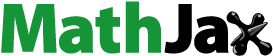
ABSTRACT
Climate smart agriculture (CSA) has been promoted by governments and international development institutions as an intervention to address climate change and deliver triple wins. Yet, the trade-offs and synergies associated with CSA practices have not been explored. This study develops composite indices for prioritizing CSA practices to better inform policy about their trade-offs, synergies and acceptability. The aim of this study was to examine smallholder farmers’ uptake of CSA practices, the drivers of such uptake and the benefits (positive and negative) of CSA practices on agriculture, livelihoods and the environment. We employed the Analytic Hierarchy Process with workshop participants across two study districts in Ghana’s transitional and Sudan savannah agroecological zones. Results showed differences in CSA practices prioritized for achieving the productivity, adaptive capacity and mitigation goals between the two agroecological zones. Results also showed synergies and trade-offs associated with the implementation of CSA interventions, for example, irrigation may increase farm productivity (synergy) while contributing to the emission of greenhouse gases (GHGs) simultaneously (trade-off). Additionally, there are various perceived acceptance and efficiency levels of CSA practices by smallholder farmers and agricultural development officers. These results have implications for the implementation of future CSA action plans in vulnerability hotspots in dryland farming systems.
1. Introduction
Climate change is a global concern that requires urgent action by individuals and governments (Goldberg et al., Citation2019; IPCC, Citation2018, Citation2021). The changing climate poses a great threat to agricultural livelihoods and global food security, due to rising sea levels, rapidly changing precipitation patterns, and intensified extreme weather events such as floods, heat stress, and increased drought, among others (Intergovernmental Panel on Climate Change (IPCC), Citation2021). Sub-Saharan Africa is particularly vulnerable due to its low adaptive capacity resulting from low levels of infrastructure and technology, inadequate resources, low levels of human development and lack of political will (Serdeczny et al., Citation2017; UNEP, Citation2011).
Agriculture accounts for more than 60% of employment across sub-Saharan Africa and contributes to about 14% of the region’s total Gross Domestic Product (GDP) (AfDB, Citation2019). Yet, the sector faces dire consequences from climate change particularly because agricultural systems are largely rainfed (Ayanlade & Radeny, Citation2020). Climate change is currently hampering gains made in the agricultural sector in recent decades and is expected to further derail productivity if left unabated (FAO, Citation2016). For example, maize yields are projected to decline between 24% and 43% (Abera et al., Citation2018) and rice yields by 45% by the end of the century (Van Oort & Zwart, Citation2018) in the absence of adaptation, with impacts affecting every aspect of daily lives and causing a significant decline in economic performance (Alagidede et al., Citation2014).
The impacts of climate change on agriculture in Ghana have multiple dimensions. For example, the total area suitable for maize cultivation is projected to reduce by 12% to 14% while longer dry spells and delayed onset of rains are expected to reduce water availability for rainfed agriculture (Amisigo et al., Citation2015). These impacts negatively affect the four dimensions of food security (availability, access, stability and utilization) (FAO, Citation2002), especially in Northern Ghana which is highly vulnerable to climate shocks and stressors (Antwi-Agyei et al., Citation2012; Klutse et al., Citation2020).
Adaptation is vital to mediate the impacts of climate change on food systems and agriculture in sub-Sahara Africa (IPCC, Citation2021). Successful adaptation measures ensure that apt adjustments are made to changes in climate and in responding to climate shocks and stress (Fadairo et al., Citation2020). Adaptation is needed by smallholder farmers as well as other actors in the food system (e.g. processors, traders, transporters). This paper focuses on smallholder farmers given their large number in Ghana and sub-Saharan Africa more generally, and the urgent need for them to adopt appropriate adaptation measures to avoid exacerbating the multiple current vulnerabilities that affect farm households (Antwi-Agyei et al., Citation2018; IPCC, Citation2014).
One key intervention promoted by governments and other agencies across the globe since 2009 to support adaptation is climate smart agriculture (CSA) (FAO, Citation2010; Lipper et al., Citation2017). Several studies have documented the importance of CSA practices in supporting food security in sub-Saharan Africa (e.g. Abegunde et al., Citation2019; Antwi-Agyei et al., Citation2022; Chinseu et al., Citation2018; Dougill et al., Citation2021; Makate, Citation2019). Climate smart agriculture aims to deliver triple wins of: (i) food security and livelihood improvement, (ii) increased farmer adaptation and (iii) mitigation of GHG emissions (Lipper et al., Citation2014). Hence, CSA practices aim to achieve three goals – productivity, mitigation and adaptive capacity. While CSA strives for multiple wins across these dimensions, trade-offs must often be made to achieve one or two goals when different CSA practices are used in combination (Andrieu et al., Citation2017). A few studies (Bryan et al., Citation2013; Swart, Citation2009) have analyzed the unintended side effects of certain CSA practices on the other goals. For example, irrigation practices may enhance productivity through increased yield and build adaptive capacity through increased income (Bryan et al., Citation2013), however, irrigation practices that rely on fossil energy may increase greenhouse gas emissions (Swart, Citation2009). Mixed cropping, another CSA practice that has the tendency to enhance the adaptive capacity of farmers through increased income from several crops, can also reduce productivity due to land degradation resulting from overcrowding of crops on a particular piece of land combined with inadequate soil nutrient replenishment.
The Government of Ghana, through its National Climate-Smart Agriculture and Food Security Action Plan: 2016–2020 and the Investment Framework for Mobilization of Resources into Climate Smart Agriculture, has sought to promote CSA by providing a pathway for the implementation and scaling up of CSA practices in all the country’s agro-ecological zones (Essegbey et al., Citation2015). Despite the development of these two policies, implementation at district/local levels remains low, leading to low adoption and effectiveness of CSA (Barasa et al., Citation2021; Djido et al., Citation2021). The limited policy implementation at the local level may be due to the top-down approach, lack of local support and/or the possible overlap of interventions coupled with weak institutional capacity to facilitate the adoption of area-specific interventions (Antwi-Agyei et al., Citation2015).
Despite the attention given to CSA over the years (Antwi-Agyei et al., Citation2022; Khatri-Chhetri et al., Citation2019; Partey et al., Citation2018; Totin et al., Citation2018), the prioritization, perceived acceptability and associated trade-offs and multiple wins across different CSA practices have not yet been adequately explored in Ghana or the wider sub-Saharan Africa region. This is problematic as existing studies elsewhere have highlighted that the adoption of several CSA practices by smallholder farmers does not automatically deliver triple wins (Girvetz et al., Citation2017). While prioritizing CSA practices is essential, the effectiveness of a practice may depend on several factors such as the biophysical, agroecological and socioeconomic context of the place (Adolph et al., Citation2021; Girvetz et al., Citation2017; Khatri-Chhetri et al., Citation2017). Multiple benefits arise when a CSA practice aimed at achieving a particular CSA goal enhances the achievement of another goal (FAO, Citation2021). In such situations, the aggregate effect of combining two or more adaptation strategies provides greater and improved benefits compared to the sum of each if they were implemented separately, delivering synergy (Zhao et al., Citation2020). Trade-offs arise when a CSA practice enables achievement of a particular CSA goal while simultaneously impeding the achievement of other goals (Akinyi et al., Citation2021; Ogunyiola et al., Citation2022). Co-benefits are sometimes used interchangeably with synergy in the literature (Berry et al., Citation2015). However, we consider a co-benefit as the additional positive impact that can be achieved on the same goal of CSA through planning and/or policy measures aimed at improving that goal (Berry et al., Citation2015; Grafakos et al., Citation2019).
