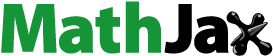
Abstract
Thin-film-transistor-liquid crystal displays (TFT-LCDs) still dominate most of the display markets due to a big leap in image quality while keeping low cost and superior reliability, and its evolution is continuing to compete with emerging emissive displays. In this paper, recent progress in liquid crystal technologies mainly focusing on fringe-field switching (FFS) and polymer-stabilized vertical alignment (PS-VA) modes and materials for improving electro-optic performances is reviewed.
1. Introduction
Since the first commercialization of TFT-LCDs for notebook displays in the early 1990s, it dominated most display application markets over three decades, even replacing plasma-display panels in the TV market. Liquid crystals (LCs) are non-emissive and viscous materials; nevertheless achieving such histories was possible, owing to many breakthroughs in display materials and components as well as wide-viewing-angle LC modes such as FFS (also called in-plane switching (IPS)) and PS-VA modes [Citation1–5]. Since about 2010, high-resolution displays like FHD TVs and retina displays (326 ppi or higher in mobile phones) in portable displays and monitors while showing excellent image quality in all viewing directions have become standard, and they were only flat panel displays in markets. One major drawback of LCDs is difficult to have a free form, especially since, foldable display is almost impossible because the thickness of the LC layer should be kept to display proper image quality. As an alternative, active matrix-driven organic light-emitting diodes (OLEDs) with plastic substrate appeared around the mid-2010s and have become a major display in high-end mobile phones nowadays [Citation6,Citation7]. Further, OLEDs are competing with LCDs in high-end TV markets and are expected to penetrate more into markets for gaming monitors and even notebooks [Citation8,Citation9].
The evolution of TFT-LCDs continues to keep its market position with enhanced electro-optic performances and compete with emissive displays like OLEDs, quantum-dot (QD) OLEDs, and micro-LEDs. For example, mini-LED, which can control an incident light intensity locally to LCD panel reflecting a displayed image of LCDs, and QD film, which can enhance the color gamut of LCDs are applied for high-end LCDs, such that recently commercialized iPad Pro has features: 1,000,000:1 contrast ratio, 1000 nits of full-screen brightness and 1600 nits of peak brightness along with advanced display technologies like P3 wide color and adaptive 120 Hz (24 Hz) refresh rate for videos (texts) [Citation10]. More recently, TFT-LCDs with 8 K resolution with 120 Hz refresh rate are commercialized [Citation11], but higher resolution and higher frame rates are under development such that 110′′ TFT-LCDs with 16 K high resolution and 600 Hz refresh rate to ensure no image trailing are reported [Citation12,Citation13]. On the other hand, TFT-LCD with a 1 Hz refresh rate is developed to minimize power consumption in a text mode [Citation14,Citation15].
Such an increase in resolution reduces pixel size, rendering control of the LC director in a voltage-on state more difficult. Consequently, it results in very poor transmittance. In displays, light efficiency reflects the cost of the products so many approaches to improving the transmittance in LCDs were reported. On the other hand, 120 Hz in video mode and 600 Hz in gaming require LC’s response time to be much faster than conventional displays so developments of low-viscosity LC materials and optimization of cell parameters were performed. 1 Hz driving in text mode raises flexoelectric coupling in the FFS mode but recent progress overcomes such problems. Besides research for display products, ferroelectric nematic LCs in which all dipoles point in the same direction and thus yield a very high dielectric anisotropy are hot materials recently because the LCDs with such materials can be driven with ultra-low field as low as 102 V/m unlike 106 V/m in conventional LCDs with nematic phase [Citation16]. In this paper, recent progress on those topics is briefly reviewed.
2. Recent progress of LC technologies
2.1. LC devices
The first commercialized TFT-LCDs in the early 90 s adopted twisted nematic (TN) mode with the highest polarization conversion efficiency at low operating voltage and strong viewing angle dependency in image quality. The film-compensated TN mode is still in production, especially for low-end monitors. Most high-end small and medium-sized LCDs with high resolution and high image quality in all viewing directions utilize FFS mode because the FFS mode can yield high transmittance for small-sized pixel structure and is suitable for touch panels without push mura while exhibiting wide-viewing angle, low operating voltage, a good response time and proper contrast ratio. For gaming monitors, the fast response time of LCs is required to meet high refresh rates like 240 Hz or 600 Hz, and some users favor curved displays to enhance immersiveness. LCDs with VA mode are advantageous to realize curved display with a smaller radius of curvature than that of the FFS mode, while the FFS mode gives rise to better image quality, so both FFS and PS-VA modes coexist. For TV applications, two homogenous alignment modes (IPS produced by LG Displays only), FFS (BOE Technology Group Co. named it ADS) and two VA modes (PS-VA, UV alignment called UV2A) exist in the present market, though FFS and PS-VA modes have most market shares.
2.2. LC materials
In FFS mode, both positive (+LC) and negative (-LC) dielectric anisotropic LC are utilized depending on application fields. In general, rotational viscosity of +LC is lower than that of –LC, –LC gives rise to higher transmittance and lower operating voltage than those of +LC for the same magnitude of dielectric anisotropy, average elastic constant of –LC is larger than that of +LC, resistivity of +LC at high temperature is higher than that of –LC, and controlling LC mixtures for getting high nematic to isotropic transition temperature and magnitude of dielectric anisotropy is more flexible in +LC than –LC. Recently, display resolution in TVs has increased from 4 K to 8 K and 16 K, and there is a worldwide strong demand to decrease power consumption in electronics to achieve about a 30% decrease in energy consumption by 2030. To meet these requirements, -LC, which exhibits about higher transmittance of about 15% than +LC is favored [Citation17]. However, the displays need to exhibit a high contrast ratio (CR), fast response time, and high reliability so extensive work on finding new single components and mixtures for -LC has been performed recently, and Merck Display Materials Co. reported specification of -LC mixture for TV/contrast ratio, IT, and fast response time comparing those with +LC mixture. One of the great advantages of designing LC mixtures in the FFS mode compared to conventional IPS mode is that LC with a low magnitude of dielectric anisotropy (Δε) can be used because of the strong field generated in the voltage-on state. As indicated in Table , +LC (mixture A) has Δε = 2.7, so its rotational viscosity (γ) is 65 while -LC mixture with Δε = −3.9 (B), −4.0 (C,D) has γ = 134, 147, 149, respectively. On the other hand, the magnitude of elastic constants like splay (K11), bend (K33), and average K (Kavg) of -LC is larger than those of +LC. Since LC is a viscoelastic material, its response time is proportional to the magnitude of viscoelastic value γ/K, that is, in case γ/K of mixture A is smaller than those of mixtures B, C, and D, indicating which +LC mixture is intrinsically advantageous for a fast response time. To achieve a comparable γ/K to +LC mixture, Merck developed new -LC mixtures, as indicated in Table , which can accelerate to use -LC mixtures in ultra-high-resolution TVs. For example, mixture J (-LC) has higher γ (81) than 65 of mixture A (+LC) but K11 (15.8) of -LC is higher than 13.1 of +LC such that γ/Ks for both mixtures become about the same, implying that -LC can exhibit fast response time like +LC. To enhance the response time, a super low viscosity (SLV) neutral LC single should be used and simultaneously the fraction of a high polar LC single must be followed, but the reliability of both SLV and the high polar single can be vulnerable to UV exposure. The higher the doping concentration of such neutral LC in the host LC mixture, the absolute value of Δε may decrease, γ decreases and so does the clearing point (Clp). One of evidence is that mixture I has a much larger rotational viscosity and the absolute value of Δε and higher Clp than mixture J [Citation18].
Table 1. Physical properties of LC mixtures for TV applications.
Table 2. Physical properties of LC mixtures for fast response time.
2.3. Contrast ratio and response time
In both IPS and PS-VA modes, the change of polarization state by LC layer is controlled by phase retardation change so that its normalized transmittance is governed by
(1)
(1) where Φ is the angle between the LC director and transmission axes of the crossed polarizers, d is the thickness of the LC layer, Δn is the birefringence of the LC layer, and λ is the wavelength of incident light. The same equation applies to both IPS and VA modes; however, the contrast ratio (CR) of VA mode exceeds 5000: 1 while it is about 2000: 1 in IPS mode. In PS-VA mode, the LC director is tilted to about 2o from the normal direction so that Δn is close to 0 so that T is close to 0, giving rise to an excellent dark state in a voltage-off state. This situation does not change much in the real manufacturing process. However, Φ is in the plane in IPS mode, so it could deviate from 0 due to manufacturing error in either the rubbing or photoalignment process. Also, there is always an error in matching the transmittance axis of the polarizer with the LC alignment direction during the polarizer-attaching process, resulting in non-zero of Δn, that is, light leakage in a black state is inevitable [Citation19]. Further, an error in the assembling process of top and bottom substrates could lead to twisting of the LC director, leading to light leakage again. Consequently, the IPS mode practically yields a lower CR than the VA mode in the normal direction. The FFS mode in a dark state faces the same situation as the IPS mode, but the full white state gives better transmittance than the IPS mode, implying LCDs with FFS mode could exhibit better CR than that with IPS mode.
