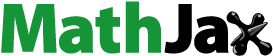
ABSTRACT
Organic selenium has been widely studied for its ability to better fulfill the physiological functions of selenium. In this study, a novel organic selenium (wheat protein hydrolyzate chelated selenium, WPH-Se) was prepared by chelating the hydrolyzate of wheat protein hydrolyzed by alkaline protease (WPH) with selenium, its preparation process was optimized; and structural and functional properties were investigated. The results showed that the highest selenium content of 10.97 mg/g was obtained in the chelate under the optimal conditions (peptide/selenium mass ratio 2:1, temperature 80°C, pH 8, time 60 min). UV-Vis, FTIR, DSC and SEM provided more information for the characterization of WPH-Se. During the chelating process, selenium ions might be effectively bound to WPH through carboxyl, carbonyl and amino ligands, and the C=O bond and -NH, -OH groups might be the sites. At different pHs, the solubility of WPH-Se was generally lower than that of WPH, which reached a maximum of 32.69% at pH 6. The EAI of WPH-Se was higher than that of WPH, which reached a maximum of 0.84 m2/g, and the ESI reached a maximum of 47.3 min at pH 10. The free sulfhydryl content of WPH-Se was greater than that of WPH, which reached a maximum of 12.78 μmol/g at pH 8. WPH-Se was superior to WPH in terms of foaming ability and emulsification properties. WPH-Se contained a certain amount of aromatic amino acids (7.962%) and a relatively high amount of hydrophobic amino acids (38.490%), and had high nutritional value. Wheat protein peptide chelated selenium would be a useful functional additive, this study would provide data to support further research and application of selenium dietary supplements.
Introduction
Selenium (Se) is an essential trace element for the human body.[Citation1] Adequate amount of selenium has irreplaceable role in improving human immunity, antioxidant, and maintaining normal physiological activities.[Citation2,Citation3] Selenium deficiency would affect the expression of selenoproteins and their biological functions, which could lead to macrosomia, cardiovascular disease, diabetes, and liver disease.[Citation4,Citation5] About 1 billion people worldwide suffer from selenium deficiency due to environmental pollution, poor living habits and aging.[Citation6] The body cannot synthesize selenium on its own, and dietary intake is the only way to supplement selenium.[Citation7] The absorption and utilization of inorganic selenium is affected by factors such as food source, chemical matrix properties and optimal nutritional levels of different forms of selenium, and the development of a better selenium supplement has become a focus of research.
Studies have shown that organic selenium was characterized by low toxicity, good absorption and high bioavailability, which could better perform the physiological functions of selenium.[Citation8,Citation9] As a kind of organic selenium, the research on selenium-enriched bioactive peptide is being carried out gradually. In general, selenium-enriched bioactive peptides can be categorized into plant sources and microbial sources. Plant sources are selenium-enriched plant foods produced by fortifying the soil with selenium, which have nucleophilic selenium amino acids, special peptide sequences, and higher bioavailability than inorganic selenium.[Citation10] For example, a novel selenopeptide (SeMet-Pro-Ser) was isolated from brown rice and showed the ability to scavenge free radicals in vitro experiments.[Citation11] Selenium-containing soy peptides showed beneficial antioxidant activity in inflammatory cell models and aging mouse models by modulating MAPK/NF-κB pathway and oxidative stress.[Citation12] Microbial sources of selenium-enriched bioactive peptides were mainly converted from inorganic selenium to bound state of and enzymatic selenium-containing proteins by microbial transformation. For example, Chen et al.[Citation13] used sodium selenite as a selenium source and obtained a selenium-enriched strain with a selenium conversion rate of (94.3 ± 0.2)% by selenium-enriched fermentation with Bacillus cereus. Fang et al.[Citation14] obtained selenium-containing peptides with immunomodulatory activity from selenium-rich rice protein hydrolyzates (SPHs) by screening, isolation, purification. Selenium-enriched bioactive peptides of plant or microbial origin were prepared from selenium-enriched foodstuffs that had been fortified or transformed, which were costly and difficult to isolate and purify. Bioactive peptides are prepared from proteins of different origins by enzymatic hydrolysis, chemical degradation or microbial fermentation, and usually consist of 2 to 20 amino acid residues.[Citation15,Citation16] In addition to serving as dietary nutrients, bioactive peptides from various sources have been shown to have important roles in promoting human health such as antioxidant,[Citation17–19] antithrombotic, antimicrobial, antihypertensive, immunomodulatory, mineral binding,[Citation20–22] and hepatoprotective functions.[Citation23] Recently, there have been reports on the preparation of selenium-enriched peptides by chelating selenium with bioactive peptides. Ye et al.[Citation24] prepared a novel organic selenium (SPIP-Se) by chelating soy protein isolated peptides (SPIPs) with selenium, which had good solubility. In vitro, SPIP-Se had stronger hydroxyl radical scavenging activity and reducing ability than SPIP. Doan et al.[Citation25] fed sodium selenite, soy protein chelated selenium, and selenite yeast to pigs for 17 d, and showed that chelated selenium had antioxidant effects comparable to those of organic selenium. Selenium chelated peptides have high safety and antioxidant capacity, but their chelating mechanism as well as physicochemical properties such as solubility, foaming capacity, and emulsification properties still need to be further investigated in order to facilitate the wide application of highly effective dietary organic selenium supplements in the future food or pharmaceutical fields.
Wheat protein, also known as gluten, is a product of wheat starch processing, and nutritious natural plant protein with a complete range of amino acids,[Citation26] and widely used in food and feed industries. In order to expand the application range of wheat protein, studies on the modification such as enzymatic modification,[Citation27] which was the partial hydrolysis of wheat protein by reacting with specific enzymes to improve its functional properties such as high water solubility.[Citation28] However, current studies on the chelation of enzymatic hydrolyzate of wheat protein with selenium have not been reported. Therefore, the aim of this study was to prepare a chelate of wheat protein enzymatic hydrolyzate (WPH) with selenium, and to characterize its structure (by UV-Vis absorption spectroscopy, FTIR spectroscopy, DSC, and SEM), analyze the chelation mechanism, and evaluate the solubility, foaming capacity, and emulsification properties of the chelate. These data would provide a theoretical basis for the research and development of WPH-Se, and a good foundation for expanding the application of wheat protein.
Materials and methods
Materials
Wheat protein powder (protein content 83.5%) was purchased from Guanxian Ruixiang Biotechnology Development Co., Ltd. (Liaocheng, China). Alkaline protease (200 U/mg) was purchased from Shanghai Yuanye Biological Co., Ltd. (Shanghai, China). Sodium selenite (Na2SeO3) was purchased from Shanghai Sinopharm Co., Ltd (Shanghai, China). Sodium dodecyl sulfate (SDS) was purchased from Tianjin Tianli Chemical Reagent Co. (Tianjin, China). Dinitrobenzoic acid (DTNB) was purchased from Shanghai Macklin Biochemical Technology Co. (Shanghai, China). Coomassie Brilliant Blue was purchased from Shanghai Yuanye Biotechnology Co. (Shanghai, China). All chemical reagents were analytical grade.
Preparation of wheat protein hydrolysate (WPH)
The preparation of WPH was performed according to the method of Yang et al.[Citation29] with some modification. In brief, Wheat protein powder was mixed with 9-time deionized water, then placed in a three frequency ultrasonic reactor (3s, Jiangsu Jiangda Fivepines Biotechnology Co., Ltd, Jiangsu, China) at 55°C and 40 kHz for 20 min. Afterwards its pH was adjusted to pH 8 and then hydrolyzed by enzymes. The hydrolysis conditions were as follows: temperature 55°C, time 2.5 h, and alkaline protease (dosage: 8000 U/g), pH 8.0 ± 0.2 with NaOH (0.5 N). The hydrolyzate was heated at 100°C for 15 min to inactivate the enzymes. After cooling to room temperature, it was centrifuged at 4000 r/min for 10 min with a high-speed freezing centrifuge (GL-21 M, Hunan Xiangyi Instrument Development Co., Ltd, Hunan, China). The supernatant was collected and dried, and then stored at 20°C. Moreover, the degrees of hydrolysis(DH) of WPH determined by pH-stat method[Citation30] was 38.72%.
