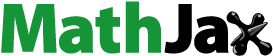
Abstract
Malting barley is an economically important crop, with strict quality standards. In some sectors of the industry, there is a bias against malting barley with blue aleurone. The rationale behind this bias is not clear. We therefore investigated the relationships between barley color and malting quality using a panel of fall-planted doubled haploid lines from the Oregon State University barley breeding program. The panel was assessed for malting and aleurone color traits using grain from the 2021 harvest and for aleurone color with grain from the 2022 harvest. The white and blue aleurone groups were found to be different for some malting traits across the entire panel of lines, but this was a result of selection history. When a biparental subset of the panel segregating for aleurone color was considered, differences between the color groups were minimal. A genome wide association study showed that the major QTL contributing to aleurone color (on the long arm of chromosome 4H) was independent of the malt quality QTL. It is concluded that there is no basis for a bias against blue aleurone: identifying new malting barley varieties should be based on their malting performance, not their color.
CORE IDEAS
The blues are all right – aleurone color and malting quality are controlled by different genes.
Observed relationships between aleurone color and specific malting quality traits are due to breeding history.
Barley varieties with specific malting quality profiles can be developed irrespective of aleurone color.
Introduction
Barley (Hordeum vulgare L) domestication occurred approximately 10,000 years ago with some of the earliest written records of the malting process dating back almost 6,000 years from ancient Sumer.[Citation1,Citation2] Since that time, malting has become a worldwide, multi-billion-dollar industry.[Citation3] According to an annual analysis conducted by the American Malting Barley Association in 2017 factoring in USDA-NASS data and survey data from their membership, over 60% of barley produced in the United States is destined for malting and brewing. Barley intended for malt receives a higher price premium compared to either feed or food barleys.[Citation4,Citation5] With malting barley being such an economically important crop, large amounts of resources are invested into the breeding of good malting varieties.
What constitutes a good malting variety can differ depending on the criterion used. There are differences in the specific recommendations depending on whether the goal is adjunct or all-malt.[Citation6] Both criteria require a high percentage of plump kernels, a high germination rate, high malt extract, low beta-glucan content, low grain protein, and low levels of hull detachment.[Citation6,Citation7] Specific ranges for free amino nitrogen, soluble/total protein, diastatic power, and alpha-amylase differ between the adjunct and all-malt criteria.[Citation6] One criterion not explicitly listed is aleurone color. Barleys with a blue aleurone are not preferred by the malting industry and in some cases, are withdrawn from production entirely.
The Australian malting variety Henley is an example of a suitable malting line being rejected based the presence of blue aleurone. In 2016, Heritage Seeds stopped selling Henley barley because a blue aleurone was noted during commercial production.[Citation8] This withdrawal occurred despite Henley meeting malting and agronomic standards during testing. The reasoning behind the aversion to blue aleurone is not well defined. Some have stated that barley with blue aleurone is not visually appealing.[Citation8] Baxter and O’Ferrell posit that the aversion to blue aleurone could be due to the structural relationships between the anthocyanins responsible for the blue coloration of the aleurone, and the colorless anthocyanogens that play a role in haze formation, haze color, and flavor.[Citation9] Other publications cite that blue aleurone is an undesired characteristic in malting, but do not address the topic in-depth.[Citation10,Citation11]
Expression of blue pigment in the aleurone of barley grain is a function of anthocyanin accumulation. The biological impacts of anthocyanins are wide-ranging. Anthocyanins offer protection from UV radiation, antioxidant activity, and can even provide antiviral effects.[Citation10,Citation12–14] The genetic basis of blue anthocyanin accumulation is complex. Five complementary genes referred to as Blx1, Blx2, Blx3, Blx4, and Blx5 are responsible for the blue aleurone phenotype in barley.[Citation15] The Blx1, Blx3, and Blx4 loci are physically linked and found on the long arm of chromosome 4H while the Blx2 and Blx5 loci are on 7H.[Citation10]
Identification of causal genes and chromosomal regions important in barley can be conducted through a genome wide association study (GWAS). GWAS has been found to be an effective tool for identifying relevant quantitative trait loci (QTL) in diverse populations and has been used to great success in barley genomic studies.[Citation16–18] GWAS via mixed modeling can be expanded to account for population structure and relatedness between genotypes.[Citation19] This population structure could otherwise lead to false positives and incorrect conclusions as to the genetic basis of traits.[Citation20] GWAS can also be useful in identifying potentially pleiotropic loci, or loci with an impact on multiple traits; however, standard methods are not sufficient to distinguish pleiotropy from linkage disequilibrium.[Citation21]
There were three primary objectives addressed as part of the present study. I) Assess a panel of fall planted barley for malt and aleurone color characters. II) Investigate relationships between aleurone color and malting phenotypes. III) Use GWAS to identify QTL relevant to aleurone color and malting characteristics. Characterization of the panel provides valuable information that can be used in the selection of highly productive malting barley varieties. Exploring the genetic and phenotypic relationships between aleurone color and malting quality of the assessed germplasm will help determine the effects of blue aleurone on malting quality.
Experimental
Germplasm selection and experimental environments
The starting point for this research was a set of 1,007 doubled haploids developed by the Oregon State University winter/facultative malting barley breeding program. The doubled haploids, produced from F1 plants using anther culture,[Citation22] traced to ten different crosses. The number of doubled haploids per cross ranged from 8 to 324. Aleurone color was not considered in designing the crosses. Based on pedigree, 67% of the doubled haploids would be expected to be white aleurone, assuming monogenic control of the trait. These doubled haploids, and a set of malting barley checks, were planted in the fall of 2020 at Corvallis, Oregon USA (44° 38′ 03″/123° 11′ 24″) in a single replicate screening nursery using small plots measuring 1.5 m × 1.5 m (2.25m2). Plots were planted using a Hege head row drill and managed using standard agronomic practice for this location, as described in Supplemental Table S1. In the summer of 2021, prior to harvest, phenotypic selections were made based on plant height, disease symptoms, and lodging resistance. Aleurone color was not considered in making these selections. Only the phenotypic selections were harvested, using a Wintersteiger Classic plot combine. Seed from these selections was subsequently assessed for the percentage of plump seed (the fraction of clean grain remaining on a 2.38 mm screen after two minutes of shaking on a Pfeuffer Sortimat). Test weight (g/l) was determined by weighing the mass of clean grain that fit into a one-liter container. Grain protein (percentage) was measured using a Foss Infratec NOVA near-infrared grain analyzer. A further round of selection was applied based on these criteria, leading to a final set of 175 selected doubled haploids (designated as Cycle 5). Aleurone color was not considered in making these selections. This Cycle 5 seed was used for malting (see Malting quality) at the Hartwick Center for Craft Food and Beverage (https://www.hartwick.edu/about-us/center-for-craft-food-and-beverage/), for characterization of aleurone color (see Aleurone color), and for planting in the fall of 2021 at Corvallis, Oregon using a single replicate of plots measuring 1.5 × 4.5 m (6.75m2). Plots were planted using a Wintersteiger XL yield trial drill and managed using standard agronomic practice for this location, as described in Supplemental Table S1. All plots were harvested, using a Wintersteiger Classic plot combine. Multiple agronomic traits were assessed in these plots, including grain yield, reaction to prevailing diseases, lodging, plant height, grain protein, percentage of plump seed, and test weight. Grain from each plot was also used for characterization of the growing season effect on aleurone color (see Aleurone color). Based on the agronomic data, but not aleurone color, forty-seven doubled haploids were selected for malting quality assessment and further agronomic assessment in the 2022/2023 season. Of these, 21 are blue aleurone.
