uric Acid and Ascorbic Acid with CTAB Modified Carbon Paste Electrode.” International Journal of Electrochemical Science 4 (4): 592–601. https://doi.org/10.1016/S1452-3981(23)15166-2
- Shashikumara, J. K., and B. E. K. Swamy. 2020. “Electrochemical Investigation of Dopamine in Presence of Uric Acid Andascorbic Acid at Poly (Reactive Blue) Modified Carbon Paste Electrode: A Voltammetric Study.” Sensors International 1: 100008. https://doi.org/10.1016/j.sintl.2020.100008
- Shashikumara, J. K., B. E. Kumara Swamy, and S. C. Sharma. 2020. “A Simple Sensing Approach for the Determination of Dopamine by Poly (Yellow PX4R) Pencil Gtaphite Electrode.” Chemical Data Collections 27: 100366. https://doi.org/10.1016/j.cdc.2020.100366
- Shashikumara, J. K., B. Kalaburgi, B. E. K. Swamy, H. Nagabhushana, S. C. Sharma, and P. Lalitha. 2021. “Effect of RGO-Y2O3 and RGO-Y2O3:Cr3+ Nanocomposite Sensor for Dopamine.” Scientific Reports 11 (1): 9372. https://doi.org/10.1038/s41598-021-87749-z
- Sobahi, N., M. Imran, M. E. Khan, A. Mohammad, M. M. Alam, T. Yoon, I. M. Mehedi, M. A. Hussain, M. J. Abdulaal, and A. Jiman. 2022. “Facile Fabrication of CuO Nanoparticles Embedded in N-Doped Carbon Nanos Tructure for Electrochemical Sensing of Dopamine.” Bioinorganic Chemistry and Applications 2022: 6482133. https://doi.org/10.1155/2022/6482133
- Sunil Kumar Naik, T. S., M. Martin Mwaurah, and B. E. KumaraSwamy. 2018. “Fabrication of Poly (Sudan III) Modifified Carbon Paste Electrode Sensor for Dopamine: A Voltammetric Study.” Jeac 834: 73.
- Xia, C., N. Wang, and L. Wang. 2020. “Optical and Electro-Catalytic Properties of Bundled ZnO Nanowires Grown on an ITO Substrate.” Journal of Nanoparticle Research 12 (5): 1869–1875. https://doi.org/10.1007/s11051-009-9748-1
- Xu, G., Z. A. Jarjes, V. Desprez, P. A. Kilmartin, and J. Travas-Sejdic. 2018. “Sensitive, Selective, Disposable Electrochemical Dopamine Sensor Based on PEDOT-Modified Laser Scribed Graphene.” Biosensors & Bioelectronics 107: 184–191.
- Yuan, Y., S. Wang, P. Wu, T. Yuan, and X. Wang. 2022. “Lignosulfonate in Situ-Modified Reduced Graphene Oxide Biosensors for the Electrochemical Detection of Dopamine.” RSC Advances 12 (48): 31083–31090. https://doi.org/10.1039/d2ra05635f
- Zestos, A. G., C. Yang, C. B. Jacobs, D. Hensley, and B. J. Venton. 2015. “Carbon Nanospikes Grown on Metal Wires as Microelectrode Sensors for Dopamine, Journal of.” The Analyst 140 (21): 7283–7292. https://doi.org/10.1039/c5an01467k
- Zhang, F., Y. Li, Y. Gu, Z. Wang, and C. Wang. 2011. “One-Pot Solvothermal Synthesis of a Cu2O/Graphene Nanocomposite and Its Application in an Electrochemical Sensor for Dopamine.” Microchimica Acta 173 (1-2): 103–109. https://doi.org/10.1007/s00604-010-0535-6
- Zhong, M., Y. Teng, S. Pang, L. Yan, and X. Kan. 2015. “Pyrrole–Phenylboronic Acid: A Novel Monomer for Dopamine Recognition and Detection Based on Imprinted Electrochemical Sensor.” Biosensors & Bioelectronics 64: 212–218. https://doi.org/10.1016/j.bios.2014.08.083