Optimal preparation conditions of WPH chelated selenium (WPH-Se)
Preparation of WPH-Se: First, the effects of mass ratio of WPH peptide to Na2SeO3 (peptide/selenium), temperature, pH, and chelating time on selenium content of chelate were studied. Based on the results of these single factor tests (Supplementary 1), a Box Behnken model with three independent variables including mass ratio of peptide/selenium (X1), temperature (X2), and pH (X3) was designed with Design-Expert Software (version 8.0, Stat-Easy Inc., USA) to investigate the optimal preparation conditions of WPH peptide-selenium chelate (Supplementary 1). The quadratic effects and central points were estimated with the selenium content of chelate as response. The specific process was as follows.
The solution of Na2SeO3 (1 mg/mL) was freshly prepared by dissolving 100 mg Na2SeO3 into 100 mL distilled water. WPH (0.1–0.3 g) were mixed with 100 mL of Na2SeO3 to adjust the mass ratio of peptide/Se to be 1:1, 2:1 and 3:1. After mixed, the chelate reaction was carried out in a stirring water bath (120 r/min) at different temperatures (70°C, 80°C, and 90°C) and pH (7, 8, and 9) for 60 min. Subsequently, anhydrous ethanol was added to the solution (5:1, v/v) to precipitate the chelate. The mixture was kept for 1 h at ambient temperature, followed by centrifugation at 8000 r/min for 10 min. The precipitate was collected and dried, which was assigned as WPH-Se.
Statistical analysis and verification
Multiple non-linear regression analysis was used to analyze the coefficients of the obtained response. Selenium content of chelate (Y, %) was expressed as function of independent variables by the following equation:
where, b0 was the intercept; bn was the linear coefficient; bnn was the quadratic coefficients and bnm was the interactive coefficients. Xn and Xm were independent variables. Seventeen experiments were run in triplicates. The model was built on basis of the variables with confidence levels higher than 95%. The optimum preparation conditions of peptide-selenium chelate were calculated from the model. Moreover, the model was verified by comparing the predicted value with experimental value under the optimal conditions.
Selenium content of chelate
Selenium content of chelate was detected by hydride generation-atomic fluorescence spectrometry, the first method for National Standard of the People’s Republic of China (GB5009.93–2017).[Citation31]
Structure analysis
Fourier transform infrared spectroscopy (FTIR): Sample (WPH and WPH-Se, 3 mg) and KBr (Dried, 100 mg) were thoroughly mixed, ground, pelleted. The absorption spectra of 400–4000 cm−1 were recorded by infrared spectrophotometer (FT-IR5500, Jiangsu Skyray Instrument Co., Ltd, Jiangsu, USA).
UV-Vis absorption spectra: WPH and WPH-Se were dissolved in deionized water at a concentration of 1 mg/mL, respectively. The UV-Vis absorption spectra of sample was determined by a UV-Vis spectrophotometer (22A1810001, Shanghai Flash Spectrum Biological Technology Co., Ltd, Shanghai, China) at the wavelength range of 200–800 nm.
Scanning electron microscopy: Sample (WPH and WPH-Se) was adhered to the sample table using conductive double-sided adhesive. After blowing gently with ear ball, ion sputtering was used to spray the gold coating. Surface microstructure was analyzed using a scanning electron microscopy (HITACHI, Dongguan Tiance Optical Equipment Co., Ltd, Guangdong, China). The sample was observed and photographed at 15 kV with a magnification of 5000×, 10000 × .
Differential scanning calorimetry (DSC) measurement: DSC measurement was performed using a differential scanning calorimeter (DSC-500 L, Shenzhen Yongda Instrument Equipment Co., Ltd, Shenzhen, China). An empty aluminum crucible was used to calibrate the instrument, and WPH and WPH-Se (3 mg, respectively) was weighted into crucible for determine. The DSC measurement was performed at a heating rate of 0.5°C/min from 40°C to 160°C with a nitrogen flow.
Functional properties analysis
Analysis of protein solubility:The protein solubility of WPH and WPH-Se was determined at different pHs, referring to the method of Ye et al.[Citation24] with some modification. Simply, sample was dissolved in deionized water to a concentration of 10% (w/v) and the pH was adjusted at 2, 4, 6, 8, and 10 with NaOH and HCl (1 N). The solution was centrifuged at 4000 r/min for 15 min. Supernatant was mixed with prepared biuret reagent (1:4, v/v), and then was bathed at 37°C for 30 min. The protein concentration was measured by the biurea method.[Citation32] The solubility was expressed as a percentage, as shown in the following formula:
where Wa-the amount of protein in the supernatant (mg),Wb-the amount of protein in the WPH and WPH-Se powders (mg).
Foam properties: Foam properties, including foaming capacity (FC) and foaming stability (FS), were analyzed with reference to the method of Chen et al. and Zhan et al.[Citation33,Citation34] with minor modification. The sample was separately dispersed in deionized water to a concentration of 1% (w/v), mixed evenly at room temperature. The sample solution (10 mL) was successively placed into a centrifuge tube. Its pH was adjusted to 2,4,6,8,10 with NaOH and HCl (1 N), and then foamed at 10,000 r/min for 1 min with a homogenizer at 25 ± 1°C. The formed dispersion was rapidly transferred into a 250-mL measuring cylinder, and the foam volume V0 at 0 min was recorded first, and then recorded as V10 at 10 min after standing for 10 min. FC and FS were computed as follows:
where V0 is the foam volume at 0 min and V10 is the foam volume at 10 min.
Emulsifying properties: Emulsifying properties included emulsifying stability index (ESI) and emulsifying activity index (EAI). EAI and ESI were measured according to the method of Tirgar et al. and Malomo et al.[Citation35,Citation36] with some modification. WPH and WPH-Se solution (10 mg/mL) was separately mixed with soybean oil (3:1, v/v) and homogenized at 10,000 r/min for 2 min. After the emulsion was formed, 50 μL was removed from the bottom of the emulsion at 0 min and 30 min respectively, which mixed evenly with sodium dodecyl sulfate (SDS, 0.1%, 5 mL). N for dilution factor was 100. The absorbance of solution was measured at 500 nm. ESI and EAI was calculated respectively by the following formula:
where, A0 is the absorbance (min 0), A30 is the absorbance (min 30), N is the dilution factor, c is the sample concentration (g/ml), ϕ is the oil volume in the emulsion.[Citation37]
Free sulfhydryl groups: Free sulfhydryl content was determined using the 5,5’-dithiodinitrobenzoate method applied by Huang et al.[Citation38] and Zhu et al.[Citation39] with slightly modification. In short, samples (1 g) were dissolved in 50 ml of Tris buffer (86 mM Tris, 90 mM glycine, 4 mM EDTA, pH 8). Sample solution (10 mL) was placed in centrifuge tubes and its pH adjusted sequentially to 2, 4, 6, 8, 10. The above sample was homogenized at 4000 r/min for 2 min, followed by centrifugation at 4000 r/min for 20 min at room temperature. The supernatant (1 mL) was removed and added to Tris buffer (5 mL) and DTNB solution (4.0 mg/mL, 50 μL), which were mixed and left for 30 min at 25°C. The absorbance (ASH) was measured at 412 nm and the sulfhydryl content (CSH) was obtained by the following equation.[Citation40]
where A412 = the absorbance at 412 nm; C = the sample concentration in mg solids/ml; D = the dilution factor; 13600 is the molar absorptivity.[Citation41]
Analysis of amino acid composition: Amino acid composition of WPH-Se was detected according to Lu et al.[Citation42] with minor modification. In brief, sample (0.5 g) was mixed thoroughly with HCl (6 N, 10 Ml) in a hydrolysis tube. After being vacuumed and filled with nitrogen gas, the tube was sealed, and then incubated at 110°C for 22 h. The solution was filtered through a 0.22 μm filter, and then used to analyze amino acid composition. Amino acid composition was detected by an automatic amino acid analyzer (membraPure A300, Germany).
