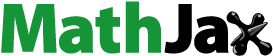
Abstract
This study investigates the effect of the black level and color gamut on the overall display brightness using a psychophysical experiment. Two stimuli with different color gamut were matched until the overall brightness appeared the same by controlling the peak display luminance. Three experimental sessions were conducted with varying black levels and color gamut. The results indicate that the effect of the color gamut on perceived brightness was significant, unlike that of the black level. The matched luminance increased by 6% to 8% as the color gamut widened from sRGB to DCI-P3. The equivalent luminance prediction models were tested using the average stimuli luminance, exhibiting poor performances.
1. Introduction
Although the peak luminance is the same, the perceived display brightness varies depending on the different display color characteristics. The authors’ previous study compared the perceived brightness of two displays with similar peak luminance but different black levels and color gamut [Citation1]. The results revealed that the black level and color gamut affect the overall image brightness.
The black level affects the perceived brightness due to the simultaneous contrast effect. This effect is a color appearance phenomenon in which brightness is perceived differently depending on the background luminance. For example, a gray patch on a black background is perceived as brighter than a gray patch on a white background. Mantiuk et al. studied the relationship between the surrounding luminance and the stimuli’s brightness of a display [Citation2]. They determined that the perceptually absolute black level, perceived as the darkest black, changed depending on the surrounding luminance. The absolute black level increased with the surrounding luminance. Baek et al. investigated the effect of the black level in complex images. They showed that people perceive an image with a lower black level as brighter as the dark area of an image increases [Citation3].
The color gamut is related to the Helmholtz-Kohlrauch (H-K) effect, which refers to the increased brightness for colors with the same luminance but a higher chroma. As the color gamut expressible by the display widens, the H-K effect becomes a more important issue for display brightness. Previous studies have explored the H-K effect using various displays [Citation4]. For instance, in the cathode-ray tube (CRT) display, the brightness-matching experiment was conducted with red, green, blue, cyan, magenta, and yellow patches by adjusting the luminance of gray color patches to have the same brightness [Citation5]. Kim et al. [Citation6] and Park [Citation7] examined the lightness in an organic light-emitting diode (OLED) display, liquid-crystal display (LCD), and quantum dot (QD) display using magnitude estimation. Oh et al. demonstrated the H-K effect in complex images with a wide color gamut LCD [Citation8]. Shizume et al. and Nakagawa et al. showed the H-K effect on the brightness of natural images reflecting the H-K effect [Citation9,Citation10].
The previous studies imply that making a display appear brighter is possible by controlling the black level and color gamut without increasing the peak luminance. Therefore, this study investigated the relationship between the display peak luminance, black level, and color gamut size to achieve the same display image brightness to identify how much the display peak luminance can be changed by black level and color gamut change while maintaining a similar display brightness.
During the experiments, two images, reference and test, with different black levels and color gamut, were shown side-by-side. The observers were asked to control the peak luminance of the test images until they showed a similar image brightness. There were three sessions, and the black level and color gamut of the images were set differently for each session.
2. Brightness matching experiment by controlling peak luminance
2.1. Experimental setting
A 30-inch OLED display (SONY BVM X-300) with a resolution of 3840 × 2160 was used in the experiment. Since the peak luminance of the OLED display is affected by the average pixel level (APL), the luminance of the peak white was measured by changing the color patch size. Figure shows that the luminance decreased when the white patch was larger than 50% of the display size. Therefore, in this study, the total stimulus size was adjusted to be 50% of the total screen area to maintain the same peak luminance regardless of the image contents.
Figure shows the monitor characteristics: the color gamut in CIE xy space and tone curve. The display gamma was 2.4, and the color gamut was similar to that of DCI-P3.
Figure illustrates an example of experimental stimuli. The images on the left and right represent the reference and test stimuli, respectively. Each image size was 25% of the total display area, corresponding to 960 × 540 pixels. The display was covered with black paper, except for the stimulus area, to minimize the effect of the black background and separate the two stimuli similar to different displays.