The overarching aim of this study is to examine smallholder farmers’ uptake of CSA practices, the drivers of such uptake and the benefits (positive and negative) of CSA practices on agriculture, livelihoods and the environment. The specific objectives are to: (1) prioritize locally predominant CSA practices, (2) assess the perceived acceptability of these practices, and (3) explore the trade-offs and synergies associated with these locally predominant CSA practices. We adopted the Analytical Hierarchy Process (AHP): a decision-making tool that requires participants to make thought-provoking prioritizations between practices through a pairwise comparison, assigning relative importance to each practice (Khalil et al., Citation2016; Shrestha & Dhakal, Citation2019).
2. Study area and research design
2.1. Study area
The study was conducted in the Bongo district and the Kintampo North municipality in the Upper East and the Bono East regions of Ghana, respectively. The Upper East region has been identified as the most climate sensitive region in Ghana, where smallholder farmers are highly vulnerable to climate impacts (Antwi-Agyei et al., Citation2012). Within the Upper East region, Bongo district was purposefully selected as the most vulnerable based on historical crop yield data (Aniah et al., Citation2016). The Kintampo North municipality was purposively selected because it lies within the woody savannah and transitional agroecology zone and it is usefully illustrative of the impacts of climate change on Ghana as a whole (Bessah et al., Citation2019). Characteristics of the study areas are presented in .
Table 1. Background characteristics of study locations (GSS, Citation2014a, Citation2014b, Citation2021).
2.2. Sampling and data collection
Data were collected in three phases. In phase one, we employed a literature review to identify CSA practices that are commonly used by farmers in the two districts (Bongo and Kintampo). To do this, a search for the keywords ‘Climate Smart Agriculture’ AND ‘Upper East Region – Ghana’ and ‘Climate Smart Agriculture’ AND ‘Brong Ahafo Region – Ghana’ was conducted using Google Scholar. The search was limited to articles that were published in the English language since 2015 to ensure that the CSA practices identified were likely still to be in use. A total of 50 articles were identified in the database in the initial stage. The articles identified were screened to find studies in the study districts. In all, 27 CSA practices were identified from five articles that focused on the districts of interest (see Table A1 in Appendix).
In phase two, two multi-stakeholder workshops were held in September and October 2021 in Bongo (n = 29 participants; 8 females and 21 males) and Kintampo (n = 24 participants; 11 females and 13 males) to further evaluate the CSA practices used in the Upper East and the Bono East regions of Ghana. Breakout discussions and a questionnaire were used to solicit data. Workshop participants included District Directors of the Ministry of Food and Agriculture (DDAs), District Agricultural Extension Officers (DAEOs), Agricultural Extension Agents (AEAs), Civil Society Organizations (CSOs), Non-Governmental Organisations (NGOs), and farmers. Participants were purposively selected based on their comprehensive understanding and experience of local climatic changes and CSA practices within the two districts, and 80% of the participants had been formally educated.
Participants selected nine of the 27 CSA practices as the most predominant practices in the study districts. Breakout discussions prioritized the CSA practices and assessed their perceived acceptability (objectives 1 and 2), whereas questionnaires explored the trade-offs and synergies associated with these practices (objective 3). Workshop proceedings and breakout sessions discussions were recorded with the consent of participants. Participants were assured of ethical considerations in relation to anonymity and confidentiality of their responses and were informed that they would not be compensated financially for their participation.
Responses were elicited through a short, self-administered questionnaire during the workshop that focused on CSA practices in the study areas. It required participants to tick checkboxes according to the importance of each CSA practice. Information to define the goals of CSA was provided to aid participants in the task (). Each participant was required to rate the importance of CSA practices in a pairwise manner on a scale of 1–9 under each of the three CSA goals (Saaty, Citation1977, Citation1990, Citation2004, Citation2008; ). Responses were analyzed using the AHP framework designed by Goepel (Citation2013).
Table 2. Goals and definitions of CSA.
Table 3. The fundamental scale for pairwise comparison in AHP.
Of the 29 participants in Bongo, 20 responded comprehensively to the pairwise comparison of CSA practices under the goals of productivity and adaptation, whereas only 17 completely responded under mitigation. In the case of Kintampo, 20 responded under the goal of productivity, and 17 under the goals of adaptation and mitigation. Results can nevertheless be considered robust despite the small sample size because there are no pre-set rules for determining a satisfactory sample size of experts for an AHP survey (Saaty & Özdemir, Citation2014). Murry and Hammons (Citation1995) and Kumar et al. (Citation2017) contend that a sample size of 5–20 respondents is adequate for generalizing findings from AHP application.
To achieve objective 2, workshop participants were asked to score nine CSA practices based on the scale ‘1 = very low’ to ‘5 = very high’ in terms of social acceptability, economic acceptability, environmental acceptability, effectiveness, coherence with local customs, farmer implementability and equity. Calculating the overall mean acceptability of CSA practice i involved two stages. We first calculated the mean acceptability of each CSA practice separately in terms of social acceptability (SA), economic acceptability (EA), environmental acceptability (EnA), effectiveness (E), coherence with local customs (C), farmer implementability (FI) and equity (Eq). The mean acceptability (MA) for each was determined by aggregating the scores of responses from each participant and dividing it by the total number of participants (Equation 1):
(1)
(1)
Second, the overall mean acceptability (OMA) for a particular CSA practice i was calculated using the sum of the mean acceptability for each practice i and dividing by the total number of components (7) making overall acceptability (Equation 2):
(2)
(2) The mean scores obtained were considered as: 1 = very low acceptability, 2 = low acceptability, 3 = moderate acceptability, 4 = high acceptability and 5 = very high acceptability.
A two-sample t-test was further used to test for differences in the various components of the mean acceptability among the two study locations. The t-test was considered appropriate for testing the difference in means of the two samples because the mean acceptability scores were continuous in nature and they approximate a normal distribution (Mishra et al., Citation2019). Moreover, the Levene’s Test for equality of variances ensured that the data fulfilled the equal variance assumption (Levene, Citation1960).
In phase 3, workshop participants were tasked to identify synergies and trade-offs associated with CSA practices as experienced in their localities (objective 3). Participants were sub-divided and engaged in 6 smaller groups in breakout sessions (3 at each workshop), with 7–11 participants per group. Breakout group discussions provided valuable insights based on the participants’ lived farming experiences. To facilitate equal opportunities for participation, each group constituted of individuals with similar occupations and ranks. Discussions were led by research assistants to balance power relations and to ensure everyone had space to speak. Each group reported back on the synergies and trade-offs identified.
Responses were corroborated with information from the literature through a further literature review conducted after the workshop. Google Scholar was used for the literature search. The search terms/keywords and phrases used for the study included ‘trade-offs’ OR ‘synergies’ OR ‘adaptation’ OR ‘mitigation’ OR ‘productivity’ OR ‘agriculture’ OR ‘climate change’ OR ‘climate smart’ OR ‘sub-Saharan Africa’ OR ‘cost-benefit’ OR ‘cost-effectiveness’ OR ‘willingness to pay’ OR ‘willingness to accept’ OR ‘interventions’ OR ‘practices’ OR ‘irrigation’ OR ‘zero/minimum tillage’ OR ‘intercropping with legumes’ OR ‘no burning of residues’ OR ‘agroforestry and woodlot’ OR ‘drought tolerant crops’ OR ‘early maturing crops’ OR ‘improved crop varieties’ OR ‘mixed cropping’ OR ‘cover cropping’ OR ‘climate information services’ OR ‘bush fallowing’ OR ‘indigenous agroecological knowledge’. A total of 67 studies were identified from the database in our initial search. The first stage entailed screening to remove all duplicates and irrelevant literature. This was followed by title screening to remove other irrelevant articles. The title screening was followed by abstract and then full text screening to complete the process. Ultimately, 25 studies were retained. Information and evidence about synergies and trade-offs of prioritized CSA practices were systematically extracted from the 25 retained studies. The extracted evidence was then compared with the responses from workshop participants to highlight similarities and differences.
The Humanities and Social Sciences Research Ethics Committee (HuSSRECC) of the Kwame Nkrumah University of Science and Technology, Ghana provided the ethical approval for this study. Formal consent for participation in the study was obtained verbally from each study participant.
