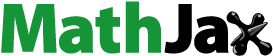
ABSTRACT
Epithelial cell adhesion molecule (EpCAM) is a transmembrane glycoprotein that plays several roles in cancer biology. EpCAM is an attractive therapeutic target because of its expression in most solid tumors. However, targeting EpCAM has been challenging because it is also highly expressed in normal epithelial tissues. Initial attempts to develop EpCAM-specific T-cell engagers were unsuccessful due to severe cytokine release effects, as well as serious on-target, off-tumor drug-related toxicities. We developed novel, conditionally active biological (CAB) bispecific antibodies that bind to both EpCAM and CD3 in an acidic tumor microenvironment. In healthy tissues, binding to EpCAM and CD3 is greatly reduced by a novel, dual CAB selection, where each binding domain is independently blocked by the presence of physiological chemicals known as Protein-associated Chemical Switches (PaCS). The CAB anti-EpCAM T-cell engagers displayed the anticipated bispecific binding properties and mediated the potent lysis of EpCAM-positive cancer cell lines through the recruitment of T cells in the tumor microenvironment. Xenograft studies showed that the efficacy of CAB bispecific antibodies is similar to that of a non-CAB anti-EpCAM bispecific antibody, but they have markedly reduced toxicity in non-human primates, indicating an unprecedentedly widened therapeutic index of over 100-fold. These preclinical results indicate that the dual CAB bispecific antibody is potentially both a powerful and safe therapeutic platform and a promising T cell-engaging treatment for patients with EpCAM-expressing tumors.
SUMMARY
Development of a novel conditionally active EpCAM-specific T-cell engager with enhanced safety and tolerability for treatment of solid tumors.
Introduction
Epithelial cell adhesion molecule (EpCAM) is a multifunctional transmembrane glycoprotein that mediates Ca2+-independent homotypic cell-cell adhesion during cell signaling, migration, proliferation, and differentiation.Citation1 EpCAM overexpression has been reported in most tumors, including adenocarcinoma of the colon, lung, breast, and prostate,Citation2,Citation3 making it a suitable target for many important solid tumor types and cancer stem cells. However, EpCAM expression in normal tissues limits its utility as a target for therapeutic antibodies due to the potential toxic effects of killing normal epithelial tissues throughout the body. In fact, many antibodies targeting EpCAM have been developed for the treatment of cancers, although there are currently no marketed drugs available due to low tolerability, or inferior efficacy from attempts to limit therapeutic-related and on-target toxicities relative to standard cancer therapy.Citation4
Immuno-oncology therapies that use bispecific antibodies to redirect the cytotoxic activity of T cells in a non-MHC-restricted fashion have been clinically validated. CD19 × CD3 bispecific blinatumomab was the first US Food and Drug Administration (FDA)-approved T-cell engager (TCE)Citation5 with outstanding single-agent activity in patients with B-cell acute lymphocytic leukemia.
More recently, additional TCEs targeting BCMA (elantramab, teclistamab), CD20 (epcoritamab, mosunetuzumab, glofitamab), DLL3 (tarlatamab), gp100 (tebentafusp), and GPCR5D (talquetamab) have been approved by the FDA (The Antibody Society. Therapeutic monoclonal antibodies approved or in regulatory review. (accessed 02/19/2024); www.antibodysociety.org/antibody-therapeutics-product-data). However, despite the clinical success of TCE, life-threatening adverse reactions, such as cytokine release syndrome (CRS), have been observed in ≥ 30% of patients.Citation6–8
TCEs targeting EpCAM have also emerged in recent years as promising agents for immune-mediated anticancer treatments. Catumaxomab,Citation9 a rat/mouse hybrid bispecific was approved in the European Union in 2009 for the treatment of malignant ascites but later withdrawn from market based on commercial reasons. Furthermore, systemic administration of catumaxomab was not possible, as repetitive intravenously administration of catumaxomab in patients with solid tumor resulted in a fatal drug-related event consisting of cytokine release syndrome, and severe hepatotoxicity leading to acute hepatic failure and termination of the study.Citation10 Solitomab (MT110),Citation11,Citation12 another EpCAM-targeting TCE showed beneficial clinical outcomes, but its development was hampered by dose-limiting toxicities. Based on the Phase 1 results, further development of solitomab in solid tumors was discontinued.Citation13
Eliminating the on-target, off-tumor toxicity associated with the EpCAM target in combination with tumor specific T-cell activation would potentially enable a powerful, broad-spectrum, pan-anti-tumor therapeutic and improve the overall survival rate of patients.
