Abstract
Context
Xiaojianzhong decoction (XJZD), classically prescribed in Chinese medicine, has protective and healing effects on gastric mucosal injury. However, the exact mechanism behind this effect remains unclear.
Objective
To investigate the effect of XJZD on gastric mucosal injury and explore its underlying mechanisms.
Materials and methods
C57BL/6 mice were randomized into six groups (n = 10): the control group receiving sterile water, the model (aspirin 300 mg/kg), the XJZD high-dose (12 g/kg), XJZD medium-dose (6 g/kg), XJZD low-dose (3 g/kg) and omeprazole (20 mg/kg) groups, by gavage daily for 14 days. The area of gastric mucosal injury, mucosal injury index and degree of histopathological damage were analysed. Gastric mucosal epithelial cell apoptosis was detected. Epithelial cell autophagy was observed. The expression levels of tight junction proteins and proteins related to apoptosis, autophagy and the pentose phosphate pathway were analysed.
Results
The results showed that after treatment with XJZD (12, 6 and 3 g/kg), the mucosal injury area was reduced (83.4%, 22.6% and 11.3%), the expression level of ZO-1 and occludin was up-regulated, the apoptosis rate of epithelial cells was reduced (40.8%, 25.4% and 8.7%), the expression of autophagy-related proteins LC3 and Beclin1 was decreased and the expression of p62 was increased, the PI3K/AKT/mTOR/ULK1(ser757) signalling pathway was activated, and the AMPK/ULK1(ser317) signalling pathway was inhibited. In addition, XJZD can antagonize the imbalance of redox homeostasis caused by aspirin and protect the gastric mucosa.
Discussion and conclusions
XJZD protects against aspirin-induced gastric mucosal injury, implying it to be a potential therapeutic agent.
Introduction
In recent years, with the widespread clinical use of non-steroidal anti-inflammatory drugs (NSAIDs), there has been an increase in the incidence of gastric ulcers caused by them. The most intuitive manifestation of gastric ulcers is gastric mucosal injury. A common gastrointestinal disease, gastric mucosal injury is the first link in a variety of gastric diseases (Kwon et al. Citation2019). NSAIDs commonly used in clinical practice include aspirin, paracetamol, diclofenac and ibuprofen (Eccleston et al. Citation2017), among which aspirin is used for the prevention of cardiovascular and cerebrovascular diseases because of its anti-platelet aggregation and anti-thrombotic properties (Fiala and Pasic Citation2020). However, even low doses of aspirin can lead to the occurrence of gastric mucosal injury with long-term use (Tseng et al. Citation2021), causing secondary damage to the patient’s body and seriously affecting their quality of life. At present, the treatment of aspirin-induced gastric mucosal injury mostly uses proton pump inhibitors, H2 receptor antagonists and gastric mucosal protectors. However, there are disadvantages to these treatments, such as unsustainable efficacy and numerous side effects (Oncel et al. Citation2021), and these disadvantages greatly limit the application of aspirin in clinics. Therefore, it is important to find a drug that can effectively protect against aspirin-induced gastric mucosal injury.
Gastric mucosal injury is closely associated with excessive apoptosis of epithelial cells (Ren et al. Citation2020). In a healthy state, the body eliminates excess, redundant, aging and unhealthy cells through apoptosis to maintain the balance and homeostasis of its internal environment (Park et al. Citation2021). In normal gastric mucosa, apoptotic cells form an ‘apoptotic cell band’ on the mucosal surface to maintain mucosal integrity and resist external stimulation. In the injury stage, apoptotic cells increase dramatically, the integrity of the gastric mucosa is destroyed, and the gastric mucosal injury is further aggravated (Li et al. Citation2021). Salah Khalil (Citation2015) found that aspirin can increase the apoptosis of gastric mucosal epithelial cells and induce gastric mucosal injury, while the inhibition of apoptosis can reduce gastric mucosal injury. As an upstream signal of apoptosis, autophagy can regulate cell apoptosis, and cells are stimulated by conditions such as starvation, hypoxia, endoplasmic reticulum stress and oxidative damage to induce autophagy (Huang et al. Citation2020). Moderate autophagy helps to maintain cellular homeostasis, thereby ensuring cell survival. However, the continuous activation of autophagy can lead to the degradation of a large number of intracellular proteins and the engulfment of anti-apoptosis-related proteins, leaving the cells unable to maintain their normal basic structures and eventually leading to cell necrosis or apoptosis (Yang et al. Citation2016; Gao et al. Citation2021). Therefore, reducing apoptosis and regulating autophagy are important strategies for alleviating gastric mucosal injury.
Xiaojianzhong decoction (XJZD), a famous Chinese herbal formula, is commonly used with remarkable clinical efficacy in the treatment of gastritis, gastric ulcer, ulcerative colitis, irritable bowel syndrome and other digestive diseases. The formula for XJZD consists of six herbs: Cinnamomum cassia Presl. (Lauraceae), Paeonia lactiflora Pall. (Paeoniaceae), Zingiber officinale Rosc. (Zingiberaceae), Glycyrrhiza uralensis Fisch. (Leguminosae), Ziziphus jujuba Mill. (Rhamnaceae) and Saccharum granorum (Maltose). Modern pharmacological studies have confirmed that ginger can effectively promote the synthesis of gastric mucosa, attenuate the digestive effect of pepsin, and exert an effective protective role on gastric mucosa (Haniadka et al. Citation2013). Cinnamaldehyde, the main component of Cinnamomum cassia, has antiulcer properties and can repair mucosal damage (Dorri et al. Citation2018). Paeoniflorin, the main component of Paeonia lactiflora, has anti-inflammatory and antioxidant activities and can reduce cell damage (Zhang et al. Citation2013). Licorice flavonoids, the main component of Glycyrrhiza uralensis, play a role in the treatment of gastric ulcers by regulating inflammatory mediators and amino acid metabolism (Yang et al. Citation2017). These findings suggest that XJZD may have the effect of preventing and treating gastric mucosal injury.
Therefore, in this study, aspirin was used to induce gastric mucosal injury, and the antagonistic effect of XJZD on aspirin-induced gastric mucosal injury in mice was evaluated. The anti-injury effects and mechanisms of XJZD were assessed based on ulcer index, histological examination, apoptosis level, autophagy level and related signalling pathways.
Materials and methods
Drugs and reagents
Aspirin was purchased from Beijing Solarbio Technology Co. Ltd. (Beijing, China, Lot No. 922J011). All herbs were purchased from Shaanxi Xingshengde Pharmaceutical Co. Ltd. (Xi’an, China). Omeprazole for injection was purchased from Zhejiang Better Pharmaceutical Co. Ltd. (Shaoxing, China, Lot No. H20084263). Primary antibodies: LC3 (no. 14600-1-AP), PI3k (no. 60225-1-Ig), AKT (no. 10176-2-AP), p-AKT (no. 66444-1-Ig), mTOR (no. 66888-1-Ig), AMPK (no. 10929-2-AP), G6PD (no. 66373-1-Ig) and β-actin (no. BM0627) were purchased from Wuhan Sanying Biotechnology Co., Ltd. (Wuhan, China). P62 (no. AF5384), p-AMPK (no. AF3423), p-ULK1(ser555) (no. AF7148) and p-ULK1(ser317) (no. AF2301) were purchased from Affinity (Changzhou, China). p-PI3K (no. Ab182651) was purchased from Abcam (Hong Kong, China). p-mTOR (bs-3495R) and ULK1 (no. bs-3495R) were purchased from Bioss (Beijing, China). p-ULK1(ser757) was purchased from CST (Boston, MA). Secondary antibodies were purchased from Boster (Wuhan, China), while the Coenzyme II NADP(H) Content Test Kit was purchased from Nanjing Jiancheng Bioengineering Institute (Nanjing, China).