3. Results
3.1. Prioritizing CSA practices under productivity, adaptation and mitigation goals
and summarize the weights and ranking participants assigned to CSA practices in Bongo and Kintampo, respectively. In Bongo, the most important CSA practice for achieving productivity was planting early maturing crop varieties followed by intercropping with legumes and the use of drought tolerant crops. In relation to adaptation, the experts ranked no burning of residues on farm as the most important, followed by cover cropping and planting early maturing varieties. In terms of mitigation, participants ranked no burning of residues on farm as the most important CSA practice followed by zero/minimum tillage and intercropping with legumes. In Kintampo however, our AHP expert ranking revealed that irrigation was perceived to be the most important CSA practice for achieving the productivity goal of CSA, followed by planting early maturing varieties of crops and the use of drought tolerant crop varieties. In terms of achieving adaptation, the ranking by workshop participants in Kintampo revealed planting of early maturing crop varieties as the most important CSA practice, followed by the use of drought tolerant crop varieties and agroforestry and woodlot schemes. No burning of farm residues was ranked as the most important CSA practice for achieving mitigation, followed by agroforestry, woodlot schemes and zero/minimum tillage.
Table 4. Summary of relative weights and ranking of CSA practices in Bongo.
Table 5. Summary of relative weights and ranking of CSA practices in Kintampo.
Participants in Bongo ranked the use of climate information services as the least important CSA practice for achieving productivity. Irrigation was considered least important for achieving adaptation and mitigation. In Kintampo however, zero/minimum tillage was ranked as the least important CSA practice for achieving productivity, while irrigation was ranked least important for achieving both adaptation and mitigtion (see and ).
4. Perceived acceptability of CSA practices
Overall mean acceptability of CSA practices was relatively higher among workshop participants in Kintampo than those in Bongo ( and ) except for zero/minimum tillage and the use of indigenous knowledge. Workshop participants in Kintampo showed a higher social acceptability towards the CSA practices than their counterparts in the Bongo District. Although economic acceptability of the various practices was relatively higher in Bongo than Kintampo except in the cases of irrigation, intercropping with legumes and the use of climate information, there were no statistically significant differences in economic acceptability between the two study locations.
Table 6. Perceived acceptability of CSA – Bongo.
Table 7. Perceived acceptability of CSA practices in Kintampo.
Irrigation and intercropping with legumes recorded higher environmental acceptability scores among participants in Bongo than in Kintampo. However, environmental acceptability of the CSA practices in Kintampo was higher than those in the Bongo district. Scores for effectiveness showed no statistically significant differences between the two study locations. Acceptability scores for coherence with local customs were significantly higher in Kintampo than in Bongo district. However, there were no significant differences in the acceptability scores in terms of farmer implementability and equity between the two study areas.
5. Synergies and trade-offs associated with prioritized CSA practices
Workshop participants were asked to identify and describe the synergies and trade-offs of CSAs prevalent in their localities, which were then compared with those identified in existing literature (). For example, participants identified that agroforestry and woodlot schemes may improve productivity due to their ability to conserve soil moisture and the fixation of nitrogen, however, harvesting of woodlots may increase emissions of GHGs and undermine mitigation efforts.
Table 8. Synergies and trade-offs of CSA practices from focused group discussions (FGD) and existing literature.
Many of the synergies associated with irrigation, zero/minimum tillage, mixed cropping and cover cropping were identified by both workshop participants and the literature. However, only a few of the trade-offs associated with these practices were identified by both workshop participants and the literature (see ). The differences in trade-offs identified result from the focus of the impact observed. For example, while the trade-offs associated with irrigation in the literature focused on the emission of GHGs, workshop participants identified trade-offs that pressurized the limited resources available to farmers.
Similarities in the synergies identified by both workshop participants and the literature were mostly associated with increased productivity, which ultimately leads to improved income and household welfare. On the contrary, disparities in the synergies identified by the workshop participants and the literature were mainly biophysical. Workshop participants mainly identified trade-offs that had a direct and immediate impact on farmers such as increased demand on income and labour while those identified in the literature mainly focused on trade-offs whose impact manifest over time, such as depleted land fertility and increased emission of GHGs (see ).
6. Discussion
No burning of crop residue was highly prioritized as aiding the attainment of the three goals of CSA among participants from both study locations. Proper management of crop residues contributes to improved soil structure and fertility, weed suppression and soil water retention (Giller et al., Citation2009; Jat et al., Citation2021; Jellason et al., Citation2021) and as such, no burning of crop residues is significant for the attainment of the three goals of CSA. The practice is highly acceptable in the study locations mainly because of its easy implementation and because it is coherent with the local customs of the people (Anderson & Siddique, Citation2015). Several studies (Alkhtib et al., Citation2017; Jaleta et al., Citation2015; Valbuena et al., Citation2015) indicate that farmers are increasingly accepting the practice of no burning because crop residues have numerous uses in the household and the community in terms of cooking fuel, livestock feed, building material and mulch. However, larger farms may find it difficult due to resource constraints associated with sustainable crop residue management practices (Adolph et al., Citation2021).
In spite of the high prioritization and acceptability of no burning of residues/crop residue retention, there are trade-offs that if not properly mitigated could adversely affect farmers’ adoption of the practice. Crop residue retention has the potential to escalate bush fire outbreaks because residues are potential fuel for any spark (Westcott, Citation2019), which could then hamper mitigation. Delayed crop residue decomposition on the farm interferes with other farming activities and may require additional farm labour, exerting more pressure on the meagre resources of smallholder farmers (Cavalli et al., Citation2018; Mizik, Citation2021) and reducing farmers’ adaptive capacity. Despite the trade-offs associated with this practice, no burning of residues has potential synergies that could promote its adoption by farmers. Several studies (Amorim et al., Citation2021; Lankoski et al., Citation2018; Shiwakoti et al., Citation2019) show that it contributes to the productivity and adaptation goals of CSA by supporting crop yields, enhancing soil organic carbon and total nitrogen, as well as retaining soil moisture and reducing yield variability.
The use of improved crops (drought tolerant and early maturing varieties) ranked highly among participants in both workshops. Improved crop varieties are essential to the fight against climate change and food insecurity (Antwi-Agyei & Nyantakyi-Frimpong, Citation2021; Seyoum et al., Citation2020). Improved crops varieties were acceptable among participants in both groups because the practice has been demonstrated to produce good yields and withstand rising temperature and erratic rainfall (Debaeke et al., Citation2017; Seyoum et al., Citation2020; Valarmathi et al., Citation2019). Another synergistic feature associated with the use of improved crop varieties is that farmers are better able to cope with the risk associated with rainfall variability (Sanou et al., Citation2016). Improved crop varieties ensure that farmers are able to cultivate in the face of uncertain rainfall patterns and still harvest. However, the continuous use of improved crop varieties requires the increasing use of agrochemicals which could contribute to emission of GHGs and further damage the soil in the long term (Miflin, Citation2000).
Another set of highly prioritized and acceptable CSA practices in the study locations was mixed cropping, intercropping with legumes and cover cropping. These cropping systems are highly similar and serve comparable purposes. Synergies associated with these cropping systems include improved biodiversity within farming systems and reduced total crop failure, which in turn reduces the likelihood of food insecurity among farm households (Bonke & Musshoff, Citation2020). Again, these practices ensure better utilization of available resources, improve natural nitrogen fixation, prevent erosion and crop failure, and assist in weed, pest and disease management (Gogoi et al., Citation2018; Maitra et al., Citation2020; Silberg et al., Citation2020; Solanki et al., Citation2020). Farmers who adopt these practices enjoy the synergies between enhanced productivity and adaptive capacity due to the varieties of crops cultivated. However, these practices have trade-offs with respect to GHG emissions as they have the potential to increase competition for moisture, solar radiation and nutrients (Layek et al., Citation2018). This can also lead to increased emission of nitrous-oxide and carbon dioxide because of increased decomposition activity by microbes (Basche et al., Citation2016; Daryanto et al., Citation2018). Additionally, these practices can increase pest and disease infestations, which may require increased use of chemicals with associated environmentally damaging effects (Nassary et al., Citation2020).