Statistical analysis
Results were expressed as the mean ± sd through triplicating calculations. Analysis of variance (ANOVA) was conducted with a p-value <.05. Multiple comparison was compared with Tukey’test with a p-value <.05. Polynomial model fitting and the optimal condition derivation were performed in Design-Expert 8.0.
Results and discussion
Optimization of preparation conditions of WPH-Se
The optimization of mass ratio of peptide/selenium, temperature, and pH for preparation of WPH-Se was evaluated for their effect on selenium content of chelate using the Box-Behnken design (Supplementary 2). The optimal regression equation of selenium content of chelate (Y) on mass ratio of peptide/selenium (X1), temperature (X2), and pH (X3) was as follows:
Y = 10880.00–1312.50*X1 +106.25* X2 +273.75* X3-405.00*X1* X2-270.00*X1* X3 +577.50* X2*X3-3016.25*X12-1888.75* X22-1628.75* X32
At the same time, the significance test of variance analysis was performed (). Analysis of variance (ANOVA) was used to verify the accuracy of the model. The quadratic regression model chosen by the test was highly significant (P < .0001), showed that there was a significant relationship between the independent variable and dependent variable of the regression equation. A lack of fit test with a p-value of 0.0721 greater than 0.05 indicated that it was not significant. The Adj R2 was 0.9899, revealed that 98.99% of the change in response value could be reasoned by the model. R2 was 0.9956, indicated that there was consistency between the experimental and predicted values. The coefficient of the variation (C.V.) was 3.03%, showed a high degree of accuracy with the experimental values. In summary, the model fitted the experimental data and theoretical values of selenium content of chelate.[Citation43]
Table 1. Regression equation variance analysis of selenium content of chelate.
As shown in a1 and b1, the selenium content of chelate increased and then decreased with the increase of the mass ratio of peptide/selenium from 1:1 to 3:1. WPH was not sufficient for the chelation with selenium under a relatively low mass ratio of peptide/selenium, resulting in lower selenium content of chelate. However, as the chelation reaction proceeded, WPH was not fully involved in the reaction due to the high mass ratio of peptide/selenium, resulting in a waste of WPH.[Citation44] As shown in b1 and c1, as the pH increased from 7 to 9, the selenium content of chelate tended to increase at first and then gradually decrease. This was due to the situation that the presence of H+ competed with selenium ions for electron groups, which hindered the formation of WPH-selenium chelates.[Citation45] As the pH continued to rise, the selenium chelating capacity rapidly increased to a certain level and then decreased, possibly due to the fact that the charge of peptides was pH-dependent,[Citation46] and a certain pH may prevent electrostatic interactions between selenium and peptides, thus affecting peptide selenium chelation.
Figure 1. Response surface plots showing the effect of three factors on selenium content of chelate. (a1, a2) Interaction of X1 (mass ratio of peptide/selenium) and X2 (temperature, ◦C), (b1, b2) Interaction of X1 (mass ratio of peptide/selenium) and X3 (pH), (c1, c2) Interaction of X2 (temperature, ◦C) and X3 (pH).

For chemical reactions, temperature was a non-negligible factor affecting the reaction process. From a1 and c1, it could be seen that as the temperature increased from 70°C to 80°C, the selenium content of chelate gradually increased, indicated that the increase in temperature contributed to the chelation reaction. When the temperature exceeded 80°C, the selenium content gradually decreased, indicated that excessively high temperature was not conducive to the reaction. Relatively high temperature may disrupt the conformation of the peptide and dissociate the ionic bonds of the chelate or a part of the chelate could also be destroyed or lost, resulting in a decrease in chelation capacity. This result was in good agreement with those reported by Wang et al.[Citation47] As chelating between peptides and ions was an endothermic reaction, appropriate heat treatment was favorable for the synthesis of the WPH-Se and would provide energy as a driving force for stabilization.[Citation48] So the relatively low temperature slowed down the reaction rate, resulting in a lower selenium content of chelate, as evidenced by our results.
The steeper and higher the inclination of the surface, the greater the influence of the interaction between factors on the response value.[Citation49] When the contour plot was elliptical, the factor interaction significantly affected the change of response value, and when it was circular, the factor interaction had no significant effect. In contrast, the interaction contour plot of temperature (X2) and pH (X3) was elliptical, with a dense distribution of contours and relatively steep corresponding surfaces, indicated that the interaction between temperature and pH had a significant effect on the response value. The interaction between peptide/selenium (X1) and temperature (X2) was second, and the interaction between peptide/selenium (X1) and pH (X3) was not significant, which had good consistency with the data in .
Based on the results of the response surface model, the optimal conditions for the preparation of WPH-Se were predicted to be as follows: peptide/selenium mass ratio 2:1, temperature 80°C, pH 8. At this point, the predicted selenium content of chelate was 11.07 mg/g. By validating the model under the above conditions, the selenium content of chelate was 10.87 mg/g, which was close to the predicted value. Therefore, the response surface model was valid, showed that the optimized process was highly efficient for the preparation of WPH-Se. Zhao et al.[Citation45] studied chelation of Juncao Ganoderma lucidum peptides with selenium, and obtained the following optimal conditions: reaction time 60 min, reaction temperature 75°C, pH 9, volume ratio of sodium selenite solution to peptide solution 1:2, selenium content of chelate was (2985.89 ± 10.59) μg/g. Differences between our results may be due to differences in raw materials as well as ultrasonic pretreatment and enzymatic hydrolysis conditions. In comparison, the selenium content obtained in this study was relatively high.
Structure analysis
UV-Vis spectroscopy: The UV-Vis absorption spectra of wheat protein peptides chelated with selenium are shown in . The formation of chelates between organic ligands and transition ions resulted in the appearance of new absorption peaks, displacement of peaks, and disappearance of existing absorption peaks.[Citation50] As can be seen, the characteristic absorption peak of the WPH was around 212 nm, which was caused by the n→π* transition of C=O in the carboxyl groups, carbonyl and amide bonds.[Citation51,Citation52] Another characteristic absorption peak near 270 nm was due to the absorption of aromatic amino acids residues.[Citation53] After chelating with Se, these two characteristic absorption peaks were displaced and significantly enhanced. The characteristic absorption peak shifted to 204 nm, showing a blue shift. It was conjectured that the binding of selenium ions to the N and O of the peptide terminus, which affected the electron transition of C=O, thus promoting the blueshift. After chelating with Se, the characteristic absorption peak shifted to 255 nm, which may be caused by the change of chromophore of peptide and electron transition. The blue shift and intensity change of absorption peaks indicated that the polarization of chromophore (e.g. C=O, COOH and NH2) had changed after chelation.[Citation54] This result is similar to that reported by Yu and Fan[Citation55] for the collagen calcium complex and Wang et al.[Citation56] for the cucumber seed peptide-calcium chelate. The formation of chelates generally resulted in a change in the light absorption properties of their organic ligands, which was caused by the different valence electron leaps of the corresponding atoms of the wheat protein peptides bound to selenium, proving that they reacted with each other to form new compounds.