Preparation and quality control of Xiaojianzhong decoction
The composition of XJZD is shown in . Shaanxi University of Traditional Chinese Medicine identified the herbs and prepared the XJZD. Except maltose, all ingredients were first soaked in tap water for 30 min and then decocted twice in water for 45 min each time. The decoction was then filtered, and the filtrate was combined. The Saccharum granorum was then added to the filtrate, and the solution was concentrated to the experimental dose.
Table 1. Contents of Xiaojianzhong decoction.
Ultra-high performance liquid chromatography (UPLC) fingerprint (Waters, Milford, MA) analysis was used to verify the main chemical composition of the XJZD extract. After weighing 9 g of Cinnamomum cassia, 9 g of Paeonia lactiflora, 6 g of Glycyrrhiza uralensis, 9 g of Zingiber officinale and 24 g of Ziziphus jujuba, 11.5 times the weight of water was added. The mixture was soaked for 30 min and decocted for 1.5 h, followed by filtering. Afterwards, eight times water was added to the filter residue, which was then decocted for 1 h. The filtrate was combined and extracted. Then, 30 g of Saccharum granorum was added to melt, and the raw drug was concentrated to the amount of 1.05 g/mL. After centrifugation, 1 mL of the supernatant was taken, and the volume was adjusted to 10 mL with methanol. The sample was separated on an Acuity-I-Class UPLC-BEH C18 chromatographic column (2.1 × 100 mm, 1.7 μm), with the mobile phase consisting of acetonitrile (B)–0.1% phosphoric acid water (A), with a gradient elution procedure as follows: 0–1 min, 5–10% B, 95–90% A; 6–12 min, 10–20% B, 90–80% A; 12–19 min, 20–30% B, 80–70% A; 19–24 min, 30–45% B, 70–55% A; 24–29 min, 45–55% B, 55–45% A; 29–32 min, 55–5% B, 45–95% A; 32–35 min, 5% B, 95% A. A volume flow rate of 0.2 mL/min, a column temperature of 30 °C, an injection volume of 2 μL, and a detection wavelength set to 210 nm.
Experimental animals and groups
Sixty male, SPF-grade, 5-week-old C57BL/6 mice weighing 20 ± 3 g (Certificate no. SCXK (chuan) 2020-034) were purchased from Chengdu Yaokang Biotechnology Co., Ltd. (Chengdu, China). The mice were housed in cages at a temperature of 24 ± 2 °C and a humidity of about 50 ± 5%, with a 12 h light/dark cycle, and provided with adequate food and water. The mice were adaptively fed for 1 week under specific pathogen-free conditions and maintained in good health. The mice received humane care in accordance with the Shaanxi University of Chinese Medicine Animal Care Committee guidelines (no. SUCMDL20211010023). After seven days of acclimatization, 60 mice were randomly divided into six groups, with 10 mice in each group: control group (control, sterile water), model group (model, aspirin 300 mg/kg), XJZD high-dose group (XJZDH), XJZD medium-dose group (XJZDM), XJZD low-dose group (XJZDL) and omeprazole group (OME). The control group was given an equal amount of sterile water, and the model group was given 300 mg/kg of aspirin by gavage for 14 consecutive days. The XJZDH, XJZDM and XJZDL groups were given 300 mg/kg of aspirin in the morning and 12, 6 and 3 g/kg XJZD in the afternoon, respectively, by gavage daily for 14 days. The omeprazole group was given 300 mg/kg of aspirin in the morning and 20 mg/kg of omeprazole sodium in the afternoon by gavage daily for 14 days. The schematic diagram is presented in . Body weight was recorded every two days.
Figure 1. XJZD attenuates aspirin-induced gastric mucosal injury. (A) Schematic diagram of administration in C57BL/6 mice. (B) A schematic diagram of the change of body weight in mice, and the body weight was measured every two days. (C) Tissue image of gastric mucosa in mice. (D) HE staining of mouse stomach tissue, magnification ×100 and ×200. (E) Gastric mucosal injury index in mice. (F) Inhibition rate of gastric mucosal injury in mice. (G) Histogram of gastric mucosal injury area in mice (n = 6). These data are means ± SD, ###p < 0.001 when compared with the control group. *p < 0.05, **p < 0.01 and ***p < 0.001 when compared with the model group.
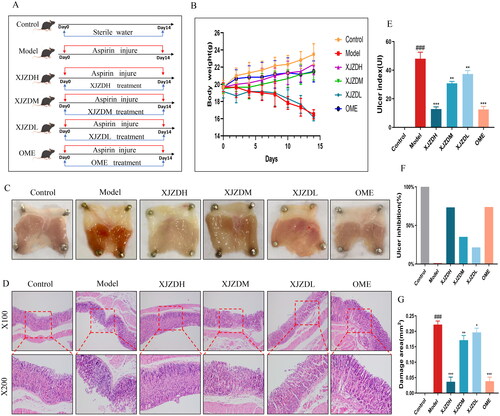
Assessment of the degree of gastric mucosal injury
After the gastric body was taken out, the stomach was cut along the greater curvature, rinsed with ice saline, and spread out on filter paper. The size of the ulcer was measured with a vernier calliper, and the ulcer index was calculated according to the Guth standard (Guth et al. Citation1979), which is expressed in mm: no lesion (0 points); one point for lesions ≤1 mm (including erosion points); two points for 1 mm < lesions ≤2 mm; three points for 2 mm < lesions ≤3 mm; four points for 3 mm < lesions ≤4 mm; and five points for >4 mm. For lesions >2 mm in width, the score was multiplied by 2, and finally the sum of the scores of all gastric lesions was used as the index of gastric mucosal injury in the mice. The injury inhibition rate was calculated as follows: injury inhibition rate = (injury index of the model group – injury index of the drug group)/injury index of the model group × 100%. Subsequently, the area of the gastric mucosal injury was analysed using ImageJ v1.44 software (Bethesda, MD).
Histopathological evaluation of the stomach
Gastric tissues (0.5 cm × 0.5 cm) were extracted, fixed in 4% paraformaldehyde, dehydrated, embedded in paraffin, routinely sectioned, stained with haematoxylin–eosin (H&E) and sealed with neutral gum. The morphological changes in gastric tissues were observed under a light microscope.
TUNEL staining
TUNEL staining was performed according to the manufacturer’s instructions. The gastric tissues were dehydrated, embedded, sectioned and deparaffinized before being rinsed with deionized water. Next, 2 mg/mL of proteinase K solution was diluted with deionized water at a ratio of 1:100, added dropwise to the samples, and incubated at room temperature for 20 min. Afterwards, 5× equilibration buffer was diluted with deionized water at a ratio of 1:5, added dropwise to the sample, and incubated at room temperature for 15 min. TdT incubation buffer was added, incubated at 37 °C for 60 min and washed three times with PBS for 5 min each. DAPI was added dropwise and incubated in the dark for 5 min. The specimen was stained for nuclear staining, the slices were sealed using a mounting solution containing an anti-fluorescence quencher, and the images were collected using a fluorescence microscope.