Over the last decade, extensive studies on improving the CR of LCD panels have been performed. In all TFT-LCDs, several optical layers such as LC, color filters, black matrix, and optical films like protection film of polarizing film and compensated film to control polarization state at off-normal axis exist under two crossed polarizers. To get a perfect dark state, any optical layers should not affect the polarization state of an incident light such that polarizer film with a high degree of polarization, backlight sheet for collimating light, reduced pigment size in color filter, controlling taper angle of electrodes and improving the rubbing conditions for a better LC alignment was applied to get CR over 600: 1 about two decades ago [Citation20]. Besides, LC cell’s scattering index Scell, indicating depolarization level of an incident polarized light was defined as
(2)
(2) where d is the thickness of the LC layer [Citation21,Citation22]. According to eqn. (2), smaller Δn and d and large Kavg are favored to have smaller Scell, which can give rise to higher CR. Further, photoalignment of LCs was applied to reduce light leakage associated with misalignment of LC directors near electrodes and column spacers, and the size of the black matrix to block any light leakage was optimized. Nevertheless, CRs in IPS and FFS mode just exceeded 2000: 1 or below 2000:1 [Citation23,Citation24]. This year, BOE Co., Ltd. reported ADS TFT-LCD with more than 3000:1 for TVs, while claiming CR for most commercialized ADS TV and monitor LCDs were 1000–1200:1 [Citation25], and LG Display Co., Ltd. reported AH-IPS TFT-LCD with more than 2000:1 for monitors. According to the study, four parameters, such as SLC, fine LC alignment process, cell gap, and color filter are optimized to give high CR, high transmittance, and fast response time simultaneously. At first, a -LC mixture with low Δn and high K is chosen to minimize SLC. The magnitude of Δn is determined by electron cloud density and conjugate effect, so low-conjugated LCs are chosen for low Δn. On the other hand, the K value is decided by intermolecular interaction forces; hence, enhancing molecular rigidity and the aspect ratio of molecules is beneficial to increase the K value. The easiest way to increase K without knowing the details of LC structure is to adopt LCs with high Clp. In this way, SLC could be reduced, but increasing LC’s Clp will increase rotational viscosity at room temperature, resulting in a slow response time. Then am -LC mixture with low dielectric anisotropy, which yields low rotational viscosity, must be used. Consequently, an operating voltage (Vop) increases, but, unlike +LC in the FFS mode, transmittance change is small around Vop because both phase retardation and polarization rotation play the role of polarization change in a fully bright state [Citation4]. BOE claims that selecting a slightly lower voltage than Vop but comparable to conventional LC solves slowed decay response time, with minimal loss of transmittance. Secondly, they chose alignment layer polyimide (PI) with strong anchoring force and tuned process parameters such as its thickness and curing temperature and achieved a 14% improvement in CR. Further, the optic axis of the LC director should match one of the crossed polarizer axes to get Φ = 0, so that an ideal dark state can be achieved as indicated in eqn.1. They controlled the rubbing process to minimize twist angle from the bottom to top LC layers and claimed a reduction of the LC twist angle in the cell from 0.2° to 0.01°, consequently, giving rise to CR over 3000:1. In addition, single-roll rubbing was slightly better than double-roll one, yielding less twisted angle. Finally, a super high CR of 3535:1 was achieved on a 75′′ UHD TV ADS panel. Thirdly, when using -LC mixture with high Clp, a slight increase in viscosity and γ/K compared to that of +LC is inevitable because the LC device’s decay time (τd) purely depends on cell parameters like τd = γd2/K. Therefore, a reduction in d is required to get a fast response time and reduced Scell, and lowering d results in a transmittance decrease in the FFS mode [Citation26]. To compensate for the transmittance loss, BOE claimed they developed a new color filter that can show high transmittance and high color gamut and also optimized the backlight system with quantum-dot (QD) film to match the spectra of the color filter and light source more correctly, finally achieving the color gamut of DCI-P3 with 100% matching rate and high transmittance.
In LCDs, high CR, and color gamut in oblique viewing directions are required [Citation27–29]. Recently, local dimming mini-LEDs have been applied in high-end LCDs to achieve a CR over 1,000,000:1 in the normal direction. However, a light leakage in oblique viewing directions so-called halo mura is inevitable [Citation19]. In the case of LCDs with 3000:1, as abovementioned, light leakage in the dark state was 0.092 nit, but off-axis light leakage will be much higher than this. Therefore, adding an extra anisotropic absorption film that absorbs the light less than 1 nit and absorbs a few nits in the viewing direction will help to remove such halo mura. The idea was reported with an optical design, as highlighted in Figure , in which a dichroic dye is embedded in a polymerized mesogen, and the experimental results revealed a much improved dark state at the off-normal axis [Citation30]. The OLED TVs do not have such a halo mura, so LCDs must be equipped with such film to achieve an excellent dark state in all viewing angles.
Figure 1. Structures of high-end TFT-LCDs with local dimming mini-LEDs and QD films. (a) a homogeneously aligned FFS LC cell with no compensation film, and (b) a thin film containing dichroic dyes on top of a conventional polarizer. Macroscopic photographs comparing dark states surrounding a bright circle image when the dichroic film formed between two glass substrates exists above an uncompensated TV panel for (c) 1 wt% and (d) 2 wt% dye-doped films. Case (d) demonstrates excellent dark states surrounding the bright circle image in all viewing directions [Citation30].
![Figure 1. Structures of high-end TFT-LCDs with local dimming mini-LEDs and QD films. (a) a homogeneously aligned FFS LC cell with no compensation film, and (b) a thin film containing dichroic dyes on top of a conventional polarizer. Macroscopic photographs comparing dark states surrounding a bright circle image when the dichroic film formed between two glass substrates exists above an uncompensated TV panel for (c) 1 wt% and (d) 2 wt% dye-doped films. Case (d) demonstrates excellent dark states surrounding the bright circle image in all viewing directions [Citation30].](/cms/asset/75a1eaf5-5328-4894-bb9a-0efb7b670b28/tjid_a_2281224_f0001_oc.jpg)
2.4. 240–600 Hz refresh rate FFS-LCDs
Most normal LCDs, like notebooks and monitors, have a refresh rate (or driving frequency) of 60 Hz but it is increased to 120 Hz in TVs to improve moving picture quality. In 2016, oxide TFTs, which have 10 times faster mobility than a-Si, were adopted to laptops and monitors over 4 K. Since 2017, consumers of gaming displays have grown up, and a higher refresh rate than 120 Hz was required to display a perfect video, and the fundamental requirement of gaming displays was fast response of LCDs. In 2017, gaming laptops with a driving frequency of 144 Hz were launched with grey-to-grey (GTG) response time enhanced from 16 ms to 5 ms (with overdriving, 3 ms) [Citation31]. In 2018, a gaming monitor was launched with GTG response time enhanced from 12 ms to 4 ms (with overdriving, 1 ms). Such a fast response time could be achieved by developing low viscosity, high elastic constant LCs, and optimizing low cell gap. At the same time, monitors require at least 20% higher CR than laptops. In 2019, the gaming monitor display market grew and extended due to the pandemic. Higher driving frequency was achieved from 144 to 240 Hz and thus physical properties of +LC including higher elastic constant were enhanced to get higher CR. In 2020, the gaming markets extended more due to the improvement of graphic card performance. The driving frequency was increased from 240 to 480 Hz, and the resolution was enhanced from FHD to QHD [Citation32]. Enhancing from 240 to 480 Hz reduces gate-on time in TFT from 3.36 µs to 1.86 µs in WUXGA (1920 × 1200). More recently, LCDs with a driving frequency of 600 Hz (gate-on time is just 1.34 µs) were developed in 2023 because refresh rate has become one of the most important indicators of high-end display panels, especially for video game usage [Citation13]. In practice, it is very difficult to drive such an ultra-high refresh rate because of the low charging ratio to a pixel and also the requirements of LC’s GTG response time of 1 ms. As a solution, BOE adopted oxide TFTs and optimized mobility to get a high Ion of 19.08 µA to meet a charging ratio of more than 95% for 600 Hz LCDs. In the FFS mode, τd depends not only on γd2/K but also slit angle, which determines an angle (α) between the LC director and in-plane field (the larger the slit angle, the angle α becomes smaller) such that LCs with low d and small γ/K must be used with larger slit angle [Citation33]. According to the study, the slit angle increased from 5° to 20°, d was reduced from 2.4 µm to 1.4 µm while LC’s Δn was increased from 0.1168 to 0.1402 and also its Δε increased from 4.1 to 4.4, realizing GTG response time of 0.99 ms. However, an increase in the slit angle and decrease in d results in much-decreased transmittance, implying LC with higher Δn but small γ/K must be developed in the future.
2.5. Flexoelectric effects in nematic LCs
The last two decades have seen a significant evolution in LCD technology, with the advent of high refresh rates between 120 and 240 Hz playing a crucial role in enhancing video playback smoothness. This development coincides with the current media environment's increasing reliance on content such as text, static images, and short videos that typically require lower refresh rates. Consequently, LCDs capable of accommodating a wide range of refresh rates - from as low as 1 Hz to as high as 240 Hz – are predicted to be one of the highest needs in the market. This prediction aligns with an ongoing trend toward energy efficiency [Citation34,Citation35]. As part of this technological progression, low-refresh-rate technology at 24 Hz has been implemented in recently launched FFS LCDs. Yet, challenges arise when the refresh rate drops to 1 Hz: image quality significantly degrades and image flicker becomes noticeable at lower refresh rates due to uneven brightness across each AC frame. The primary cause for these issues is the flexoelectric effect seen in nematic LCs [Citation36–40].
More than 50 years ago, R.B. Meyer observed the flexoelectric effect in nematic LCs [Citation41]. When the frequency of the AC frame is about 60 Hz and above, LC molecules have no time to rotate and nematic invariance is maintained. On the other hand, when the frequency is about 30 Hz and below, LC molecules have sufficient time to rotate, and nematic symmetry becomes broken. Consequently, molecular asymmetric shape gives rise to geometric deformation as shown in Figure . At low frequencies, flexoelectric coupling occurs based on the interaction between applied electric field and flexoelectric polarization, and the phenomenon arises along with spatial and temporal optical appearance. The brightness difference between frames measured above approximately 55 dB can be determined as an optical flicker which would irritate human eyes.
Figure 2. LC molecular responses under different field frequencies (e.g. 60 Hz [left] and 1 Hz [right]). At 1 Hz, geometrical deformation can occur having flexoelectric polarization.