Table 1. Total number of genotypes (#G), number of blue genotypes (#B) identified by color values measured via colorimeter (bB, see materials and methods) in the 2021 season of the present study, and proportion of blue genotypes (#B/#G) for each family observed in this study.
Phenotyping
Aleurone color
Three methods were used for assessing aleurone color in the 2021 samples: visual assessment, colorimeter assay of untreated grain, and colorimeter assay of grain treated with acid. For all methods, 70 g of grain of each genotype was de-hulled using a pearling machine (model 17810, Strong-Scott, Seedburo Equipment Co., Chicago, IL) for 15 sec. For the visual test, genotypes were classified as dark blue (1), blue (2), medium or light blue (3) and white (4) by four different people (technical reps). See for a visual representation of the scale used in the visual test.
Figure 1. Pearled grain used in the scoring of aleurone color with dark blue receiving a 1, blue receiving a 2, medium or light blue receiving a 3, and white receiving a 4.

Scores based on visual assessment for blue aleurone are referred to as intensity in this study. For the colorimeter assays (untreated and treated with acid) the L*, a* and b* chromatic values were measured with a Minolta CR-410 colorimeter (Konica Minolta Sensing Americas, Inc, NJ, USA). The L* value corresponds to the lightness (varying between 0 for white to 100 for black), a* expresses color values from green to red (negative values indicate green and positive values red), and b* represents values from blue to yellow (negative values indicate blue and positive values yellow). An acid treatment was also used, which involved immersion of the grain in 1% hydrochloric acid (HCl) to intensify color expression as described by Dhesi et al.[Citation23] Briefly, the grain was placed in a glass petri dish, to which 40 ml of 1% HCl was added, completely covering the grain. The grains in each dish were stirred with a glass rod to ensure complete immersion. After 16 h, the remaining liquid was removed by pouring the contents of each dish through filter paper. The grain was then assayed using the colorimeter. Chromatic values of untreated grain are denoted as LB, aB and bB in this work. The “B” in LB, aB, and bB represent “Blue” as values of untreated grain are measuring the blue color of the aleurone directly. Values of treated grain are denoted as LP, aP and bP. The “P” in LP, aP, and bP represents “Pink” as the reaction between anthocyanin and HCl leads to a pink hue in the aleurone. Based on the results of the 2021 assays, in 2022 only pearled untreated grain was assayed using the colorimeter. No visual assessments were made on the grain harvested in 2022.
Total anthocyanin content
Anthocyanin content was measured as µg cyanidin 3-glucoside/g sample for each of the genotypes determined to have a blue aleurone based on the visual assessment of the 2021 samples. The total anthocyanins were analyzed according to the method proposed by Abdel-Aal and Hucl.[Citation24] Briefly, a 1.6 mL of solution of 85% ethanol and 15% HCl 1 M was added to 100 mg of flour sample. Then, samples were mixed for 15 min and centrifuged at 27,200 × g for 30 min. Absorbance readings at 536 nm were taken using a microplate reader (Molecular Device VersaMax, USA). The results were expressed as μg of cyanidin-3 glucoside equivalents/g sample.
Malting quality
Assessment of malt quality and water sensitivity was performed by the Hartwick College Center for Craft Food and Beverage (CCFB). The ASBC methods[Citation25] were used to collect data on grain protein (GP; %), grain moisture (GM; %), 4 mL test results (%), 8 mL test results (%), malt moisture (MM; %), friability (FR; %), fine extract (FE; %), wort color (WC; °), beta-glucan (BG; ppm), total malt protein (MP; %), soluble malt protein (SP; %), free amino nitrogen (FAN; mg/L), diastatic power (DP; °L), alpha amylase (AA; DU), pH, partially unmodified grains (PUG; %), and wholly unmodified grains (WUG; %). Before malt analysis was conducted the proportion of blue kernels in each sample was visually assessed by counting the number of blue and number of white kernels in a sample of 100 kernels (referred to as “proportion” in the following analysis). Water sensitivity (WS) was measured as the difference between 4 mL and 8 mL germination test results (ASBC method Barley-3C). Of the measures of malt quality collected, FR, FE, WC, BG, MP, S/T, FAN, DP, AA, and WS are used in the following analysis. This reduces the computation burden and increases the readability of results while capturing the majority of the relevant malt quality data available for the Cycle 5 population.
Analysis of phenotypic data
Correlation of aleurone color and malting traits
Pearson correlations between aleurone color and ten of the assessed malting traits were calculated and displayed via correlation plots in the R software version 4.0.3[Citation26] in the corrplot package version 0.92.[Citation27] Correlations between traits are based on pairwise-complete observations only. Correlation analysis was performed both across all pedigrees of the Cycle 5 population and within the DH120304/Flavia pedigree separately to remove genetic background effects due to the segregation of aleurone color in doubled haploids derived from this pedigree.
Comparison of aleurone color and malting traits irrespective of genetic background
A linear model was fit in the R software to test the effect of blue aleurone on the ten malting traits used to represent malting quality. The bB value was used to separate the blue from the white aleurone barleys (and confirmed via the intensity assessment). A one-way analysis of variance (ANOVA) was used to determine significant effects of aleurone color on each of the parameters at the α = 0.05 level. Boxplots were also constructed in the R software to visually assess differences between trait distributions for each of the aleurone color categories. In the cases where a significant effect of aleurone color on any of the tested traits was identified in the ANOVA, a Fisher’s Least Significant Difference (LSD; Citation28] test was conducted to identify statistically significant differences between individual categories. Differences in the anthocyanin content for each of the different intensities of blue aleurone grains were also assessed.