2.2. Stimulus
Complex images were used as stimuli. All stimuli were categorized into three images: overall dark, colorful, and overall bright. Figure exemplifies each type.
Figure 4. Sample images of different stimulus: (a) overall dark, (b) colorful image, and (c) overall bright.
The experiment involved three sessions, each with a different number of stimuli. Six stimuli overlapped in all sessions.
Table summarizes the stimulus sets. The black level and color gamut of the test stimulus were set to zero and sRGB, respectively. The black level and color gamut settings for reference stimuli were changed for each session. Only the peak luminance of the reference was fixed at 414.14 cd/m2. All the settings were simulated using the gain-offset-gamma (GOG) model.
Table 1. Black level and color gamut stimuli settings in each session.
In Session 1, the black level was changed in eight steps from 0.003–0.5 cd/m2, and the color gamut was unchanged in the reference and test stimuli. Sessions 2 and 3 were conducted using different black levels and color gamut settings. Session 3 included Session 2 stimuli with two additional colorful images. Figure presents the measured color gamut in Session 3, in which Gamut 2 and 3 denote sRGB and DCI-P3, respectively. Gamut 1 was designed for this experiment to simulate the smaller color gamut than sRGB. The chromaticity of the Gamut1 primary was on the line connecting the DCI-P3 primary and D65 white, which is the blue line in Figure . Gamut1 covers 76% of sRGB and 54% of DCI-P3.
Figure shows the image manipulation process, which involves simulating the stimulus to have the color gamut and black level specified in Table . First, the digital RGB values of the original image are converted to linear RGB after dividing the digital RGB values by 255 and applying a 2.4 gamma (i.e. ). Next, the tristimulus values XYZ were calculated from the linear RGB using a 3 × 3 matrix, which represents the target color gamut. The XYZ value of the black level was added to the original XYZ values to obtain the target XYZ. The inverse process was applied to the target XYZ values to generate the stimulus. The target XYZ values were transformed back to linear RGB using a 3 × 3 matrix based on the display color gamut. Finally, the linear RGB was converted to the digital RGB using the inverse display gamma function to obtain the experimental stimulus.
The performance of the image manipulation was evaluated by comparing the predicted and measured values of CIELAB color difference. The result showed that the average difference was 0.8 ΔE*ab.
2.3. Experimental method
The brightness-matching experiment was conducted in a dark room. Observers sat in front of the display. The distance between observers and the display was approximately 1 m.
The observers adjusted the peak luminance of the test stimuli until the reference and test stimuli had the same overall perceived brightness. The starting peak luminance of the test stimulus was randomly set between 350 cd/m2 to 450 cd/m2 for each stimulus. The stimuli were shown randomly. The peak luminance of the test stimulus can be controlled using the arrow keys on the keyboard. The experiment set was repeated twice in Session 1 and thrice in Sessions 2 and 3. The matching task has no time limit. The next stimulus was immediately presented when the matching stimulus was completed.
Ten observers participated in Sessions 1 and 3, and five participated in Session 2. All observers had normal color vision and were in their 20s or 30s. Different participants observed each session.
3. Experiment results
The matched peak luminance of all the observers was averaged for analysis. , , and show each session’s average peak luminance results for each session, where the x-axis indicates the stimulus number, and the y-axis indicates the average peak luminance. The average peak luminance above the reference line means that the reference stimulus appears brighter than the test stimuli at the same peak luminance.
According to Session 1’s results (Figure ), the average peak luminance has no significant difference by the black level, except for the first stimulus.
The matched peak luminance of the first stimulus, an image of a star floating on a dark night, decreases as the black level increases, indicating that the lower black level makes the peak white less bright. However, other images did not show such trend. For example, Figure compares the images 1 and 2 results. Even though both images have the similar characteristics, image 2 did not show the systematic matching luminance change by the black level. Thus, the image dependency shown in Baek et al.’s study [Citation3] was not applicable in our study. Specifically, this means the overall brightness change by the black level does not directly correspond to the peak luminance change.