Fourier transform infrared spectroscopy (FTIR): The molecular interaction of ions with organic ligand groups can be well reflected by the characteristic absorption peak changes of FTIR.[Citation57,Citation58] As shown in , the FTIR spectra of WPH changed significantly after chelating selenium ions, such as the displacement, increase or disappearance of the spectral bands, which indicated the formation of chelates. The overlapping broad absorption peaks of WPH at 3312 cm−1, which were the characteristic peaks of –NH in the amide-I band and –OH in the carboxyl groups, displayed a redshift to 3301 cm−1 and a weakened intensity in spectra of WPH-Se. Similarly, Wang et al.[Citation59] reported that the absorption peak of – NH was redshifted after the wheat germ peptide chelating with calcium. The characteristic absorption peaks of WPH at 1673 cm−1 was caused by the telescopic vibration of the C=O bonds in the amide-I band, which moved to 1650 cm−1after chelation. It was also shown that the vibrational intensity of C = O in WPH-Se chelate was increased, which implied that the intramolecular hydrogen bonding force of the chelate was reduced and more C = O provided the coordination site for the Se ions. Malison et al.[Citation60] reported a new peptide-calcium chelate from chicken foot broth by-product, which also showed this migration of the amide-I ban. The stretching vibrations of C–O and bending vibrations of –OH occurred in the range of 1100 ~ 1000 cm−1,[Citation61] those absorption peaks disappeared after chelation. The absorption peaks of WPH-Se appeared at 802–710 cm−1, which were generated by C–H and N–H bond vibrations.[Citation62] The characteristic peak of the -coo− group was blue-shifted from 1397 cm−1 to 1408 cm−1 after chelation, indicated that the selenium chelation site was also related to the C-terminal carboxylate group. Zhang et al.[Citation63] also reported a change in the characteristic peak of the -coo− group in iron-chelating peptides from mung beans. The FTIR spectra showed that the C=O bonds and –NH, –OH groups were the site of the selenium chelation reaction. Similar studies pointed out the binding sites for peptides were amino groups, carbonyl and carboxyl in whey peptide-calcium chelate and protein hydrolyzates-zinc complex from oyster.[Citation64,Citation65]
Differential scanning calorimeter analysis: The DSC was used to analyze the thermal stability and the change of tertiary structure. There were significant differences of peak temperature between WPH-Se and WPH (). Changes in DSC could also determine that WPH-Se was a different substance from WPH. The DSC curves in showed that the thermal denaturation reaction of WPH-Se was not a single throughout the process. The endothermic peaks of WPH-Se were at 129.4°C and 134.1°C, while that of WPH was at 146.7°C. The appearance of endothermic peaks was caused by the breaking of C-N bonds at high temperature.[Citation66,Citation67] The different temperatures and energies required may be due to differences in the position of the C-N bond after chelation of selenium. Zhang et al.[Citation68] and Wang et al.[Citation69] prepared algal calcium-chelating peptide and sheep bone collagen peptide-calcium chelate, respectively, and both of them showed a significant increase in thermal stability after chelation of calcium. Fathima et al.[Citation70] studied the iron-tetrakis (hydroxymethyl) phosphonium (THP) complex with type I collagen and found that during collagen denaturation, the phase transition temperature of the prepared Fe-THP complex was increased. Our findings differ from theirs, probably due to the different ions chelated. Overall, WPH-Se was found to be thermally stable, although the thermal denaturation temperature decreased after chelating selenium.
SEM: shows the surface morphology of the samples. The surface of WPH was rough. The structure of WPH-Se was more dense and the surface became smooth and delicate, showing obvious porous after chelation. This was similar to the surface microstructural features of corn ACE inhibitory peptide-ferrous chelate.[Citation71] It was hypothesized that this was related to the formation of a cyclic structure during the chelation reaction, which was formed when selenium chelates with the peptide through carboxyl, carbonyl and amino groups.[Citation60] SEM revealed differences between WPH and WPH-Se, indicating the formation of new peptide-selenium chelate. Similarly, the surface morphology of the prepared oyster peptide-zinc nanocomposite both differed from the peptide and calcium-chelating Auxis thazard peptide.[Citation65,Citation72]
Functional properties analysis
Analysis of protein solubility
Solubility was not only related to protein content and structure, but was also affected by pH conditions. showed the solubility of WPH and WPH-Se. At pH 2, both WPH and WPH-Se exhibited relatively low solubility. With the increased of pH, both showed a trend of increasing first and then decreasing. The solubility of WPH-Se increased to a maximum of 32.69% at pH 6 and that of WPH increased to a maximum of 60.40% at pH 8. The solubility of WPH-Se was generally lower than that of WPH at different pHs. In the process of WPH preparation, the degradation of proteins lead to the high solubility due to the release of soluble aggregates, the unfolding of peptide chains and the decrease of the molecular weight of the structure.[Citation73,Citation74] After chelation, the complex structure of the new substance formed made the solubility of WPH-Se not as good as WPH due to the effect of selenium on the C=O bonds and –NH, –OH groups. It also showed that WPH-Se was more soluble in alkaline conditions than acidic conditions. This was similar to the results of Y et al.[Citation24]
Figure 6. Solubility of WPH and WPH-Se chelate at different pHs. (a–c) indicate a significant difference of WPH between different pHs (p < .05). (A–D) indicate a significant difference of WPH-Se between different pHs (p < .05).

Determination of Foam properties: FC and FS were important for foods associated with foam, such as cakes, ice creams and carbonated drinks,[Citation75] which were shown in . The results showed that FC and FS of WPH and WPH-Se chelate were different under different pHs. In the range of pH 2–6, the foaming ability of WPH gradually decreased, and increased under alkaline conditions. However, the foaming stability reached a maximum of 2 at pH 6. The foaming ability of WPH-Se decreased first, and then increased and then decreased in the range of pH6–10. However, the foaming stability reached a minimum of 0.565 at pH 4, which probably due to close to the isoelectric point of the involved protein.[Citation74] The high foaming properties away from the isoelectric point was mainly due to the high solubility of the protein, its small molecular weight and flexible side chains.[Citation76] With the increasing or decreasing of pH, the number of polar groups on the peptide surface increased, which increased the hydrophobicity of the protein and thus improved the foaming property. Overall, the foaming ability of WPH-Se was superior to WPH, which could be attributed to the interaction of peptides and selenium ions that may change the interfacial properties of lactoferrin membranes.
Figure 7. FC (a) and FS (b) of WPH and WPH-Se chelate at different pHs. In fig. a, (a–c) indicate a significant difference of WPH between different pHs (p < .05), (A–C) indicate a significant difference of WPH-Se between different pHs (p < .05). In fig. b, (a–b) indicate a significant difference of WPH between different pHs (p < .05), (A–B) indicate a significant difference of WPH-Se between different pHs (p < .05).

Emulsifying properties: The emulsification process can be summarized that the proteins were absorbed onto the surface of the newly formed oil droplets due to the homogenization.[Citation77] EAI and ESI of WPH and WPH-Se at different pH conditions were shown in . EAI of both was lowest at pH 4. EAI gradually increased with the improvement of solubility when the pH was greater than 4. EAI of WPH-Se was higher than that of WPH at various pHs, which reached the maximum value of 0.84 at pH 10 and that of WPH reached the maximum value of 0.37 at pH 8. This indicated that the newly formed chelate can stabilize more interfacial area.[Citation78] ESI results changed similarly to the EAI. ESI of WPH-Se enhanced with the increase of pH, reached a maximum of 47.3 at pH 10, which correlates with the solubility.[Citation79] In general, EAI and ESI under alkaline conditions were greater than those under acidic conditions. Emulsifying properties depended strongly on ionic charge and hydrophobicity. More hydrophobic amino acids were exposed due to unfolding or hydrolysis of peptide chains at high alkaline pH.[Citation80,Citation81] The maximum values of EAI and ESI for WPH-Se (0.835, 47.82%) were at pH 10, which was slightly higher or close to the values of bovine serum albumin (BSA) in the literature.[Citation82] Thus, wheat protein peptide chelated selenium would be a useful functional additive in emulsified foods.
Figure 8. ESI (a) and EAI (b) of WPH and WPH-Se chelate at different pHs. In fig. a, (a–e) indicate a significant difference of WPH between different pHs (p < .05), (A–E) indicate a significant difference of WPH-Se between different pHs (p < .05). In fig. b, (a–e) indicate a significant difference of WPH between different pHs (p < .05), (A–D) indicate a significant difference of WPH-Se between different pHs (p < .05).