Not all of the categories of aleurone color met the assumption of normality imposed by an ANOVA for each of the traits. In cases where departures from normality were detected in residual histograms or in qq-plots, a Box-Cox transformation on the data was used to meet this assumption.[Citation29] See and in the results section for specifics on the transformation used for each of the malting traits.
Table 2. Results from the ANOVA and variance component estimation of the across-year values.
Comparison of aleurone color and malting traits within a bi-parental subset
The above analysis for effects of blue aleurone regardless of pedigree was conducted again on a bi-parental subset of the Cycle 5 population (pedigree DH120304/Flavia). This was performed to control the effects of the genetic background on malting quality. As one parent had blue aleurone and one parent had white aleurone, the progenies were segregating for aleurone color. Only this subset was included in this portion of the analysis, either because no segregation for aleurone color was observed in the other families or their population size was too small (). Performing the analysis in this way has the benefit of removing the effects of population structure on malting quality; however, the lowered population size does decrease statistical power.
Comparison of aleurone color traits across seasons
The LB, aB, and bB traits were measured across seasons allowing for an investigation of how aleurone color changed across years in a given location. A mixed model of the form:
(1)
(1)
was fit in the SAS software[Citation30] to determine the effects of genotype, year, and genotype by year. Where yijk is the observed value for genotype i, environment j, and technical replicate k. G is the fixed genetic main effect of genotype i, Y is the fixed main effect of year j, GY is the interaction term of genotype I in year j, R is the random effect of technical replicate k nested within year j with R ∼ N(0,
), and e is the residual term with e ∼ N(0,
). An ANOVA was used to assess the significance of each of these effects and their interaction at the α = 0.05 level. Model (1) was also fit as a random effects model to estimate the contributions of each of the effects to the observed variance of a given trait via restricted maximum likelihood (REML). Variance component estimates were used to calculate the broad-sense heritability on an entry-mean basis following the equation:
(2)
(2)
where
is the genetic variance,
is the genotype by year variance, and
is the residual variance all estimated in the random effects model (1). The y term is the number of years (two in this case), and r is the number of biological replicates per year (one in this case).
Analysis of genetic data and integration of genetic and phenotypic data
Genotyping
Genotyping was performed using the Illumina 50 K SNP Chip at the USDA-ARS genotyping laboratory in Fargo, ND. Monomorphic markers, markers with more than 10% missing data, markers with a minor allele frequency less than 5% were filtered out in Illumina’s Genome Studio version 2.0. Also, in Genome Studio, markers with low-quality calls (GenCall Score < 0.30) or a high proportion of heterozygotes (AB Frequency > 0.6) were filtered out. Linkage disequilibrium (LD) between pairs of markers was calculated as the correlation coefficient in the R package, SNPRelate.[Citation31] Markers in perfect LD (r2 = 1.0) were removed from the analysis. After filtering, there was marker data available for 179 individuals at 9,960 loci. Additionally, after filtering, missing markers were imputed by the mean of the population at each marker for the purposes of producing the realized additive relationship matrix, K, and for testing in GWAS. Fractional imputed values were used for constructing K, while values rounded to the nearest integer were used for testing in the GWAS.
Genome wide association study
A GWAS was performed using the GWASPoly package by Rosyara et al.[Citation32] in the R software to detect QTL for the assessed malting and aleurone color traits. The model used for this analysis included both the K matrix to account for marker-based kinship and principal components of the centered and scaled genotype data as fixed effects to capture additional population stratification.[Citation33] The mixed model equation for the GWAS was fit as:
(3)
(3)
where y is the n-length vector of observed phenotypic values (n is the total number of individuals), α is a one-length vector of the fixed SNP effect with incidence vector S of length 9,960, v is the five-length vector of fixed effects of each principal component (five PCs were maintained for this analysis, referred to as p) with matrix P relating each observation in y to its respective value in v via PCA scores, g is the n-length vector of polygenetic background effects with incidence matrix Z, and e is the vector of residual effects. Also, where Var(g)=
and Var(e)=I
. K is the n × n realized additive relationship matrix calculated as the cross product of the centered and scaled genotype scores for each genotype and σ2g is the genetic variance. I is an n × n identity matrix and σ2e is the error variance.
For the traits measured in more than one-year (i.e., LB, bB, and aB) model (3) was run three times. Once as written above for both 2021 and 2022 independently, and once with the term included to include data from both years in one analysis. The two-length vector b includes the fixed effect of each season with incidence matrix X. This allows for the detection of significant year-specific associations as well as associations relevant to the full dataset.
The number of principal components maintained was set at five for this analysis based on the results of a principal component analysis on the centered and scaled genotype data. The eigenvectors for each principal component (PC) were plotted in a bar plot, and the fewest number of PCs explaining the largest proportion of the observed variance were selected.
Markers were determined to be significantly associated with a given trait if they exceeded a threshold controlling the family-wise-error-rate at the α = 0.05 level. This threshold was set using a method similar to the Bonferroni correction, but accounting for the effective number of markers rather than the total number of markers used in the analysis.[Citation34] The associated marker reported for each QTL (Supplemental Table S2) is the highest scoring marker in a 150mB window. A monotone-decreasing plot of linkage disequilibrium present in the Cycle 5 panel plotted in GWASpoly was used to determine the window size.
Results
Phenotypic analysis
Characterization of aleurone color in the Cycle 5 population
describes the pedigrees, and presence of the blue aleurone phenotype for each of the Cycle 5 experimental genotypes used in this study. Four of the eight experimental families were segregating for aleurone color. The largest family, DH140963/Flavia, consisted completely of genotypes with blue aleurone. The second largest family, DH120304/Flavia, was segregating for aleurone color. Of the 53 doubled haploid genotypes from the DH120304/Flavia pedigree, 36 of them were white and 17 were blue ().
Check genotypes Wintmalt ((Opal × 3087/96) × 1922-23), Thunder (Wintmalt/Charles), Lightning (SHORT11-7 (TC6W265)/HERZ 29494/2991 (35)), and Endeavor (ORWM8406/Harrington) had blue, blue, white, and white aleurones respectively (as observed in year 2021 of the present study).