Unlike Session 1, all average peak luminance values were above the reference luminance because of the effect of the color gamut in Session 2 (Figure ). The stimulus with DCI-P3 color gamut was perceived as brighter than the sRGB color gamut regardless of the black level and image category. In particular, Image 4, the first image in Figure (b), has the highest peak luminance because it mostly contains a highly saturated red region.
Session 3 results suggest that the peak luminance increases as the color gamut widens (Figure ), regardless of the black level confirming Session 1 and Session 2 results. The ‘colorful images’ exhibit the largest peak luminance increment (Gamut 3) or decrement (Gamut 1), while ‘overall dark images’ and ‘overall brightness images’ demonstrate smaller matching differences. This effect implies that Helmholtz-Kohlrauch (H-K) effect, the phenomenon that colorful colors look brighter than desaturated colors with the same luminance, affected the experiment’s result.
Table details the matched peak luminance of each gamut in Session 3. When the color gamut changed from Gamut 1 to sRGB and sRGB to DCI-P3, the matched peak luminance increased by 6% and 8%.
Table 2. Matching peak luminance (cd/m2) of each gamut in Session 3.
4. Equivalent luminance prediction model test (Helmholtz-kohlrauch effect)
According to the experiment results, the effect of the black level and image category on the matching peak luminance is insignificant. The impact of the color gamut on the brightness was confirmed in Session 2 and 3 results, where the Helmholtz-Kohlrausch effect exists on complex images. The equivalent luminance prediction models considering the H-K effect were tested using the results of Sessions 2 and 3.
The prediction models used were the CIE 200 and variable achromatic color (VAC) method developed by Nayatani [Citation11,Citation12]. CIE200 was proposed by the International Commission on Illumination (CIE) [Citation13,Citation14] to predict the brightness of colored objects (or light), including the mesopic vision range. Equivalent luminance, the luminance of specified reference light with the same brightness, can be obtained using both prediction models.
4.1. Test methods
The equivalent luminance of each pixel was obtained to test the experimental results. Figure illustrates a simple scheme of the test process. First, the digital RGB values of all pixels were converted to tristimulus values (XYZ) using the display characterization model. The matched peak luminance was applied as the peak luminance of the test stimuli, and the reference stimuli was fixed at 414cd/m2. Second, the equivalent luminance of all pixels was obtained using two existing prediction models. Third, the difference between the equivalent luminance of the reference and test, ΔYeq, was calculated from the average equivalent luminance obtained. If the equivalent luminance difference, ΔYeq, is near zero, the model can predict the H-K effect.
4.2. Test results
The performance of the VAC and CIE200 models was evaluated by calculating how much the equivalent luminance difference is reduced after applying the model compared to the original average luminance difference between the reference and the matched test image. Figure shows the result. The equivalent luminance difference calculated by the two models remained almost the same compared with the original luminance data, implying that the existing models fail to predict our experimental data.
Figure 12. The performance of the VAC and CIE200 models: (a) Session 2, (b) Session 3, Gamut 1, and (c) Session 3, Gamut 3.
The low performance of the existing models may be caused by the different experimental conditions. Both models were developed based on the brightness-matching experimental data with lower luminance, and the simple color patch was used instead of the complex image used for this experiment. Therefore, the perceived brightness models must be further improved to predict perceived brightness on wider color gamut displays.
5. Conclusions
This study assessed the effect of the black level, color gamut, and display peak luminance on the perceived brightness of an image. The display image’s brightness-matching experiment was conducted using two images with different black levels and color gamut sizes shown side-by-side. The observers were asked to adjust the peak luminance of the test stimuli until the reference stimuli had the same overall perceived brightness. The experimental results expressed that the observers set the higher luminance for the test stimuli when the reference stimuli had the larger color gamut, while the black level did not affect the matching results. The matched luminance increased by 6% to 8% as the color gamut widened from sRGB to DCI-P3. This result demonstrated that the display’s color gamut increase makes the display more vivid and brighter because of the H-K effect.