Observation of autophagosomes by transmission electron microscopy
Gastric mucosal tissue blocks measuring 1 mm × 1 mm were fixed in 2.5% glutaraldehyde for 3–4 h. Cells were then collected by being scraped, centrifuged at 1000 rpm to be precipitated, incubated with fresh 2.5% glutaraldehyde, and placed in a 4 °C refrigerator. After sufficient fixation, the tissues were rinsed three times with 0.1 M of phosphate buffer (pH 7.4) for 15 min each time. The tissues were then fixed with 1% osmic acid–0.1 M phosphate buffer (pH 7.4) at room temperature (20 °C) for 2 h. Afterward, the tissues were rinsed three times with 0.1 M phosphate buffer (pH 7.4) for 15 min each. The tissues were then dehydrated through an alcohol gradient, infiltrated, embedded, sectioned, double-stained with uranium and lead, and dried overnight at room temperature. Observations were made using a transmission electron microscope (Hitachi, Chiyoda, Japan).
Immunohistochemistry
The paraffin sections were dewaxed and hydrated, and antigen retrieval was performed with 0.01 M of citrate buffer (pH 6.0) for 15 min. Three percent hydrogen peroxide was added dropwise to the tissue to block endogenous peroxidase and incubated at room temperature for 15 min. The sections were rinsed three times with PBS for 3 min each and then blocked with serum for 30 min. The sections were incubated with primary antibody: occludin (1:100) and caspase-3 (1:100) overnight at 4 °C. An enzyme-labelled secondary antibody was added and incubated at 37 °C for 30 min. DAB chromogenic solution was added dropwise. The tissues were then counterstained with Mayer’s haematoxylin for 2 min, washed with PBS to make them blue again, dehydrated in a conventional alcohol gradient, and mounted. The images were collected using a microscope (Olympus BX53, Shinjuku, Japan).
Immunofluorescence
The paraffin sections were dewaxed, hydrated and placed in a beaker. Then, 0.01 M of citrate buffer was added for antigen retrieval. Goat serum was added dropwise and blocked at room temperature for 30 min. Excess liquid was shaken off, and the primary antibody was added and incubated in a humidified box at 4 °C for 15 h. The antibody dilution ratios of the proteins, in order, were as follows: zonula occludens-1 (ZO-1) (1:100), LC3 (1:100), Beclin1 (1:100), ULK1 (1:100) and G6PD (1:100). After the sections were rinsed three times with PBST, a fluorescent secondary antibody was added dropwise and incubated in a humidified box at 37 °C for 1 h. The sections were then rinsed four times with PBST for 3 min each time. DAPI was added and incubated in the dark for 5 min to stain the nuclei of the tissues. The slices were mounted with a mounting solution containing an anti-fluorescence quencher. The images were observed and collected under a fluorescence microscope.
Biochemical detection
The protein samples and standards were diluted according to the manufacturer’s instructions and added into the 96-well plates. Solution A and solution B in the BCA kit were mixed at a ratio of 50:1, added to the 96-well plates, and incubated at 37 °C for 30 min in the dark. After that, the OD values were detected using a microplate reader and the sample protein concentrations were calculated.
Western blot (WB) analysis
The gastric tissue blocks were placed in EP tubes, homogenized with 200 μL of detergent lysis buffer, placed on ice for lysis for 30 min, and centrifuged at 12,000 rpm for 5 min at 4 °C. The supernatant was taken for protein concentration determination. The extracted protein supernatant and a 5× protein loading buffer were placed in a boiling water bath for 10 min for protein denaturation. The electrophoresis gel was prepared and fixed onto the electrophoresis tank. The proteins were separated by electrophoresis and then electrotransferred to PVDF membranes. The PVDF membranes were soaked with TBST (blocking solution) containing 5% skimmed milk powder and blocked in a shaker at room temperature for 2 h. The phosphorylated proteins were blocked with 1% BSA. The corresponding primary antibodies: P62 (1:1000), PI3k (1:1000), p-PI3k (1:1000), p-AKT (1:1000), p-mTOR (1:1000), p-AMPK (1:2000), ULK1 (1:1000), p-ULK1(ser555) (1:1000), p-ULK1(ser757) (1:1000), p-ULK1(ser317) (1:1000), mTOR (1:2000), AMPK (1:1000), LC3 (1:4000), AKT (1:4000), G6PD (1:4000) and β-actin (1:1000) were diluted with the blocking solution and incubated with the PVDF membranes overnight at 4 °C. The corresponding HRP-labelled secondary antibodies were diluted with TBST (1:10,000) and incubated with the PVDF membranes in a shaker at room temperature for 2 h. The PVDF membranes were fully washed five times with TBST to wash off the excess secondary antibodies. The colour was developed and the grey values of the film were analysed using Bandscan software (US).
Quantitative reverse transcription-polymerase chain reaction (qRT-PCR) analysis
The qRT-PCR analysis was used to detect the mRNA expression of PI3K, AKT, mTOR and ULK1 in gastric tissues. Total RNA was extracted using Trizol reagent (Ambion, China) and reverse transcribed into cDNA according to the manufacturer’s experimental procedure. After genomic DNA was removed and cDNA was prepared, the real-time quantitative PCR was performed. The number of replicate wells was 3 and the cDNA dilution was five times. β-actin was used as the endogenous reference. The 2–ΔΔCt method was used to calculate the experimental data. The primers used in this study are listed in .
Table 2. Primers used for qPCR assays.
Statistical analysis
The experimental data were expressed as mean ± standard deviation (SD), and statistical analysis was performed using GraphPad Prism version 7 (GraphPad Software Inc., San Diego, CA). One‐way analysis of variance (ANOVA) and Tukey’s post hoc test were used for the statistical analysis of more than two groups, while Student’s t‐test was used for the statistical analysis of two groups. For all analyses, p < 0.05 was considered statistically significant.
Results
Ultra-high performance liquid chromatography analysis of Xiaojianzhong decoction
The HPLC results are shown in . By the comparison with the chromatogram of the mixed reference substance (), a total of five chemical components were identified in XJZD, including albiflorin (no. 1), paeoniflorin (no. 2), liquiritin (no. 3), cinnamaldehyde (no. 4) and 6-gingerol (no. 5) ().
Xiaojianzhong decoction attenuates aspirin-induced gastric mucosal injury
To assess the protective effect of XJZD on aspirin-induced gastric mucosal injury in mice, we evaluated the degree of injury in six aspects: body weight, degree of gastric mucosal tissue damage, H&E staining of gastric tissues, injury index, injury inhibition rate and injury area. The results showed that after aspirin administration, the body weight of the mice decreased significantly (); obvious bleeding spots were visible to the naked eye on the surface of the gastric mucosa (); the glands in the upper layer of the gastric mucosa were destroyed; a large number of red blood cells were in the mucosal layer; there was severe mucosal cell abscission, and the injury index and injury area increased (). After treatment with XJZD, the above changes improved significantly: the body weight increased, the gastric mucosal surface bleeding was reduced, the mucosal pathological damage was alleviated, the injury index and area decreased, and the injury inhibition rate increased compared with that of the model group. The injury degree became less severe with the increase in the dose of XJZD (). These data suggest that XJZD attenuates aspirin-induced gastric mucosal injury.
Xiaojianzhong decoction alleviates the disruption of gastric mucosal barrier integrity by aspirin
The tight junction proteins ZO-1 and occludin, located between epithelial cells, play a key role in maintaining the integrity of the gastric mucosal barrier (Qiu et al. Citation2022). To evaluate the effect of XJZD on the gastric mucosal barrier, we examined the expression of ZO-1 and occludin using immunofluorescence and immunohistochemistry, respectively. The results showed that on the gastric mucosal surfaces of normal mice, ZO-1 and occludin were located between epithelial cells, arranged neatly and distributed continuously and uniformly (). After aspirin administration, the expression of ZO-1 and occludin in the epithelium of the gastric mucosal superficial layer was attenuated and their distribution was scattered, accompanied by mucosal damage and structural tissue defects. After XJZD treatment, the expression of ZO-1 and occludin was enhanced, a continuous distribution was restored, the mucosal damage was reduced, and the integrity of the gastric mucosal barrier was protected ().