There was a difference in the distribution of bB values across genotypes between the 2021 and 2022 harvest seasons. In the 2021 season, there is a clear division in the genotypes expressing a blue aleurone and those with a white aleurone (). In the 2022 season (), this division is much less clear.
Correlation of aleurone color and malting traits
Pearson correlations between the different measures of aleurone color and anthocyanin content can be found in . It should be noted that a high intensity, b*, a*, or L* score indicate a whiter aleurone while a high proportion indicates more seeds with blue aleurone. This means that the negative correlation between proportion and intensity (or any of the other color measures) indicates a positive relationship with blueness of the aleurone and proportion of kernels with blue aleurone.
Figure 3. Pearson correlation matrix relating the different measures of grain aleurone color. All values were recorded in 2021 at minimum, with the LB, aB, and bB values also collected in 2022.

Pearson correlations between the bB measure of aleurone color and malting traits can be found in . It should be noted that a higher bB score indicates a whiter aleurone. This means that a positive correlation between bB and a given malting parameter indicates that darker blues have a negative relationship with the trait in question and vice versa. The left correlation matrix shows the relationship between traits across the entire Cycle 5 population. The right correlation matrix shows the relationship between traits within the DH120304/Flavia subset. Correlations between all measured traits across the entire Cycle 5 population can be found in Supplemental Figure S1.
Comparison of aleurone color traits across seasons
For the aleurone color traits LB, bB, and aB measured in both 2021 and 2022, a mixed model and a random effects model were fit to perform ANOVA and estimate variance components, respectively. Contributions of genotype, environment, genotype by environment, technical replicate within environment, and residual are displayed in along with the calculated broad-sense heritability.
Comparison of aleurone color and malting traits irrespective of genetic background
Boxplots of malting traits by the categorical visual score are displayed in . For brevity, plots of S/T, DP, FE, and BG are displayed. These are key malting parameters that display interesting comparisons between the blue and white genotypes tested.
Figure 5. Boxplots of A) S/T, B) DP, C) FE, and D) BG broken out by the observed aleurone color from the 2021 harvest season. Letters at the base of each plot are the result of a Fisher’s LSD Test to detect significant differences between groups. A jitter plot is overlaid on each boxplot to show exact data values. The Fisher’s Test for beta-glucan is based on values transformed via Box-Cox transformation to account for non-normality; however, the displayed data values are untransformed.

Results from the ANOVA and Fisher’s LSD analyses are presented in . Of the 10 tested malting traits, eight of them show significant differences between the category of aleurone color expressed in the grain. Total protein and fine extract were not different between aleurone color categories across the whole Cycle 5 population.
Table 3. Results from the ANOVA and Fisher’s LSD test comparing the performance of each observed aleurone color group with regards to ten of the measured malting traits.
There were also significant differences detected in the anthocyanin content of the different categories of blue barley aleurone. The dark blue, blue, and light blue aleurones had concentrations of 38.07, 30.00, and 24.74 µg cyanidin 3-glucoside/g sample, respectively. Each of these categories were significantly different from each other as determined by the Fisher’s LSD test. displays the relationships between the measured anthocyanin content, the bB value, and the visual intensity score.
Comparison of aleurone color and malting traits within a bi-parental subset
Boxplots of malting traits by the categorical visual score within the DH120304/Flavia pedigree are displayed in .
Figure 7. Boxplots of A) S/T B) DP, C) FE, and D) BG broken out by the observed aleurone color from the 2021 harvest season within the DH120304/Flavia Pedigree. Letters at the base of each plot are the result of a Fisher’s LSD Test to detect significant differences between groups. A jitter plot is overlaid on each boxplot to show exact data values. The Fisher’s Test for beta-glucan is based on values transformed via Box-Cox transformation to account for non-normality; however, the displayed data values are untransformed.

Few malting traits showed significant differences between the blue and white aleurone genotypes from the DH120304/Flavia subset (). Blue genotypes from this subset showed a significantly higher fine extract percentage compared to the white genotypes (a difference of 1% approximately). White genotypes from this subset showed a significantly higher diastatic power compared to the blue genotypes (a difference of 14°L approximately).
Table 4. Results from the ANOVA and Fisher’s LSD test comparing the performance of the blue and white aleurone color groups within the DH120304/Flavia subset.
Genome wide association study
Selected Manhattan plots from the GWAS analysis for color traits are presented in . Of the eight aleurone color traits assessed in this study, each of them identified a major-effect locus on the long arm of chromosome 4H. This major-effect QTL was persistent across both years of data for the LB, ab, and bB traits (the only traits measured across years). There were also a number of other QTL identified by individual measures of aleurone color or within individual years on 1H, 2H, 3H, the short arm of 4H, 5H, 6H, and 7H. Positions for the QTL associated with the bB measurement can be found in . The full list of identified color QTL with the name/position of the most significant marker, additive effect, and proportion of the phenotypic variance explained (R2) can be found in Supplemental Table S2. Manhattan plots for aleurone color traits with significant marker-trait associations not displayed in can be found in Supplemental Figure S2.
Figure 8. Manhattan plots with a significance threshold resulting from the GWAS analysis showing significant peaks for the aleurone color traits A) bB as measured from the 2021 harvested grain, B) bB as measured from the 2022 harvested grain, C) intensity of blue aleurone measured from the 2021 harvested grain, and D) proportion of grains with blue aleurone measured from the 2021 harvested grain.

Figure 9. Manhattan plots with a significance threshold resulting from the GWAS showing significant peaks for the malting traits A) FAN, B) WC, C) S/T, and D) AA.

Selected Manhattan plots from the GWAS analysis for malting traits are presented in . Of the 18 malting traits assessed in this study, significant marker-trait associations were identified for 11 of them. Six of these traits had QTL of large effect mapping to the long arm of chromosome 5H. Additional significant associations were found for individual traits across chromosomes 1H, 2H, 4H, 6H, and 7H. Positions for the QTL associated with malting traits can be found in . The full list of identified color QTL with the name/position of the most significant marker, additive effect, and proportion of the phenotypic variance explained can be found in Supplemental Table S2. Manhattan plots for malting traits with significant marker-trait associations not displayed in can be found in Supplemental Figure S3.
outlines the positions of the selected subset of QTL within the barley genome. Positions of markers significantly associated with a given trait are highlighted in red. QTL for the bB color value were found on the long arm of 1H, the short and long arm of 4H, and the short arm of 7H. The 4HL QTL was stable across the 2021 and 2022 seasons. QTL for various malting parameters were identified on the short arm of 4H, the long arm of 5H, the short arm of 6H, and the long arm of 7H.
