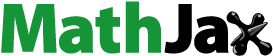
Abstract
Content
Plant-based natural products have served as sources of remedies against pathogenic microorganisms. Although the biological activities of Viscum (Santalaceae) species are widely recognized, there is no scientific evidence for Viscum tuberculatum A. Rich. in Ethiopia.
Objective
To investigate the antimicrobial, acute toxicity, anti-inflammatory properties and phytochemical constituents of an aqueous extract of V. tuberculatum from Ethiopia.
Materials and methods
The antibacterial activity of the aqueous leaf extract of V. tuberculatum was tested against Escherichia coli, Pseudomonas aeruginosa and Staphylococcus aureus. The minimum inhibitory concentration (MIC) and minimum bactericidal concentration (MBC) of this extract were determined using the broth macrodilution method. The acute toxicity and anti-inflammatory effects of the extract were investigated using standard procedures on female and male white albino mice, aged 8 and 10 weeks, respectively. The phytochemical constituents of V. tuberculatum were determined using LC–MS QTOF.
Results
The MIC and MBC values against S. aureus were found to be 6.25 and 100 mg/mL. The LD50 value was more than 2000 mg/kg body weight of the mouse. The 400 mg/kg dose exerts 87% inhibition after 5 h of carrageenan injection. Twenty-five different metabolites, mainly flavonoids, phenolic acids and alkaloids, were identified.
Conclusions
These findings demonstrate the potential antimicrobial and anti-inflammatory potential of the aqueous extract of V. tuberculatum.
Introduction
Antibiotic-resistance has caused a considerable threat in the treatment of human and animal infectious diseases (Leonard et al. Citation2022). The problem with the multi-drug resistance profiles of the most commonly prescribed drugs is well recognized in several African countries, including Ethiopia (Efa et al. Citation2019). A recent literature review on the status of antimicrobial resistance in Ethiopia revealed a high prevalence of resistance to common and clinically important bacteria, including Staphylococcus aureus (Fujita et al. Citation2022). The emergence of antimicrobial resistance even to third generation drugs has sparked a great interest in discovering new antimicrobial agents from natural sources, such as plants (Malada et al. Citation2022).
Viscum L. genera (Santalaceae, Viscaceae), commonly known as mistletoe, comprises about 100 species of mistletoe. These species are distributed in Africa, Asia, Australia and Europe (Polhill and Wiens Citation1998). Several Viscum species are rich in diverse biologically active secondary metabolites that treat various health ailments, including antimicrobial activities against various pathogenic bacteria (Amabeoku et al. Citation1998; Erturk et al. Citation2003; Ahmmed et al. Citation2013; Assaf et al. Citation2013; Nag et al. Citation2016).
Viscum tuberculatum A. Rich. (syn. V. camporum, V. holstii, V. spragueanum and V. stuhlmannii) is a hemi-parasitic shrub that grows on a wide range of host plants (Gilbert Citation1989; Polhill and Wiens Citation1998; Yirgu and Tesfaye Citation2018). It is distributed in several African countries (Polhill and Wiens Citation1998). In traditional medicine, it treats pneumonia and the liver (Idris Citation2004), diarrhoea, vomiting, fevers, and headaches (Issa Citation2015), cough (Demie et al. Citation2018), malaria, and acute diseases (Abdeta et al. Citation2020). It also serves as food for wild animals (Bussmann Citation2006). The phytochemical constituents and biological activity of V. tuberculatum are not well known. Therefore, this study investigated the phytochemical constituents, antimicrobial activity, acute toxicity and anti-inflammatory activities of the aqueous extract of V. tuberculatum from Ethiopia.
Materials and methods
Plant collection and identification
Matured, fresh and healthy leaves of V. tuberculatum were collected from Teclea nobilis Delile in the Shashemene District of Central Ethiopia between April and June 2018. The taxonomic identity of V. tuberculatum was authenticated by Mr. Melaku Wondafrash a herbarium specialist at Addis Ababa University National Herbarium (ETH), Ethiopia. A voucher specimen (AY24) was deposited at ETH.
Preparation of extracts
The leaf samples of the mistletoe were washed in running tap water, air-dried under shade at room temperature, and finely powdered using an electric grinder. The dried powder (20 g) was macerated in 200 mL of sterile distilled water at room temperature. The solution was agitated on a daily basis for three consecutive days, filtered through Whatman no. 1 filter paper and dried with a lyophilizer (CHRIST®, Osterode, Germany).