The equivalent luminance prediction models were tested, and the test results suggested poor performances. This means the existing models have low accuracy in predicting the H-K effect in complex images.
Consequently, color gamut effects should be considered when assessing the display brightness. Moreover, a brightness model that can be applied to displays with a wider color gamut must be developed. In addition, because the difference in the image contents also affects the brightness, image dependency should be considered.
Disclosure statement
No potential conflict of interest was reported by the author(s).
Additional information
Funding
Notes on contributors

Garam Seong
Garam Seong received her B.S. (electrical engineering) and M.S. (human factors engineering) in 2018 and 2020 from the Ulsan National Institute of Science and Technology (UNIST), Ulsan, South Korea. She is a PhD student at UNIST. Her main research interests include displays’ color appearance and image quality.

Youngshin Kwak
Youngshin Kwak received her B.S. (physics) and M.S. (physics) degrees in 1995 and 1997 from Ewha Women’s University, Seoul, South Korea. After completing her Ph.D. at the Colour & Imaging Institute, University of Derby, UK, in July 2003, she worked for Samsung Electronics South Korea. In February 2009, she became a professor at the Ulsan National Institute of Science and Technology (UNIST), South Korea. Her main research interests include color appearance, color emotion, and the quality of 2D and 3D images.

Hyosun Kim
Hyosun Kim received her B.S. degree in Psychology and M.S. and Ph.D. degrees in Cognitive Science from Yonsei University in 1997, 2003, and 2012, respectively. From 2003 to 2007, she was a Research Assistant with the Institute of Cognitive Science in Yonsei University, Seoul, South Korea. She is currently with Samsung Display, Yongin, South Korea. Her research interests include human perception and eye fatigue.
References
- G. Seong, Y. Lee, and Y. Kwak, Presented at Electronic Imaging 2021 (16), 326–1 (2021).
- R. Mantiuk, S. Daly, and L. Kerofsky, Presented at Human Vision and Electronic Imaging XV 2010, SPIE 7527, 264–271 (2010).
- Y.S. Baek, Y. Kwak, and S. Park, CIC 2019 (1), 104–107 (2019).
- R.L. Donofrio, J. Soc. Inf. Disp 19 (10), 658–664 (2011).
- J.M. Sanchez, and M.D. Fairchild, Presented at the AIC 2002, SPIE 4421, 607–610 (2002).
- M. Kim, J.-H. Jo, Y. Park, and S.-W. Lee, Displays 56, 1–10 (2019).
- Y. Park, J. Inf. Disp 23 (3), 235–242 (2022).
- S. Oh, and Y. Kwak, Presented at the Color Imaging XX: Displaying, Processing, Hardcopy, and Applications, SPIE 9395, 244–249 (2015).
- T. Shizume, G. Ohashi, H. Takamatsu, and Y. Shimodaira, J. Soc. Inf. Disp 22 (11), 588–596 (2014).
- K. Nakagawa, Y. Hayami, H. Aoyanagi, H. Takamatsu, Y. Shimodaira, and G. Ohashi, J. Soc. Inf. Disp 29 (6), 476 (2021).
- Y. Nayatani, C. Tanaka, N. Tanaka, and J. Light, Visual Env 22 (2), 48 (1998).
- Y. Nayatani, Color Res. Appl 22 (6), 385–401 (1997).
- International Commission on Illumination. CIE Supplementary System of Photometry. Commission Internationale de l’Eclairage, CIE Central Bureau, (2011).
- K. Sagawa, Ophthalmic Physiol. Optics 26 (3), 240–245 (2006).