Discussion
An overview of blue aleurone
Anecdotal feedback from members of the US malting industry was that blue aleurone is undesirable, despite the presence of blue aleurone varieties such as Flavia, Violetta, Wintmalt, and Thunder. This aversion is reflected in some of the available literature.[Citation10,Citation11] An interesting feature of Flavia, Violetta, Wintmalt, and Thunder is that they are all two-row varieties with blue aleurone, winter growth habit, and of recent European origin (except Thunder, which has Wintmalt as a parent). In the US, there has been long-term deliberate selection for white aleurone in spring growth habit malting varieties, and spring types dominate the malting barley variety acreage. Interestingly, in Canada six-row malting types were at one time required to have blue aleurone in to easily distinguish them from white aleurone feed types. The blue six-row requirement has since been dropped and eventually six-row varieties have fallen out of favor entirely in North America, by and large taking the blue aleurone trait with them. The recent interest in winter and facultative barley, for ecosystem services and supply chain sustainability, has brought blue back. Before wholesale embrace of blue, or at least not selecting against it, there is the cautionary tale of the blue aleurone variety Henley in Australia, which was heralded as a success until customer complaints about its color led to its abandonment and a redoubled effort to focus on white aleurone exclusively.[Citation8]
This tally of blue vs. white, however, belies the complexity of the trait. Blue aleurone is under multigenic control with blue anthocyanin accumulation being driven by the genotypes at the Blx1, Blx2, Blx3, Blx4, and Blx5 loci.[Citation10,Citation15] To further muddy the picture, QTL for aleurone color are reported on all barley chromosomes and these may contribute to the reported gradation/intensity of blueness.[Citation35] Furthermore, the trait is known to be environmentally labile and to show genotype × environment interaction (). The 2021 season proved to be a very good screening environment for blue aleurone. The Cycle 5 population showed a relatively discrete distribution of aleurone color () and that each of the crosses followed approximately the expected patterns of inheritance. Crosses between blue aleurone lines (e.g., DH140963 × Flavia) produced only blue progeny whereas blue × white crosses (e.g., DH120304 × Flavia) produced progeny with both aleurone color types. A robust genetic analysis of segregation patterns in this germplasm is not warranted, due to the small population sizes for six of the crosses, but there appears to be a deficiency of blue aleurone types in each of the crosses that would be expected to segregate. Investigation of parent phenotypic descriptions revealed the first of several levels of ambiguity. For example, the International Union for the Protection of New Varieties of Plants (UPOV) variety aleurone color descriptions state that Flavia and Mateo are “weakly colored” while Lyberac is “whitish.”[Citation36] Of the OSU pedigrees, Lightning, DH120304, DH141944, and DH150115 are unambiguously white. Thunder and DH140963 are unambiguously blue. DH142000, while initially described as white based on visual assessment of pearled grain, appeared bluish after 24 h of immersion in tap water. In the present study, assessment of aleurone color was first attempted using visual assessment of imbibed, intact grains (Blue Proportion). However, this was challenging based on the different intensities of blue present in completely blue grains and/or the presence of blue color in only portions of a kernel. Furthermore, assessment of color on a sample of 100 grains was quite time-consuming.
Based on this, aleurone color was measured based on visual assessment (Blue intensity; 1–4 scale) of dry, pearled grains. In general, the panel of four independent assessors agreed on a blue (1,2,3,) vs white (4) classification, but diverged on assessments of the degree of blueness (ratings 1–3). Nonetheless, mean anthocyanin concentrations within each of the blue groups were significantly different, lending some credence to the rating system and panelist expertise. However, based on the ambiguity in degree of blueness visual ratings, a colorimeter was used to precisely quantify color. In this case, the most robust colorimeter measure for expression of blue in the aleurone to be b*, the chromatic value differentiating blue from yellow. The acid treatment before colorimeter measurement conducted in 2021 involved an additional step and was more labor intensive because of the nature of working with HCl. Additionally, HCl reacts with all anthocyanins in the grain, not just those responsible for the blue aleurone. While this led to high correlations with measured total anthocyanin (), it was not optimal for use in this study. Using bB as the best measure of aleurone color, we found that correlations between aB and bB with visual rating were high and significant (0.94; ) in 2021. Correlations between other colorimeter parameters inter se were also high, but lower with visual ratings.
Comparison of aleurone color and malting traits irrespective of genetic background
Across the entire Cycle 5 population, significant differences were identified in the malting characters of the blue and the white aleurone barleys (, ). Furthermore, there were moderate to high correlations identified between the bB aleurone color and various malting characteristics when the entire panel was considered (). This is not altogether unexpected. The Cycle 5 panel consists of doubled haploid genotypes resulting from eight different crosses. The genetic diversity in this germplasm, while excellent from a QTL discovery perspective, introduces population structure and is a confounding factor when it comes to interpretation of results. Viewing the panel as a whole, one would conclude that blue aleurone might be better suited for all malt profile and white aleurone for adjunct malt profile. Across the entire population, both white and blue aleurone barley showed similar malt extract values. Malt extract is a “gateway” trait where high levels are important for all malt types. The differences identified in this study across the entire population may be the grain of truth that the aversion to blue is built on; however, a more comprehensive analysis that accounts for population structure (similar to the one performed in the present study) is required to see the full picture. The germplasm assessed in this study was biased towards blue aleurone. In total, there were 34% white aleurone and 66% blue aleurone types in this population. Over 75% of the blue aleurone genotypes traced to a single cross between a blue variety (Flavia) and a blue selection (DH140963). This means that the observed differences in the blue and white aleurone barleys in terms of malt quality could be driven by the genetic background of the DH140963/Flavia pedigree rather than the genes actually responsible for blue aleurone. Fortuitously, 30% of the total germplasm traced to a cross between a white aleurone selection (DH120304) and Flavia. This DH120304/Flavia pedigree was considered separately in the additional analysis comparing the blue and white aleurone barleys to remove the effects of population structure from the phenotypic analysis.