Antibacterial assay
Test microorganisms
The bacteria (Escherichia coli ATTC 25922, Pseudomonas aeruginosa ATTC 27853 and Staphylococcus aureus ATCC 25923) were obtained from the Ethiopian Public Health Institute (EPHI), Addis Ababa, Ethiopia. These bacteria were sub-cultured overnight in nutrient agar medium at 35 ± 2 °C. Four to five isolated colonies of the same morphological type of bacteria were picked with a sterile loop and dissolved in a 0.9% physiological saline solution.
Antibacterial activity of plant extracts
The antimicrobial activity of V. tuberculatum was tested using Whatman filter paper no. 1 disks impregnated with plant extract as described by Hudzicki (Citation2009) and Ghaedi et al. (Citation2015). The turbidity of the bacterial suspension was adjusted to 0.5 McFarland standards (1–2 × 108 CFU/mL). Bacterial suspension (100 µL) was transferred and gently spread over the Mueller–Hinton Agar (MHA) surface using a sterile cotton swab. Disks were aseptically placed on an MHA plate inoculated with each of the test bacteria, and incubated at 35 ± 2 °C for 16–18 h.
Determination of the minimum inhibitory concentration (MIC)
The MIC of V. tuberculatum against bacterial strains was determined by a rapid p-iodonitrotetrazolium chloride (INT; ROTH, Carl Roth GmbH Co., Karlsruhe, Germany) colorimetric assay using the macrodilution method (Chukwujekwu and van Staden 2016; Mfengwana and Mashele Citation2016). A two-fold serial dilution of plant extract (1 mL) ranging from 100 up to 0.098 mg/mL was aseptically transferred into 10 sterile test tubes containing 2 mL of Mueller–Hinton Broth (MHB). Test tubes no. 11 (containing MHB and test bacterium) and 12 (containing MHB, test bacterium and standard reference drug) were prepared as negative and positive controls, respectively. Finally, a 100 µL bacterial suspension adjusted to 0.5 McFarland standards was added to each of these 12 test tubes. These test tubes were incubated at 35 ± 2 °C for 18 h (Smith et al. Citation2020). Then, 60 µL of INT (2 mg/mL of INT in sterile distilled water) was added to all test tubes and further incubated for 2 h at 35 ± 2 °C. Active bacterial growth reduces the yellow INT dye to a pink colour (Sati et al. Citation2019). The MIC was determined as the lowest concentration of the aqueous extract that resulted in the absence of bacterial growth in the test tube detected by the unaided eye and the absence of colour change (Eloff Citation1998).
Determination of minimum bactericidal concentration (MBC)
The MBC was determined using the macrodilution broth method as described by Yilmaz (Citation2012) with slight modification. Aliquots (100 µL) of each gradient concentrated solution from the MIC activity were transferred into 12 test tubes filled with fresh MHB medium. These tubes were incubated at 35 ± 2 °C for 48 h. The MBC endpoint was considered as the lowest concentration of plant extract that showed no colour change after the addition of INT, which showed a complete absence of microbial growth.
Ethical issues
The use of Swiss albino mice in the investigation of acute toxicity and anti-inflammatory activity of V. tuberculatum was approved by the College of Natural and Computational Sciences Institutional Review Board (CNS-IRB) of Addis Ababa University (Ref: CNCSDO/424/13/2021), Ethiopia. All experimental procedures conducted in the study were compliant with the internationally accepted principles for laboratory animal use and care.
Acute oral toxicity evaluation
The acute toxicity of the aqueous extract of V. tuberculatum was determined according to the up-and-down procedure of Organization for Economic Cooperation and Development (OECD) Guidelines No. 425 (OECD Citation2008) with slight modification. Twenty-five Swiss female albino mice (healthy, nulliparous, non-pregnant and 8–10 weeks old) were obtained from EPHI Animal House. These mice were randomly divided into five groups (n = 5) based on body weight (bw). They were acclimatized for five days to a 12 h light/dark cycle and had free access to food and water. All the mice fasted for food with free access to water the night before the experiment. Mice in groups I and II served as controls and received tap water and physiological saline (vehicle) solution, respectively. Groups III, IV and V mice received 175, 550 and 2000 mg/kg bw of dry plant extract dissolved in physiological saline, respectively. Food was provided after 2 h of dosing. The signs of toxicity, behavioural changes and mortality of mice were observed during the first 30 min, 4 h, 24 h, and once a day for 14 successive days after administration of the treatments.