Unlike other practices, the prioritization and acceptability of irrigation diverged among study participants. The scores on economic acceptability and farmer implementability indicated that the adoption of proper irrigation systems was economically prohibitive. A smart irrigation system that can save about 80% of water is expensive and can affect farmers’ ability to adapt to the impact of climate change (Asare-Nuamah et al., Citation2021; Darshna et al., Citation2015). Economically, farmers with low resource endowments cannot meet the financial requirements for rolling out a climate-smart irrigation scheme and may thus be unwilling to accept such a practice (Waaswa et al., Citation2022). However, farmers that are able to adopt smart-irrigation systems are likely to achieve high yields and reduced production variability (Lankoski et al., Citation2018; Zaveri & Lobell, Citation2019), further increasing their income, while also building their resilience because they can engage in dry season farming. Such synergistic effects are more likely to occur in large farms than small farms because large farms can achieve economies of scale which can translate into higher production efficiency (Borychowski et al., Citation2022). However, irrigation may fuel competition for water resources which may erupt into resource conflict, especially in scarce water environments (Antwi-Agyei et al., Citation2015; Asare-Nuamah et al., Citation2021). Also, irrigation systems that are mostly adopted by smallholder farmers tend to be non-smart and powered by fossil fuel energy sources, leading to an increased GHG emissions (Sapkota et al., Citation2020). GHG emissions from irrigation may arise in four different ways: from surface water pumping, ground water pumping, water conveyance, and production and construction of irrigation facilities (Zou et al., Citation2015).
Given the difference in prioritizations and the level of acceptability of CSA practices coupled with their varying synergies and trade-offs, it is essential for farmers to adopt a combination of CSA practices that complement each other. It is also important that farmers fully exploit the synergies and trade-offs associated with the adoption of CSAs to suit their specific circumstances (Ochieng et al., Citation2022). This requires consideration of not just the synergies and tradeoffs in terms of environmental outcomes but also in terms of factors such as seasonal labour demands, when in the agricultural cycle each practice needs to be implemented, and temporal variations in household income that is available for investment in CSA. When these kinds of supporting factors align, farmers in Bongo district may obtain synergies by combining the use of e.g. no burning of crop residues, drought tolerant varieties and smart irrigation practices given that farmers in that part of the country are more prone to drought. Farmers in Kintampo may have to adopt a different combination of practices such as no burning of crop residues, intercropping with legumes and the planting of early maturing varieties because they face different climatic challenges. Each combination of practices has different effects on the overall outcomes and requires further research, while the characteristics of the farmers and their resource endowments are known to affect the uptake of CSA practices.
Socio-economic factors such as farm size, education level, age, gender, availability of resources and the main source of income of a farmer interact to influence CSA adoption (Waaswa et al., Citation2022). Additionally, institutional factors such as the land tenure system, access to credit, access to training on CSAs, membership of farmer-based groups and receipt of support from non-governmental groups have also been shown to affect the adoption of CSA practices (Anuga et al., Citation2019). Recognizing these factors, and in addition to them, synergies and trade-offs associated with CSA practices can advance or hamper their adoption among farmers, and also can shape their disadoption (Chinseu et al., Citation2019a, Citation2019b). This study adds to the extant literature and improves our knowledge on the drivers (acceptability) of CSA practices by assessing these synergies and trade-offs.
7. Conclusion and policy implications
The results from our study bring to light the differences in the prioritization and acceptance of CSA practices among Ghanaian farmers based on their location, suggesting that CSA design and implementation need to be location-specific and that there is not a one-size-fits-all approach relevant across all of sub-Saharan Africa. Farmers in different agroecological zones in Ghana have unique characteristics peculiar to their context in addition to the general conditions of the Ghanaian farmers. As such, understanding the conditions within farmers environment and providing fit-for-purpose, acceptable interventions that harness synergies would contribute immensely to achieving triple wins. Our results also demonstrate that while farmers have highly prioritized and acceptable CSA practices in the study locations, the synergies and trade-offs associated with these CSA practices can greatly influence farmers’ decisions to adopt them.
Based on our findings, we recommend that implementation of future CSA action plans must be location-specific and not a blanket policy from the national level. Thus, the biophysical and socioeconomic contexts of farmers are critical to scaling up effective CSA practices and farmer participation and engagement in the design and delivery of CSA becomes vital. CSA practices must be piloted to ensure that locals prioritize and accept them especially as favourable synergy-trade-off outcomes emerge over longer time frames. Additionally, a combination of CSA practices, such as mixed cropping, intercropping with legumes, cover cropping and no burning of crop residues, among others that have proven highly effective and acceptable in dryland farming systems, could be usefully promoted at scale among local farmers. This will minimize policy and programme failures and ensure that the limited resources available to local governments will be spent on proven practices that are more likely to be taken up. In addition, CSA practices that put less pressure on the already constrained budget of farmers should be promoted because farmers regard implementation cost as an key factor of CSA adoption. This is evident from the finding that the economic acceptability of CSA practices such as irrigation, improved crop varieties and the use of climate information remains relatively low as they are capital intensive and farmers have limited capacities and resources to adopt such practices. Given the poverty levels among smallholder farmers in Ghana, economic viability of CSA remains a priority, which may relegate mitigation concerns. Social interventions that improve farmers’ access to socioeconomic resources should equally be prioritized by state and local institutions to enhance comprehensive achievement of CSA goals. Findings from this paper have implications for the adoption of CSA practices in different agro-ecological zones in Ghana as different combinations of practices are more or less suitable depending on the context, and deliver different balances of synergy and trade-offs. This insight also applies at the wider sub-Saharan Africa scale, where agro-ecological, socioeconomic and political characteristics need to be factored into the design and implementation of location-specific CSA practices aimed at improving productivity, enhancing adaptation and reducing GHGs emissions.
Acknowledgements
The authors would like to express their gratitude to the workshop participants.
Additional information
Funding
References
- Abegunde, V. O., Sibanda, M., & Obi, A. (2019). The dynamics of climate change adaptation in sub-Saharan Africa: A review of climate-smart agriculture among small-scale farmers. Climate, 7(11), 132. https://doi.org/10.3390/cli7110132
- Abera, K., Crespo, O., Seid, J., & Mequanent, F. (2018). Simulating the impact of climate change on maize production in Ethiopia, East Africa. Environmental Systems Research, 7(1), 1–12. https://doi.org/10.1186/s40068-017-0104-7
- Adolph, B., Allen, M., Beyuo, E., Banuoku, D., Barrett, S., Bourgou, T., Bwanausi, N., Dakyaga, F., Derbile, E. K., Gubbels, P., Hié, B., Kachamba, C., Naazie, G. K., Niber, E. B., Nyirengo, I., Tampulu, S. F., & Zongo, A. F. (2021). Supporting smallholders’ decision making: Managing trade-offs and synergies for sustainable agricultural intensification. International Journal of Agricultural Sustainability, 19(5–6), 456–473. https://doi.org/10.1080/14735903.2020.1786947
- African Development Bank. (2019). Feed Africa.
- Agula, C., Akudugu, M. A., Dittoh, S., & Mabe, F. N. (2018). Promoting sustainable agriculture in Africa through ecosystem-based farm management practices: Evidence from Ghana. Agriculture & Food Security, 7(1), 1–11. https://doi.org/10.1186/s40066-018-0157-5
- Akinyi, D. P., Karanja Ng’ang’a, S., & Girvetz, E. H. (2021). Trade-offs and synergies of climate change adaptation strategies among smallholder farmers in sub-Saharan Africa: A systematic review. Regional Sustainability, 2(2), 130–143. https://doi.org/10.1016/j.regsus.2021.05.002
- Alagidede, P., Adu, G., & Frimpong, P. B. (2014). The effect of climate change on economic growth (Working Paper 17/2014). United Nations University.