Conditionally Active Biologic (CAB) technology is a novel antibody generation platform that leverages the differential binding of naturally-occurring Protein-associated Chemical SwitchesTM (PaCSTM) on target molecules, yielding antibodies with no or very little binding to the target antigens in healthy tissue (normal alkaline physiological conditions, pH7.4 and above), but strong binding in the context of diseased tissues (acidic tumor microenvironment, TME).Citation14 CAB technology addresses the issue of on-target off-tumor immunotoxicities by limiting the formation of an immunological synapse between cancer cells expressing EpCAM and T cells in the TME. We have developed a new CAB bispecific TCE, BF-588-DualCAB, which has diminished binding to either the TCE target, CD3, or to the tumor target, EpCAM antigen, in healthy tissue. However, under acidic TME conditions, BF-588-DualCAB has a high binding affinity to both CD3 and EpCAM targets. This dual-selective binding to the targets in the bispecific format yields an overall synergistic selectivity of the TCE, which is the product of individual target selectivity. The data from this dual-conditional, CAB TCE antibody suggests that the conditional, preferential binding of BF-588-DualCAB in the TME allows for the potential use of EpCAM as an important cancer target for immunotherapy in the clinic by eliminating EpCAM-positive tumors but sparing normal tissues and the toxic activation of peripheral T cells.
Results
Engineering of EpCAM x CD3 bispecific conditional active biologics
This study aimed to generate CAB bispecific antibodies with reduced binding to EpCAM and CD3 under normal alkaline physiological conditions (≥pH7.4), while maintaining good binding under TME conditions (pH5.8–6.7).Citation15 A CAB TCE that is active only in the TME is expected to have reduced peripheral on-target off-tumor toxicity. The bispecific molecules described in this study are based on an IgG1-N297Q backbone (non-glycosylated IgG1 without effector functions) for the tumor-targeting arm. An anti-CD3 single chain variable fragment (anti-CD3-scFv) antibody was fused to the C-terminus of each light chain. The resulting molecules are homodimers with a molecular weight of ~ 200 kDa, with two binding domains for EpCAM and two binding domains for CD3 ().
Figure 1. In vitro characterization of BF-588-DualCAB. (a) Schematic overview of the structure. BF-588-DualCAB is based on a regular humanIgg1 antibody specific for EpCAM. At the C-terminus of each lightchain a CD3ε-specific scFv is fused via a short linker (red line).Line). The molecule has two binding sites for EpCAM and two for CD3ε. (b) pHrange ELISA. Binding to both, EpCAM and CD3, was determined by sandwich ELISA at different pH values as indicated on the graph. Antibodies were captured with CD3 immobilized on the plate and incubated with EpCAM-mouse fc. Bound proteins were detected with anti-mouse antibody-HRP conjugate. Binding of BF-588-MonoCAB andBF-588-DualCAB is highest at pH6.0 and drops with increased pH. ThepH inflection point (50% signal compared to pH6.0, which was set as100%) for BF-588-MonoCAB and DualCAB were determined by GraphPadPrism software. The signal for BF-588-WT is not pH-dependent. x-axis,pH of the ELISA buffers; y-axis, optical density (OD) at 450 nm. (c) pHaffinity ELISA. Binding to both, EpCAM and CD3, was determined by sandwich ELISA with a serial of antibody concentrations as indicated on the graph. Antibodies were captured with CD3 immobilized on the plate and incubated with EpCAM-mouse fc. The bound proteins were detected with anti-mouse antibody-HRP conjugate. The EC50values of BF-588-MonoCAB, BF-588-DualCAB and BF-588-WT at pH6.0 or pH7.4 were determined using the GraphPad prism software. BF-588-WT, red lines; BF-588-MonoCAB, blue lines; BF-588-DualCAB, green lines; pH6.0: solid line; pH7.4: dashed line. x-axis, antibody concentration (log nanomolar); y-axis, OD 450 nm. (d) Potency of BF-588-WT, BF-588-MonoCAB, and BF-588-DualCAB in pH affinity ELISA of Human EpCAM and CD3 at pH6.0 and pH7.4.EC50, half-maximal effective concentration.