![Figure 2. LC molecular responses under different field frequencies (e.g. 60 Hz [left] and 1 Hz [right]). At 1 Hz, geometrical deformation can occur having flexoelectric polarization.](/cms/asset/2211a743-f9cd-42bb-a56e-5df8fc7e2b28/tjid_a_2281224_f0002_oc.jpg)
In response to these challenges, several strategies have been proposed. The first strategy addresses changes in the AC field by spatially and/or temporally modulating symmetric electric field formation that can reconstruct the asymmetric field formation in FFS LCDs caused by electrodes existing in uneven layers as shown in Figure . This can be accomplished through various methods, including adopting bipolar schemes [Citation37], altering electrode structures [Citation38,Citation42–44], or adjusting insulation layer thicknesses in FFS mode [Citation42].
Figure 3. Brightness difference between frames based on flexoelectric effect in an FFS electrode structure [Citation42].
![Figure 3. Brightness difference between frames based on flexoelectric effect in an FFS electrode structure [Citation42].](/cms/asset/d0871526-b3a3-4e7c-904e-e30acabec5f7/tjid_a_2281224_f0003_oc.jpg)
The second strategy seeks a more profound understanding of flexoelectric effects through observation and study of phenomena within simple in-plane switching cells and FFS cells [Citation14,Citation15,Citation39,Citation43], as emphasized in Figure . Depending on the constructive and destructive direction of flexoelectric polarization and electric polarity, the local brightness changes. When constructive, splay, or bend deformation gets strong, whereas when destructive, splay, or bend deformation gets weak, twist deformation would mostly cover the dielectric free energy.
Figure 4. Structural difference and comparison between FFS and IPS modes in which flexoelectric effect exists at low-frequency driving [Citation42].
![Figure 4. Structural difference and comparison between FFS and IPS modes in which flexoelectric effect exists at low-frequency driving [Citation42].](/cms/asset/3ee817b6-778d-42e2-8ce6-36f2a5a00f05/tjid_a_2281224_f0004_oc.jpg)
Lastly, the third strategy places on manipulating the physical properties of LCs through control over dielectric anisotropy and elastic constants or by adjusting flexoelectric coefficients via doping bent-core LCs [Citation44–46]. The flexoelectric polarization is defined as Pf = es n(∇·n) + eb n × (∇ × n), where es and eb are splays and bend flexoelectric coefficients. Based on statistical approaches, the flexoelectric coefficients can be determined as es = α (a/b)1/3N1/3(2µ∥︀K11/kBT) and eb = α (b/a)2/3N1/3(2µ⊥K33/2kBT) where a and b are the major and minor axes of molecules to consider an approximated molecular ellipsoid; α is the molecular-kink angle; µ, kB, T, and N are the dipole moments (µ∥︀ and µ⊥: dipole moments parallel and perpendicular to the long axis of molecules, respectively), Boltzmann constant, temperature, and molecular polarity-excess number (N = N+ - N-), respectively. More recently, the flexoelectric coefficients were controlled by mixing dipoles and quadrupoles in optimum concentration, in this case, the absolute value of flexoelectric coefficients is similar, the brightness difference between frames can be reduced, so does flickers, as shown in Figure [Citation14,Citation15].
Figure 5. Flexoelectric polarization of pear and banana-shaped molecules and the contribution of dipoles of -LC (bend) and quadrupoles (splay) to flexoelectric coefficients.

Despite being pursued independently, these strategies will need integration for effective application into real-world products. This necessitates extensive knowledge across multiple disciplines, such as theory construction, materials engineering, optics, and display technologies. Recently, Jeonbuk National University, LG Display, and Merck co-worked and developed the world’s first 1 Hz refresh rate flicker-free FFS LCD, which shows no flickers and power consumption is reduced by about 83% less than when the refresh rate is 60 Hz [Citation14,Citation15].
2.6. Optimization of pixel structure to maximize transmittance in the FFS mode and its application to large-sized and 16K high-Resolution LCDs
The FFS mode is well known for its suitability for high-resolution display because a storage capacitor (Cst) composed of two transparent electrodes is formed in a light-transmitted active pixel area, unlike conventional IPS and VA modes. The transmittance of LCDs is mainly determined by the transmittance of the polarizer and color filter, LC’s polarization change efficiency, and an aperture ratio. The aperture ratio can be maximized by well-controlling LC’s field response behavior near signal lines and followingly minimizing the width of the black matrix on the top substrate.
The breakthrough level of transmittance improvement by maximizing transmittance near data signal lines was made around the middle of the 2000s when Hydis Co. and Seiko Epson attemprted to apply the FFS mode to super high-resolution mobile LCDs. In the revolutionary pixel structure called H-FFS mode, the common electrode exists above the pixel electrode, unlike that in conventional FFS mode, and covers the data line to shield off any noise field coming from the data line so that the transmittance near the data line is maximized by removing LC’s disclination lines and minimizing the width of black matrix on top substrates, as indicated in Figure . The transmittance of the 2.0′′ QVGA HFFS-LCD was improved by more than 50% compared to that of the AFFS-LCD and was even higher than that of the TN-LCD, as depicted in Figure . To our knowledge, the product showed the best transmittance among all TFT-LCDs, which proved the FFS mode was the right one for high-resolution LCDs. In this structure, a relatively thick inorganic insulator was used between data lines and the common electrode, in which a relatively thick layer of about 1 µm was applied to minimize RC delay and capacitance coupling between signal lines and the common electrode. The HFFS structure becomes the foundation stone for presenting all high-resolution FFS-LCDs from small to large-sized displays, including 110′′ LCDs with 16 K resolution [Citation12].
Figure 6. Schematic comparison of pixel structures and simulated transmittance between AFFS and HFFS modes [Citation47].
![Figure 6. Schematic comparison of pixel structures and simulated transmittance between AFFS and HFFS modes [Citation47].](/cms/asset/0ab69603-084a-4806-a435-559efa6ddc5d/tjid_a_2281224_f0006_oc.jpg)
Figure 7. Comparison of voltage-dependent transmittance curves in high-resolution mobile TFT-LCDs. The HFFS mode shows much-improved transmittance, as also shown in the microscopic image of a pixel [Citation47].
![Figure 7. Comparison of voltage-dependent transmittance curves in high-resolution mobile TFT-LCDs. The HFFS mode shows much-improved transmittance, as also shown in the microscopic image of a pixel [Citation47].](/cms/asset/b8dd661c-8b44-42a2-aced-2a116c33f7eb/tjid_a_2281224_f0007_oc.jpg)
Since 2010, most high resolution and high image quality LCDs for mobiles and tablet PCs adopted the FFS mode such that the widely used twisted nematic (TN) mode, which cannot realize high-performance LCDs, was replaced by the FFS mode even in notebooks, and monitors with +LC in 2012. When the HFFS mode is used for super-high resolution FFS-LCD with a large size over 47′′ or higher, the electrical load becomes too high, which makes driving very difficult. Consequently, several companies, including Panasonic LCD Co., proposed a new electrode structure named IPS-Pro-Next in which an organic resin with a thickness of more than 2 µm was coated above data lines and the plane shape of common electrodes covered all signal lines to shield any noise electric field coming from TFT addressing, and then inorganic insulator is deposited above common electrode and finally, slit type of pixel electrode is formed, as displayed in Figure [Citation48,Citation49]. Here, the insulator between the data line and the common electrode is not indicated as an organic resin; however, large-sized LCDs with over 10′′ and super high resolution adopted the organic resin with a thickness of over 1 µm. Panasonic LCD Co. claimed that the high transmittance FFS-LCD can be realized even if the display resolution increases from full HD to 4K2K, as shown in Figure . For instance, the resolution is increased by 4 times but the width of the black matrix (BM) on the top substrate can be minimized with the proposed structure so that the aperture ratio keeps 70% which is about the same value as that of the full HD one. The proposed pixel structure opens a new paradigm such that while the resolution of LCDs increases by 4 times, the aperture ratio remains about constant.
Figure 8. Comparison of pixel structures between (a) IPS-Pro pixel and (b) IPS-Pro Next pixel [Citation48,Citation49].
![Figure 8. Comparison of pixel structures between (a) IPS-Pro pixel and (b) IPS-Pro Next pixel [Citation48,Citation49].](/cms/asset/d40eaddd-42e4-44ee-9020-87eb95cf885d/tjid_a_2281224_f0008_oc.jpg)
Figure 9. Comparison of pixels of 47′′ IPS-LCDs [Citation48].
![Figure 9. Comparison of pixels of 47′′ IPS-LCDs [Citation48].](/cms/asset/0191119d-892d-46a8-9ede-f7c343b65ee4/tjid_a_2281224_f0009_oc.jpg)
In 2023, BOE Co. reported 4K 65′′ LCDs with a transmittance of 6.4%, which is about 30% higher than that of the conventional one [Citation50]. Two cross-sectional regions along the gate and above data lines in the panel were revealed. The first ITO playing the role of the pixel electrode is formed at first. A passivation layer (PVX) and an organic film (ORG) are formed above the source (S)/drain (D) electrode and data line passivation while it is formed above the gate inorganic insulator (GI) above the gate line. Then, the second patterned ITO playing the role of the common electrode is formed above the PVX + ORG layer covering the data line, as shown in Figure . Very interestingly, full-tone (FT) ORG is used above the data line and gate line, while half-tone (HT) ORG is used in the pixel aperture area to form the height difference between two regions, that is, thick and thin ORG above the signal lines and in a pixel active area, respectively. This approach minimizes capacitance formed between the 2nd ITO and data line due to the thick layer and any noise field from the data line could be shielded such that light leakage coming from the noise field near the data and gate line can be avoided. Consequently, the width of the black matrix on the top substrate can be minimized, increasing in the aperture ratio by 14.5%. Further, -LC, slit angle, and low reflection polarizer are utilized to maximize the transmittance and thus the overall increasing ratio of transmittance by 30% is achieved. According to the report, the ORG with FT above the gate lines forms the post-spacer (PS) platform, combined with the laminated resin and then acts on the PS support structure after the substrates are assembled. One more noticeable thing is that the Cst of a pixel can be quite reduced in the proposed structure, which helps to solve a charging issue in the FFS panel.