- Alkhtib, A., Wamatu, J., Kassie, G. T., & Rischkowsky, B. (2017). Analysis of crop residue use in small holder mixed farms in Ethiopia. Renewable Agriculture and Food Systems, 32(5), 454–462. https://doi.org/10.1017/S1742170516000399
- Amisigo, B. A., McCluskey, A., & Swanson, R. (2015). Modeling impact of climate change on water resources and agriculture demand in the Volta Basin and other basin systems in Ghana. Sustainability, 7(6), 6957–6975. https://doi.org/10.3390/su7066957
- Amorim, H. C., Ashworth, A. J., Brye, K. R., Wienhold, B. J., Savin, M. C., Owens, P. R., & Silva, S. H. (2021). Soil quality indices as affected by long-term burning, irrigation, tillage, and fertility management. Soil Science Society of America Journal, 85(2), 379–395. https://doi.org/10.1002/saj2.20188
- Anderson, W. K., & Siddique, K. H. M. (2015). The role and value of crop residues in dryland agriculture. Indian Journal of Agronomy, 60(3), 332–340.
- Andrieu, N., Sogoba, B., Zougmore, R., Howland, F., Samake, O., Bonilla-Findji, O., Lizarazo, M., Nowak, A., Dembele, C., & Corner-Dolloff, C. (2017). Prioritizing investments for climate-smart agriculture: Lessons learned from Mali. Agricultural Systems, 154, 13–24. https://doi.org/10.1016/j.agsy.2017.02.008
- Aniah, P., Kaunza-Nu-Dem, M. K., Abindaw, B. A., & Millar, D. (2016). Characterizing and explaining smallholder households’ views and understanding of climate change in the Bongo district of Ghana. Earth Sciences, 5(2), 26. https://doi.org/10.11648/j.earth.20160502.12
- Antwi-Agyei, P., Abalo, E. M., Dougill, A. J., & Baffour-Ata, F. (2022). Motivations, enablers and barriers to the adoption of climate-smart agricultural practices by smallholder farmers: Evidence from the transitional and savannah agroecological zones of Ghana. Regional Sustainability, 2(4), 375–386. https://doi.org/10.1016/j.regsus.2022.01.005
- Antwi-Agyei, P., Amanor, K., Hogarh, J. N., & Dougill, A. J. (2021). Predictors of access to and willingness to pay for climate information services in north-eastern Ghana: A gendered perspective. Environmental Development, 37, Article 100580. https://doi.org/10.1016/j.envdev.2020.100580
- Antwi-Agyei, P., Dougill, A. J., & Stringer, L. C. (2015). Barriers to climate change adaptation: Evidence from northeast Ghana in the context of a systematic literature review. Climate and Development, 7(4), 297–309. https://doi.org/10.1080/17565529.2014.951013
- Antwi-Agyei, P., Dougill, A. J., Stringer, L. C., & Codjoe, S. N. A. (2018). Adaptation opportunities and maladaptive outcomes in climate vulnerability hotspots of northern Ghana. Climate Risk Management, 19, 83–93. https://doi.org/10.1016/j.crm.2017.11.003
- Antwi-Agyei, P., Fraser, E. D., Dougill, A. J., Stringer, L. C., & Simelton, E. (2012). Mapping the vulnerability of crop production to drought in Ghana using rainfall, yield and socioeconomic data. Applied Geography, 32(2), 324–334. https://doi.org/10.1016/j.apgeog.2011.06.010
- Antwi-Agyei, P., & Nyantakyi-Frimpong, H. (2021). Evidence of climate change coping and adaptation practices by smallholder farmers in northern Ghana. Sustainability, 13(3), 1308. https://doi.org/10.3390/su13031308
- Anuga, S. W., Gordon, C., Boon, E., & Surugu, J. M. I. (2019). Determinants of climate smart agriculture (CSA) adoption among smallholder food crop farmers in the Techiman Municipality, Ghana. Ghana Journal of Geography, 11(1), 124–139. https://doi.org/10.4314/gjg.v11i1.8
- Asare-Nuamah, P., Dick-Sagoe, C., & Ayivor, R. (2021). Farmers’ maladaptation: Eroding sustainable development, rebounding and shifting vulnerability in smallholder agriculture system. Environmental Development, 40, Article 100680. https://doi.org/10.1016/j.envdev.2021.100680
- Ayanlade, A., & Radeny, M. (2020). COVID-19 and food security in sub-Saharan Africa: Implications of lockdown during agricultural planting seasons. NPJ Science of Food, 4(1), 1–6. https://doi.org/10.1038/s41538-020-00073-0
- Barasa, P. M., Botai, C. M., Botai, J. O., & Mabhaudhi, T. (2021). A review of climate-smart agriculture research and applications in Africa. Agronomy, 11(6), 1255. https://doi.org/10.3390/agronomy11061255
- Basche, A. D., Kaspar, T. C., Archontoulis, S. V., Jaynes, D. B., Sauer, T. J., Parkin, T. B., & Miguez, F. E. (2016). Soil water improvements with the long-term use of a winter rye cover crop. Agricultural Water Management, 172, 40–50. https://doi.org/10.1016/j.agwat.2016.04.006
- Beedy, T. L., Nyamadzawo, G., Luedeling, E., Kim, D. G., Place, F., & Hadgu, K. (2014). Agroforestry for small landholders of eastern and southern Africa. In: Lal R, Stewart BA (eds) Soil management of smallholder agriculture. CRC Press, Taylor and Francis Group, Boca Raton (pp. 238–259).