Figure 3. XJZD alleviates the disruption of gastric mucosal barrier integrity by aspirin. (A) The distribution and expression of ZO-1 were detected by immunofluorescence assay, Magnification ×400, scale bar: 20 µm. (B) The distribution and expression of occludin were detected by immunohistochemical analysis, magnification ×100 and ×400. (C) Using ImageJ software to analyse the fluorescence intensity of ZO-1. (D) Using ImageJ software to analyse the average optical density of occludin (n = 6). These data are means ± SD, ###p < 0.001 when compared with the control group. **p < 0.01 and ***p < 0.001 when compared with the model group.
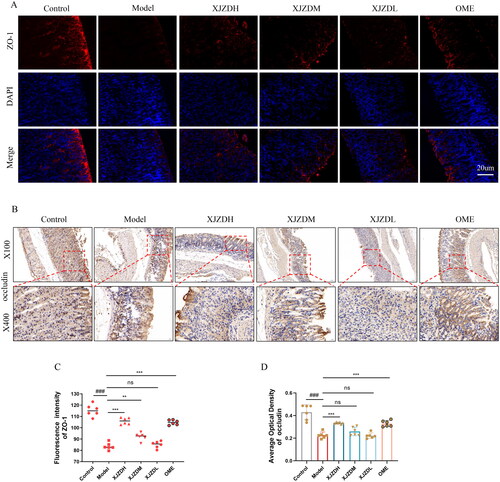
Xiaojianzhong decoction inhibits aspirin-induced apoptosis of gastric mucosal epithelial cells
Excessive apoptosis of gastric mucosal epithelial cells is an important mechanism of gastric mucosal injury caused by NSAIDs (Cheng et al. Citation2017). Therefore, we examined apoptosis-related indicators. The TUNEL staining results showed that the apoptosis of gastric mucosal epithelial cells in the mice increased after aspirin administration (). Immunohistochemistry and WB results indicated that the expression of pro-apoptotic proteins caspase-3 and Bax increased, and the expression of anti-apoptotic protein Bcl-2 decreased in gastric mucosal tissues of mice after aspirin administration, decreased ratio of Bcl-2/Bax (), which is consistent with previously reported results (Kusuhara et al. Citation1998). The levels of cleaved caspase-3 and the ratio of cleaved caspase-3/pro-caspase-3 were also elevated (), indicating increased apoptosis in the gastric mucosal epithelium. The administration of XJZD reduced the apoptosis of epithelial cells, reversed the aspirin-induced changes in caspase-3, Bax and Bcl-2, increased ratio of Bcl-2/Bax, and decreased the levels of cleaved caspase-3 and cleaved caspase-3/pro-caspase-3 (). These results confirm that XJZD can reduce aspirin-induced apoptosis of gastric mucosal epithelial cells and protect against gastric mucosal injury.
Figure 4. XJZD inhibits aspirin-induced apoptosis of gastric mucosal epithelial cells. (A) Fluorescence micrographs of TUNEL-stained gastric mucosal epithelial cells indicating apoptotic cells (green). Magnification ×400, scale bar: 20 µm. (B) Using ImageJ software to analyse the TUNEL positive cell rate. (C) The distribution and expression of caspase-3 were detected by immunohistochemical analysis. Magnification ×100 and ×400 (n = 6). (D) Using ImageJ software to analyse the average optical density of caspase-3. (E) Western blotting bands of apoptosis-related proteins. (F–K) Grey intensity analysis of Bax/β‐actin, Bcl-2/β‐actin, Bcl-2/Bax ratio, pro-caspase-3/β‐actin, cleaved-caspase-3/β‐actin and cleaved-caspase-3/pro-caspase-3 (n = 3). These data are means ± SD, ##p < 0.01 and ###p < 0.001 when compared with the control group. *p < 0.05, **p < 0.01 and ***p < 0.001 when compared with the model group. ns: no significant difference.
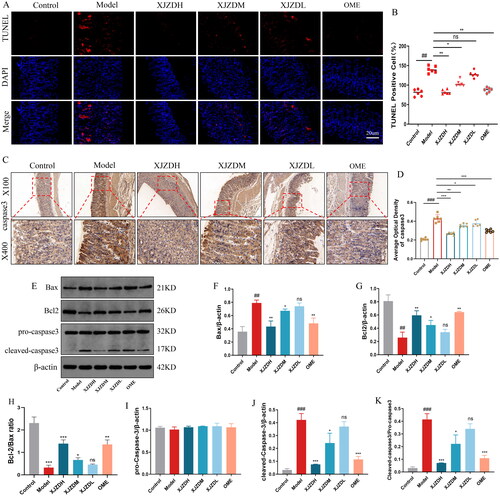
Xiaojianzhong decoction inhibits autophagy in gastric mucosal epithelial cells
Autophagy is a double-edged sword that plays an important role in maintaining the homeostasis of the intracellular environment. It can not only remove damaged or unnecessary proteins in cells and promote cell survival but also induce apoptosis due to excessive autophagy, leading to cell dysfunction and death (Kim et al. Citation2020). To evaluate the effect of XJZD on the autophagy of gastric mucosal epithelial cells, we observed the changes in the ultrastructure of gastric mucosal epithelial cells using transmission electron microscopy. The results showed that after aspirin (300 mg/kg) administration, the swelling of mitochondria in the gastric tissues of mice was obvious, the endoplasmic reticulum was dilated, a large number of lysosomes and autophagosomes had formed, and a typical double-layer membrane structure appeared (). These changes were ameliorated by XJZD treatment, as evidenced by reduced mitochondrial swelling and fewer lysosomes and autophagosomes (). To further investigate the effect of XJZD on the level of autophagy, we detected the expression levels of autophagy related proteins using immunofluorescence and WB, respectively (Condello et al. Citation2019). The results showed that after aspirin administration, the fluorescence intensity of LC3 and Beclin1 in the gastric mucosal tissues of the mice was enhanced, the LC3-II/LC3-I ratio significantly increased, and the p62 protein expression was significantly reduced. After treatment with XJZD, the above changes were reversed in a dose-dependent manner (). Our results suggest that XJZD can inhibit the overactivation of autophagy and reduce the level of autophagy in gastric tissues.
Figure 5. XJZD inhibits autophagy in gastric mucosal epithelial cells. (A) The number of autophagosomes in gastric mucosal epithelial cells was shown by transmission electron microscopy (indicated by red arrows), Magnification ×12,000. (B) The expression of LC-3 was detected by immunofluorescence assay, magnification ×400; scale bar: 20 µm (n = 6). (C) Using ImageJ software to analyse the fluorescence intensity of LC-3. (D) The expression of Beclin1 was detected by immunofluorescence assay, magnification ×400; scale bar: 20 µm (n = 6). (E) Using ImageJ software to analyse the fluorescence intensity of Beclin1. (F) Western blotting bands of autophagy-related proteins. (G,H) Grey intensity analysis of P62/β‐actin and LC-3II/LC-3I ratio (n = 3). These data are means ± SD, ###p < 0.001 when compared with the control group. *p < 0.05, **p < 0.01 and ***p < 0.001 when compared with the model group. ns: no significant difference.