Antibodies against human EpCAM were developed by immunizing BALB/c mice (Genscript) with a recombinant human EpCAM extracellular domain (huEpCAM-ECD). Variable domains from hybridomas exhibiting binding activities to recombinant human and cynoEpCAM extracellular domain (ECD), as well as human and cynomolgus EpCAM expressed on the cell surface, were cloned, and further characterized for their binding and in vitro functional activities. Hybridoma clone BA-105-12C10F12 was selected for the CAB process.Citation14
Acidic extracellular pH is a key characteristic of the TME because of the glycolytic metabolism of cancer cells that underpins the continuous replication of cancer cells.Citation15 A library of variants of clone BA-105-12C10F12 with mutations introduced in all six CDR loops was screened for binding to recombinant human EpCAM ECD at pH6.0 (TME condition) and pH7.4 (normal alkaline physiological condition). Screening yielded several clones with the desired properties (low binding at pH7.4; high binding at pH6.0). One of these conditionally active anti-EpCAM variants was humanized. A panel of CAB anti-CD3 scFv fragments was fused to the C terminus of the light chain of the humanized clone to create a library of anti-EpCAM × CD3 bispecific antibodies with conditional binding to EpCAM and CD3. One of these anti-EpCAM × CD3 bispecific antibody clones, clone BF-588-DualCAB (CAB function at both EpCAM and CD3 binding domains), is described in detail here and compared to clones BF-588-MonoCAB (CAB function only at the CD3 binding domain) and BF-588-WT (no CAB function).
In vitro characterization of BF-588-WT, MonoCAB and DualCAB
The binding affinity to recombinant human EpCAM and human CD3 at different pH was determined by sandwich ELISA. Both BF-588-MonoCAB and BF-588-DualCAB showed strong binding to human EpCAM and human CD3 at a pH6.0. The binding activity decreased when the pH in the assay increased, and very little binding signal was observed at pH7.4 (). BF-588-MonoCAB showed similar pH-dependent binding compared to BF-588-DualCAB, the pH inflection point (pH with 50% signal compared to pH6.0, which was set as 100%) was at pH6.6, while the binding of BF-588-WT (no CAB) was independent of the pH of the assay solution (). The EC50 of BF-588-DualCAB was 1.52 nM at pH6.0 and increased ~ 6-fold to 9.09 nM at pH7.4. BF-588-MonoCAB showed an increase in EC50 from 0.35 nM at pH6.0 to 3.14 nM at pH7.4, while the EC50 of the wild type clone (BA-588-WT, no CAB function) did not change significantly (EC50 was 0.61 nM at pH6.0 and 0.40 nM at pH7.4) ()). In addition to the increase in EC50, the maximum signal for clones BF-588-MonoCAB and BF-588-DualCAB drops at pH7.4 compared to pH6.0 ().