- Berry, P. M., Brown, S., Chen, M., Kontogianni, A., Rowlands, O., Simpson, G., & Skourtos, M. (2015). Cross-sectoral interactions of adaptation and mitigation measures. Climatic Change, 128(3), 381–393. https://doi.org/10.1007/s10584-014-1214-0
- Bessah, E., Bala, A., Agodzo, S. K., Okhimamhe, A. A., Boakye, E. A., & Ibrahim, S. U. (2019). The impact of crop farmers’ decisions on future land use, land cover changes in Kintampo North Municipality of Ghana. International Journal of Climate Change Strategies and Management. https://doi.org/10.1108/IJCCSM-05-2017-0114
- Blaser, W. J., Oppong, J., Hart, S. P., Landolt, J., Yeboah, E., & Six, J. (2018). Climate-smart sustainable agriculture in low-to-intermediate shade agroforests. Nature Sustainability, 1(5), 234–239. https://doi.org/10.1038/s41893-018-0062-8
- Bonke, V., & Musshoff, O. (2020). Understanding German farmer’s intention to adopt mixed cropping using the theory of planned behavior. Agronomy for Sustainable Development, 40(6), 1–14. https://doi.org/10.1007/s13593-020-00653-0
- Borychowski, M., Sapa, A., Czyżewski, B., Stępień, S., & Poczta-Wajda, A. (2022). Interactions between food and nutrition security and the socio-economic and environmental dimensions of sustainability in small-scale farms: Evidence from a simultaneous confirmatory factor analysis in Poland. International Journal of Agricultural Sustainability, 20(5), 998–1014. https://doi.org/10.1080/14735903.2022.2041230
- Bryan, E., Ringler, C., Okoba, B., Koo, J., Herrero, M., & Silvestri, S. (2013). Can agriculture support climate change adaptation, greenhouse gas mitigation and rural livelihoods? Insights from Kenya. Climatic Change, 118(2), 151–165. https://doi.org/10.1007/s10584-012-0640-0
- Cavalli, E., Lange, A., Cavalli, C., & Behling, M. (2018). Decomposition and release of nutrients from crop residues on soybean-maize cropping systems. Brazilian Journal of Agrarian Sciences, 13(2), 1–8. https://doi.org/10.5039/agraria.v13i2a5527
- Chataway, R. G., Cooper, J. E., Orr, W. N., & Cowan, R. T. (2011). The role of tillage, fertiliser and forage species in sustaining dairying based on crops in southern Queensland 2. Double-crop and summer sole-crop systems. Animal Production Science, 51(10), 904–919. https://doi.org/10.1071/AN11032
- Chinseu, E., Stringer, L., & Dougill, A. (2018). Policy integration and coherence for conservation agriculture initiatives in Malawi. Sustainable Agriculture Research, 7(4), 51–62. https://doi.org/10.5539/sar.v7n4p51
- Chinseu, E. L., Stringer, L. C., & Dougill, A. J. (2019a). An empirically derived conceptual framework to assess dis-adoption of conservation agriculture. Journal of Sustainable Development, 12(5), 48–64. https://doi.org/10.5539/jsd.v12n5p48
- Chinseu, E. L., Stringer, L. C., & Dougill, A. J. (2019b). Why do smallholder farmers dis-adopt conservation agriculture? Insights from Malawi. Land Degradation and Development, 30(5), 533–543. https://doi.org/10.1002/ldr.3190
- Darshna, S., Sangavi, T., Mohan, S., Soundharya, A., & Desikan, S. (2015). Smart irrigation system. IOSR Journal of Electronics and Communication Engineering (IOSR-JECE), 10(3), 32–36. https://doi.org/10.9790/2834-10323236
- Daryanto, S., Fu, B., Wang, L., Jacinthe, P. A., & Zhao, W. (2018). Quantitative synthesis on the ecosystem services of cover crops. Earth-Science Reviews, 185, 357–373. https://doi.org/10.1016/j.earscirev.2018.06.013
- Debaeke, P., Pellerin, S., & Scopel, E. (2017). Climate-smart cropping systems for temperate and tropical agriculture: Mitigation, adaptation and trade-offs. Cahiers Agricultures, 26(3), 1–12. https://doi.org/10.1051/cagri/2017028
- Djido, A., Segnon, A. C., Botchway, V. A., Karbo, N., Sam, K. O., Essegbey, G. O., Nutsukpo, D. K., Asafu-Adjaye, N. Y., Agyemang, K., Whitbread, A., Thornton, T., & Zougmoré, R. B. (2021). Implementation, usage, and effectiveness of Ghana climate change policies: An assessment of the national CSA action plan and CSA investment framework. CCAFS Info Note.
- Dougill, A. J., Hermans, T. D., Eze, S., Antwi-Agyei, P., & Sallu, S. M. (2021). Evaluating climate-smart agriculture as route to building climate resilience in African food systems. Sustainability, 13(17), 9909. https://doi.org/10.3390/su13179909
- Essegbey, G. O., Nutsukpo, D., Karbo, N., & Zougmoré, R. (2015). National climate-smart agriculture and food security action plan of Ghana (2016–2020). Ghana Government.
- Fadairo, O., Williams, P. A., & Nalwanga, F. S. (2020). Perceived livelihood impacts and adaptation of vegetable farmers to climate variability and change in selected sites from Ghana, Uganda and Nigeria. Environment, Development and Sustainability, 22(7), 6831–6849. https://doi.org/10.1007/s10668-019-00514-1
- Food and Agriculture Organisation. (2002). The state of food and agriculture 2002.
- Food and Agriculture Organization of the United Nations. (2010). Climate smart agriculture: Policies, practices and financing for food security, adaptation and mitigation.
- Food and Agriculture Organization of the United Nations. (2016). The state of food and agriculture: Climate change, agriculture and food security.
- Food and Agriculture Organization of the United Nations. (2021). Synergies and trade-offs in climate-smart agriculture – an approach to systematic assessment.
- Ghana Statistical Service. (2014a). 2010 population and housing census. Bongo District analytical report. https://www2.statsghana.gov.gh/docfiles/2010_District_Report/Upper%20East/Bongo.pdf
- Ghana Statistical Service. (2014b). 2010 population and housing census. Kintampo South District analytical report. https://www2.statsghana.gov.gh/docfiles/2010_District_Report/Brong%20Ahafo/Kintampo%20South_.pdf
- Ghana Statistical Service. (2021). Ghana population and housing census (General Report. Vol. 3A).
- Giller, K. E., Witter, E., Corbeels, M., & Tittonell, P. (2009). Conservation agriculture and smallholder farming in Africa: The heretics’ view. Field Crops Research, 114(1), 23–34. https://doi.org/10.1016/j.fcr.2009.06.017
- Girvetz, E. H., Corner-Dolloff, C., Lamanna, C., & Rosenstock, T. S. (2017). ‘CSA-plan’: Strategies to put climate-smart agriculture (CSA) into practice. Agriculture for Development, 30, 12–16. https://hdl.handle.net/10568/81374.
- Goepel, K. D. (2013, June). Implementing the analytic hierarchy process as a standard method for multi-criteria decision making in corporate enterprises – a new AHP excel template with multiple inputs. In Proceedings of the International Symposium on the Analytic Hierarchy Process (Vol. 2, No. 10, pp. 1–10). Creative Decisions Foundation.
- Gogoi, N., Baruah, K. K., & Meena, R. S. (2018). Grain legumes: Impact on soil health and agroecosystem. In Meena, R. S., Das, A., Yadav, G. S., & Lal, R. (Eds.) Legumes for soil health and sustainable management (pp. 511–539). Springer.
- Goldberg, M. H., van der Linden, S., Maibach, E., & Leiserowitz, A. (2019). Discussing global warming leads to greater acceptance of climate science. Proceedings of the National Academy of Sciences, 116(30), 14804–14805. https://doi.org/10.1073/pnas.1906589116
- Grafakos, S., Trigg, K., Landauer, M., Chelleri, L., & Dhakal, S. (2019). Analytical framework to evaluate the level of integration of climate adaptation and mitigation in cities. Climatic Change, 154(1), 87–106. https://doi.org/10.1007/s10584-019-02394-w
- IPCC. (2014). Summary for policymakers. Climate change 2014: Impacts, adaptation, and vulnerability. Part A: Global and sectoral aspects (pp. 1–32). Contribution of Working Group II to the Fifth Assessment Report of the Intergovernmental Panel on Climate Change. Cambridge University Press.
- IPCC. (2018). Intergovernmental Panel on Climate Change, ‘global warming of 1.5°C’.
- IPCC. (2021). Climate change 2021: The physical science basis. Contribution of Working Group I to the Sixth Assessment Report of the Intergovernmental Panel on Climate Change.