Figure 10. Cross-sectional view of a pixel structure of BOE’s high transmittance ADS mode along data and gate line. The thickness of an organic (ORG) layer is controlled by a full-tone (FT) and half-tone (HT) mask [Citation50].
![Figure 10. Cross-sectional view of a pixel structure of BOE’s high transmittance ADS mode along data and gate line. The thickness of an organic (ORG) layer is controlled by a full-tone (FT) and half-tone (HT) mask [Citation50].](/cms/asset/d4e09915-a590-41b9-b5e8-299cadfd208d/tjid_a_2281224_f0010_oc.jpg)
Recently, BOE Co. reported high-resolution and large-sized LCDs such as 98′′ and 110′′ with 8K and 55′′ with 8K and claimed that 110 ‘8K and 110’ 4K products are in mass production. In 2023, they developed 110′′ 16 K LCDs [Citation12], in which a pixel structure utilizing ORG layer and -LC was adopted, IGZO oxide TFT was adopted to fulfill gate-on array (GOA) driving and source driving and design optimization reducing associated with splicing (stitching) mura was performed. Figure shows how the process route has evolved from conventional ADS to high transmittance ADS (HADS) utilizing the ORG layer. In ADS, 1st ITO for a common electrode is formed at first, and 2nd ITO for a pixel electrode is formed at last. In this case, thick passivation layers of SiOx/SiOx/SiNx exist between two ITO layers, resulting in relatively small Cst. Too much large Cst in a large-sized pixel is troublesome because it requires high on-current to charge the pixel fully. However, the pixel size becomes smaller as the resolution of displays increases, and then Cst becomes too small, giving rise to crosstalk, which is the case of ADS mode. To solve that issue, i-ADS pixel mode in which only passivation layer SiNx exists between two electrodes. The high value of Cst was achieved, but the transmittance 110′′ 16 K panel, even with -LC was less than 2%, which is too low to be competitive. Therefore, the ORG layer was adopted, but unlike the structure proposed by Panasonic Co., 1st ITO for a pixel is formed and 2nd ITO for a common electrode is formed above PVX. Owing to the existence of a thick ORG layer, the common electrode could cover signal lines, which blocks any noise field from signal lines. Consequently, an aperture is maximized owing to minimal BM size and a panel of more than 2% transmittance is obtained. BOE claims that the 27′′ UHD panel also adopted HADS + ORG pixel mode.
Figure 11. Evolution of process routes from ADS to i-ADS and high transmittance ADS (HADS) utilizing ORG layer. ADS requires two ITO layers and 1ITO and 2ITO represent first and second layers, respectively [Citation12].
![Figure 11. Evolution of process routes from ADS to i-ADS and high transmittance ADS (HADS) utilizing ORG layer. ADS requires two ITO layers and 1ITO and 2ITO represent first and second layers, respectively [Citation12].](/cms/asset/f0b6e097-d3fd-45e1-b1b6-2a25b7e302f5/tjid_a_2281224_f0011_oc.jpg)
2.7. Carbon nanotubes in LCDs as anti-static layer and UV polarizer
LCDs with IPS and FFS modes do not require transparent ITO electrodes on the inside of the top substrate such that once electrostatic charge is generated on the backside of the substrate by any means, there is no path of discharge because all materials on top substrates are composed of organic insulators. The electrostatic charge generates an electric field that disturbs LC orientation inside the substrate, that is, a dark state of LCD is disturbed. As a solution to solving the issue, ITO is deposited on the backside of the substrate; however, when a capacitive touch sensor is equipped inside an LCD panel the ITO is troublesome, which makes it malfunction. In such a case, a new transparent material with a proper surface resistance of 106 ∼ 108 Ω/□ must be used to play the role of both capacitive touch and permanent anti-static property. For this purpose, a conductive coating solution with carbon nanotubes (CNTs) is coated and then the film is formed [Citation51].
For photoalignment of LCs, irradiation of polarized UV is required and a wire-grid polarizer mask is mainly used for that purpose, although it is highly costive and its size is limited due to the nano-lithographic process in fabrication. CNTs can be quite straight rods if they are cut into a few hundred nanometers [Citation52] or if they are stretched along one direction [Citation53], which can show anisotropic light absorption in a broad range owing to the π plasmon-originated broad absorption spectrum. Therefore, either aligned or stretched CNTs in two dimensions could play the role of UV polarizer, which displays strong anisotropic optical activity at both visible and UV wavelengths. Two CNT films formed by aligning stretched CNT yarns [Citation54] or by aligning CNTs with a shear flow [Citation55,Citation56] were tested for UV polarizer of photo-aligning LC in IPS cells and comparable electro-optics to IPS cells with rubbed PI were successfully obtained.
2.8. Polyimide (PI)-less LCDs
All conventional and most of the present commercialized TFT-LCDs adopt a polyimide (PI) layer for an LC alignment either homogeneously or vertically. In production, PI is dissolved in solvent and then coated on top and bottom substrates with a thickness of about 100 nm. Then, it needs pre-baking to evaporate the solvent and post-baking to produce a thin solid film consecutively, which requires long processing time and electrical energy consumption.
In the case of PS-VA mode, a pretilt angle from vertical alignment must be formed to reduce field-on response time. Merck KGaA reported the structure of self-aligned vertical alignment (SA-VA) materials. The representative additive (5) for vertical alignment, which comprises a mesogenic core and a polar (anchor) group, such as an alcohol and its SA process, is described in Figure . In PI-less PS-VA mode, reactive mesogens (RMs) and the additive are mixed into an LC mixture. Once the mixture is filled into the panel, the SA-VA material diffuses on the inner surfaces of the substrate and spontaneously gives a vertical alignment on substrates without an alignment layer. Next, the voltage is applied to induce a pretilt angle, and then UV is irradiated to polymerize RMs on both surfaces, which can fix = vertical alignment and pretilt angle [Citation57,Citation58]. To our knowledge, it is commercialized, and, in some cases, the conventional PI layer is applied to one of two substrates, indicating SA-VA materials and process still require further improvement.
Figure 12. Typical structure of SA-VA additive (e.g. Compound 5) and SA-VA process. After LC filling, the polar (blackhead groups) interact with the substrates and give a vertical orientation. Under the application of a voltage and irradiation by UV light, the tilt is formed and fixed. The RMs polymerize to form a film at the substrate surfaces [Citation57].
![Figure 12. Typical structure of SA-VA additive (e.g. Compound 5) and SA-VA process. After LC filling, the polar (blackhead groups) interact with the substrates and give a vertical orientation. Under the application of a voltage and irradiation by UV light, the tilt is formed and fixed. The RMs polymerize to form a film at the substrate surfaces [Citation57].](/cms/asset/077ea3b3-354a-45f1-b0fd-29d3d5e0a228/tjid_a_2281224_f0012_oc.jpg)
PI-less homogeneous alignment and application to FFS/IPS/ADS mode are reported [Citation59–63]. According to Muzusaki et al in Sharp Co. in 2018, a mixture of LC material having a concentration of the additive about 1 wt% that induces the homogeneous alignment is injected into an empty FFS cell. Then, polarized UV light is exposed to the cell from the normal direction of the substrate having the electrodes to induce the homogeneous alignment with the preparation of the SA layer (SAL). During the exposure of the polarized UV light, the temperature of the cell should be adjusted above the nematic-to-isotropic transition temperature of the LC material because the LC layer depolarizes the polarized UV light by scattering, as shown in Figure . The additive agent consists of two parts: a polarized UV-responsive unit and a polymerizable unit. Concerning the polarized UV responsive unit, the photo-reactive functional groups of photo-isomerization and photo-crosslinked types were selected and the monomer-carrying chalcone unit as the additive agent was designed. The FFS cell showed comparable electro-optics to conventional FFS cells with PI. In 2019, BOE Co. reported a prototype of a 1.54′′ ADS panel with 293 ppi exhibiting the same level of performance compared to the conventional one, emphasizing significant shortening of cell process flow and reduction of highest temperature during cell process which can open new possibilities to the LCD industry in performance improving and cost. In 2021, Wuhan CSOT Co. reported a prototype of 6′′ FFS-mode SA-LCDs with the same level of electro-optic performance though its CR was slightly lower than that of the PI-FFS panel.
Figure 13. Schematic illustration of the fabrication process for the self-alignment FFS-mode LC cell (Self-FFS cell): (a) before preparing the SAL and (b) after preparing the SAL and obtaining the homogeneous alignment, (c) the material design concept of the additive agents, and (d) the monomer-carrying chalcone unit used in this study [Citation59,Citation60].