Comparison of aleurone color and malting traits within a bi-parental subset
To better understand the true impact of aleurone color on malting quality, it is important to remove the genetic background. To this end, an analysis mirroring the full population analysis was run, considering just the DH120304/Flavia cross where aleurone color was segregating. In this analysis, the apparent differences between malting quality traits between blue and white are negligible, except for diastatic power where white aleurone barleys were higher, and extract where blue aleurone barleys were higher (, ). Additionally, when only the DH120304/Flavia subset is considered the moderate to high correlations between malt quality and aleurone color observed across the whole population for the most part disappear (). It is interesting that diastatic power remains significantly higher in the white aleurone lines and fine extract remains significantly higher in the blue aleurone lines, even when those lines result from the same cross. These significant effects may be an artifact of the smaller population size when considering only the DH120304/Flavia subset. These findings overall outline the conclusion that, in a consistent genetic background, the genes responsible for aleurone color matter very little in the malting character of barley. This conclusion has additional support in the literature. In their study, Baxter and O’Farrell, did not identify any decrease in malting capability related to blue aleurone.[Citation9] Barley Australia, despite having no tolerance for barley with blue aleurone, recognizes that empirical studies do not support the bias against blue.[Citation37] In fact, in the past, blue aleurone has been used as a visual, selectable marker for six-row malting barley in Canada.[Citation11] In Europe, barley with blue aleurone is frequently used for malting.[Citation36]
Comparison of aleurone color traits across seasons
Ideally, a systematic study of the impact of aleurone color on malting quality would involve a large sample of genetically diverse germplasm assessed in multiple environments. However, the imperative of making progress in variety development programs focused on elite germplasm with limited genetic diversity, coupled with the high cost of measuring malting quality, drove the decision to take a snapshot of current doubled haploid germplasm in the Oregon State University barley breeding program, developed and selected without attention to aleurone color. In the two seasons of available colorimeter data, there were found to be significant effects of genotype, environment, and genotype by environment (). This means that there was a substantial contribution of genotype to aleurone color. To generalize, there are blue genotypes (such as Thunder), there are white genotypes (such as Lightning), and there are genotypes with gradations between blue and white (such as Lyberac and Mateo), and it is these gradating genotypes that are most likely to defy easy classification). These results also show that aleurone color was heavily impacted by year-to-year effects, and even showed differences in the response to years across genotypes. The effect of year was also observed in the lower correlations between measures of aleurone color across years than within years (). This highlights the complexity of what may otherwise be considered a straightforward trait.
The 2021 season was an excellent time to measure aleurone color as a clear bimodal distribution in bB values is visible (). This relatively straightforward, discrete classification of aleurone color, was not possible in 2022 as the phenotypic frequency distribution for bB was not bimodal (). On closer examination, nearly all tested germplasm in 2022 fell into the range of bB values of the white germplasm from 2021. Temperature and light have both been found to have an impact on anthocyanin accumulation. Heat stress leads to increased antioxidant capacity of the grain and UV radiation positively regulates anthocyanin biosynthesis.[Citation38] UV radiation was not directly measured in this experiment; however, temperature and precipitation were tracked by a weather station at the experimental site. There was higher average daily maximum temperature and less precipitation in the late season (May and June) during 2021 (76.25 °F and 2.62in) compared to 2022 (68.75 °F and 6.81in). By the end of June in 2021, 1868 degree-days had accumulated compared to 1658 degree-days in 2022. This potentially led to an increase in heat stress in the 2021 season compared to 2022, resulting in a better screening environment for blue aleurone. There was one case of a doubled haploid rated as blue in 2021 by the bB value but classified as white in the 2021 visual analysis. This was the case of DH200149 from the cross of Flavia and DH140963. All four panelists rated the selection as white (4) whereas it would be classified as blue according to the colorimeter data.
Genome wide association study
GWAS is usually thought of as an approach to finding genomic regions that drive a target trait in order to ultimately isolate the genes responsible, and to use gene sequence information to design diagnostics and/or therapies. In the current application, it can also be used as a technique to determine the genetic basis of observed correlations and significant differences (or lack thereof). Correlations and significant differences, in turn, can be due to linkage, pleiotropy, or population structure. While linkage and pleiotropy cannot be differentiated in the present analysis,[Citation21] a realized additive relationship matrix and principal component analysis were used to account for individual relatedness based on the available marker data,[Citation33] so the effect of population structure on the identified QTL has been minimized.
All measures of barley aleurone color identified a locus on the long arm of chromosome 4H to be a main driver of differences in aleurone color in the Cycle 5 population (). Based on the position of the most significant markers for these traits (Supplemental Table S1), the observed peak is near the reported position of the Blx1 locus (linked to Blx3 and Blx4) that is part of the complex responsible for the expression of blue aleurone.[Citation10] Mapping the Blx complex on 4H as the key driver of color in the Cycle 5 population provides a direct “quality control” metric and confirms that all of the methods of measuring aleurone color used in this study would be appropriate in assessing and selecting grain based on aleurone color. Three minor QTL for aleurone color (measured via bB) were identified in either the 2021 or 2022 season, but were not stable across years. The additional measures of color also identified similar minor QTL (Supplemental Table S1). These associations may be modifiers of the major gene responsible for small variations in aleurone color in certain environmental conditions. Explicitly modeling the genetic basis of the response to environmental changes in aleurone color is beyond the scope of the present study.
In terms of malting quality QTL, wort color, FAN, S/T, and alpha-amylase all map to a locus on the long arm of chromosome 5H (; Supplemental Table S1). The underlying feature in this case is likely the Sd2 locus. In a study by Sayre-Chavez et al.[Citation39] the Sd2 locus was found to be the largest driver of flavor and degree of modification. Surprisingly, no water sensitivity QTL was identified at this locus in the present study. This study was not successful in identifying QTL for malt extract, diastatic power, total malt protein, and wort beta-glucan. Additional power in the form of increased genetic variance or more replications (possibly across years) could remedy this issue. The 179 genotypes assessed for malt quality and aleurone color already represented a large commitment of resources.
One of the minor QTL for bB (R2<.01) on the short arm of 4H identified in 2021, was less than 500 bp from an identified wort color QTL, and approximately 8.1 Mb from an identified water sensitivity QTL. As stated previously, the analysis used in this study does not differentiate linkage and pleiotropy; however, the partial R2 values for the wort color and WS were small (0.04 and 0.06 respectively), and the association with bB with this region was not observed in 2022. Furthermore, this locus is not near the reported positions of the Blx genes responsible for blue aleurone (the long arm of 4H or on 7H), and its effects on both malt and color are small. If this association is identified in future studies, and either linkage or pleiotropy were identified, it could represent an interesting link between grain color and malting quality. A key result in the GWAS is that no malting quality genetic determinants map to the long arm of chromosome 4H where the Blx1/3/4 loci are found (, Supplemental Table S1).