- Issahaku, G., & Abdulai, A. (2020). Can farm households improve food and nutrition security through adoption of climate-smart practices? Empirical evidence from northern Ghana. Applied Economic Perspectives and Policy, 42(3), 559–579. https://doi.org/10.1093/aepp/ppz002
- Jaleta, M., Kassie, M., & Erenstein, O. (2015). Determinants of maize stover utilization as feed, fuel and soil amendment in mixed crop-livestock systems, Ethiopia. Agricultural Systems, 134, 17–23. https://doi.org/10.1016/j.agsy.2014.08.010
- Jat, H. S., Datta, A., Choudhary, M., Sharma, P. C., & Jat, M. L. (2021). Conservation agriculture: Factors and drivers of adoption and scalable innovative practices in Indo-Gangetic plains of India – a review. International Journal of Agricultural Sustainability, 19(1), 40–55. https://doi.org/10.1080/14735903.2020.1817655
- Jellason, N. P., Conway, J. S., & Baines, R. N. (2021). Understanding impacts and barriers to adoption of climate-smart agriculture (CSA) practices in North-Western Nigerian drylands. The Journal of Agricultural Education and Extension, 27(1), 55–72. https://doi.org/10.1080/1389224X.2020.1793787
- Khalil, N., Kamaruzzaman, S. N., & Baharum, M. R. (2016). Ranking the indicators of building performance and the users’ risk via Analytical Hierarchy Process (AHP): Case of Malaysia. Ecological Indicators, 71, 567–576. https://doi.org/10.1016/j.ecolind.2016.07.032
- Khatri-Chhetri, A., Aggarwal, P. K., Joshi, P. K., & Vyas, S. (2017). Farmers’ prioritization of climate-smart agriculture (CSA) technologies. Agricultural Systems, 151, 184–191. https://doi.org/10.1016/j.agsy.2016.10.005
- Khatri-Chhetri, A., Pant, A., Aggarwal, P. K., Vasireddy, V. V., & Yadav, A. (2019). Stakeholders prioritization of climate-smart agriculture interventions: Evaluation of a framework. Agricultural Systems, 174, 23–31. https://doi.org/10.1016/j.agsy.2019.03.002
- Klutse, N. A. B., Owusu, K., & Boafo, Y. A. (2020). Projected temperature increases over northern Ghana. SN Applied Sciences, 2(8), 1–14. https://doi.org/10.1007/s42452-020-3095-3
- Kumar, N., Singh, S. K., Mishra, V. N., Reddy, G. P., & Bajpai, R. K. (2017). Soil quality ranking of a small sample size using AHP. Journal of Soil and Water Conservation, 16(4), 339–346. https://doi.org/10.5958/2455-7145.2017.00050.9
- Kumar, S., Mamidanna, S., Rao, K. P. C., & Whitbread, A. M. (2018). Identifying low emissions development pathways–synergies and trade-offs: A case study of Mahbubnagar District, Telangana, India.
- Lankoski, J., Ignaciuk, A., & Jésus, F. (2018). Synergies and trade-offs between adaptation, mitigation and agricultural productivity: A synthesis report.
- Layek, J., Das, A., Mitran, T., Nath, C., Meena, R. S., Yadav, G. S., Shivakumar, B. G., Kumar, S., & Lal, R. (2018). Cereal+ legume intercropping: An option for improving productivity and sustaining soil health. In Meena, R. S., Das, A., Yadav, G. S., & Lal, R. (Eds.). Legumes for soil health and sustainable management (pp. 347–386). Springer.
- Levene, H. (1960). Robust tests for equality of variances. In I. Olkin, S. G. Ghurye, W. Hoeffding, W. G. Madow, & H. B. Mann (Eds.), Contributions to probability and statistics: Essays in honor of Harold hotelling (pp. 278–292). Stanford University Press.
- Lipper, L., McCarthy, N., Zilberman, D., Asfaw, S., & Branca, G. (2017). Climate smart agriculture: Building resilience to climate change (p. 630). Springer Nature.
- Lipper, L., Thornton, P., Campbell, B. M., Baedeker, T., Braimoh, A., Bwalya, M., Caron, P., Cattaneo, A., Garrity, D., Henry, K., Hottle, R., Jackson, L., Jarvis, A., Kossam, F., Mann, W., McCarthy, N., Meybeck, A., Neufeldt, H., Remington, T., … Torquebiau, E. F. (2014). Climate-smart agriculture for food security. Nature Climate Change, 4(12), 1068–1072. https://doi.org/10.1038/nclimate2437
- Loboguerrero, A. M., Campbell, B. M., Cooper, P. J., Hansen, J. W., Rosentsock, T., & Wollenberg, E. (2019). Food and earth systems: Priorities for climate change adaptation and mitigation for agriculture and food systems. Sustainability, 11(5), 1372. https://doi.org/10.3390/su11051372
- Maitra, S., Shankar, T., & Banerjee, P. (2020). Potential and advantages of maize-legume intercropping system. In A. Hossain (Ed.), Maize – production and use. IntechOpen. https://doi.org/10.5772/intechopen.91722
- Makate, C. (2019). Effective scaling of climate smart agriculture innovations in African smallholder agriculture: A review of approaches, policy and institutional strategy needs. Environmental Science & Policy, 96, 37–51. https://doi.org/10.1016/j.envsci.2019.01.014
- Miflin, B. (2000). Crop improvement in the 21st century. Journal of Experimental Botany, 51(342), 1–8. https://doi.org/10.1093/jexbot/51.342.1
- Mishra, P., Singh, U., Pandey, C. M., Mishra, P., & Pandey, G. (2019). Application of student’s t-test, analysis of variance, and covariance. Annals of Cardiac Anaesthesia, 22(4), 407. https://doi.org/10.4103/aca.ACA_94_19
- Mizik, T. (2021). Climate-smart agriculture on small-scale farms: A systematic literature review. Agronomy, 11(6), 1096. https://doi.org/10.3390/agronomy11061096
- Murry, J. W., Jr., & Hammons, J. O. (1995). Assessing the managerial and leadership ability of community college administrative personnel. Community College Journal of Research and Practice, 19(3), 207–216. https://doi.org/10.1080/1066892950190303
- Nassary, E. K., Baijukya, F., & Ndakidemi, P. A. (2020). Sustainable intensification of grain legumes optimizes food security on smallholder farms in sub-Saharan Africa – a review. International Journal of Agriculture and Biology, 23(1), 25–41. https://doi.org/10.17957/IJAB/15.1254
- Obalum, S. E., Buri, M. M., Nwite, J. C., Watanabe, Y., Igwe, C. A., & Wakatsuki, T. (2012). Soil degradation-induced decline in productivity of sub-Saharan African soils: The prospects of looking downwards the lowlands with the Sawah ecotechnology. Applied and Environmental Soil Science, 2012. https://doi.org/10.1155/2012/673926
- Ochieng, J., Afari-Sefa, V., Muthoni, F., Kansiime, M., Hoeschle-Zeledon, I., Bekunda, M., & Thomas, D. (2022). Adoption of sustainable agricultural technologies for vegetable production in rural Tanzania: Trade-offs, complementarities and diffusion. International Journal of Agricultural Sustainability, 20(4), 478–496. https://doi.org/10.1080/14735903.2021.1943235
- Ogunyiola, A., Gardezi, M., & Vij, S. (2022). Smallholder farmers’ engagement with climate smart agriculture in Africa: Role of local knowledge and upscaling. Climate Policy, 22(4), 411–426. https://doi.org/10.1080/14693062.2021.2023451
- Owens, J. S., Cox, H., deVoil, P., Power, B., Conway, M., & Routley, R. (2009, July). APSFarm: A new framework for modelling mixed cropping and grazing farm businesses. In Presentation at 18th World IMACS/MODSIM Congress, Cairns, Australia (pp. 13–17).
- Partey, S. T., Zougmoré, R. B., Ouédraogo, M., & Campbell, B. M. (2018). Developing climate-smart agriculture to face climate variability in West Africa: Challenges and lessons learnt. Journal of Cleaner Production, 187, 285–295. https://doi.org/10.1016/j.jclepro.2018.03.199
- Rao, A. S., Lenka, N. K., Biswas, A. K., & Ramesh, K. (2015). Soil health enhancement. Indian Journal of Fertilisers, 11(4), 28–37.