The bw of mice was taken before treatment (day 0), on day 7, and on day 14 after the administration of crude plant extract. At the end of 14 days of observation, the liver, kidneys, heart and lungs of each mouse were removed and weighed. The 50% lethal dose (LD50) was estimated. The percent body and the relative vital organ weights of animals were calculated as stated by Porwal et al. (Citation2017).
Anti-inflammatory activity
The anti-inflammatory activity of the plant extract was evaluated on carrageenan-induced inflammatory paw oedema, as described by Adnan et al. (Citation2019) and Bezerra et al. (Citation2017) with some modifications. Swiss male albino mice were divided based on bw into four groups of six animals in each. These animals (healthy, 8–10 weeks old) were deprived of food overnight and allowed free access to water prior to the experiment. Group I mice were treated with vehicle as a negative control, group II with Indomethacin (10 mg/kg bw) served as a positive control, and groups III and IV received 200 and 400 mg/kg bw of plant extract, respectively. After 1 h of oral administration of these substances, oedema was induced by injecting a suspension of 0.1 mL of 1% carrageenan (w/v) in physiological saline into the sub-plantar tissue of the right hind paw of each mouse. The initial thickness of the hind paw was measured before the injection of carrageenan suspension. Subsequent measurement of paw thickness was measured at 1 h intervals for 5 h using a Digital LCD Vernier Caliper Gauge micrometer. The percent inhibition of paw volume was calculated using the following equation (Boutennoun et al. Citation2017).
where Vt and Vo denote the right hind paw thickness volume (in milliliters) at time t and before carrageenan injection, respectively.
Liquid chromatography–mass spectrometry (LC–MS) analysis
The crude aqueous leaf extract of V. tuberculatum with active antimicrobial activity against a test pathogen was analysed on an Agilent HP 1260 Infinity Series liquid chromatograph (Agilent Technologies, Santa Clara, CA) equipped with a diode array detector (DAD) system (Agilent Technologies, Santa Clara, CA) and coupled to a quadrupole-time of flight (Q-TOF) mass spectrometer model G6540B (Agilent Technologies, Santa Clara, CA) with a dual electrospray ionization (ESI) source (Agilent Technologies, Santa Clara, CA). Chromatographic separation was conducted using a Zorbax Eclipse Plus C-18 column (4.6 × 50 mm, with 3.5 µm particle size), SepaChrom Srl, Rho, Italy) at 37 °C. The elution solvent consists of eluent A: 0.1% (v/v) formic acid (FA) in water and, eluent B: 0.1% (v/v) FA in acetonitrile at a flow rate of 0.5 mL/min. The gradient elution was started with 5% B to 70% B for the first 4 min, 70–80% B in 3 min, 80% B to 100% B in 2 min, 100% B in 4–5 min, and equilibrating at 95% A, 10–14 min. UV spectra were collected by DAD every 0.4 s from 190 to 750 nm with a resolution of 2 nm. MS parameters were set using Mass Hunter Qualitative Analysis Software version B.06.00 (Agilent Technologies, Santa Clara, CA), raw data were processed based on retention time and characteristic behaviour of MS, including the exact mass, quasimolecular ions and in-source fragmentation, data were compared with known compounds in an in-house plant database and existing literature. The acquisition was performed from 100 to 1700 m/z, with three scans per second. Positive identifications of plant metabolites were considered for analysis if the compound was detected with a mass error of below 10 ppm and with a sufficient score. Analytical and LC–MS grade chemicals were used throughout the metabolic profiling.
Statistical analysis
Experimental results on the antimicrobial activity, anti-inflammatory effects and acute toxicity tests are presented as mean ± standard deviation (SD). These data were subjected to analysis of variance (ANOVA) using SAS v. 9 Statistical Software (Cary, NC). A Tukey’s HSD post hoc test for multiple comparisons was performed to detect the differences between treatment means. A p≤ 0.05 was considered statistically significant. Descriptive statistical analysis was used for non-parametric data.