Summary
During this study, it was found that the white and blue aleurone barley groups did have differences in malting character across the whole population and there were correlations present between malt quality and aleurone color; however, these relationships did not hold within a biparental subset of the population. These relationships were instead a likely result of population structure. Additionally, chromosomal regions important to both aleurone color and malting quality traits were identified. No malting parameters were associated with the Blx complex on the long arm of chromosome 4H. It was also found that no color traits were associated with the major QTL contributing to malt quality (primarily Sd2 on the long arm of chromosome 5H). A minor aleurone color QTL was identified near the QTL for wort color and water sensitivity; however, these were all determined to explain little of the observed variance in any of their respective traits. Furthermore, the minor color QTL was not stable across the seasons considered in this study. Based on these results, it can be concluded that the presence of blue aleurone has little bearing on whether or not a barley line is suitable for malting. Instead, these decisions should be made based on thorough malt analysis.
Abbreviations: | ||
AA | = | alpha-amylase |
ANOVA | = | analysis of variance |
BG | = | beta-glucan |
DP | = | diastatic power |
FAN | = | free amino nitrogen |
FE | = | fine extract |
FR | = | friability |
GM | = | grain moisture |
GP | = | grain protein |
GWAS | = | genome wide association study |
MM | = | malt moisture |
MP | = | total malt protein |
PUG | = | partially unmodified grains |
SP | = | soluble malt protein |
S/T | = | soluble over total protein |
WC | = | wort color |
WS | = | water sensitivity |
WUG | = | wholly unmodified grains |
Supplemental Material
Download PNG Image (34.5 KB)Supplemental Material
Download PNG Image (92.5 KB)Supplemental Material
Download PNG Image (78.2 KB)Supplemental Material
Download MS Excel (22.1 KB)Acknowledgments
Our thanks to Teepakorn Kongraksawech and Andrew Ross for use of the colorimeter and spectrophotometer.
Data availability
Phenotypic data will be available on the Triticae Toolbox T3/Barley.
Declaration of interest
The authors declare no conflict of interest.
Additional information
Funding
Literature cited
- Briggs, D. E. 1998. Malts and Malting. London. Blackie Academic & Professional.
- Newman, C. W.; Newman, R. K. A Brief History of Barley Foods. Cereal Foods World. 2006, 51, 4–7. DOI: 10.1094/cfw-51-0004.
- Ullrich, S. Significance, Adaptation, Production, and Trade of Barley. In Barley Production, Improvement, and Uses. Ames, Iowa. Wiley-Blackwell, 2011, pp 3–13.
- Hernandez, J.; Meints, B.; Hayes, P. Introgression Breeding in Barley: Perspectives and Case Studies. Front. Plant Sci. 2020, 11, 761. DOI: 10.3389/fpls.2020.00761.
- Meints, B.; Cuesta-Marcos, A.; Fisk, S.; Ross, A.; Hayes, P. Food barley Quality Improvement and Germplasm Utilization. In Exploration, Identification and Utilization of Barley Germplasm, Academic Press, 2016; pp 41–73. DOI: 10.1016/b978-0-12-802922-0.00003-0.
- AMBA. 2021 Malting Barley Breeding Guidelines Ideal Commercial Malt Criteria. American Malting Barley Association. https://ambainc.org/wp-content/uploads/2021/07/Malting-Barley-Breeding-Guidelines_2021_June.pdf.
- Rani, H.; Bhardwaj, R. D. Quality attributes for Barley Malt: “The Backbone of Beer. J. Food Sci. 2021, 86, 3322–3340. DOI: 10.1111/1750-3841.15858.
- Government of Western Australia, Department of Primary Industries and Regional Development. 2016, September 20. Barley: Blue aleurone. Agriculture and Food. https://www.agric.wa.gov.au/barley/barley-blue-aleurone (accessed Nov. 7, 2022).
- Baxter, E. D.; O’Farrell, D. D. Malting and Brewing with Barleys Having Blue Aleurones. J. Inst. Brewing. 1987, 93, 308–312. DOI: 10.1002/j.2050-0416.1987.tb04508.x.
- Jia, Y.; Selva, C.; Zhang, Y.; Li, B.; McFawn, L. A.; Broughton, S.; Zhang, X.; Westcott, S.; Wang, P.; Tan, C.; et al. Uncovering the Evolutionary Origin of Blue Anthocyanins in Cereal Grains. Plant J. 2020, 101, 1057–1074. DOI: 10.1111/tpj.14557.
- Schwarz, P.; Horsley, R. 2001, November 30. North American 2 Row VS 6 Row Malting Barley. MoreBeer! https://www.morebeer.com/articles/Comparison_Two_Six_Row. (Accessed Nov 7, 2022).
- Agati, G.; Brunetti, C.; Di Ferdinando, M.; Ferrini, F.; Pollastri, S.; Tattini, M. Functional Roles of Flavonoids in Photoprotection: New Evidence, Lessons from the Past. Plant Physiol. Biochem. 2013, 72, 35–45. DOI: 10.1016/j.plaphy.2013.03.014.
- Chalker-Scott, L. Environmental Significance of Anthocyanins in Plant Stress Responses. Photochem. Photobiol. 1999, 70, 1–9. DOI: 10.1111/j.1751-1097.1999.tb01944.x.
- Gould, K. S. Nature’s Swiss Army Knife: The Diverse Protective Roles of Anthocyanins in Leaves. J. Biomed. Biotechnol. 2004, 2004, 314–320. DOI: 10.1155/s1110724304406147.
- Strygina, K. V.; Börner, A.; Khlestkina, E. K. Identification and Characterization of Regulatory Network Components for Anthocyanin Synthesis in Barley Aleurone. BMC Plant Biol. 2017, 17 (Suppl 1), 184. DOI: 10.1186/s12870-017-1122-3.
- Gutiérrez, L.; Cuesta-Marcos, A.; Castro, A. J.; von Zitzewitz, J.; Schmitt, M.; Hayes, P. M. Association Mapping of Malting Quality Quantitative Trait Loci in Winter Barley: Positive Signals from Small Germplasm Arrays. The Plant Genome 2011, 4, 256–272. DOI: 10.3835/plantgenome2011.07.0020.