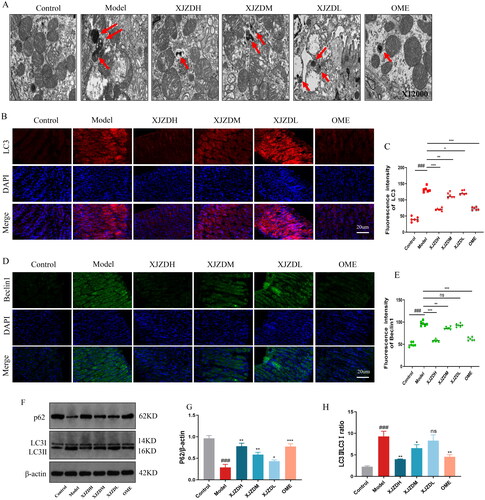
Xiaojianzhong decoction activates the PI3K/AKT/mTOR/ULK1(ser757) pathway
Studies have shown that the PI3K/AKT/mTOR/ULK1(ser757) signalling pathway is the main pathway involved in autophagy (Teng et al. Citation2019). Therefore, we detected it using PCR and WB. The results showed that the protein expression of p-PI3K, p-Akt, p-mTOR and p-ULK1(ser757) and the mRNA levels of PI3K, Akt and mTOR decreased in the gastric mucosal tissues of mice after aspirin administration. These changes were reversed after high-dose administration of XJZD (). Thus, our study suggests that XJZD can activate the PI3K/AKT/mTOR/ULK1(ser757) signalling pathway.
Figure 6. The expression of the PI3K/AKT/mTOR/ULK1(ser757) signalling pathway proteins. (A–C) The mRNA levels of PI3K, AKT and mTOR in stomach tissue. (D–G) Representative Western blot bands of relevant proteins. (H–K) Protein levels of p-PI3K/PI3K, p-AKT/AKT, p-mTOR/mTOR and p-ULK1(ser757)/ULK1 (n = 3). These data are means ± SD, ##p < 0.01 and ###p < 0.001 when compared with the control group. *p < 0.05, **p < 0.01 and ***p < 0.001 when compared with the model group.
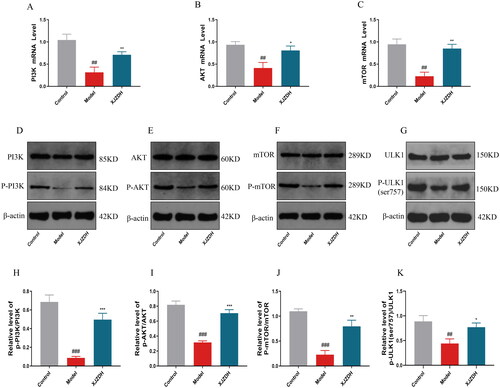
Xiaojianzhong decoction inhibits the AMPK/ULK1(ser317) pathway
AMPK is an energy regulator of eukaryotic cells that activates autophagy by directly phosphorylating the ser317 and ser555 sites of autophagy initiation factor ULK1 (Holczer et al. Citation2020). Therefore, we detected the levels of AMPK and ULK1. The results showed that the fluorescence intensity of ULK1 was enhanced, and the p-AMPK, p-ULK1(ser317), and mRNA levels of ULK1 were elevated in the gastric mucosal tissues of mice after aspirin administration. After high-dose treatment with XJZD, the fluorescence intensity and mRNA expression levels of ULK1 decreased, as did p-AMPK, p-ULK1(ser317) and ULK1 (). No obvious differences in p-ULK1(ser555) were found in any groups (). Our results suggest that XJZD can inhibit the AMPK/ULK1(ser317) signalling pathway.
Figure 7. XJZD inhibits the AMPK/ULK1(ser317) pathway. (A) The expression of ULK1 was detected by immunofluorescence assay, magnification ×400, scale bar: 20 µm (n = 6). (B) Using ImageJ software to analyse the fluorescence intensity of ULK1. (C) Representative Western blot bands of AMPK and p-AMPK. (D) Protein levels of p-AMPK/AMPK. (E) The mRNA levels of ULK1. (F) Representative Western blot bands of ULK1, p-ULK1(ser555) and p-ULK1(ser317). (G, H) Protein levels of p-ULK1(ser555)/ULK1 and p-ULK1(ser317)/ULK1 (n = 3). These data are means ± SD, ##p < 0.01 and ###p < 0.001 when compared with the control group. *p < 0.05, **p < 0.01 and ***p < 0.001 when compared with the model group. ns: no significant difference.
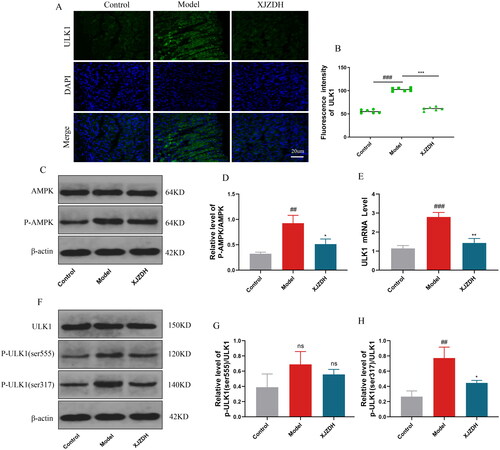
Xiaojianzhong decoction activates the pentose phosphate pathway to maintain redox homeostasis and reduce damage
Glucose-6-phoshate dehydrogenase (G6PD) is the main regulatory enzyme in the pentose phosphate pathway, which maintains cellular redox homeostasis and reduces oxidative damage by producing nicotinamide adenine dinucleotide phosphate (NADPH) (Stanton Citation2012). To further investigate whether XJZD affects the pentose phosphate pathway, we analysed the expression level of G6PD using immunofluorescence. The results showed that G6PD expression at the site of gastric mucosal injury was attenuated after aspirin administration. The opposite was observed after XJZD treatment (). The WB results further confirmed our conclusion (). Meanwhile, we measured the expression of NADPH in gastric mucosal tissues using biochemical detection. The results showed that NADPH expression decreased after aspirin administration and increased after XJZD treatment (). These results suggest that XJZD can reduce aspirin-induced gastric mucosal injury by maintaining redox homeostasis.
Figure 8. XJZD activates the pentose phosphate pathway to maintain redox homeostasis and reduce damage. (A) The expression of G6PD was detected by immunofluorescence assay, magnification ×400; scale bar: 20 µm (n = 6). (B) Using ImageJ software to analyse the fluorescence intensity of G6PD. (C) Representative Western blot bands of G6PD. (D) Protein levels of G6PD. (E) The levels of NADPH in stomach tissue (n = 3). These data are means ± SD, ##p < 0.01 and ###p < 0.001 when compared with the control group. **p < 0.01 and ***p < 0.001 when compared with the model group. ns: no significant difference.
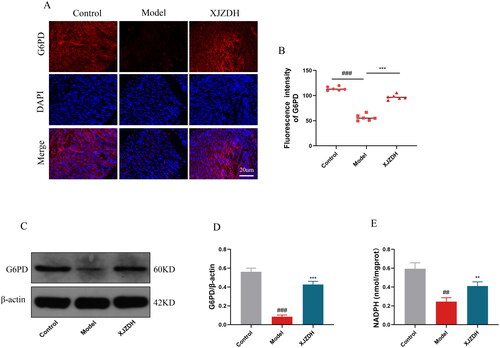
Discussion
This study confirmed the protective effects of XJZD, a traditional Chinese medicine, on aspirin-induced gastric mucosal injury in mice, as demonstrated by the inhibition of body weight loss, alleviation of pathological damage, reduction of injury index and injury area, protection of gastric mucosal barrier integrity, and inhibition of gastric mucosal epithelial cell apoptosis and autophagy, with its potential mechanisms of action related to the activation of the PI3K/AKT/mTOR/ULK1(ser757) pathway and inhibition of the AMPK/ULK1(ser317) pathway. In addition, we found that XJZD can activate the pentose phosphate pathway to maintain redox homeostasis and reduce gastric mucosal injury. In the present study, the gastroprotective effect of XJZD was comparable to that of a widely used standard drug. These findings suggest that XJZD treatment can protect against aspirin-induced gastric mucosal injury.