Analyse of free sulfhydryl groups: The comparison of free sulfhydryl content is shown in . Free sulfhydryl content of WPH and WPH-Se was different at different pHs. The free sulfhydryl group of WPH reached a maximum value of 5.44 at pH 8, while WPH-Se reached a maximum value of 12.78 at pH 8, which was consistent with its maximum solubility at this point. Overall, the free sulfhydryl group content of WPH-Se was greater than that of WPH in the experimental range, presumably because the internal structure of the WPH molecule was changed after chelation and more sulfhydryl groups were exposed on the surface of the molecule due to structural unfolding.[Citation83] Whether there was a certain relationship between free sulfhydryl and the antioxidant ability of WPH-Se needed further study.
Figure 9. SH of WPH and WPH-Se chelate at different pHs. (a–d) indicate a significant difference of WPH between different pHs (p < .05), (A–E) indicate a significant difference of WPH-Se between different pHs (p < .05).

Amino acid composition: It was believed that the functional properties of peptides were related to the amino acid composition. The amino acid compositions of WPH-Se were shown in . Glu, Pro were the most abundant amino acids in WPH-Se. Besides, the hydrophobic amino acids and acidic amino acids were the majority in WPH-Se, accounting for 38.490% and 41.218%, respectively. It was reported that carboxyl groups of negatively charged acidic amino acids and hydrophobic amino acid residues played an important role in the metal chelation reaction.[Citation59,Citation84,Citation85] Jiang et al. reported that the amount of acidic amino acid residues favors metal chelation and carboxyl group may be the main binding site for zinc ions.[Citation86] Thus, the relatively large proportion of hydrophobic amino acid and acidic amino acids residues may be an important reason for the high rate of WPH chelation with selenium. This result was consistent with the results of structural characterization.
Table 2. Amino acid analysis of WPH-Se.
Also from the nutritional quality point of view, WPH-Se contained a certain amount of aromatic amino acids (7.962%) and a relatively high amount of hydrophobic amino acids (38.490%), which can be used as antioxidants to enhance the antioxidant activity of food products.[Citation87] Furthermore, WPH-Se was considered to have high nutritional value due to its high content of essential amino acids (25.784%). Therefore, WPH-Se could be used as both a selenium supplement and a good peptide supplement.
Conclusion
Wheat protein was used as raw material, enzymatically treated by alkaline protease to obtain WPH with some selenium chelating ability. Under the optimal conditions (peptide/selenium mass ratio 2:1, temperature 80°C, pH 8), the highest selenium content of 10.97 mg/g was obtained in the chelate. The results of UV-Vis absorption spectroscopy, FTIR spectroscopy, DSC, and SEM showed that the structure of WPH-Se was different from WPH. The intensity of the absorption peaks of UV-Vis spectra increased and blue-shifted after the chelation of WPH with selenium, indicating that the chelation produced new substances. The FTIR spectral data indicated that selenium ions might be effectively bound to WPH through carboxyl, carbonyl and amino ligands, and the C=O bond and -NH, -OH groups might be the sites of chelation reaction. The microstructure was observed by SEM, and it was found that the structure of WPH became more dense and the surface became smooth after chelated with selenium. In addition, WPH-Se was more soluble under alkaline condition, with certain foaming ability and good emulsification properties under alkaline conditions. WPH-Se had high nutritional value and a certain amount of aromatic amino acids and a relatively high amount of hydrophobic amino acids, so the antioxidant properties, the gastrointestinal absorption and safety of WPH-Se need to be further investigated.
Author Contribution Shengru Yang, Yinchen Hou, Jihong Huang: methodology, conceptualization. Shengru Yang, Jihong Huang, Qiushuang Zhang and Xiaolin Shen: investigation, data curation, and writing-original draft preparation. Yinchen Hou: resources. Shengru Yang, Aimei Liao, Xiaoqing Yuan, Mengxing Lu: writing-review and editing. Yinchen Hou and Jihong Huang: supervision. Qiushuang Zhang and Aimei Liao: project administration. All authors: have read and agreed to the published version of the manuscript.
Supplemental Material
Download MS Word (77.8 KB)Disclosure statement
No potential conflict of interest was reported by the author(s).
Supplemental data
Supplemental data for this article can be accessed online at https://doi.org/10.1080/10942912.2023.2270726.
Additional information
Funding
References
- Avery, J.; Hoffmann, P. R. Selenium, Selenoproteins, and Immunity. Nutrients. 2018, 10(9), 1203. DOI: 10.3390/nu10091203.
- Kieliszek, M.; Błażejak, S. Current Knowledge on the Importance of Selenium in Food for Living Organisms: A Review. Molecules. 2016, 21(5), 609. DOI: 10.3390/molecules21050609.
- Shu, Y.; Wu, M.; Yang, S.; Wang, Y.; Li, H. Association of Dietary Selenium Intake with Telomere Length in Middle-Aged and Older Adults. Clin. Nutr. 2020, 39(10), 3086–3091. DOI: 10.1016/j.clnu.2020.01.014.
- Zhang, L.; Gao, Y.; Feng, H.; Zou, N.; Wang, K.; Sun, D. Effects of Selenium Deficiency and Low Protein Intake on the Apoptosis Through a Mitochondria-Dependent Pathway. J. Trace Elem. Med. Biol. 2019, 56, 21–30. DOI: 10.1016/j.jtemb.2019.06.019.
- Ying, H.; Zhang, Y. Systems Biology of Selenium and Complex Disease. Biol. Trace Elem. Res. 2019, 192(1), 38–50. DOI: 10.1007/s12011-019-01781-9.
- Hu, J.; Zhao, Q.; Cheng, X.; Selomulya, C.; Bai, C.; Zhu, X.; Li, X.; Xiong, H. Antioxidant Activities of Se-SPI Produced from Soybean as Accumulation and Biotransformation Reactor of Natural Selenium. Food Chem. 2014, 146, 531–537. DOI: 10.1016/j.foodchem.2013.09.087.
- Trippe, R. C.; Pilon-Smits, E. A. H. Selenium Transport and Metabolism in Plants: Phytoremediation and Biofortification Implications. J. Hazard. Mater. 2021, 404, 124178. DOI: 10.1016/j.jhazmat.2020.124178.
- Rayman, M. P. The Use of High-Selenium Yeast to Raise Selenium Status: How Does It Measure Up? Br. J. Nutr. 2004, 92(4), 557–573. DOI: 10.1079/BJN20041251.
- Musik, I.; Kozioł-Montewka, M.; Toś-Luty, S.; Donica, H.; Pasternak, K.; Wawrzycki, S. Comparison of Selenium Distribution in Mice Organs After the Supplementation with Inorganic and Organic Selenium Compound Selenosemicarbazide. Ann. Univ. Mariae Curie Sklodowska Med. 2002, 57(1), 15–22.
- Zhang, X.; He, H.; Xiang, J.; Yin, H.; Hou, T. Selenium-Containing Proteins/Peptides from Plants: A Review on the Structures and Functions. J. Agric. Food. Chem. 2020, 68(51), 15061–15073. DOI: 10.1021/acs.jafc.0c05594.
- Liu, K.; Du, R.; Chen, F. Stability of the Antioxidant Peptide SeMet-Pro-Ser Identified from Selenized Brown Rice Protein Hydrolysates. Food Chem. 2020, 319, 126540. DOI: 10.1016/j.foodchem.2020.126540.
- Zhang, X.; He, H.; Xiang, J.; Li, B.; Zhao, M.; Hou, T. Selenium-Containing Soybean Antioxidant Peptides: Preparation and Comprehensive Comparison of Different Selenium Supplements. Food Chem. 2021, 358, 129888. DOI: 10.1016/j.foodchem.2021.129888.
- Chen, X.; Li, S.; Cong, X.; Yu, T.; Zhu, Z.; Barba, F. J.; Marszalek, K.; Puchalski, C.; Cheng, S. Optimization of Bacillus Cereus Fermentation Process for Selenium Enrichment as Organic Selenium Source. Front Nutr. 2020, 7, 543873. DOI: 10.3389/fnut.2020.543873.