Results
Anti-bacterial activity
The aqueous leaf extract of V. tuberculatum inhibited the bacterial growth of E. coli, P. aeruginosa and S. aureus. The macrodilution determination of the MIC and MBC was conducted against S. aureus for its higher susceptibility compared with E. coli and P. aeruginosa. Hence, erythromycin was selected as a standard antibacterial agent in determining the MIC and MBC of the plant extract against S. aureus. The MIC () and MBC () showed the growth inhibition of S. aureus at ≤6.25 and ≥100 mg/mL, respectively. The negative control in both MIC and MBC showed active growth, indicated by a colour change from yellow to pink. The standard drug (in test tube 12) inhibited the growth of S. aureus so that the solution was colourless. In contrast to the lower MIC value (≤6.25), the MBC test revealed S. aureus growth at all test concentrations, which indicated the MBC value is higher than 100 mg/mL.
Acute oral toxicity
Behavioural changes, mortality and body weight gain of mice
The oral administration of three doses (175, 550 and 2000 mg/kg bw) of the aqueous V. tuberculatum extract did not show any behavioural changes compared to the controls. In addition, no cases of coma or mortality of mice were observed in the two-weeks period of observation. On the other hand, all mice showed a progressive weight gain during the two weeks of observation. Nevertheless, the weight gain in treated mice showed a non-significant difference compared with the control animals ().
Table 1. The body weight of mice before and after treatments with crude extract, vehicle and tap water.
Effect of acute oral toxicity test on vital organ weight of mice
The effect of aqueous extract on the relative organ weights of the heart, lungs, liver and kidneys of all treated mice was determined at the end of the acute toxicity test. There was no significant difference (p > 0.05) in the organ weights of all the treated animals (). The visual inspection of these organs also revealed no evidence of injury to these vital organs at all doses.
Table 2. The relative vital organ to body weight ratio of mice after acute oral toxicity test.
Anti-inflammatory effect
The in vivo anti-inflammatory activity of V. tuberculatum at 200 and 400 mg/kg bw in carrageenan-induced paw oedema is shown in . The extract caused dose-dependent inhibition of paw oedema in the 5 h of observation. The highest percent (i.e., 87%) inhibition of oedema was observed in the 400 mg/kg bw after 5 h after carrageenan injection. A significant difference in the percentage of inhibition between indomethacin and the plant extract occurred in the 200 mg/kg dose after 2 h of carrageenan injection. A similar significant difference occurred between the 200 mg/kg dose and the control after 4–5 h of carrageenan administration. The percentage inhibition of paw oedema in mice treated with indomethacin increased to 85% 5 h after being treated with carrageenan solution ().
Table 3. Thickness of paw oedema and inhibition percentage of paw oedema induced by carrageenan suspension.
Identification of metabolites by LC–MS
Twenty-five different metabolomes were identified in the aqueous leaf extract of V. tuberculatum (). Eighteen metabolomes were identified in the positive ionization mode and 14 in the negative ionization mode. Six compounds were commonly found in both modes of analysis. These 25 compounds were distributed into nine different classes of compounds, viz., flavonoids (13), phenolic acids (3), alkaloids (2), sugars (2), indoles (1), coumarins (1), lignans (1), carboxylic acids (1) and polyphenols (1). The retention times, mass data and molecular formulas of these metabolomes are listed in .
Table 4. LC–MS QTOF identified metabolites in the aqueous extract of V. tuberculatum.
Discussion
In this study, the aqueous leaf extract of V. tuberculatum inhibited the growth of E. coli ATTC 25922, P. aeruginosa ATTC 27853 and S. aureus ATCC 25923. A relatively higher inhibitory activity of the plant extract was observed against S. aureus (Gram-positive bacteria) than the two Gram-negative bacteria, which may be attributed to the presence of lipopolysaccharide in Gram-positive bacteria (Breijyeh et al. Citation2020). Previous studies have also showed the antimicrobial properties of Viscum species. For instance, the hexane, ethyl acetate, acetone and methanol extracts of V. rotundifolium L.f. showed antimicrobial activity against E. coli, P. aeruginosa, S. aureus and Enterococcus faecalis (Malada et al. Citation2022). The methanol extract of V. articulatum Burm. F. has also inhibited S. aureus and E. coli (Nag et al. Citation2016). In addition, the ethyl acetate, chloroform, ethanol, methanol and water leaf extracts of V. album L. inhibited E. coli, P. aeruginosa, S. aureus and other Gram-positive and Gram-negative bacteria (Hussain et al. Citation2011).