- Saaty, T. L. (1977). A scaling method for priorities in hierarchical structures. Journal of Mathematical Psychology, 15(3), 234–281. https://doi.org/10.1016/0022-2496(77)90033-5
- Saaty, T. L. (1990). An exposition of the AHP in reply to the paper “remarks on the analytic hierarchy process”. Management Science, 36(3), 259–268. https://doi.org/10.1287/mnsc.36.3.259
- Saaty, T. L. (2004). Decision making – the analytic hierarchy and network processes (AHP/ANP). Journal of Systems Science and Systems Engineering, 13(1), 1–35. https://doi.org/10.1007/s11518-006-0151-5
- Saaty, T. L. (2008). Decision making with the analytic hierarchy process. International Journal of Services Sciences, 1(1), 83–98. https://doi.org/10.1504/IJSSCI.2008.017590
- Saaty, T. L., & Özdemir, M. S. (2014). How many judges should there be in a group? Annals of Data Science, 1(3–4), 359–368. https://doi.org/10.1007/s40745-014-0026-4
- Sanou, J., Bationo, B. A., Barry, S., Nabie, L. D., Bayala, J., & Zougmore, R. (2016). Combining soil fertilization, cropping systems and improved varieties to minimize climate risks on farming productivity in northern region of Burkina Faso. Agriculture & Food Security, 5(1), 1–12. https://doi.org/10.1186/s40066-016-0067-3
- Sapkota, A., Haghverdi, A., Avila, C. C., & Ying, S. C. (2020). Irrigation and greenhouse gas emissions: A review of field-based studies. Soil Systems, 4(2), 20. https://doi.org/10.3390/soilsystems4020020
- Segnon, A. C., Achigan-Dako, E. G., Gaoue, O. G., & Ahanchédé, A. (2015). Farmer’s knowledge and perception of diversified farming systems in sub-humid and semi-arid areas in Benin. Sustainability, 7(6), 6573–6592. https://doi.org/10.3390/su7066573
- Serdeczny, O., Adams, S., Baarsch, F., Coumou, D., Robinson, A., Hare, W., Schaeffer, M., Perrette, M., & Reinhardt, J. (2017). Climate change impacts in sub-Saharan Africa: From physical changes to their social repercussions. Regional Environmental Change, 17(6), 1585–1600. https://doi.org/10.1007/s10113-015-0910-2
- Seyoum, A., Nega, A., Wagaw, K., Tadesse, T., Tadesse, D., Tirfessa, A., Amare, S., Amare, N., Kedanemaryam, W., Taye, T., Diriba, T., Alemu, T., Habte, N., Adane, G., Sewmehone, S., Tsegaye, G., Chalachew, E., Hailemariam, S., Tamirat, B., … Bogale, M. (2020). Multi environment and spatial analysis of early maturing sorghum [Sorghum bicolor (L.) Moench] genotypes in dry lowland areas of Ethiopia. African Journal of Agricultural Research, 15(2), 278–290. https://doi.org/10.5897/AJAR2019.14495
- Shiwakoti, S., Zheljazkov, V. D., Gollany, H. T., Kleber, M., Xing, B., & Astatkie, T. (2019). Micronutrients in the soil and wheat: Impact of 84 years of organic or synthetic fertilization and crop residue management. Agronomy, 9(8), 464. https://doi.org/10.3390/agronomy9080464
- Shrestha, S., & Dhakal, S. (2019). An assessment of potential synergies and trade-offs between climate mitigation and adaptation policies of Nepal. Journal of Environmental Management, 235, 535–545. https://doi.org/10.1016/j.jenvman.2019.01.035
- Silberg, T. R., Richardson, R. B., & Lopez, M. C. (2020). Maize farmer preferences for intercropping systems to reduce Striga in Malawi. Food Security, 12(2), 269–283. https://doi.org/10.1007/s12571-020-01013-2
- Solanki, M. K., Wang, F. Y., Li, C. N., Wang, Z., Lan, T. J., Singh, R. K., Yang, L.-T., & Li, Y. R. (2020). Impact of sugarcane–legume intercropping on diazotrophic microbiome. Sugar Tech, 22(1), 52–64. https://doi.org/10.1007/s12355-019-00755-4
- Swart, R. J. (2009). Climate change versus development: Trade-offs and synergies. Policy Network.
- Teklewold, H., Mekonnen, A., Gebrehiwot, T., & Bezabih, M. (2020). Open access post-harvest grazing and farmers’ preferences for forage production incentives in Ethiopia. Land Use Policy, 96, Article 104685. https://doi.org/10.1016/j.landusepol.2020.104685
- Tongwane, M. I., & Moeletsi, M. E. (2018). A review of greenhouse gas emissions from the agriculture sector in Africa. Agricultural Systems, 166, 124–134. https://doi.org/10.1016/j.agsy.2018.08.011
- Toth, G. G., Nair, P. R., Duffy, C. P., & Franzel, S. C. (2017). Constraints to the adoption of fodder tree technology in Malawi. Sustainability Science, 12(5), 641–656. https://doi.org/10.1007/s11625-017-0460-2
- Totin, E., Segnon, A. C., Schut, M., Affognon, H., Zougmoré, R. B., Rosenstock, T., & Thornton, P. K. (2018). Institutional perspectives of climate-smart agriculture: A systematic literature review. Sustainability, 10(6), 1990. https://doi.org/10.3390/su10061990
- UNEP. (2011). State of the environment report.
- Valarmathi, M., Muthukumar, M., Rahman, H., & Sasikala, R. (2019). Development of early maturing, high yielding, drought tolerant rice variety with superior grain quality through molecular breeding and its performance evaluation. Journal of Pharmacognosy and Phytochemistry, 8(2), 338–346.
- Valbuena, D., Tui, S. H. K., Erenstein, O., Teufel, N., Duncan, A., Abdoulaye, T., Swain, B., Mekonnen, K., Germaine, I., & Gérard, B. (2015). Identifying determinants, pressures and trade-offs of crop residue use in mixed smallholder farms in sub-Saharan Africa and South Asia. Agricultural Systems, 134, 107–118. https://doi.org/10.1016/j.agsy.2014.05.013
- Van Oort, P. A., & Zwart, S. J. (2018). Impacts of climate change on rice production in Africa and causes of simulated yield changes. Global Change Biology, 24(3), 1029–1045. https://doi.org/10.1111/gcb.13967
- Vom Brocke, K., Kondombo, C. P., Guillet, M., Kaboré, R., Sidibé, A., Temple, L., & Trouche, G. (2020). Impact of participatory sorghum breeding in Burkina Faso. Agricultural Systems, 180, Article 102775. https://doi.org/10.1016/j.agsy.2019.102775
- Waaswa, A., Oywaya Nkurumwa, A., Mwangi Kibe, A., & Ngeno Kipkemoi, J. (2022). Climate-smart agriculture and potato production in Kenya: Review of the determinants of practice. Climate and Development, 14(1), 75–90. https://doi.org/10.1080/17565529.2021.1885336
- Westcott, R. A. (2019, April 29–May 3). Farms, fire and fuels: Exploring the relationship between cropland fires and modern farming. Proceedings for the 6th International Fire Behavior and Fuels Conference, Sydney, NSW, Australia.
- Zakaria, A., Alhassan, S. I., Kuwornu, J. K., Azumah, S. B., & Derkyi, M. A. (2020). Factors influencing the adoption of climate-smart agricultural technologies among rice farmers in northern Ghana. Earth Systems and Environment, 4(1), 257–271. https://doi.org/10.1007/s41748-020-00146-w
- Zaveri, E., & Lobell, D. B. (2019). The role of irrigation in changing wheat yields and heat sensitivity in India. Nature Communications, 10(1), 1–7. https://doi.org/10.1038/s41467-019-12183-9
- Zhao, J., Yang, Y., Zhang, K., Jeong, J., Zeng, Z., & Zang, H. (2020). Does crop rotation yield more in China? A meta-analysis. Field Crops Research, 245, Article 107659. https://doi.org/10.1016/j.fcr.2019.107659
- Zou, X., Li, Y. E., Li, K., Cremades, R., Gao, Q., Wan, Y., & Qin, X. (2015). Greenhouse gas emissions from agricultural irrigation in China. Mitigation and Adaptation Strategies for Global Change, 20(2), 295–315. https://doi.org/10.1007/s11027-013-9492-9
Appendix
Table A1. List of CSA practices adopted in Ghana.