BA-588-DualCAB shows similar pH-dependent binding to EpCAM and CD3 from cynomolgus monkey, but does not cross-react with canine, rat, or mouse proteins (Fig. S1A). In addition, no binding to Trop2, the only other member of the EpCAM gene family,Citation16 was detected (Fig. S2C)
We previously demonstrated that CAB selectivity affects both affinity and binding due to PaCS pH-dependent blocking; therefore, surface plasmon resonance (SPR) measurements were used to determine both characteristics.Citation14
The binding kinetics for clone BF-588-DualCAB were determined by SPR (). Varying antibody concentrations were injected over a sensor surface with an immobilized human EpCAM ECD or human CD3ε/δ heterodimer. Experiments were carried out at pH6.0, pH6.5, and pH7.4 with 30 mM of the PaCS molecule sodium bicarbonate in the assay solution. The results showed that clone BF-588-Dual-CAB has a dissociation constant (KD) to human EpCAM of 1.3 nM at pH6.0, 1.22 nM at pH6.5, and 6.73 nM at pH7.4. For human CD3ε/δ the affinity was determined as 8.1 nM at pH6.0, 9.1 nM at pH6.5, and 35 nM at pH7.4 (). In addition to the change in KD, we also observed a sharp drop in the maximum SPR signal from pH6.0 to pH7.4 (from ~100 resonance units [RU] to ~ 25 RU for human EpCAM, and from ~ 100 RU to ~ 10 RU for human CD3ε/δ (), which is characteristic of CABs. We have previously shown that this signal drop is caused by a change in the reversible availability of the reactive ligands on the sensor surface.Citation14 The availability of reactive ligands is caused by the interaction of the proteins with PaCS molecules, i.e., bicarbonate ions, which block the binding of BF-588-DualCAB in a concentration-dependent manner. Therefore, the pH selectivity is a combination of the change in KD and the fold change in the maximum signal observed. BF-588-DualCAB also showed pH-dependent binding characteristics cynoEpCAM and cynoCD3, as measured by SPR analysis ( and Figure S2).
Figure 2. Binding kinetics of clone BF-588-DualCAB to human EpCAM (left) or human CD3 (right) at pH6.0 (top), pH6.5 (middle) and pH7.4 (bottom). Shown are the SPR sensorgrams of one representative experiment at each pH. The binding to human EpCAM is similar at pH6.0 and pH6.5 (1.3 nM and 1.22 nM, respectively), but drops to 6.7 nM at pH7.4. In addition, the SPR signal drops from ~ 100 RU at pH6.0 to ~ 30 RU at pH7.4. The binding affinity to human CD3 drops from 8.1 nM at pH6.0 to 9.1 nM at pH6.5 and 35 nM at pH7.4. In addition, the SPR signal drops from ~ 100 RU at pH6.0 to ~ 20 RU at pH7.4. X axis, Time [s]; Y axis, response units [RU]. (a) Binding kinetics of clone BF-588-DualCAB to human EpCAM (left) or human CD3(right) at pH6.0 (top), pH6.5 (middle) and pH7.4 (bottom). Shown are the SPR sensorgrams of one representative experiment at each pH. The binding to human EpCAM is similar at pH6.0 and pH6.5 (1.3 nM and 1.22 nM, respectively), but drops to 6.7 nM at pH7.4. In addition, the SPRsignal drops from ~ 100 RU at pH6.0 to ~ 30 RU at pH7.4. The binding affinity to human CD3 drops from 8.1 nM at pH6.0 to 9.1 nM at pH6.5 and 35 nM at pH7.4. In addition, the SPR signal drops from ~ 100 RU at pH6.0 to ~ 20 RU at pH7.4. X axis, Time [s]; Y axis, response Units[RU]. (b) Measured binding affinities of clone BF-588-DualCAB to human and cynomolgusEpCAM and CD3 at pH6.0, pH6.5, and pH7.4 (average of three experiments).