The acute toxicity study carried out using the 175, 550 and 2000 mg/kg bw doses did not reveal any observable behavioural changes or deaths of mice. The insignificant weight gain following the administration of the plant extract suggests the physiological wellness of mice (Belghoul et al. Citation2020). This indicates that the medium lethal dose (LD50) value of the orally administered V. tuberculatum is greater than 2000 mg/kg bw, which can classify this plant extract under category 5 of the Globally Harmonized System of Classification and Labeling of Chemicals (UN Citation2011). A previous study into the acute toxicity of methanolic leaf extracts of V. capitellatum Sm. revealed the safety of this extract up to 2000 mg/kg bw but toxic at 5000 mg/kg (Jadhav et al. Citation2022). In addition, the ethyl acetate–water extracts of V. album exhibited no mortality in animals at a dose of 5000 mg/kg (Pozdnyakov et al. Citation2021).
In the in vivo anti-inflammatory activity, a significantly higher anti-inflammatory activity of V. tuberculatum was shown at a 400 mg/kg dose induced by the carrageenan suspension. The studies on V. album (Murthuza and Manjunatha Citation2018), V. articulatum (Leu et al. Citation2006), V. coloratum (Hwang et al. Citation2006) and V. cruciatum Sieber ex Boiss. (Assaf et al. Citation2013) have also shown the anti-inflammatory properties of these Viscum species.
The phytochemical analysis of V. tuberculatum revealed 25 metabolites, belonging to different classes of natural compounds, such as flavonoid derivatives, phenolic acids, alkaloids, sugars, indoles, coumarins, lignans, carboxylic acids and polyphenols. Some of these natural products are recognized for their antimicrobial and anti-inflammatory activities. For instance, quinic acid (Adamczak et al. Citation2019) and gallic acid (Olmedo-Juarez et al. Citation2019) are known for their antimicrobial against E. coli, P. aeruginosa and S. aureus and anti-inflammatory activities in previous studies. In this study, they are found to account for 39.41 and 13.11% of the total abundance. 4-Hydroxybenzoic acid, which accounted for 71.07% of the total abundance in this study, has been reported to antimicrobial and fungicidal activity (Anand et al. Citation2019). Similarly, metabolites such as (–)-epicatechin (Bettaieb et al. Citation2016; Araujo et al. Citation2019), gallic acid (Li et al. Citation2017) and isorhamnetin (Tian et al. Citation2021) have exhibited both antimicrobial and anti-inflammatory properties. Epicatechin and isorhamnetin, which were identified in this study accounted for 29.02% and 70.13% of total abundance, respectively. The antioxidant activity of quinic acid (Karaman et al. Citation2021), catechin and gallic acid (Đorđević et al. Citation2018) were also reported in previous studies. Additionally, marmesin (which account for 95.85% of total abundance) has been reported for its potent anti-proliferative property (Dong et al. Citation2018), and trigonelline (that account for 21.07% of total abundance) has antidiabetic, antioxidant, anti-inflammatory and neuroprotective effects (Khalili et al. Citation2018), respectively.
Conclusions and recommendations
V. tuberculatum is one of the most widely distributed mistletoes used in the treatment of various health ailments. Understanding the limitation of data on the haematology and pathology of experimental mice, this study provides the first scientific report on the antimicrobial and anti-inflammatory activities of the aqueous V. tuberculatum collected from Teclea nobilis in Ethiopia. The identified secondary metabolites are, in part, responsible for the biological activities of the plant. This highlights the importance of using the aqueous extract of V. tuberculatum as an alternative therapeutic source in the future. Therefore, it is necessary to collect and investigate the contributions of pure compounds for their therapeutic potential, including other human and animal diseases. As the existence of several species of parasitic plants, including V. tuberculatum, is threatened by various factors, there is a need for more attention to conserve these species for their ecological and therapeutic benefits in the future.
Author contributions
AY developed the project, collected the samples; AY and YM proposed and planned the work; AY and AE performed animal experiments; AS and FV performed metabolomic analysis; AY analysed the data and prepared the original manuscript; YM supervised the work; YM and FV reviewed the manuscript and provided comments. All authors read and approved the manuscript.