- Jannink, J.-L.; Bink, M. C. A. M.; Jansen, R. C. Using complex Plant Pedigrees to Map Valuable Genes. Trends Plant Sci. 2001, 6, 337–342. DOI: 10.1016/S1360-1385(01)02017-9.
- Locatelli, A.; Cuesta-Marcos, A.; Gutiérrez, L.; Hayes, P. M.; Smith, K. P.; Castro, A. J. Genome-Wide Association Mapping of Agronomic Traits in Relevant Barley Germplasm in Uruguay. Mol. Breeding 2013, 31, 631–654. DOI: 10.1007/s11032-012-9820-x.
- Yu, J.; Pressoir, G.; Briggs, W. H.; Vroh Bi, I.; Yamasaki, M.; Doebley, J. F.; McMullen, M. D.; Gaut, B. S.; Nielsen, D. M.; Holland, J. B.; et al. A Unified Mixed-Model Method for Association Mapping That Accounts for Multiple Levels of Relatedness. Nat. Genet. 2006, 38, 203–208. DOI: 10.1038/ng1702.
- Thornsberry, J. M.; Buckler, E. S. Structure of Linkage Disequilibrium in Plants. Annu. Rev. Plant Biol. 2003, 54, 357–374. DOI: 10.1146/annurev.arplant.54.031902.134907.
- Fernandes, S. B.; Zhang, K. S.; Jamann, T. M.; Lipka, A. E. How Well Can Multivariate and Univariate GWAS Distinguish between True and Spurious Pleiotropy? Front. Genet. 2020, 11, 602526. DOI: 10.3389/fgene.2020.602526.
- Cistue, L.; Ziauddin, A.; Simion, E.; Kasha, K. J. Effects of Culture Conditions on Isolated Microspore Response of Barley Cultivar Igri. Plant Cell. Tiss. Organ Cult. 1995, 42, 163–169. DOI: 10.1007/BF00034234.
- Dhesi, N. S.; Fedak, G.; Desormeaux, R. W. Evaluation of a Confirmatory Test and Environmental Influence on Aleurone Colour Expression of Barley. Proceedings of the Association of Official Seed Analysts. Vol. 65, 1975, pp 66–74 (9 pages) Published by: Association of Official Seed Analysts and the Society of Commercial Seed Technologists (SCST) https://www-jstororg.oregonstate.idm.oclc.org/stable/23432541.
- Abdel-Aal, E. S. M.; Hucl, P. A Rapid Method for Quantifying Total Anthocyanins in Blue Aleurone and Purple Pericarp Wheats. Cereal Chem. 1999, 76, 350–354. DOI: 10.1094/CCHEM.1999.76.3.350.
- ASBC. 2022. ASBC Methods of Analysis. asbcnet.org. https://www.asbcnet.org/methods/pages/default.aspx (accessed Nov 9, 2022).
- R Core Team. 2022. R: A Language and Environment for Statistical Computing. R Foundation for Statistical Computing, Vienna, Austria. https://www.R-project.org/.
- Wei, T.; Simko, V. 2021. R Package ‘corrplot’: Visualization of a Correlation Matrix. (Version 0.92), https://github.com/taiyun/corrplot.
- Fisher, R. A. Design of Experiments. Br. Med. J. 1936, 1, 554–554. DOI: 10.1136/bmj.1.3923.554-a.
- Box, G. E. P.; Cox, D. R. An Analysis of Transformations. J. Roy. Stat. Soc. Ser. B Methodol. 1964, 26, 211–243. http://www.jstor.org/stable/2984418. DOI: 10.1111/j.2517-6161.1964.tb00553.x.
- SAS Institute Inc. 2022. SAS/ACCESS® 9.4 interface to ADABAS: Reference. SAS Institute.
- Zheng, X.; Levine, D.; Shen, J.; Gogarten, S.; Laurie, C.; Weir, B. A High-Performance Computing Toolset for Relatedness and Principal Component Analysis of SNP Data. Bioinformatics. 2012, 28, 3326–3328. DOI: 10.1093/bioinformatics/bts606.
- Rosyara, U. R.; De Jong, W. S.; Douches, D. S.; Endelman, J. B. Software for Genome‐Wide Association Studies in Autopolyploids and Its Application to Potato. Plant Genome. 2016, 9, 1–10. DOI: 10.3835/plantgenome2015.08.0073.
- Price, A. L.; Patterson, N. J.; Plenge, R. M.; Weinblatt, M. E.; Shadick, N. A.; Reich, D. Principal components Analysis Corrects for Stratification in Genome-Wide Association Studies. Nat. Genet. 2006, 38, 904–909. DOI: 10.1038/ng1847.
- Moskvina, V.; Schmidt, K. M. On multiple-Testing Correction in Genome-Wide Association Studies. Genet. Epidemiol. 2008, 32, 567–573. DOI: 10.1002/gepi.20331.
- Jia, Y.; Westcott, S.; He, T.; McFawn, L. A.; Angessa, T.; Hill, C.; Tan, C.; Zhang, X.; Zhou, G.; Li, C. Genome-Wide Association Studies Reveal QTL Hotspots for Grain Brightness and Black Point Traits in Barley. Crop J. 2021, 9, 154–167. DOI: 10.1016/j.cj.2020.04.013.
- UPOV. 2022. PLUTO Plant Variety Database. UPOV Pluto. https://pluto.upov.int/login1 (accessed Nov 10, 2022).
- Barley Australia. 2016, July 12. Blue Aleurone Layer Barley. https://www.barleyaustralia.com.au/wp/wp-content/uploads/2020/02/Media-Releas-Blue-Aleurone-Barley-2016.pdf.
- Martínez-Subirà, M.; Moralejo, M.; Puig, E.; Romero, M.-P.; Savin, R.; Romagosa, I. Impact of Rising Temperature in the Deposition Patterns of Bioactive Compounds in Field Grown Food Barley Grains. Plants 2021, 10, 598. DOI: 10.3390/plants10030598.
- Sayre-Chavez, B.; Bettenhausen, H.; Windes, S.; Aron, P.; Cistué, L.; Fisk, S.; Helgerson, L.; Heuberger, A. L.; Tynan, S.; Hayes, P.; Muñoz-Amatriaín, M. Genetic basis of Barley Contributions to Beer Flavor. J. Cereal Sci. 2022, 104, 103430. DOI: 10.1016/j.jcs.2022.103430.