![Figure 2. Binding kinetics of clone BF-588-DualCAB to human EpCAM (left) or human CD3 (right) at pH6.0 (top), pH6.5 (middle) and pH7.4 (bottom). Shown are the SPR sensorgrams of one representative experiment at each pH. The binding to human EpCAM is similar at pH6.0 and pH6.5 (1.3 nM and 1.22 nM, respectively), but drops to 6.7 nM at pH7.4. In addition, the SPR signal drops from ~ 100 RU at pH6.0 to ~ 30 RU at pH7.4. The binding affinity to human CD3 drops from 8.1 nM at pH6.0 to 9.1 nM at pH6.5 and 35 nM at pH7.4. In addition, the SPR signal drops from ~ 100 RU at pH6.0 to ~ 20 RU at pH7.4. X axis, Time [s]; Y axis, response units [RU]. (a) Binding kinetics of clone BF-588-DualCAB to human EpCAM (left) or human CD3(right) at pH6.0 (top), pH6.5 (middle) and pH7.4 (bottom). Shown are the SPR sensorgrams of one representative experiment at each pH. The binding to human EpCAM is similar at pH6.0 and pH6.5 (1.3 nM and 1.22 nM, respectively), but drops to 6.7 nM at pH7.4. In addition, the SPRsignal drops from ~ 100 RU at pH6.0 to ~ 30 RU at pH7.4. The binding affinity to human CD3 drops from 8.1 nM at pH6.0 to 9.1 nM at pH6.5 and 35 nM at pH7.4. In addition, the SPR signal drops from ~ 100 RU at pH6.0 to ~ 20 RU at pH7.4. X axis, Time [s]; Y axis, response Units[RU]. (b) Measured binding affinities of clone BF-588-DualCAB to human and cynomolgusEpCAM and CD3 at pH6.0, pH6.5, and pH7.4 (average of three experiments).](/cms/asset/04ea64ec-8bd4-460f-8b8a-661de36411ab/kmab_a_2322562_f0002_oc.jpg)
Target-dependent T-cell activation
The ability of BF-588-DualCAB to activate TCR signaling and induce T cell activation in vitro was investigated using a T cell activation bioassay. Engineered Jurkat T cells were used as reporter cells, and HCT116, Chinese hamster ovary (CHO)-huEpCAM and naïve CHO cell lines were used as target cells. BF-588-DualCAB engaged in the TCR/CD3 complex and activated the reporter cells in a pH-dependent manner (). The wild-type EpCAM x CD3 bispecific antibody without CAB function, clone BF-588-WT, engaged the TCR/CD3 complex and induced the activation of the reporter cells independent of the pH (). EpCAM × CD3 bispecific antibodies did not activate the reporter cells in the absence of EpCAM-expressing target cells (). The calculated EC50 values for each antibody are shown in . The results showed that BF-588-MonoCAB and BF-588-DualCAB were ~ 4–6-fold more potent in inducing the activation of Jurkat reporter cells under conditions that mimic the TME (pH6.0), compared to the normal physiological alkaline condition (≥pH7.4). Under TME conditions, the potency of the BF-588-DualCAB antibody in inducing reporter cell activation via the TCR/CD3 complex against HCT116 cancer cells was similar to that of BF-588-WT (). Under physiological conditions (pH7.4), a marked decrease in the potency of BF-588-DualCAB was observed, EC50 value of 342.5 pM at pH7.4 versus 76.54 pM at pH6.0. No change in BF-588-WT EC50 values at pH6.0 and pH7.4 was observed, 58.44 pM and 58.87 pM, respectively. BF-588-DualCAB was more potent than the BF-588-WT antibody in engaging TCR/CD3 complex in the presence of CHO-huEpCAM at pH6.0, EC50 values of 24.35 pM versus 148.1 pM, but with similar potency at pH7.4, EC50 values of 144.3 pM for BF-588-DualCAB and 190 pM for BF-588-WT. These results showed that both BF-588-MonoCAB and BF-588-DuaCAB were less potent in inducing reporter cell activation via the TCR/CD3 complex at physiological pH.