Acknowledgements
We thank Addis Ababa University (AAU) and the EPHI for providing technical support and bacterial strains. AY is grateful to Marlies Spohn, Helmut Spohn, Dr. Adey Feleke, Dr. Rahul Datta, Mr. Melaku Wondafrash and his colleagues at Ethiopian Forest Development, Central Ethiopia Center and AAU for their unreserved assistance and encouragement throughout his study.
Disclosure statement
The authors report that there are no competing interests to declare.
Additional information
Funding
References
- Abdeta D, Amante M, Tamiru Y. 2020. Survey on ethnobotany and medicinal animals at Sayo and Hawa Gelan Districts of Kelem Wollega Zone, Western Ethiopia. Biomed J Sci Techn Res. 28:21408–21420.
- Adamczak A, Ożarowski M, Karpiński TM. 2019. Antibacterial activity of some flavonoids and organic acids widely distributed in plants. J Clin Med. 9(1):109.
- Adnan M, Chy MN, Kamal ATMM, Barlow JW, Faruque MO, Yang X, Uddin SB. 2019. Evaluation of anti-nociceptive and anti-inflammatory activities of the methanol extract of Holigarna caustica (Dennst.) Oken leaves. J Ethnopharmacol. 236:401–411.
- Ahmmed F, Sarkar KK, Hossain ML, Hossin A, Mondal H, Islam J, Ahmed MI. 2013. Phytochemical and pharmacological investigation of Viscum monoicum (whole plant). Pharmacologyonline. 1:237–243.
- Amabeoku GJ, Leng MJ, Syce JA. 1998. Antimicrobial and anticonvulsant activities of Viscum capense. J Ethnopharmacol. 61(3):237–241.
- Anand S, Deighton M, Livanos G, Morrison PD, Pang ECK, Mantri N. 2019. Antimicrobial activity of Agastache honey and characterization of its bioactive compounds in comparison with important commercial honey. Front Microbiol. 10:263.
- Araujo CRR, de Melo Silva T, Dos Santos MG, Ottoni MH, de Souza Fagundes EM, de Sousa Fontoura H, de Melo GE, de Carvalho Alcantara AF. 2019. Anti-inflammatory and cytotoxic activities of the extracts, fractions, and chemical constituents isolated from Luehea ochrophylla Mart. BMC Complement Altern Med. 19(1):284.
- Assaf AM, Haddadin RN, Aldouri NA, Alabbassi R, Mashallah S, Mohammad M, Bustanji Y. 2013. Anti-cancer, anti-inflammatory and anti-microbial activities of plant extracts used against hematological tumors in traditional medicine of Jordan. J Ethnopharmacol. 145(3):728–736.
- Belghoul M, Baghiani A, Khennouf S, Arrar L. 2020. Sub-acute oral toxicity and in vivo antioxidant properties of Oxalis cernua. S Afr J Bot. 133:91–97.
- Bettaieb A, Cremonini E, Kang H, Kang J, Haj FG, Oteiza PI. 2016. Anti-inflammatory actions of (–)-epicatechin in the adipose tissue of obese mice. Int J Biochem Cell Biol. 81(Pt B):383–392.
- Bezerra AN, Massing LT, de Oliveira RB, Mourao RH. 2017. Standardization and anti-inflammatory activity of aqueous extract of Psittacanthus plagiophyllus Eichl. (Loranthaceae). J Ethnopharmacol. 202:234–240.
- Boutennoun H, Boussouf L, Kebieche M, Al-Qaoud K, Madani K. 2017. In vivo analgesic, anti-inflammatory and antioxidant potentials of Achillea odorata from north Algeria. S Afr J Bot. 112:307–313.
- Breijyeh Z, Jubeh B, Karaman R. 2020. Resistance of Gram-negative bacteria to current antibacterial agents and approaches to resolve it. Molecules. 25(6):1340.
- Bussmann RW. 2006. Ethnobotany of the Samburu of Mt. Nyiru, South Turkana, Kenya. J Ethnobiol Ethnomed. 2:35.
- Chukwujekwu JC, van Staden J. 2016. In vitro antibacterial activity of Combretum edwardsii, Combretum krausii, and Maytenus nemorosa and their synergistic effects in combination with antibiotics. Front Pharmacol. 7:208.
- Demie G, Negash M, Awas T. 2018. Ethnobotanical study of medicinal plants used by indigenous people in and around Dire Sheikh Hussein heritage site of South-eastern Ethiopia. J Ethnopharmacol. 220:87–93.