- Fang, Y.; Pan, X.; Zhao, E.; Shi, Y.; Shen, X.; Wu, J.; Pei, F.; Hu, Q.; Qiu, W. Isolation and Identification of Immunomodulatory Selenium-Containing Peptides from Selenium-Enriched Rice Protein Hydrolysates. Food Chem. 2019, 275, 696–702. DOI: 10.1016/j.foodchem.2018.09.115.
- Sila, A.; Bougatef, A. Antioxidant Peptides from Marine By-Products: Isolation, Identification and Application in Food Systems. A Review. J. Funct. Foods. 2016, 21, 10–26. DOI: 10.1016/j.jff.2015.11.007.
- Chakrabarti, S.; Guha, S.; Majumder, K. Food-Derived Bioactive Peptides in Human Health: Challenges and Opportunities. Nutrients. 2018, 10(11), 1738. DOI: 10.3390/nu10111738.
- Wen, C.; Zhang, J.; Zhang, H.; Duan, Y.; Ma, H. Plant Protein-Derived Antioxidant Peptides: Isolation, Identification, Mechanism of Action and Application in Food Systems: A Review. Trends Food Sci. Technol. 2020, 105, 308–322. DOI: 10.1016/j.tifs.2020.09.019.
- Sheng, Y.; Wang, W. Y.; Wu, M. F.; Wang, Y. M.; Zhu, W. Y.; Chi, C. F.; Wang, B. Eighteen Novel Bioactive Peptides from Monkfish (Lophius Litulon) Swim Bladders: Production, Identification, Antioxidant Activity, and Stability. Mar. Drugs. 2023, 21(3), 169. DOI: 10.3390/md21030169.
- Kong, J.; Hu, X. M.; Cai, W. W.; Wang, Y. M.; Chi, C. F.; Wang, B. Bioactive Peptides from Skipjack Tuna Cardiac Arterial Bulbs (II): Protective Function on UVB-Irradiated HaCat Cells Through Antioxidant and Anti-Apoptotic Mechanisms. Mar. Drugs. 2023, 21(2), 105. DOI: 10.3390/md21020105.
- Sánchez, A.; Vázquez, A. Bioactive Peptides: A Review. Food Quality and Safety. Food Qual. Saf. 2017, 1(1), 29–46. DOI: 10.1093/fqs/fyx006.
- Chakrabarti, S.; Jahandideh, F.; Wu, J. Food-Derived Bioactive Peptides on Inflammation and Oxidative Stress. Biomed Res. Int. 2014, 2014, 1–11. DOI: 10.1155/2014/608979.
- Rutherfurd-Markwick, K. J. Food Proteins as a Source of Bioactive Peptides with Diverse Functions. Br. J. Nutr. 2012, 108(S2), S149–S157. DOI: 10.1017/S000711451200253X.
- Zheng, S. L.; Wang, Y. Z.; Zhao, Y. Q.; Chi, C. F.; Zhu, W. Y.; Wang, B. High Fischer Ratio Oligopeptides from Hard-Shelled Mussel: Preparation and Hepatoprotective Effect Against Acetaminophen-Induced Liver Injury in Mice. Food Biosci. 2023, 53, 102638. DOI: 10.1016/j.fbio.2023.102638.
- Ye, Q.; Wu, X.; Zhang, X.; Wang, S. Organic Selenium Derived from Chelation of Soybean Peptide-Selenium and Its Functional Properties in vitro and in vivo. Food Funct. 2019, 10(8), 4761–4770. DOI: 10.1039/C9FO00729F.
- Doan, N.; Liu, Y.; Xiong, X.; Kim, K.; Wu, Z.; Bravo, D. M.; Blanchard, A.; Ji, P. Organic Selenium Supplement Partially Alleviated Diquat-Induced Oxidative Insults and Hepatic Metabolic Stress in Nursery Pigs. Br. J. Nutr. 2020, 124(1), 23–33. DOI: 10.1017/S0007114520000689.
- Lu, X.; Ma, R.; Zhan, J.; Liu, C.; Tian, Y. Starch Digestion Retarded by Wheat Protein Hydrolysates with Different Degrees of Hydrolysis. Food Chem. 2023, 408, 135153. DOI: 10.1016/j.foodchem.2022.135153.
- Ahmedna, M.; Prinyawiwatkul, W.; Rao, R. M. Solubilized Wheat Protein Isolate: Functional Properties and Potential Food Applications. J. Agric. Food. Chem. 1999, 47(4), 1340–1345. DOI: 10.1021/jf981098s.
- Zhang, R.; Zhang, J.; Liu, Y.; Fang, L.; Wei, Y.; Gu, R.; Lin, M.; Chen, L.; Zhou, Z. The Effect of Fermented Wheat Protein Hydrolysate on the Exercise Performance in Mice. J. Funct. Foods. 2022, 97, 105217. DOI: 10.1016/j.jff.2022.105217.
- Yang, X.; Li, Y.; Li, S.; Oladejo, A. O.; Wang, Y.; Huang, S.; Zhou, C.; Wang, Y.; Mao, L.; Zhang, Y., et al. Effects of Multi-Frequency Ultrasound Pretreatment Under Low Power Density on the Enzymolysis and the Structure Characterization of Defatted Wheat Germ Protein. Ultrason. Sonochem. 2017, 38, 410–420. DOI: 10.1016/j.ultsonch.2017.03.001.
- Saidi, S.; Deratani, A.; Belleville, M.-P.; Amar, R. B. Production and Fractionation of Tuna By-Product Protein Hydrolysate by Ultrafiltration and Nanofiltration: Impact on Interesting Peptides Fractions and Nutritional Properties. Food Res. Int. 2014, 65, 453–461. DOI: 10.1016/j.foodres.2014.04.026.
- National Health and Family Planning Commission of PRC, China Food and Drug Administration. National Standards for Food Safety the Determination of Selenium in Food: GB 5009.93-2017; Standards Press of China: Beijing, 2017.
- Sapan, C. V.; Lundblad, R. L.; Price, N. C. Colorimetric Protein Assay Techniques. Biotechnol. Appl. Biochem. 1999, 29(2), 99–108. DOI: 10.1111/j.1470-8744.1999.tb00538.x.
- Chen, X.; Chen, Y.; Zou, L.; Zhang, X.; Dong, Y.; Tang, J.; McClements, D. J.; Liu, W. Plant-Based Nanoparticles Prepared from Proteins and Phospholipids Consisting of a Core–Multilayer-shell Structure: Fabrication, Stability, and Foamability. J. Agric. Food. Chem. 2019, 67(23), 6574–6584. DOI: 10.1021/acs.jafc.9b02028.
- Zhan, F.; Li, J.; Wang, Y.; Shi, M.; Li, B.; Sheng, F. B. Foam, and Interfacial Properties of Tannic Acid/Sodium Caseinate Nanocomplexes. J. Agric. Food. Chem. 2018, 66(26), 6832–6839. DOI: 10.1021/acs.jafc.8b00503.
- Tirgar, M.; Silcock, P.; Carne, A.; Birch, E. J. Effect of Extraction Method on Functional Properties of Flaxseed Protein Concentrates. Food Chem. 2017, 215, 417–424. DOI: 10.1016/j.foodchem.2016.08.002.
- Malomo, S. A.; He, R.; Aluko, R. E. Structural and Functional Properties of Hemp Seed Protein Products: Structure-Function of Hemp Seed Proteins. J. Food Sci. 2014, 79(8), C1512–C1521. DOI: 10.1111/1750-3841.12537.
- Mcclements, D. J. Critical Review of Techniques and Methodologies for Characterization of Emulsion Stability. Crit. Rev. Food Sci. Nutr. 2007, 47(7), 611–649. DOI: 10.1080/10408390701289292.
- Huang, Y.; Hua, Y.; Qiu, A. Soybean Protein Aggregation Induced by Lipoxygenase Catalyzed Linoleic Acid Oxidation. Food Res. Int. 2006, 39(2), 240–249. DOI: 10.1016/j.foodres.2005.07.012.