Figure 3. In vitro functional characterization of BF-588-DualCAB. Target-dependentT-cell activation and cytotoxicity of EpCAM-expressing cells. EpCAM × CD3-based bispecific antibodies induce in vitro activation ofT cells in the presence of EpCAM-expressing target cells. (a) Jurkat/NFAT/NFAT-luc reporter cells were incubated with human EpCAM-expressing cells and serial dilutions of BF-588-WT (red curve), BF-588-MonoCAB (bluecurve) and BF-588-DualCAB (green curve) bispecific antibodies at pH6.0 and pH7.4. Isotype control shown in black. HCT116: human colorectal cancer cell line; CHO-huEpCAM: CHO cells stably expressinghuEpCAM antigen. x-axis, log antibody concentration in picomolar(pM); y-axis, relative luciferase units (RLU).

Figure 3. (b) Potency of BF-588-WT, BF-588-MonoCAB and BF-588-DualCAB in mediating T cell activation when cocultured with HCT116 and CHO-huEpCAM cells at pH6.5 and pH7.4. EC50, half-maximal effective concentration in picomolar (pM). (c) Effector PBMCs,PBMCs, E:T ratio 5:1, were co-cultured with HCT116 cells and treated with serially diluted BF-588-DualCAB or BF-588-WT antibodies for 80 hours. Co-cultures were incubated in culture media under TME (pH6.5) or physiological (pH7.4) conditions. Cytolysis was monitored in real time using Agilent x Celligence real time cell analysis technology(RTCA). The rate of target cell cytolysis was calculated by referencing the target cell growth without treatment. x-axis, Logantibody concentration in picomolar (pM); y-axis, % of Cytolysis. (d) Potency of BF-588-DualCAB and BF-588-WT in inducing cytotoxicity of HCT116 cells by human PBMCs at pH6.5 and pH7.4.EC50, half-maximal effective concentration. pM, picomolar. BF-588-WT: red lines; BF-588-MonoCAB: blue lines; BF-588-DualCAB: green lines; pH6.0 or pH6.5: solid line; pH7.4: dashed line.

BF-588-Dual-CAB mediates T cell-mediated cytolysis of EpCAM-expressing cells
The functional activity of BF-588-DualCAB was further characterized and compared to BF-588-WT using CD3-mediated peripheral blood mononuclear cells (PBMC) killing of the target cells. Human PBMCs were co-cultured for 80 h with HCT116 cells and various concentrations of BF-588-DualCAB. Cell growth was determined using xCELLigence Real-Time Cell Analysis technology. Cytotoxicity was determined using the following equation:
The results showed that BF-588-DualCAB was more potent in inducing human PBMC (huPBMC)-mediated cytotoxicity of HCT116 cells in the tumor environment (acidic pH, pH6.5) and less active at normal physiological alkaline pH (pH7.4) (). BF-588-WT was more potent in promoting cancer cell killing by human PBMC in physiological pH (pH7.4). The EC50 of BF-588-DualCAB in TME pH (pH6.5) was 0.24 pM and 2.2 pM at pH7.4. The EC50 of BF-588-WT in TME pH (pH6.5) was 0.06 pM and 0.01 pM at pH7.4.
BF-588-DualCAB was over 200-fold less active in this assay compared to the non-CAB BF-588-WT molecule under physiological conditions. No cytolysis was observed when using the isotype control bispecific antibody in this assay and when EpCAM-non-expressing cells were used (data not shown). The difference in the activities of BF-588-DualCAB responses in the TME pH and in the physiological alkaline conditions was consistent with the expected results, as BF-588-DualCAB was engineered to increase T-cell activation in conditions mimicking the TME.