![Figure 13. Schematic illustration of the fabrication process for the self-alignment FFS-mode LC cell (Self-FFS cell): (a) before preparing the SAL and (b) after preparing the SAL and obtaining the homogeneous alignment, (c) the material design concept of the additive agents, and (d) the monomer-carrying chalcone unit used in this study [Citation59,Citation60].](/cms/asset/8fc1b1e8-71ca-46db-8f54-3c4265857e21/tjid_a_2281224_f0013_oc.jpg)
2.9. Major issues in VA mode
Many companies produce PS-VA mode mainly for TV applications and gaming monitors. In PS-VA mode, the fishbone-shaped pixel electrode is utilized, and the additive RMs in the LC mixture should be polymerized by UV irradiation to form a surface pretilt angle of about 88.5o in four different directions, while a bias voltage between the pixel and top plane-shaped electrode is applied [Citation2,Citation5]. In addition, 4-domain PS-VA mode was not enough to reduce color shift along off-normal viewing directions, and thus 8-domain PS-VA mode in which a pixel is divided into two regions and its voltage-on tilt angles are different from each other was applied to panels with resolution up to UHD (4K) [Citation64,Citation65]. However, as the panel resolution increases from 4K to 8K in TV, the aperture ratio drops to about 40% and the pixel size becomes too smaller, increasing the area ratio of trunk to fishbone [Citation65–67]. In PS-VA mode, the fishbone-shaped electrode could not control LC’s tilting directions well enough to have φ = ±45o in all pixel areas, and the trunk area does not contribute much to transmittance, as shown in Figure (a) and (b). Consequently, forming an 8-domain could result in severe transmittance loss in such a high-resolution display. The main challenge in VA mode is to keep high transmittance while pixel density increases. On the other hand, instead of forming an 8-domain, a super-viewing film that controls light propagation direction is added above the 4-domain VA panel to compensate for color shift along the horizontal direction [Citation68].
Figure 14. Simulated transmittance in a pixel of PS-VA mode: (a) Fishbone electrode (pixel) and plane electrode (common), (b) Transmittance profile in different resolution, and comparison of transmittance with LC orientation profile in (c) PS-VA and (d) C-PS-VA mode [Citation65].
![Figure 14. Simulated transmittance in a pixel of PS-VA mode: (a) Fishbone electrode (pixel) and plane electrode (common), (b) Transmittance profile in different resolution, and comparison of transmittance with LC orientation profile in (c) PS-VA and (d) C-PS-VA mode [Citation65].](/cms/asset/8be878d6-e6bd-4eb4-8489-370107de64e8/tjid_a_2281224_f0014_oc.jpg)
As one of the solutions to improve transmittance in PS-VA mode, two approaches have recently been proposed: a new LC mixture [Citation65,Citation69] and a new pixel design [Citation66,Citation67,Citation70]. In the first case, a chiral dopant is doped into -LC mixture with higher Δn while the same pixel structure is used (named C-PS-VA). In this approach, LC tilts down while twisting in a voltage-on state so that the polarization change occurs not by phase retardation but polarization rotation, indicating higher retardation of the LC layer like 450 nm is required to get maximized transmittance. In PS-VA mode, the LC director could tilt down even above the trunk part of a fishbone electrode, but φ = 0 or ±90o, so that the transmittance does not occur, generating cross-shaped disclination lines (see Figure [c]). The portion of the disclination lines becomes higher as the pixel size becomes smaller, which is the origin of low transmittance in PS-VA mode. In C-PS-VA mode, the LC director tilts down while twisting over a whole pixel area including the trunk part, and its twisting angle depends on the ratio of cell gap to chiral pitch. Then, a whole pixel area could contribute to transmittance, claiming more than a 10% increase in transmittance in optimized retardation compared to that of PS-VA mode, especially in smaller pixel size, as represented in Figure (d). However, this approach brings up some issues such as slower τd and higher light leakage in diagonal directions of the crossed polarizer, decreasing CR at the off-normal axis, compared to those of PS-VA mode. S Chuang et al. claim that the τd can be improved by doping an LC with super low viscosity its associated reliability is improved by optimizing RMs, and the light leakage can be reduced with the optimized compensation film. In the latter case, a transparent ITO electrode is adopted to replace the metallic common electrode in an active pixel and serves as a Cst, that is, one more ITO common electrode is formed in a TFT array while removing the common line inside a pixel region and covering metallic signal lines and forms Cst with another pixel ITO [Citation65]. Like in the FFS mode, the existence of Cst does not block an incident light, improving transmittance. In addition, the metallic signal lines are shielded by common ITO to avoid any light leakage by a noise field associated with signal lines, and thus the aperture ratio can be further increased by reducing the width of BM. The final aperture ratio of the subpixel is 89.2%, increased by 26% compared with the original design but the transmittance improvement ratio is 15%, requiring more analysis. Adding one more ITO layer may cost the process time and product cost, but it helps to improve transmittance in 8K and to get an excellent dark state to give rise to CR over 6000:1.
As mentioned, 8-domain PS-VA sacrifices transmittance severely, so the recent technology trend is to use light control film above the 4-domain panel surface to minimize color shift in a horizontal direction, as shown in Figure . As indicated, when light coming from an LCD panel in a normal direction spreads out horizontally, the gamma-distortion-index (GDI) in a horizontal direction will be lowered while image blurring in a normal direction might be increased. H. Lee et al. reported a super-view film in which two optical layers with trapezoid patterned structure and different refractive indices n1 and n2 are composed as one film [Citation65]. In a condition n1 < n2, total internal reflection could be generated, which can change beam direction laterally and iso-luminance contour proves its function. Here, the main factors affecting visibility and readability at normal and off-normal axes are the shape of resin material, laminated structure, and lens profile, and the optimized structure is commercialized to most UHD TVs with PS-VA mode. Nevertheless, some level of image blurring at normal direction is not escapable while it does not exist in TVs with FFS LCDs and OLEDs. Therefore, other ways of improving transmittance and minimizing GDI distortion at the off-normal axis need to be developed further.
Figure 15. (a) Structure of wide-viewing-angle film with representative three optical rays and (b) Comparison of iso-luminance contours [Citation68]. AG indicates anti-glare film.
![Figure 15. (a) Structure of wide-viewing-angle film with representative three optical rays and (b) Comparison of iso-luminance contours [Citation68]. AG indicates anti-glare film.](/cms/asset/9eb31c02-b1aa-4699-baf4-f036b0ffade4/tjid_a_2281224_f0015_oc.jpg)
3. Emerging LC materials
LCDs have been leading the display industry and market, and thus, LC materials science and technologies have grown with a large research community. Researchers in this field have sought and developed new LC materials that can be emerged in a new LC science and technology in and beyond the display applications.
3.1. Blue phase LCs
Among thousands of LC phases, blue phases (BPs) fall into somewhere in the highest crystalline structure, except for amorphous BPIII. In the general classification of BPs, BPI, and BPII are body-centered cubic (BCC) and simple cubic (SC) structures, and BPIII is an amorphous structure, as revealed in Figure (BPI) and Figure (BPIII), respectively [Citation71,Citation72]. Although their structures represent periodicity, they are optically isotropic, which can give excellent properties for display applications. Table summarizes the main advantage is the optically isotropic state. This can contribute to a good dark property to enhance the contrast ratio of LCDs.
Figure 16. Structure of blue phase I [Citation72].
![Figure 16. Structure of blue phase I [Citation72].](/cms/asset/4bd6198f-295b-4a91-9b86-9fd005ae5b92/tjid_a_2281224_f0016_oc.jpg)
Figure 17. Structure of blue phase III [Citation72]
![Figure 17. Structure of blue phase III [Citation72]](/cms/asset/1906ea6f-f317-47d9-8af9-1d1f328fafe7/tjid_a_2281224_f0017_oc.jpg)
Table 3. Normal mode and blue phase mode [Citation72–74].
Although BPs were discovered more than 120 years ago; for about 80 years, they had not attracted much attention due to two main reasons. First, unobservable birefringence. Secondly, a narrow temperature range, like a few degrees. The system can be mainly described with free energies of elastic deformation, topological defects, interfacial elastic deformation, and interfacial energy. The free energetic balance can be collapsed easily under a small temperature change. In 2002, Kikuchi et al. successfully stabilized these soft lattice structures by mixing with prepolymers and photopolymerization, as illustrated in Figure [Citation75], and Samsung Electronics demonstrated a PSBP LCD prototype in the exhibition of the Society for Information Display (SID) 2008 [Citation76]. The isotropic monomers are miscible within the isotropic disclination lines in the BP structure. An optimum condition of photopolymerization can form the polymer network entangled within the disclination lines and polymer-stabilized blue phases (PSBPs) were able to extend the narrow temperature range to wide enough to cover room temperature. Applying voltage to the PSBPs induces birefringence based on the Kerr effect, which attracted LCD researchers’ huge attention because of the possibility of next-generation LCDs as its different features are synthesized in Table . The induced birefringence can be determined as Δnind(E) = λKcE2, where λ is the wavelength, Kc is the Kerr constant, and E is the electric field strength [Citation75,Citation77–79].
Figure 18. Polymer-stabilized blue phase I and its world’s prototype were made by Samsung Electronics in the exhibition of the SID 2008 [Citation75,Citation76].
![Figure 18. Polymer-stabilized blue phase I and its world’s prototype were made by Samsung Electronics in the exhibition of the SID 2008 [Citation75,Citation76].](/cms/asset/08104f72-6b47-4af4-b5b5-d0b199393a5d/tjid_a_2281224_f0018_oc.jpg)
The main drawbacks of the PSBP mode are high driving voltage, hysteresis on electro-optic switching, residual birefringence after switching, and bluish initial state due to the BPI or BPII cubic structures. The operating voltage of PSBPs and OILCs is one of the main points to overcome to implement this idea. Many approaches were reported, and among them is the modification of electrodes to enhance the lateral field vector by increasing electrode height [Citation76,Citation79–83]. Among these drawbacks, optically isotropic LC (OILC) and BPI can solve hysteresis, residual birefringence, and bluish initial state [Citation72–74,Citation84–89]. The amorphous structure of BPIII appears without color, and the polymer network strongly interacts with BPLCs due to the random entanglement of disclinations, which brings no hysteresis and residual birefringence, as presented in Figure [Citation72,Citation73]. Also, using PSBPs and OILCs, viewing angle switchable films can be made for functional display applications [Citation90,Citation91].
Figure 19. Comparison of polymer-stabilized BPI, isotropic phase, and BPI and the switching property of BPIII [Citation72,Citation73].