The tight junction protein located in the epithelium of the gastric mucosal superficial layer is the key to maintaining the gastric mucosal barrier, which acts as a ‘fence’ in the body to protect the submucosa from harmful substances (Oshima and Miwa Citation2016). ZO-1 is the cytoskeletal protein of tight junction protein (Müller et al. Citation2005), while occludin is its transmembrane protein (Osanai et al. Citation2007). Tight junction proteins are essential for maintaining mucosal homeostasis and ensuring cellular integrity (Bendriem et al. Citation2019; Kuo et al. Citation2021). Our study showed that XJZD treatment increased the expression intensity of ZO-1 and occludin, maintained the integrity of the gastric mucosal barrier, and reduced aspirin-induced gastric mucosal injury.
In the process of apoptosis, the anti-apoptotic protein Bcl-2 performs a ‘master switch’ role, inhibiting the activation of downstream caspase-3 and the onset of apoptosis (Grilo and Mantalaris Citation2019). The pro-apoptotic protein Bax, the central activator of apoptosis, is translocated to mitochondria after being stimulated by apoptotic signals; it triggers the caspase-related apoptotic cascade response and antagonizes the anti-apoptotic effect of Bcl-2 at the same time, promoting the occurrence of apoptosis (Edlich Citation2018). Caspase-3, the key effector protein in the apoptosis pathway (Lei et al. Citation2022), can cause a series of waterfall responses after activation, making apoptosis into an irreversible condition (McIlwain et al. Citation2015). Our study showed that after aspirin administration, the apoptosis of gastric mucosal epithelial cells increased, the pro-apoptotic proteins Bax and cleaved caspase 3 were up-regulated, and the anti-apoptotic protein Bcl-2 was down-regulated, which is consistent with the study by Gu et al. (Citation2005). The XJZD administration reduced the apoptosis of gastric mucosal epithelial cells, increased the expression level of anti-apoptotic protein Bcl-2, decreased the expression levels of pro-apoptotic proteins Bax and cleaved caspase-3, increased the ratio of Bcl-2/Bax, and decreased the ratio of cleaved caspase-3/pro-caspase-3. These results suggest that XJZD can inhibit aspirin-induced apoptosis of gastric mucosal epithelial cells.
Autophagy is a ‘double-edged sword’. It promotes cell survival and maintains the homeostasis of the intracellular environment through the removal and reuse of intracellular components. Conversely, continuous excessive autophagy exceeds the adaptive capacity of cells and contributes to cell death (Yu et al. Citation2013). In the basal state, autophagy exerts anti-apoptotic and cytoprotective effects to enable cells to function normally (Mizushima and Levine Citation2020). Under stress, autophagy rebuilds the structure and maintains cell survival by removing denatured proteins and necrotic substances in cells. Autophagic activation beyond a certain threshold may result in autophagic cell death directly or the execution of apoptotic cell death (Levine and Kroemer Citation2008; Wang Citation2015). Currently, the effect of aspirin on autophagy in the process of gastric mucosal injury remains controversial. Hernández et al. showed that aspirin (150 mg/kg) inhibited basal autophagy in gastric mucosal epithelial cells (Hernández et al. Citation2016). Interestingly, we found that aspirin (300 mg/kg) activated autophagy in these cells. The formation of autophagosomes in mouse gastric mucosal epithelial cells under an electron microscope increased; the expression of LC3 and Beclin1 proteins, which are positively associated with autophagy, increased; the expression of p62 protein, which is negatively related to autophagy, decreased; and the ratio of LC3-II/LC3-I, which reflects the level of autophagy, increased. These results all suggest that aspirin activated autophagy in gastric mucosal epithelial cells. But why are the levels of autophagy diametrically opposite when aspirin induced gastric mucosal injury in both studies? We speculate that the reason for this phenomenon may be related to the dose of aspirin administration. Aspirin activated autophagy in a dose-dependent manner in coronary artery endothelial cells (Chen et al. Citation2020). Similarly, 400 mg/kg of aspirin was found to induce autophagy by inhibiting mTOR signalling and activating AMPK-activated protein kinase in rectal cancer cell experiments (Din et al. Citation2012). Our results also suggest that XJZD reverses aspirin-induced changes in autophagy levels, reduces autophagosome formation, attenuates the immunofluorescence intensity of LC3 and Beclin1, decreases the LC3-II/LC3-I ratio, elevates p62 protein expression, and inhibits the overactivation of autophagy.
On one hand, the PI3K/AKT/mTOR signalling pathway has been shown to negatively regulate autophagy, and the activation of mTOR is a key link in the inhibition of autophagy (Zhu et al. Citation2018). On the other hand, ULK1 serves as a downstream signalling molecule for mTOR, where mTOR phosphorylates ULK1 at Ser757 and represses its protein kinase activity, thereby blocking the initiation of autophagy (Mohamed et al. Citation2021). Our study showed that XJZD activated the PI3K/AKT/mTOR/ ULK1(ser757) signalling pathway. In addition, the AMPK/ULK1 pathway is also critical for the regulation of autophagy. When AMPK is activated by phosphorylation, p-AMPK further phosphorylates ULK1 and positively regulates the Ser317 and Ser555 sites of ULK1, thereby inducing autophagy (Chen et al. Citation2019). Our study showed that XJZD inhibited the activation of the AMPK/ULK1(ser317) pathway and inhibited autophagy in gastric mucosal epithelial cells.
Accumulation of oxidative stress causes oxidization and damage to cells, which turns on the autophagic process (Scherz-Shouval and Elazar Citation2011). Long-term use of aspirin can elevate oxidative stress levels and aggravate gastric mucosal injury (Mahmoud and Abd El-Ghffar Citation2019). The pentose phosphate pathway can improve the ability of cells to resist oxidative stress and reduce damage (Cao et al. Citation2017). G6PD, as the first enzyme in the initial stage of the pentose phosphate pathway, can promote the entry of glucose into this pathway, generate NADPH, scavenge peroxides, maintain redox homeostasis, and prevent oxidative damage (Joshi et al. Citation2020). We examined the expression levels of G6PD and NADPH, and the results showed that XJZD increased both, confirming that XJZD can maintain redox homeostasis by activating the pentose phosphate pathway, thereby reducing gastric mucosal injury.
Conclusions
Our study showed that XJZD restored the expression level of gastric tight junction protein, maintained the integrity of the gastric mucosal barrier, and resisted aspirin-induced gastric mucosal injury by reducing gastric mucosal epithelial cell apoptosis and inhibiting autophagy. The mechanism may be related to the activation of the PI3K/AKT/mTOR/ULK1(ser757) pathway and the inhibition of the AMPK/ULK1(ser317) pathway. Meanwhile, XJZD can activate the pentose phosphate pathway to maintain redox homeostasis and protect the gastric mucosa (). These results suggest that XJZD is an effective drug for preventing and treating gastric mucosal injury. In terms of autophagy, this study provides new insights into the mechanism by which XJZD ameliorates aspirin-induced gastric mucosal injury.
Author contributions
Ting Chen: data curation, investigation, methodology, writing – original draft, project administration; Shengchuan Bao: conceptualization, methodology, validation, formal analysis, investigation; Juan Chen: formal analysis, methodology, data curation; Jiaxiang Zhang: validation, formal analysis; Hailiang Wei: formal analysis, investigation; Xin Hu: investigation, writing – review and editing; Yan Liang: methodology, writing – review and editing; Jingtao Li: methodology, writing – review and editing, data curation; Shuguang Yan: methodology, writing – review and editing, data curation.