- Zhu, Z.; Mao, X.; Wu, Q.; Zhang, J.; Deng, X. Effects of Oxidative Modification of Peroxyl Radicals on the Structure and Foamability of Chickpea Protein Isolates. J. Food Sci. 2021, 86(3), 824–833. DOI: 10.1111/1750-3841.15643.
- Beveridge, T. T. S. J.; Toma, S. J.; Nakai, S. Determination of SH‐And SS‐Groups in Some Food Proteins Using Ellman’s Reagent. J. Food Sci. 1974, 39(1), 49–51. DOI: 10.1111/j.1365-2621.1974.tb00984.x.
- Ellman, G. L. Tissue Sulfhydryl Groups. Archives of Biochemistry and Biophysics. Archiv. Biochem. Biophys. 1959, 82(1), 70–77. DOI: 10.1016/0003-9861(59)90090-6.
- Lu, K.; Zhao, Z.; Ho, S.-H.; Ma, R.; Xie, X.; Chen, C. Biorefining and the Functional Properties of Proteins from Lipid and Pigment Extract Residue of Chlorella Pyrenoidosa. Mar. Drugs. 2019, 17(8), 454. DOI: 10.3390/md17080454.
- Coelho, T. L. S.; Braga, F. M. S.; Silva, N. M. C.; Dantas, C.; Lopes Júnior, C. A.; de Sousa, S. A. A.; Vieira, E. C. Optimization of the Protein Extraction Method of Goat Meat Using Factorial Design and Response Surface Methodology. Food Chem. 2019, 281, 63–70. DOI: 10.1016/j.foodchem.2018.12.055.
- Huang, W.; Lan, Y.; Liao, W.; Lin, L.; Liu, G.; Xu, H.; Xue, J.; Guo, B.; Cao, Y.; Miao, J. P. Preparation, Characterization and Biological Activities of Egg White Peptides-Calcium Chelate. LWT. 2021, 149, 112035. DOI: 10.1016/j.lwt.2021.112035.
- Zhao, L.; CHen, Z. H.; CHen, H.; HUA, P.; LIU, B. Optimization of Celation of Juncao Ganoderma Lucidum Peptides with Selenium by Response Surface Methodology. Food Sci. 2017, 38(14), 187–192.
- Lin, J.; Cai, X.; Tang, M.; Wang, S. Preparation and Evaluation of the Chelating Nanocomposite Fabricated with Marine Algae Schizochytrium Sp. Protein Hydrolysate and Calcium. J. Agric. Food. Chem. 2015, 63(44), 9704–9714. DOI: 10.1021/acs.jafc.5b04001.
- Wang, X.; Li, M.; Li, M.; Mao, X.; Zhou, J.; Ren, F. Preparation and Characteristics of Yak Casein Hydrolysate–Iron Complex. International Journal Of Food Science & Technology. 2011, 46(8), 1705–1710. DOI: 10.1111/j.1365-2621.2011.02672.x.
- Ke, H.; Ma, R.; Liu, X.; Xie, Y.; Chen, J. Highly Effective Peptide-Calcium Chelate Prepared from Aquatic Products Processing Wastes: Stickwater and Oyster Shells. LWT. 2022, 168, 113947. DOI: 10.1016/j.lwt.2022.113947.
- Ünsal, M.; Işık-Gülsaç, I.; Üresin, E.; Budak, M. S.; Özgür-Büyüksakallı, K.; Sayar, A.; Aksoy, P.; Ünlü, N.; Okur, O.; Şahin, H., et al. Optimisation of Biomass Catalytic Depolymerisation Conditions by Using Response Surface Methodology. Waste Manag. Res. 2020, 38(3), 322–331. DOI: 10.1177/0734242X19890647.
- Blat, D.; Weiner, L.; Youdim, M. B. H.; Fridkin, M. A Novel Iron-Chelating Derivative of the Neuroprotective Peptide NAPVSIPQ Shows Superior Antioxidant and Antineurodegenerative Capabilities. J. Med. Chem. 2008, 51(1), 126–134. DOI: 10.1021/jm070800l.
- Yan, M.; Li, B.; Zhao, X.; Ren, G.; Zhuang, Y.; Hou, H.; Zhang, X.; Chen, L.; Fan, Y. Characterization of Acid-Soluble Collagen from the Skin of Walleye Pollock (Theragra Chalcogramma). Food Chem. 2008, 107(4), 1581–1586. DOI: 10.1016/j.foodchem.2007.10.027.
- Zhu, C.; Sun, Y.; Wang, Y.; Luo, Y.; Fan, D. The Preparation and Characterization of Novel Human-Like Collagen Metal Chelates. Mater. Sci. Eng. C. 2013, 33(5), 2611–2619. DOI: 10.1016/j.msec.2013.02.028.
- Vavrusova, M.; Skibsted, L. H. Calcium Nutrition. Bioavailability and Fortification. LWT - Food Sci. Technol. 2014, 59(2), 1198–1204. DOI: 10.1016/j.lwt.2014.04.034.
- Sun, N.; Wu, H.; Du, M.; Tang, Y.; Liu, H.; Fu, Y.; Zhu, B. Food Protein-Derived Calcium Chelating Peptides: A Review. Trends Food Sci. Technol. 2016, 58, 140–148. DOI: 10.1016/j.tifs.2016.10.004.
- Yu, Y.; Fan, D. Characterization of the Complex of Human-Like Collagen with Calcium. Biol. Trace Elem. Res. 2012, 145(1), 33–38. DOI: 10.1007/s12011-011-9167-x.
- Wang, X.; Gao, A.; Chen, Y.; Zhang, X.; Li, S.; Chen, Y. Preparation of Cucumber Seed Peptide-Calcium Chelate by Liquid State Fermentation and Its Characterization. Food Chem. 2017, 229, 487–494. DOI: 10.1016/j.foodchem.2017.02.121.
- Zhu, K.-X.; Wang, X.-P.; Guo, X.-N. Isolation and Characterization of Zinc-Chelating Peptides from Wheat Germ Protein Hydrolysates. J. Funct. Foods. 2015, 12, 23–32. DOI: 10.1016/j.jff.2014.10.030.
- Liu, F.-R.; Wang, L.; Wang, R.; Chen, Z.-X. Calcium-Binding Capacity of Wheat Germ Protein Hydrolysate and Characterization of Peptide–Calcium Complex. J. Agric. Food. Chem. 2013, 61(31), 7537–7544. DOI: 10.1021/jf401868z.
- Wang, L.; Ding, Y.; Zhang, X.; Li, Y.; Wang, R.; Luo, X.; Li, Y.; Li, J.; Chen, Z. Isolation of a Novel Calcium-Binding Peptide from Wheat Germ Protein Hydrolysates and the Prediction for Its Mechanism of Combination. Food Chem. 2018, 239, 416–426. DOI: 10.1016/j.foodchem.2017.06.090.
- Malison, A.; Arpanutud, P.; Keeratipibul, S. Chicken Foot Broth Byproduct: A New Source for Highly Effective Peptide-Calcium Chelate. Food Chem. 2021, 345, 128713. DOI: 10.1016/j.foodchem.2020.128713.
- Ren, Z.; Huang, G.; Jiang, J.; Chen, W. Preparation and Characteristic of Iron-Binding Peptides from Shrimp Processing Discards Hydrolysates. 2011.
- Silverstein, R. M.; Bassler, G. C. Spectrometric Identification of Organic Compounds. ACS Publications. 10.1021/ed039p546.
- Zhang, Y.; Ding, X.; Li, M. P. Preparation, Characterization and in vitro Stability of Iron-Chelating Peptides from Mung Beans. Food Chem. 2021, 349, 129101. DOI: 10.1016/j.foodchem.2021.129101.
- Zhao, L.; Huang, S.; Cai, X.; Hong, J.; Wang, S. A Specific Peptide with Calcium Chelating Capacity Isolated from Whey Protein Hydrolysate. J. Funct. Foods. 2014, 10, 46–53. DOI: 10.1016/j.jff.2014.05.013.