![Figure 19. Comparison of polymer-stabilized BPI, isotropic phase, and BPI and the switching property of BPIII [Citation72,Citation73].](/cms/asset/ce94ebcf-c6ce-4046-804f-d26702ef02a4/tjid_a_2281224_f0019_oc.jpg)
Another important application of BPs is photonic crystal devices that can be tunable by electric fields, as reflected in Figure [Citation92,Citation93]. As the monodomain [Citation94,Citation95] or single crystal [Citation96,Citation97] BPs were successful, long-range order of different periodic lattice structures can generate different colors based on the interaction with visible wavelength. The selective wavelength of Bragg reflection can be described as λs = 2na/(h2 + k2 + l2)1/2, where n, a, and λs denote the average refractive index of a medium in a composite, the lattice constant of blue phases, and the selective wavelength, respectively. The h, k, and l denote the Miller indices. The refractive index and lattice constant can be adjusted by an applied electric field, so that the wavelength changes, showing tuneable colors.
Figure 20. BP color tuneable Photonic soft crystals [Citation84].
![Figure 20. BP color tuneable Photonic soft crystals [Citation84].](/cms/asset/de7ef6d8-bd21-4edc-988c-8395a6930073/tjid_a_2281224_f0020_oc.jpg)
3.2. Ferroelectric nematic LCs
In 1916, Born theoretically predicted that all dipoles may point along the same direction [Citation98], and recently, Chen et al. reported a principle experiment to demonstrate the phase exists in 2020 [Citation99]. Nematic LC has head-to-tail invariance because permanent electric dipoles of LC molecules are randomly distributed and give nematic symmetry. In the ferroelectric nematic fluid, the permanent dipole moments can withstand thermal fluctuation, which can be briefly estimated as (µ2/ε0εV) > kBT, where V is the molecular volume [Citation16]. Assuming 5CB at room temperature with V = 2 nm3 and ε = 10, the ferroelectric state would be stable when µ > ∼ 2.7 × 10−29 Cm, which is ∼ 8.2 D. Dipole moment of 5CB is typically estimated by ∼ 6.5 D, which implies no ferroelectricity in 5CB at room temperature. Kikuchi and coworkers reported a material in a unidirectional highly ferroelectric-like polar order with a high dipole moment ∼ 9.4 D [Citation100], and modified molecular structures to study smectic and nematic polar orderings. Although simplifying the molecular structure can reduce the dipole moment, the dipole–dipole interactions in molecular assemblies give highly ordered ferroelectricity. Figure (a) shows the related molecular structures [Citation101]. Also, Mandle et al. reported a rod-like highly polar material with the occurrence of the lower-temperature nematic phase [Citation102]. Then they modified terminal chains, polar terminal groups, lateral bulky groups, and linking unit orientation in the molecular structures, as indicated in Figure (b) [Citation103]. The electric field strength to reorient the polar ordering only requires 10−2 V/µm due to their high dielectric anisotropy ∼104. Along with the development and modification of such high polar order materials, further device application may exhibit excellent performance and applicability in research and application.
Figure 21. Molecular structures and modification of ferroelectric LCs in nematic phase [Citation87,Citation103].
![Figure 21. Molecular structures and modification of ferroelectric LCs in nematic phase [Citation87,Citation103].](/cms/asset/bbec628e-b2e9-4d88-a1a9-64ba29b192a8/tjid_a_2281224_f0021_oc.jpg)
4. Future perspectives
Every year, the electro-optic performances of LCDs are enhanced while the product cost goes down, rendering LCDs more valuable in cost-performance efficiency. However, it has intrinsic demerits, such as flexible and free-form factor, color gamut, and lightness compared to OLEDs. OLEDs can overcome those demerits of LCDs and keep extending their market share with aggressive market entry, a subject extensively reported by various media outlets. More recently, other emissive displays, such as QD-OLEDs and micro-LEDs, have emerged and commercialized in large-sized displays, indicating that the market share of LCDs will go down and shrink in some application fields.
For small-sized high-end displays, the transition from LCD to OLED has occurred for only 10 years because OLEDs can carry inherent added values, considering the nature of the products and the compatibility of OLED technology in their usage environments and thus huge investments had made acceleration of OLED mobile business. However, for medium and large-sized displays, this shift has not been done rapidly because the displays are usually used at a fixed distance and may not exhibit significant differences between LCD and OLED displays. Moreover, along with a prolonged economic downturn, businesses confront the challenge of generating profits through their products. In such circumstances, investing heavily in OLED technology to compete with LCD in product segments where differentiation is less pronounced becomes increasingly arduous. On the other hand, the automotive display sector has witnessed a swift transition akin to small-sized displays, although LCDs currently dominate the market share. The primary reason for this shift is to differentiate from the conventional car style by adding values to the end product with more form factor. Though this is interesting to watch out for because we believe voltage-driven LCDs can offer much better reliability than current-driven OLEDs.
A decade ago, self-emissive OLEDs were anticipated with a distinct cost advantage and a clear technological differentiator over LCDs would eventually permeate into most product categories. Nevertheless, continuous technological advancements and performance enhancements in LCD technology, coupled with its cost competitiveness by many companies and research institutions, make it likely that LCD will persist as a cost-effective and high-reliability display solution for an extended period despite the significant investments and growth in the OLED sector.
5. Summary
TFT-LCDs continue to evolve to display natural images with a high dynamic range of brightness, CR, and colors closest to what we see with our eyes and enhance light efficiency for low power consumption. This paper reviewed the recent progress of materials, optical components, optimization of the pixel structure, and process to maximize electro-optic performances. Improvement in key materials like LC mixtures and alignment layers, including self-aligning, is performed yearly, and inorganic materials like CNTs and QDs are utilized in LCDs. Functional optical components like light-guiding films, compensation films, QD-embedded films, and mini-LEDs continuously enhance LCDs for high color purity, high CR, and low power consumption. Along with those breakthroughs, 110′′ 16K LCD with oxide TFT, 600 and 1 Hz frame-rate LCDs, and high-end LCDs with CR of 1,000,000:1 and 1600 nits of peak brightness are reported. Further, new LC materials that show optical isotropy and ferroelectric nematic phase are reviewed. We hope these materials can be applied to practical TFT-LCDs in the near future. In conclusion, we expect LCDs to continue their market share as a valuable option in the display technology field, thanks to their ongoing performance strengths and cost competitiveness.
Acknowledgments
This research was supported by the Basic Science Research Program through the National Research Foundation of Korea (NRF) funded by the Ministry of Education [2021R1I1A1A01060001] and the Ministry of Science and ICT (MSIT) [2022R1A2C2091671] by the Technology Innovation Program funded by the Ministry of Trade, Industry & Energy (MOTIE, Korea) [20022529].
Disclosure statement
No potential conflict of interest was reported by the author(s).
Additional information
Funding
Notes on contributors

Junho Jung
Junho Jung received his M.S. and B.S. degrees from Jeonbuk National University in 2010 and 2008. His research focuses on performance improvement of fringe-field switching liquid crystal displays. He currently serves as a senior engineer in LG Display, working on advanced panel technologies and cell material development.

HanByeol Park
HanByeol Park received his B.S. degree from the Department of Polymer Nano Science and Technology, Jeonbuk National University, South Korea, in 2022. He is currently working toward his M.S. degree in Nano Convergence Engineering, at Jeonbuk National University. He has been researching LC devices.

Ha Young Jung
Ha Young Jung received her B.S. Polymer Nano Engineering degree from Jeonbuk National University (Republic of Korea) in 2023. She is currently an M.S. student at Jeonbuk National University. She has been researching quantum dots for display applications.

Se Eun Jung
Se Eun Jung received her B.S. Polymer Nano Engineering degree from Jeonbuk National University, South Korea, in 2022. She is currently working toward an M.S. Nanoconvergence Engineering degree from Jeonbuk National University. She has been researching CNT polarizer.

Seo Gyun Kim
Seo Gyun Kim received his Ph.D. from Dong-A University in 2020. He is currently a senior researcher at the Korea Institute of Science and Technology.

Tae Hyung Kim
Tae Hyung Kim received his Ph.D. degree from the Graduate School of Flexible & Printable Electronics, Jeonbuk National University, South Korea, in 2019. He is presently working as a research assistant professor in the Department of Nano Convergence Technology, at Jeonbuk National University. His research is about developing CNT Polarizers and Energy storage devices.

Young Jin Lim
Young Jin Lim received her M.S. degree from the School of Advanced Materials Engineering and her Ph.D. degree from the Department of Polymer Nano Science and Technology at Jeonbuk National University in 2006 and 2010, respectively. She is presently working as a research professor in the Department of JBNU-KIST Industry-Academia Convergence Research at the same university. Her research topics are on developing high-performance transmissive LCDs, light shutters using carbon black or LC, and orientation and interaction of the LC in nanoscale.

Bon-Cheol Ku
Bon-Cheol Ku received his Ph.D. from the University of Massachusetts Lowell in 2005. He is currently a principal research scientist at the Korea Institute of Science and Technology.

MinSu Kim
MinSu Kim received his M.S. and B.S. degrees from Jeonbuk National University (Republic of Korea) in 2010 and 2008. He received his Ph.D. degree from Liquid Crystal Institute (LCI, currently name changed to Advanced Materials and Liquid Crystal Institute), Kent State University (USA) in 2015. He continued his research as a postdoctoral research fellow in Physics and Astronomy, at Johns Hopkins University (USA) from 2017 to 2021. He is now affiliated with Nano Convergence Engineering, Jeonbuk National University as a research professor, focusing on functional materials and their applications.