Acknowledgements
LetPub (http://www.LetPub.com) was applied for linguistic assistance during the preparation of this manuscript.
Disclosure statement
The authors declare that they have no known competing financial interests or personal relationships that could have appeared to influence the work reported in this paper.
Data availability statement
The datasets used in the current study are available from the corresponding author on reasonable request.
Additional information
Funding
References
- Bendriem RM, Singh S, Aleem AA, Antonetti DA, Ross ME. 2019. Tight junction protein occludin regulates progenitor self-renewal and survival in developing cortex. eLife. 8:e49376. DOI: 10.7554/eLife.49376.
- Cao L, Zhang D, Chen J, Qin YY, Sheng R, Feng X, Chen Z, Ding Y, Li M, Qin ZH. 2017. G6PD plays a neuroprotective role in brain ischemia through promoting pentose phosphate pathway. Free Radic Biol Med. 112:433–444. DOI: 10.1016/j.freeradbiomed.2017.08.011.
- Chen J, Wang L, Liu WH, Shi J, Zhong Y, Liu SJ, Liu SM. 2020. Aspirin protects human coronary artery endothelial cells by inducing autophagy. Physiol Int. 107(2):294–305. DOI: 10.1556/2060.2020.00029.
- Chen X, Luo Y, Wang M, Sun L, Huang K, Li Y, Chen Y, Ding Y, Zhang X, Jiao L, et al. 2019. Wuhu decoction regulates dendritic cell autophagy in the treatment of respiratory syncytial virus (RSV)-induced mouse asthma by AMPK/ULK1 signaling pathway. Med Sci Monit. 25:5389–5400. DOI: 10.12659/MSM.917692.
- Cheng YT, Lu CC, Yen GC. 2017. Phytochemicals enhance antioxidant enzyme expression to protect against NSAID-induced oxidative damage of the gastrointestinal mucosa. Mol Nutr Food Res. 61:10–1002. DOI: 10.1002/mnfr.201600659.
- Condello M, Pellegrini E, Caraglia M, Meschini S. 2019. Targeting autophagy to overcome human diseases. Int J Mol Sci. 20:725. DOI: 10.3390/ijms20030725.
- Din FV, Valanciute A, Houde VP, Zibrova D, Green KA, Sakamoto K, Alessi DR, Dunlop MG. 2012. Aspirin inhibits mTOR signaling, activates AMP-activated protein kinase, and induces autophagy in colorectal cancer cells. Gastroenterology. 142(7):1504–1515.e3. DOI: 10.1053/j.gastro.2012.02.050.
- Dorri M, Hashemitabar S, Hosseinzadeh H. 2018. Cinnamon (Cinnamomum zeylanicum) as an antidote or a protective agent against natural or chemical toxicities: a review. Drug Chem Toxicol. 41(3):338–351. DOI: 10.1080/01480545.2017.1417995.
- Eccleston C, Cooper TE, Fisher E, Anderson B, Wilkinson NM. 2017. Non-steroidal anti-inflammatory drugs (NSAIDs) for chronic non-cancer pain in children and adolescents. Cochrane Database Syst Rev. 8(8):CD012537. DOI: 10.1002/14651858.CD012537.pub2.
- Edlich F. 2018. BCL-2 proteins and apoptosis: recent insights and unknowns. Biochem Biophys Res Commun. 500(1):26–34. DOI: 10.1016/j.bbrc.2017.06.190.
- Fiala C, Pasic MD. 2020. Aspirin: bitter pill or miracle drug? Clin Biochem. 85:1–4. DOI: 10.1016/j.clinbiochem.2020.07.003.
- Gao J, Chen X, Shan C, Wang Y, Li P, Shao K. 2021. Autophagy in cardiovascular diseases: role of noncoding RNAs. Mol Ther Nucleic Acids. 23:101–118. DOI: 10.1016/j.omtn.2020.10.039.
- Grilo AL, Mantalaris A. 2019. Apoptosis: a mammalian cell bioprocessing perspective. Biotechnol Adv. 37(3):459–475. DOI: 10.1016/j.biotechadv.2019.02.012.
- Gu Q, Wang JD, Xia HH, Lin MC, He H, Zou B, Tu SP, Yang Y, Liu XG, Lam SK, et al. 2005. Activation of the caspase-8/Bid and Bax pathways in aspirin-induced apoptosis in gastric cancer. Carcinogenesis. 26(3):541–546. DOI: 10.1093/carcin/bgh345.
- Guth PH, Aures D, Paulsen G. 1979. Topical aspirin plus HCl gastric lesions in the rat. Cytoprotective effect of prostaglandin, cimetidine, and probanthine. Gastroenterology. 76(1):88–93. DOI: 10.1016/S0016-5085(79)80133-X.
- Haniadka R, Saldanha E, Sunita V, Palatty PL, Fayad R, Baliga MS. 2013. A review of the gastroprotective effects of ginger (Zingiber officinale Roscoe). Food Funct. 4(6):845–855. DOI: 10.1039/c3fo30337c.
- Hernández C, Barrachina MD, Vallecillo-Hernández J, Álvarez Á, Ortiz-Masiá D, Cosín-Roger J, Esplugues JV, Calatayud S. 2016. Aspirin-induced gastrointestinal damage is associated with an inhibition of epithelial cell autophagy. J Gastroenterol. 51(7):691–701. DOI: 10.1007/s00535-015-1137-1.
- Holczer M, Hajdú B, Lőrincz T, Szarka A, Bánhegyi G, Kapuy O. 2020. Fine-tuning of AMPK-ULK1-mTORC1 regulatory triangle is crucial for autophagy oscillation. Sci Rep. 10(1):17803. DOI: 10.1038/s41598-020-75030-8.
- Huang CY, Deng JS, Huang WC, Jiang WP, Huang GJ. 2020. Attenuation of lipopolysaccharide-induced acute lung injury by hispolon in mice, through regulating the TLR4/PI3K/Akt/mTOR and Keap1/Nrf2/HO-1 pathways, and suppressing oxidative stress-mediated ER stress-induced apoptosis and autophagy. Nutrients. 12:1742. DOI: 10.3390/nu12061742.
- Joshi SR, Kitagawa A, Jacob C, Hashimoto R, Dhagia V, Ramesh A, Zheng C, Zhang H, Jordan A, Waddell I, et al. 2020. Hypoxic activation of glucose-6-phosphate dehydrogenase controls the expression of genes involved in the pathogenesis of pulmonary hypertension through the regulation of DNA methylation. Am J Physiol Lung Cell Mol Physiol. 318(4):L773–L786. DOI: 10.1152/ajplung.00001.2020.
- Kim KA, Kim D, Kim JH, Shin YJ, Kim ES, Akram M, Kim EH, Majid A, Baek SH, Bae ON. 2020. Autophagy-mediated occludin degradation contributes to blood–brain barrier disruption during ischemia in bEnd.3 brain endothelial cells and rat ischemic stroke models. Fluids Barriers CNS. 17(1):21. DOI: 10.1186/s12987-020-00182-8.
- Kuo WT, Zuo L, Odenwald MA, Madha S, Singh G, Gurniak CB, Abraham C, Turner JR. 2021. The tight junction protein ZO-1 is dispensable for barrier function but critical for effective mucosal repair. Gastroenterology. 161(6):1924–1939. DOI: 10.1053/j.gastro.2021.08.047.
- Kusuhara H, Matsuyuki H, Matsuura M, Imayoshi T, Okumoto T, Matsui H. 1998. Induction of apoptotic DNA fragmentation by nonsteroidal anti-inflammatory drugs in cultured rat gastric mucosal cells. Eur J Pharmacol. 360(2–3):273–280. DOI: 10.1016/s0014-2999(98)00679-7.