- Zhang, Z.; Zhou, F.; Liu, X.; Zhao, M. Particulate Nanocomposite from Oyster (Crassostrea Rivularis) Hydrolysates via Zinc Chelation Improves Zinc Solubility and Peptide Activity. Food Chem. 2018, 258, 269–277. DOI: 10.1016/j.foodchem.2018.03.030.
- Zhao, L.; Cai, X.; Huang, S.; Wang, S.; Huang, Y.; Hong, J.; Rao, P. Isolation and Identification of a Whey Protein-Sourced Calcium-Binding Tripeptide Tyr-Asp-Thr. Int. Dairy J. 2015, 40, 16–23. DOI: 10.1016/j.idairyj.2014.08.013.
- Zhao, L.; Huang, Q.; Huang, S.; Lin, J.; Wang, S.; Huang, Y.; Hong, J.; Rao, P. Novel Peptide with a Specific Calcium-Binding Capacity from Whey Protein Hydrolysate and the Possible Chelating Mode. J. Agric. Food. Chem. 2014, 62(42), 10274–10282. DOI: 10.1021/jf502412f.
- Zhang, L.; Lin, Y.; Wang, S. Purification of Algal Calcium-Chelating Peptide and Its Physical Chemical Properties. J. Aquat. Food Prod. Technol. 2018, 27(4), 518–530. DOI: 10.1080/10498850.2018.1449153.
- Wang, X.; Zhang, Z.; Xu, H.; Li, X.; Hao, X. Preparation of Sheep Bone Collagen Peptide–Calcium Chelate Using Enzymolysis-Fermentation Methodology and Its Structural Characterization and Stability Analysis. Rsc. Adv. 2020, 10(20), 11624–11633. DOI: 10.1039/D0RA00425A.
- Fathima, N. N.; Bose, M. C.; Rao, J. R.; Nair, B. U. Stabilization of Type I Collagen Against Collagenases (Type I) and Thermal Degradation Using Iron Complex. J. Inorg. Biochem. 2006, 100(11), 1774–1780. DOI: 10.1016/j.jinorgbio.2006.06.014.
- Qu, W.; Feng, Y.; Xiong, T.; Li, Y.; Wahia, H.; Ma, H. Preparation of Corn ACE Inhibitory Peptide-Ferrous Chelate by Dual-Frequency Ultrasound and Its Structure and Stability Analyses. Ultrason. Sonochem. 2022, 83, 105937. DOI: 10.1016/j.ultsonch.2022.105937.
- Chen, M.; Ji, H.; Zhang, Z.; Zeng, X.; Su, W.; Liu, S. A Novel Calcium-Chelating Peptide Purified from Auxis Thazard Protien Hydrolysate and Its Binding Properties with Calcium. J. Funct. Foods. 2019, 60, 103447. DOI: 10.1016/j.jff.2019.103447.
- Mokni Ghribi, A.; Maklouf Gafsi, I.; Sila, A.; Blecker, C.; Danthine, S.; Attia, H.; Bougatef, A.; Besbes, S. Effects of Enzymatic Hydrolysis on Conformational and Functional Properties of Chickpea Protein Isolate. Food Chem. 2015, 187, 322–330. DOI: 10.1016/j.foodchem.2015.04.109.
- Liu, Y.; Li, X.; Chen, Z.; Yu, J.; Wang, F.; Wang, J. Characterization of Structural and Functional Properties of Fish Protein Hydrolysates from Surimi Processing By-Products. Food Chem. 2014, 151, 459–465. DOI: 10.1016/j.foodchem.2013.11.089.
- Gharbi, N.; Labbafi, M. Influence of Treatment-Induced Modification of Egg White Proteins on Foaming Properties. Food Hydrocoll. 2019, 90, 72–81. DOI: 10.1016/j.foodhyd.2018.11.060.
- Omana, D. A.; Xu, Y.; Moayedi, V.; Betti, M. Alkali-Aided Protein Extraction from Chicken Dark Meat: Chemical and Functional Properties of Recovered Proteins. Process Biochem. 2010, 45(3), 375–381. DOI: 10.1016/j.procbio.2009.10.010.
- Noman, A.; Qixing, J.; Xu, Y.; Ali, A. H.; Al-Bukhaiti, W. Q.; Abed, S. M.; Xia, W. Influence of Degree of Hydrolysis on Chemical Composition, Functional Properties, and Antioxidant Activities of Chinese Sturgeon (Acipenser Sinensis) Hydrolysates Obtained by Using Alcalase 2.4L. J. Aquat. Food Prod. Technol. 2019, 28(6), 583–597. DOI: 10.1080/10498850.2019.1626523.
- Amza, T.; Balla, A.; Tounkara, F.; Man, L.; Zhou, H. M. Effect of Hydrolysis Time on Nutritional, Functional and Antioxidant Properties of Protein Hydrolysates Prepared from Gingerbread Plum (Neocarya Macrophylla) Seeds. Int. Food Res. J. 2013, 20(5), 2081.
- Noman, A.; Xu, Y.; AL-Bukhaiti, W. Q.; Abed, S. M.; Ali, A. H.; Ramadhan, A. H.; Xia, W. Influence of Enzymatic Hydrolysis Conditions on the Degree of Hydrolysis and Functional Properties of Protein Hydrolysate Obtained from Chinese Sturgeon (Acipenser Sinensis) by Using Papain Enzyme. Process Biochem. 2018, 67, 19–28. DOI: 10.1016/j.procbio.2018.01.009.
- Jiang, J.; Chen, J.; Xiong, Y. L. Structural and Emulsifying Properties of Soy Protein Isolate Subjected to Acid and Alkaline PH-Shifting Processes. J. Agric. Food. Chem. 2009, 57(16), 7576–7583. DOI: 10.1021/jf901585n.
- Zhang, T.; Jiang, B.; Mu, W.; Wang, Z. Emulsifying Properties of Chickpea Protein Isolates: Influence of PH and NaCl. Food Hydrocoll. 2009, 23(1), 146–152. DOI: 10.1016/j.foodhyd.2007.12.005.
- Ge, Y.; Sun, A.; Ni, Y.; Cai, T. Some Nutritional and Functional Properties of Defatted Wheat Germ Protein. J. Agric. Food. Chem. 2000, 48(12), 6215–6218. DOI: 10.1021/jf000478m.
- Wang, Y.; Wang, Z.; Handa, C. L.; Xu, J. Effects of Ultrasound Pre-Treatment on the Structure of β-Conglycinin and Glycinin and the Antioxidant Activity of Their Hydrolysates. Food Chem. 2017, 218, 165–172. DOI: 10.1016/j.foodchem.2016.09.069.
- Saiga, A.; Tanabe, S.; Nishimura, T. Antioxidant Activity of Peptides Obtained from Porcine Myofibrillar Proteins by Protease Treatment. J. Agric. Food. Chem. 2003, 51(12), 3661–3667. DOI: 10.1021/jf021156g.
- Bao, X.-L.; Lv, Y.; Yang, B.-C.; Ren, C.-G.; Guo, S.-T. A Study of the Soluble Complexes Formed During Calcium Binding by Soybean Protein Hydrolysates. J. Food Sci. 2008, 73(3), C117–C121. DOI: 10.1111/j.1750-3841.2008.00673.x.
- Jiang, L.; Wang, B.; Li, B.; Wang, C.; Luo, Y. Preparation and Identification of Peptides and Their Zinc Complexes with Antimicrobial Activities from Silver Carp (Hypophthalmichthys Molitrix) Protein Hydrolysates. Food Res. Int. 2014, 64, 91–98. DOI: 10.1016/j.foodres.2014.06.008.
- Siahbalaei, R.; Kavoosi, G.; Noroozi, M. Protein Nutritional Quality, Amino Acid Profile, Anti-Amylase and Anti-Glucosidase Properties of Microalgae: Inhibition and Mechanisms of Action Through in vitro and in silico Studies. LWT. 2021, 150, 112023. DOI: 10.1016/j.lwt.2021.112023.