Seung Hee Lee
Seung Hee Lee received his Ph.D. degree from the Physics Department of Kent State University in 1994. From 1995 to August 2001, he worked at the LCD Division of Hyundai Electronics and made a big contribution to inventing and commercializing new wide-viewing-angle technology called ‘fringe-field switching (FFS),’ which is mainly applied for all kinds of high image quality TFT-LCDs at present. In September 2001, he became a professor at Jeonbuk National University, working on the development of new electro-optic materials and light modulation devices at present. He was awarded ‘King of Invention’ twice in the industry, ‘Fellow’ in 2008, ‘Special Recognition Award’ in 2012, and ‘Jan-Rajchman Prize’ in 2016 from SID, and ‘Merck Award-Major’ from Korean Information Display Society in 2013.
References
- S.H. Lee, S.L. Lee, and H.Y. Kim, Appl. Phys. Lett. 73, 2881 (1998).
- K. Hanaoka, Y. Nakanishi, Y. Inoue, S. Tanuma, Y. Koike, and K. Okamoto, SID Symp. Dig. Tech. Pap. 35, 1200 (2004).
- D.H. Kim, Y.J. Lim, D.E. Kim, H. Ren, S.H. Ahn, and S.H. Lee, J. Inf. Disp. 15, 99 (2014).
- E. Lueder, P. Knoll, and S.H. Lee, Liquid Crystal Displays: Addressing Schemes and Electro-Optical Effects, Third Edition (John Wiley & Sons, Inc., 2022).
- Y.W. Lim, K. Park, and H.H. Kim, SID Symp. Dig. Tech. Pap. 53, 1445 (2022).
- C.W. Tang, and S.A. Vanslyke, Appl. Phys. Lett. 51, 913 (1987).
- T. Tsujimura, OLED Display Fundamemtals and Applications (John Wiley & Sons, Inc., 2017).
- I. Hendy, and P. Gray, Inf. Disp. 36, 28 (2020).
- H.J. Shin, Y.H. Kim, D.M. Kim, S.H. Yun, S.J. Bae, H.S. Kim, H.W. Lee, and C.H. Oh, J. Soc. Inf. Disp. 31, 253 (2023).
- iPad Pro Apple, <https://www.Apple.Com/Ipad-Pro/>.
- N.Q. 8k Samsung, <https://www.Samsung.Com/Us/Televisions-Home-Theater/Tvs/Samsung-Neo-Qled-8k>.
- C. Li, X. Lin, P. Hu, B. Hu, J. Wang, W. Wang, X. Xu, X. Fang, R. Zhou, Y. Liao, S. Lee, B. Wang, L. Lin, and S. Park, SID Symp. Dig. Tech. Pap. 54, 811 (2023).
- Z. Huang, X. Shao, Z. Wang, Z. Zou, H. Zhang, Z. Li, X. Wang, T. Min, Y. Zhang, J. Sha, and Z. Zhang, SID Symp. Dig. Tech. Pap. 54, 799 (2023).
- S.-H. Baek, J.-W. Jung, H.-J. Park, D.-J. Lee, S.-M. Park, K.-S. Kwon, H.-S. Jin, H. Park, D. Lee, M.S. Kim, and S.H. Lee, SID Symp. Dig. Tech. Pap. 54, 803 (2023).
- D.Y. Lee, H.Y. Jung, J. Jung, S.-H. Baek, J.-W. Jung, S.-M. Park, K.-S. Kwon, H.-S. Jin, D.-J. Lee, G. Kim, J. Park, S.H. Lee, and M.S. Kim, 2023, Research Square (Preprint)
- O.D. Lavrentovich, Proc. Natl. Acad. Sci. U. S. A. 117, 14629 (2020).
- A. Goetz, S. Laut, J. Laur, and H. Hirschmann, SID Symp. Dig. Tech. Pap. 52, 640 (2021).
- P. Zhao, L. Huang, S.C. Laut, and J.-H. Jung, Information Display Workshops, p. 18 (2022).
- E.-L. Hsiang, Q. Yang, Z. He, J. Zou, and S.-T. Wu, Opt. Express 28, 36822 (2020).
- J.H. Kim, J.H. Lee, J.C. Lim, C.H. Lee, M.H. Song, J.Y. Yoon, H.D. Choi, J.J. Kim, C.H. Oh, and S.D. Yeo, SID Symp. Dig. Tech. Pap. 35, 115 (2004).
- Y. Utsumi, D. Kajita, S. Takeda, H. Kagawa, I. Hiyama, Y. Tomioka, and K. Ono, Jpn. J. Appl. Phys. 47, 2205 (2008).
- Y. Utsumi, S. Takeda, I. Hiyama, Y. Tomioka, M. Ishii, and K. Ono, Jpn. J. Appl. Phys. 47, 2144 (2008).
- H.J. Ahn, C. Lim, D. Kim, J. Lee, H. Park, S. Lee, J. Woo, W. Shin, and M. Jun, SID Symp. Dig. Tech. Pap. 43, 1432 (2012).
- H. Matsukawa, Y. Shimano, M. Suefuji, Y. Umeda, and K. Mimura, in International Display Workshops in conjunction with Asia Display (2012), p. 1581.
- L. Guo, X. Shao, H. Hu, J. Liu, Y. Qu, Z. Wang, Y. Liao, K. Wang, K. Dai, W. Zhang, D. Chen, Y. Guo, and P. Lee, SID Symp. Dig. Tech. Pap. 54, 923 (2023).
- J.W. Ryu, J.Y. Lee, H.Y. Kim, J.W. Park, G.D. Lee, and S.H. Lee, Liq. Cryst. 35, 407 (2008).
- J. Lee, J.-H. Lee, J.-H. Kim, C. Lim, S.-O. Mun, C.-H. Oh, J. Chang Kim, and G.-D. Lee, SID Symp. Dig. Tech. Pap. 36, 642 (2005).
- D. Kajita, I. Hiyama, Y. Utsumi, M. Ishii, and K. Ono, SID Symp. Dig. Tech. Pap. 36, 1160 (2005).
- N. Aoki, and S.I.D. Symp, Dig. Tech. Pap. 37, 1087 (2006).
- Y.J. Lim, H.S. Jeon, Y.J. Han, J. Jung, W. Kim, Y.H. Park, G. Kim, J. Park, M.S. Kim, and S.H. Lee, Opt. Mater. Express 13, 1563 (2023).
- D.J. Lee, J.H. Jung, J.S. Shin, G.T. Kim, and J.K. Park, SID Symp. Dig. Tech. Pap. 50, 160 (2019).
- LG Display Co. Ltd. (2023), <https://Www.Lgdisplay.Com/Eng/Company/Investment/Regular-Report?Path=quarterly-Report>.
- J.D. Noh, H.Y. Kim, J.H. Kim, S.H. Nam, and S.H. Lee, Jpn. J. Appl. Phys. 42, 1290 (2003).
- B. You, H. Nam, and H. Lee, SID Symp. Dig. Tech. Pap. 51, 676 (2020).
- Y. Zhang, C. Shen, J. Sha, Z. Zhang, X. Wang, and Z. Sun, SID Symp. Dig. Tech. Pap. 54, 648 (2023).
- T. Tsuruma, Y. Goto, A. Higashi, M. Watanabe, H. Yamaguchi, T. Tomooka, and H. Kikkawa, Proc. 31st Int. Disp. Res. Conf., pp. 13–16 (2011).
- S.-W. Oh, J.-H. Park, J.-H. Lee, and T.-H. Yoon, Opt. Express 23, 24013 (2015).
- S.W. Oh, J.H. Park, J.M. Baek, T.H. Choi, and T.H. Yoon, Liq. Cryst. 43, 972 (2016).
- M.S. Kim, P.J. Bos, D.-W. Kim, D.-K. Yang, J.H. Lee, and S.H. Lee, Sci. Rep. 6, 35254 (2016).
- M.S. Kim, S.L. Lee, and S.H. Lee, in High Quality Liquid Crystal Displays and Smart Devices: Development, Display Applications and Components (Institution of Engineering and Technology, 2019). pp. 55–77. London, United Kingdom.
- R.B. Meyer, Phys. Rev. Lett. 22, 918 (1969).
- M.S. Kim, P.J. Bos, D.-W. Kim, C.-M. Keum, D.-K. Yang, H.G. Ham, K.-U. Jeong, J.H. Lee, and S.H. Lee, Opt. Express 24, 29568 (2016).
- M.S. Kim, H.G. Ham, H.-S. Choi, P.J. Bos, D.-K. Yang, J.H. Lee, and S.H. Lee, Opt. Express 25, 5962 (2017).
- M.S. Kim, H.S. Jin, S.J. Lee, Y.H. Shin, H.G. Ham, D.K. Yang, P.J. Bos, J.H. Lee, and S.H. Lee, Adv. Opt. Mater. 6, 1800022 (2018).
- J.-W. Kim, T.-H. Choi, T.-H. Yoon, E.-J. Choi, and J.-H. Lee, Opt. Express 22, 30586 (2014).
- H. Chen, F. Peng, M. Hu, and S.-T. Wu, Liq. Cryst. 42, 1730 (2015).
- D. Lim, H. Lee, J. Kim, K. Lee, H. Yun, K. Han, and Y. Jeong, Int. Disp. Work., p. 807 (2006).
- H. Kikuo and I. Ono, in International Display Workshops in Conjunction with Asia Display (2012), p. 933.
- K. Ono and H. Matsukawa, in International Display Works (2013), p. 24.
- X. Zhou, L. Zhang, J. Gao, W. Zhang, K. Dai, Y. Gao, Z. Wang, and X. Shao, SID Symp. Dig. Tech. Pap. 54, 814 (2023).
- Nano Solution, <http://www.Nano-Sol.Com/Conductive.Php>.
- B.G. Kang, Y.J. Lim, K.U. Jeong, K. Lee, Y.H. Lee, and S.H. Lee, Nanotechnology 21, 405202 (2010).