- Kwon DA, Kim YS, Baek SH, Kim SK, Kim HK, Jo SK, Jung U, Park HR, Lee HS. 2019. Protective effects of a standardized extract (HemoHIM) using indomethacin-and ethanol/HCl-induced gastric mucosal injury models. Pharm Biol. 57(1):543–549. DOI: 10.1080/13880209.2019.1651875.
- Lei Q, Huang X, Zheng L, Zheng F, Dong J, Chen F, Zeng W. 2022. Biosensors for caspase-3: from chemical methodologies to biomedical applications. Talanta. 240:123198. DOI: 10.1016/j.talanta.2021.123198.
- Levine B, Kroemer G. 2008. Autophagy in the pathogenesis of disease. Cell. 132(1):27–42. DOI: 10.1016/j.cell.2007.12.018.
- Li M, Lv R, Xu X, Ge Q, Lin S. 2021. Tricholoma matsutake-derived peptides show gastroprotective effects against ethanol-induced acute gastric injury. J Agric Food Chem. 69(49):14985–14994. DOI: 10.1021/acs.jafc.1c07050.
- Mahmoud YI, Abd El-Ghffar EA. 2019. Spirulina ameliorates aspirin-induced gastric ulcer in albino mice by alleviating oxidative stress and inflammation. Biomed Pharmacother. 109:314–321. DOI: 10.1016/j.biopha.2018.10.118.
- McIlwain DR, Berger T, Mak TW. 2015. Caspase functions in cell death and disease. Cold Spring Harb Perspect Biol. 7:a026716. DOI: 10.1101/cshperspect.a026716.
- Mizushima N, Levine B. 2020. Autophagy in human diseases. N Engl J Med. 383(16):1564–1576. DOI: 10.1056/NEJMra2022774.
- Mohamed DZ, El-Sisi AEE, Sokar SS, Shebl AM, Abu-Risha SE. 2021. Targeting autophagy to modulate hepatic ischemia/reperfusion injury: a comparative study between octreotide and melatonin as autophagy modulators through AMPK/PI3K/AKT/mTOR/ULK1 and Keap1/Nrf2 signaling pathways in rats. Eur J Pharmacol. 897:173920. DOI: 10.1016/j.ejphar.2021.173920.
- Müller SL, Portwich M, Schmidt A, Utepbergenov DI, Huber O, Blasig IE, Krause G. 2005. The tight junction protein occludin and the adherens junction protein alpha-catenin share a common interaction mechanism with ZO-1. J Biol Chem. 280(5):3747–3756. DOI: 10.1074/jbc.M411365200.
- Oncel S, Gupta R, Wang Q, Basson MD. 2021. ZINC40099027 promotes gastric mucosal repair in ongoing aspirin-associated gastric injury by activating focal adhesion kinase. Cells. 10:908. DOI: 10.3390/cells10040908.
- Osanai M, Murata M, Nishikiori N, Chiba H, Kojima T, Sawada N. 2007. Occludin-mediated premature senescence is a fail-safe mechanism against tumorigenesis in breast carcinoma cells. Cancer Sci. 98(7):1027–1034. DOI: 10.1111/j.1349-7006.2007.00494.x.
- Oshima T, Miwa H. 2016. Gastrointestinal mucosal barrier function and diseases. J Gastroenterol. 51(8):768–778. DOI: 10.1007/s00535-016-1207-z.
- Park MY, Ha SE, Vetrivel P, Kim HH, Bhosale PB, Abusaliya A, Kim GS. 2021. Differences of key proteins between apoptosis and necroptosis. Biomed Res Int. 2021:3420168. DOI: 10.1155/2021/3420168.
- Qiu Y, Lin X, Chen Z, Li B, Zhang Y. 2022. 5-Hydroxymethylfurfural exerts negative effects on gastric mucosal epithelial cells by inducing oxidative stress, apoptosis, and tight junction disruption. J Agric Food Chem. 70(12):3852–3861. DOI: 10.1021/acs.jafc.2c00269.
- Ren S, Wei Y, Wang R, Wei S, Wen J, Yang T, Chen X, Wu S, Jing M, Li H, et al. 2020. Rutaecarpine ameliorates ethanol-induced gastric mucosal injury in mice by modulating genes related to inflammation, oxidative stress and apoptosis. Front Pharmacol. 11:600295. DOI: 10.3389/fphar.2020.600295.
- Salah Khalil M. 2015. The postulated mechanism of the protective effect of ginger on the aspirin induced gastric ulcer: histological and immunohistochemical studies. Histol Histopathol. 30:855–864.
- Scherz-Shouval R, Elazar Z. 2011. Regulation of autophagy by ROS: physiology and pathology. Trends Biochem Sci. 36(1):30–38. DOI: 10.1016/j.tibs.2010.07.007.
- Stanton RC. 2012. Glucose-6-phosphate dehydrogenase, NADPH, and cell survival. IUBMB Life. 64(5):362–369. DOI: 10.1002/iub.1017.
- Teng JF, Qin DL, Mei QB, Qiu WQ, Pan R, Xiong R, Zhao Y, Law BY, Wong VK, Tang Y, et al. 2019. Polyphyllin VI, a saponin from Trillium tschonoskii Maxim. induces apoptotic and autophagic cell death via the ROS triggered mTOR signaling pathway in non-small cell lung cancer. Pharmacol Res. 147:104396. DOI: 10.1016/j.phrs.2019.104396.
- Tseng ZF, Hsu PI, Peng NJ, Kao SS, Tsay FW, Cheng JS, Chen WC, Tsai KF, Tang SY, Chuah SK, et al. 2021. Omeprazole vs famotidine for the prevention of gastroduodenal injury in high-risk users of low-dose aspirin: a randomized controlled trial. J Chin Med Assoc. 84(1):19–24. DOI: 10.1097/JCMA.0000000000000465.
- Wang K. 2015. Autophagy and apoptosis in liver injury. Cell Cycle. 14(11):1631–1642. DOI: 10.1080/15384101.2015.1038685.
- Yang L, Meng H, Yang M. 2016. Autophagy protects osteoblasts from advanced glycation end products-induced apoptosis through intracellular reactive oxygen species. J Mol Endocrinol. 56(4):291–300. DOI: 10.1530/JME-15-0267.
- Yang Y, Wang S, Bao YR, Li TJ, Yang GL, Chang X, Meng XS. 2017. Anti-ulcer effect and potential mechanism of licoflavone by regulating inflammation mediators and amino acid metabolism. J Ethnopharmacol. 199:175–182. DOI: 10.1016/j.jep.2017.01.053.
- Yu Y, Shiou SR, Guo Y, Lu L, Westerhoff M, Sun J, Petrof EO, Claud EC. 2013. Erythropoietin protects epithelial cells from excessive autophagy and apoptosis in experimental neonatal necrotizing enterocolitis. PLOS One. 8(7):e69620. DOI: 10.1371/journal.pone.0069620.
- Zhang MH, Feng L, Zhu MM, Gu JF, Wu C, Jia XB. 2013. Antioxidative and anti-inflammatory activities of paeoniflorin and oxypaeoniflora on AGEs-induced mesangial cell damage. Planta Med. 79(14):1319–1323. DOI: 10.1055/s-0033-1350649.
- Zhu J, Chen Y, Ji Y, Yu Y, Jin Y, Zhang X, Zhou J. 2018. Gemcitabine induces apoptosis and autophagy via the AMPK/mTOR signaling pathway in pancreatic cancer cells. Biotechnol Appl Biochem. 65(5):665–671. DOI: 10.1002/bab.1657.