BF-588-MonoCAB and BF-588-DualCAB inhibit tumor growth in vivo
The anti-tumor activity of EpCAM × CD3 bispecific antibodies was evaluated in NOG mice inoculated with HCT116 cells and engrafted with non-activated huPBMCs from healthy subjects. Mice were dosed intravenously (i.v.) with BF-588-WT, BF-588-MonoCAB, BF-588-DualCAB and Isotype × WT CD3 bispecific antibodies at 1 mg/kg (mpk) twice a week for four weeks. Treatment was initiated when the tumor volume was approximately 130 mm3. All antibodies completely eradicated established tumors in vivo (p ≤ 0.001; Dunnett’s test) (). In vivo efficacy of BF-588-DualCAB was also tested in a BT474 CDX humanized mouse model. BT474 cells were grafted with non-activated huPBMCs from healthy donors in the mammary fat pads of NCG mice. When tumor volumes reached ~90 mm3, mice were dosed twice a week for four weeks with 0.5 mpk of BF-588-DualCAB or Isotype x WT CD3 control antibodies. Treatment of mice with BF-588-DualCAB significantly inhibited tumor growth, with a TGI of 106.8% (p ≤ 0.001) on day 27 post-dose ().
Figure 4. Invivoefficacy of EpCAM T-cell engagers. EpCAM× CD3 bispecific antibodies promote anti-tumor immunity in a cell line-derived xenograft humanized mouse model of human colorectal and breast cancers. (a-c) HCT116 human colon carcinoma was implanted in NOG mice. huPbmcs were inoculated intraperitoneally 2 hours after tumor inoculation. Animals were randomized to treatment groups when tumor volume reached approximately 130 mm3. Following randomization, animals were dosed i.V. With isotype x CD3WT control antibody or BF-588-WT (A), BF-588-MonoCAB (B) and BF-588-DualCAB (C) at 1mpk twice per week for four weeks. (d) NCGmice were implanted with estrogen pellets on day 0. On day one animals were implanted with a mixture of BT474 cells and huPbmcs(1:1) subcutaneously in PBS-Matrigel at the right mammary fat pad. Animals were randomized, and treatment was initiated when tumor volume was approximately 95 mm3.Mice were administered i.V. With BF-588-DualCAB or isotype x WT CD3 control bispecific antibodies at 0.5 mpk twice per week for four weeks. mpk: milligram per kilogram. x-axis:Days after treatment; y-axis mean tumor volume ± SEM. BF-588-WT:red line; BF-588-MonoCAB: blue line; BF-588-DualCAB: green line.

BF-588-DualCAB shows significant reduction of immunotoxicity and highly increased tolerability in cynomolgus monkeys
The safety profile of EpCAM × CD3 bispecific antibodies was evaluated in a single-dose and repeated-dose toxicity study in cynomolgus monkeys. In the single-dose toxicity study, monkeys (2 animals/group, 1 male and 1 female) were administered BF-588-WT at 0.05 mpk; BF-588-MonoCAB at 0.05, 0.125, 0.25, 0.5 mpk and BF-588-DualCAB at 0.05, 0.5, 2.5 mpk as an i.v. bolus injection. A summary of EpCAM × CD3 bispecific antibody-related toxicities is shown in . Assessment of tolerability and pharmacological activity in the animals was based on clinical observations, qualitative food consumption, body weight, vital signs, and clinical and anatomic pathology upon completion of the experiment. Blood samples were collected for cytokine profiling and immunophenotyping analysis using flow cytometry.