- T.H. Kim, J.G. Park, Y.K. Kim, Y.J. Lim, J.W. Kang, E.S. Kim, J.Y. Kwon, Y.H. Lee, and S.H. Lee, Nanoscale 12, 17698 (2020).
- H.S. Shin, R. Manda, T.H. Kim, J.G. Park, Y.J. Lim, B.K. Kim, Y.H. Lee, and S.H. Lee, Liq. Cryst. 47, 1604 (2020).
- S.E. Jung, S.G. Kim, T.H. Kim, J. Kim, M.S. Kim, D. Lee, D.Y. Lee, B.C. Ku, and S.H. Lee, Adv. Mater. Technol., 2301176 (2023).
- S.E. Jung, S.G. Kim, M.S. Kim, T.H. Kim, M. Ju, E. Kim, B.-C. Ku, and S.H. Lee, in 23rd Korean Liquid Crystal Conference (2023), p. Poster.
- E.A. Plummer, M. Klasen-Memmer, R. Fortte, H. Haensel, and G. Archetti, SID Symp. Dig. Tech. Pap. 49, 449 (2018).
- G.Y. Lee, J.H. Lee, S.M. Song, R. Manda, Y.J. Lim, and S.H. Lee, SID Symp. Dig. Tech. Pap. 51, 1982 (2020).
- M. Mizusaki, H. Tsuchiya, T. Itoh, and K. Minoura, SID Symp. Dig. Tech. Pap. 49, 455 (2018).
- M. Mizusaki, T. Okazaki, K. Okamoto, and T. Shibata, SID Symp. Dig. Tech. Pap. 51, 975 (2020).
- R. Yang, J. Wu, R. Wang, X. Wu, L. Qu, X. Xu, Y. You, H. Zhao, Y. Feng, F. Yang, X. Qi, Y. Qiu, and D. Wang, SID Symp. Dig. Tech. Pap. 50, 481 (2019).
- R. He, P. Wen, S.W. Kang, S.H. Lee, and M.H. Lee, Liq. Cryst. 45, 1342 (2018).
- H. Jiang, R. He, Q. Tang, W. Cheng, C. Huang, G. Liu, L. Sun, J. Yuan, Z. Li, and X. Zhang, SID Symp. Dig. Tech. Pap. 52, 590 (2021).
- S.S. Kim, SID Symp. Dig. Tech. Pap. 36, 1842 (2005).
- S. Chuang, F. Chen, R. Chou, J. Hsieh, R. Lin, and Z. Chen, SID Symp. Dig. Tech. Pap. 53, 417 (2022).
- S. Borjigin, Z. Feng, Z. Shi, L. Zeng, S.J. Chen, C.Y. Lee, and X. Zhang, SID Symp. Dig. Tech. Pap. 51, 13 (2020).
- J. Liu, L. Li, Y. Um, H. Xu, and C.C. Hsieh, Displays 77, 102407 (2023).
- H. Lee, Y. Lee, and S. Jo, SID Symp. Dig. Tech. Pap. 52, 511 (2021).
- M. Chen, Q. Song, X. Chen, T.J. Tseng, D. Li, L. Chen, X. Zhang, and H. Meng, SID Symp. Dig. Tech. Pap. 51, 154 (2020).
- H.L. Ong, J. Chou, Y. Zhao, and Z. Zhu, SID Symp. Dig. Tech. Pap. 52, 637 (2021).
- O. Henrich, K. Stratford, M.E. Cates, and D. Marenduzzo, Phys. Rev. Lett. 106, 107801 (2011).
- M.S. Kim, Liquid Crystalline Amorphous Blue Phase: Tangled Topological Defects, Polymer-Stabilization, and Device Application (2015).
- M.S. Kim, and L.C. Chien, Soft Matter 11, 8013 (2015).
- M.S. Kim, and L.-C. Chien, SID Symp. Dig. Tech. Pap. 46, 1524 (2015).
- H. Kikuchi, M. Yokota, Y. Hisakado, H. Yang, and T. Kajiyama, Nat. Mater. 1, 64 (2002).
- H. Lee, H.-J. Park, O.-J. Kwon, S.J. Yun, J.H. Park, S. Hong, and S.-T. Shin, SID Symp. Dig. Tech. Pap. 42, 121 (2011).
- Z. Ge, S. Gauza, M. Jiao, H. Xianyu, and S.T. Wu, Appl. Phys. Lett. 94 (2009).
- M.S. Kim, M. Kim, J.H. Jung, K.S. Ha, S. Yoon, E.G. Song, A.K. Srivastava, S.-W. Choi, G.-D. Lee, and S.H. Lee, SID Symp. Dig. Tech. Pap. 40, 1615 (2009).
- M. Kim, M.S. Kim, B.G. Kang, M.-K. Kim, S. Yoon, S.H. Lee, Z. Ge, L. Rao, S. Gauza, and S.-T. Wu, J. Phys. D. Appl. Phys. 42, 235502 (2009).
- G.H. Yang, M.-K. Kim, B.G. Kang, S. Yoon, M.S. Kim, M. Chae, S.H. Lee, L. Rao, and S.-T. Wu, SID Symp. Dig. Tech. Pap. 41, 170 (2010).
- S. Yoon, M. Kim, M.S. Kim, B.G. Kang, M.-K. Kim, A.K. Srivastava, S.H. Lee, Z. Ge, L. Rao, S. Gauza, and S.-T. Wu, Liq. Cryst. 37, 201 (2010).
- S. Yoon, M. Kim, M.S. Kim, B.G. Kang, M.-K. Kim, S.-W. Kang, S.H. Lee, W.S. Kang, and G.-D. Lee, Mol. Cryst. Liq. Cryst. 529, 95 (2010).
- M.-K. Kim, M.S. Kim, B.G. Kang, G.H. Yang, A. Mukherjee, S.-W. Kang, H. Lee, S.-T. Shin, and S.H. Lee, Mol. Cryst. Liq. Cryst. 543, 194/[960] (2011).
- Y.-C. Yang, and D.-K. Yang, Appl. Phys. Lett. 98, 023502 (2011).
- N.H. Park, S.C. Noh, P. Nayek, M.-H. Lee, M.S. Kim, L.-C. Chien, J.H. Lee, B.K. Kim, and S.H. Lee, Liq. Cryst. 42, 530 (2015).
- E.J. Shin, S.C. Noh, T.H. Kim, J.H. Kim, P. Nayek, M.-H. Lee, M.S. Kim, L.-C. Chien, J.H. Lee, B.K. Kim, and S.H. Lee, SID Symp. Dig. Tech. Pap. 46, 1483 (2015).
- S.S. Gandhi, M.S. Kim, J.-Y. Hwang, and L.-C. Chien, Adv. Mater. 28, 8998 (2016).
- R. Manda, S. Pagidi, M.S. Kim, C.H. Park, H.S. Yoo, K. Sandeep, Y.J. Lim, and S.H. Lee, Liq. Cryst. 45, 736 (2018).
- S. Pagidi, R. Manda, H.S. Shin, J. Lee, Y.J. Lim, M.S. Kim, and S.H. Lee, J. Mol. Liq. 322, 114959 (2021).
- M.S. Kim, Y.J. Lim, S. Yoon, S.-W. Kang, S.H. Lee, M. Kim, and S.-T. Wu, J. Phys. D. Appl. Phys. 43, 145502 (2010).
- M.S. Kim, Y.J. Lim, S. Yoon, M.-K. Kim, P. Kumar, S.-W. Kang, W.-S. Kang, G.-D. Lee, and S.H. Lee, Liq. Cryst. 38, 371 (2011).
- S.-Y. Lu, and L.-C. Chien, Opt. Lett. 35, 562 (2010).
- R. Manda, S. Pagidi, Y. Heo, Y.J. Lim, M.S. Kim, and S.H. Lee, NPG Asia Mater. 12, 1 (2020).
- P. Nayek, H. Jeong, H.R. Park, S.W. Kang, S.H. Lee, H.S. Park, H.J. Lee, and H.S. Kim, Appl. Phys. Express 5, 051701 (2012).
- Y. Chen, and S.T. Wu, Appl. Phys. Lett. 102, 171110 (2013).
- J.A. Martínez-González, X. Li, M. Sadati, Y. Zhou, R. Zhang, P.F. Nealey, and J.J. De Pablo, Nat. Commun. 8, 1 (2017).
- X. Li, J.A. Martínez-González, J.P. Hernández-Ortiz, A. Ramírez-Hernández, Y. Zhou, M. Sadati, R. Zhang, P.F. Nealey, and J.J. De Pablo, Proc. Natl. Acad. Sci. U. S. A. 114, 10011 (2017).
- M. Born, Sitzungsberichte Der Königlich Preuss. Akad. Der Wissenschaften Zu Berlin 30, 614 (1916).
- X. Chen, E. Korblova, D. Dong, X. Wei, R. Shao, L. Radzihovsky, M.A. Glaser, J.E. MacLennan, D. Bedrov, D.M. Walba, and N.A. Clark, Proc. Natl. Acad. Sci. U. S. A. 117, 14021 (2020).
- H. Nishikawa, H. Higuchi, Y. Okumura, H. Kikuchi, K. Shiroshita, Y. Haseba, S. Yamamoto, and K. Sago, Adv. Mater. 29, 1702354 (2017).
- H. Kikuchi, H. Matsukizono, K. Iwamatsu, S. Endo, S. Anan, and Y. Okumura, Adv. Sci. 9, 2202048 (2022).
- R.J. Mandle, S.J. Cowling, and J.W. Goodby, Phys. Chem. Chem. Phys. 19, 11429 (2017).
- R.J. Mandle, S.J. Cowling, and J.W. Goodby, Chem. – A Eur. J. 23, 14554 (2017).