- Dong L, Xu W-W, Li H, Bi K-H. 2018. In vitro and in vivo anticancer effects of marmesin in U937 human leukemia cells are mediated via mitochondrial-mediated apoptosis, cell cycle arrest, and inhibition of cancer cell migration. Oncol Rep. 39(2):597–602.
- Đorđević N, Todorović N, Novaković I, Pezo L, Pejin B, Maraš V, Tešević V, Pajović S. 2018. Antioxidant activity of selected polyphenolics in yeast cells: the case study of Montenegrin Merlot wine. Molecules. 23(8):1971.
- Efa F, Alemu Y, Beyene G, Gudina EK, Kebede W. 2019. Methicillin-resistant Staphylococcus aureus carriage among medical students of Jimma University, Southwest Ethiopia. Heliyon. 5(1):e01191.
- Eloff JN. 1998. A sensitive and quick microplate method to determine the minimal inhibitory concentration of plant extracts for bacteria. Planta Med. 64(8):711–713.
- Erturk O, Kati H, Yayli N, Demirbag Z. 2003. Antimicrobial activity of Viscum album L. subsp. abietis (Wiesb). Turk J Biol. 27:255–258.
- Fujita AW, Werner K, Jacob JT, Tschopp R, Mamo G, Mihret A, Abdissa A, Kempker R, Rebolledo PA. 2022. Antimicrobial resistance through the lens of one health in Ethiopia: a review of the literature among humans, animals, and the environment. Int J Infect Dis. 119:120–129.
- Ghaedi M, Yousefinejad M, Safarpoor M, Khafri HZ, Purkait MK. 2015. Rosmarinus officinalis leaf extract mediated green synthesis of silver nanoparticles and investigation of its antimicrobial properties. J Ind Eng Chem. 31:167–172.
- Gilbert MG. 1989. Viscaceae. In: Egziabher TB, OlovHedberg MT, IbFriis IH, Edwards S, editors. Flora of Ethiopia. Vol. 3. Sweden: The National Herbarium, Biology Department, Science Faculty, Addis Ababa University, Ethiopia and The Department of Systematic Botany, Uppsala University; p. 375–378.
- Hudzicki J. 2009. Kirby-Bauer disk diffusion susceptibility test protocol. Am Soc Microbiol. 15:55–63.
- Hussain MA, Khan MQ, Hussain N, Habib T. 2011. Antibacterial and antifungal potential of leaves and twigs of Viscum album L. J Med Plant Res. 5:5545–5549.
- Hwang T-L, Leu Y-L, Kao S-H, Tang M-C, Chang H-L. 2006. Viscolin, a new chalcone from Viscum coloratum, inhibits human neutrophil superoxide anion and elastase release via a cAMP-dependent pathway. Free Radic Biol Med. 41(9):1433–1441.
- Idris EOE. 2004. The effect of Loranthus acaciae and Nymphaea lotus extracts on chicks and rats [MSc thesis]. Sudan: Department of Veterinary Medicine, Pharmacology and Toxicology University of Khartoum.
- Issa A. 2015. Ethno medicinal study of plants in Jigjiga woreda, Eastern Ethiopia [MSc thesis]. Addis Ababa, Ethiopia: Addis Ababa University.
- Jadhav VS, Ghawate VB, Jadhav RB, Surana SJ, Kalaskar MG. 2022. Neuropharmacological activities of methanolic extract of leaves of Viscum capitellatum Smith. Bull Pharm Sci Assiut Univ. 45(1):129–137.
- Karaman M, Tesanovic K, Gorjanovic S, Pastor FT, Simonovic M, Glumac M, Pejin B. 2021. Polarography as a technique of choice for the evaluation of total antioxidant activity: the case study of selected Coprinus comatus extracts and quinic acid, their antidiabetic ingredient. Nat Prod Res. 35(10):1711–1716.
- Khalili M, Alavi M, Esmaeil-Jamaat E, Baluchnejadmojarad T, Roghani M. 2018. Trigonelline mitigates lipopolysaccharide-induced learning and memory impairment in the rat due to its anti-oxidative and anti-inflammatory effect. Int Immunopharmacol. 61:355–362.
- Leonard AFC, Morris D, Schmitt H, Gaze WH. 2022. Natural recreational waters and the risk that exposure to antibiotic resistant bacteria poses to human health. Curr Opin Microbiol. 65:4–46.
- Leu Y-L, Hwang T-L, Chung Y-M, Hong P-Y. 2006. The inhibition of superoxide anion generation in human neutrophils by Viscum coloratum. Chem Pharm Bull. 54(7):1063–1066.
- Li Z-Y, Liu M, Dawuti G, Dou Q, Ma Y, Liu H-G, Aibai S. 2017. Antifungal activity of gallic acid in vitro and in vivo. Phytother Res. 31(7):1039–1045.
- Malada PM, Mogashoa MM, Masoko P. 2022. The evolution of cytotoxic effects, antimicrobial activity, antioxidant activity and combination effect of Viscum rotundifolium and Mystroxylon aethiopicum. S Afr J Bot. 147:790–798.
- Mfengwana PMAH, Mashele SS. 2016. Antimicrobial activity screening of Philenoptera violacea (Klotzsch) Schrire and Xanthocercis zambesiaca (Baker) Dumaz-Le-Grand. J. Pharm Sci Res. 8:1132–1135.
- Murthuza S, Manjunatha BK. 2018. In vitro and in vivo evaluation of anti-inflammatory potency of Mesua ferrea, Saraca asoca, Viscum album and Anthocephalus cadamba in murine macrophages raw 264.7 cell lines and Wistar albino rats. Beni-Seuf Univ J Appl Sci. 7:719–723.
- Nag M, Mukherjee PK, Biswas R, Chanda J, Kar A. 2016. Evaluation of antimicrobial potential of some Indian Ayurvedic medicinal plants. Pharmacogn J. 8(6):525–533.
- Olmedo-Juarez A, Briones-Robles TI, Zaragoza-Bastida A, Zamilpa A, Ojeda-Ramirez D, de Gives PM, Olivares-Perez J, Rivero-Perez N. 2019. Antibacterial activity of compounds isolated from Caesalpinia coriaria (Jacq) Willd against important bacteria in public health. Microb Pathog. 136:103660.
- [OECD] Organization for Economic Cooperation and Development Guideline for Testing of Chemicals. 2008. Acute oral toxicity-up-and-down-procedure (UDP). Guideline no. 425. Rome: OECD.
- Polhill R, Wiens D. 1998. Mistletoes of Africa. Kew: The Royal Botanic Gardens.
- Porwal M, Khan NA, Maheshwari KK. 2017. Evaluation of acute and subacute oral toxicity induced by ethanolic extract of Marsdenia tenacissima leaves in experimental rats. Sci Pharm. 85(3):29.
- Pozdnyakov DI, Adzhiahmetova SL, Chervonnaya NM, Voronkov AV, Oganesyan ET. 2021. Some aspects of the adaptogenic potential of European mistletoe (Viscum album L.) extracts under variable physical performance. J Med Plants. 20(77):60–78.
- Sati P, Dhyani P, Bhatt ID, Pandey A. 2019. Ginkgo biloba flavonoid glycosides in antimicrobial perspective with reference to extraction method. J Tradit Complement Med. 9(1):15–23.
- Smith BE, Orders T, Slate J, Bauldry S, Emrani J, Idassi J, Ahmed M, Cecile J, Bailey CS, Nguyen NA, et al. 2020. Proteomics analysis reveal that Moringa oleifera kills Escherichia coli by altering multiple biological processes. S Afr J Bot. 129:366–378.
- Tian X, Peng X, Lin J, Zhang Y, Zhan L, Yin J, Zhang R, Zhao G. 2021. Isorhamnetin ameliorates Aspergillus fumigatus keratitis by reducing fungal load, inhibiting pattern-recognition receptors and inflammatory cytokines. Invest Ophthalmol Vis Sci. 62(3):38.
- [UN] United Nations. 2011. Globally harmonized system of classification and labeling of chemicals (GHS). New York and Geneva: United Nations (UN).
- Yilmaz MT. 2012. Minimum inhibitory and minimum bactericidal concentrations of boron compounds against several bacterial strains. Turk J Med Sci. 42:1423–1429.
- Yirgu A, Tesfaye G. 2018. Observation on parasitic plants in southwestern and central part of Ethiopia. In: Mulatu Y, Yirgu A, Anjulo A, Adela Y, editors. Proceedings of the 2nd Annual Research Outputs Dissemination Workshop; Jan 26–27. Bin International Hotel, Bishoftu, Ethiopia.