Figure 5. Cytokine release and tolerability of EpCAM x CD3 bispecific antibodies in cynomolgus monkeys. Cytokinerelease and tolerability of EpCAM × CD3 bispecific antibodies in a single- and repeat-dose toxicity study in cynomolgus monkeys. Clinical outcomes of the maximum tolerated or maximum tested doses of BF-588-WT, BF-588-MonoCAB, and BF-588-DualCAB in a single-dose non-GLP toxicity study in cynomolgus monkeys and of the maximum tested dose of BF-588-DualCAB in a repeat-dose GLP toxicity study in cynomolgus monkeys. (a) Cytokine release in cynomolgus monkeys treated with a single dose of BF-588-WT (0.05 mpk, red bars), BF-588-MonoCAB (0.25 mpk = MTD, blue bars) or BF-588-DualCAB (2.5 mgk= max. dose tested, green bars). Cytokine concentration in the monkey serum was measured 0–24 hours after treatment as indicated.IL-2: Interleukin-2; IL-6: interleukin-6 and MCP-1: monocyte chemoattractant protein-1.X-axis: time after dosing in hours; y-axis: cytokine concentration in pg/mL. (b) Summaryof EpCAM x CD3 bispecific antibodies-related toxicity and clinical outcome. Major clinical findings in cynomolgus monkeys treated with the maximum tolerated dose or maximum dose of BF-588-WT,BF-588-MonoCAB and BF-588-DualCAB in a single-dose toxicity study and in a 1-month repeat-dose once-weekly dosing (five doses total) of BF-588-DualCAB in cynomolgus monkeys. (c) Increase in tolerability of CAB EpCAM T-cell engagers in cynomolgus monkeys. BF-588-WT was not tolerated at 0.05 mpk (only dose tested). The MTD for BF-588-MonoCAB was determined to be 0.25 mpk. (5-fold improvement over the wild type). BF-588-DualCAB clone was very well-tolerated at 5 mpk and the MTD was not reached, indicating another ≥ 20-fold improvement over the MonoCAB and ≥ 100-fold improvement over the wild type EpCAM x CD3 TCE. x-axis: dose in mg/kg; y-axis: EpCAM x CD3bispecific antibodies. BF-588-WT, red bars; BF-588-MonoCAB, blue bars; BF-588-DualCAB, green bars. Abbreviations: MTD, maximum tolerated dose; MPK, milligrams per kilogram; BWL, bodyweight loss. a– number of animals.

Consistent with the expected pharmacological immune system activation by a CD3 TCE, cytokine release and T-cell activation were observed in monkeys treated with BF-588-WT (). BF-588-WT was not tolerated at 0.05 mpk. Animals treated with BF-588-WT showed more than 20% body weight loss accompanied by severe lethargy, emesis, liquid feces, and hunched posture, which led to the early termination of both female and male monkeys. Changes in clinical chemistry associated with BF-588-WT included moderate or marked elevations in alanine aminotransferase (ALT), aspartate aminotransferase (AST), alkaline phosphatase (ALP), and gamma glutamyl transferase (GGT) levels, as well as increased concentrations of total bilirubin, total cholesterol, and triglycerides. These findings are consistent with those of hepatocellular and hepatobiliary tissue injuries. Histological microscopic findings in these animals included mild to moderate periportal mixed inflammatory cell infiltration with edema, mild centrilobular hepatocyte vacuolation in the liver, moderate mucosal mixed inflammatory cell infiltration in the jejunum/ileum, moderately decreased lymphocyte cellularity in the cortex of the thymus, and minimal mineralization of the tubules in the cortex of the kidneys. Acute inflammatory changes in the liver and intestines were considered to be major contributors to the moribundity of early terminated animals.
BF-588-MonoCAB was well tolerated up to 0.25 mpk. At this concentration cytokines interleukin (IL)-6 and monocyte chemoattractant protein-1 (MCP-1) increased to similar levels as seen for BF-588-WT at 0.05 mpg (). In the monkeys treated with BF-588-MonoCAB, no clinical signs or changes in immunophenotype parameters were denoted. Serum chemistry revealed mildly elevated levels of ALT, AST, GGT and total bilirubin, accompanied by elevated levels of cholesterol and triglycerides. These findings were not associated with changes in clinical signs, vital signs, or body weight. Clinical chemistry returned to baseline by day 15, when the animals returned to the colony.
In this study, BF-588-DualCAB was well tolerated up to 2.5 mpk, the highest dose tested (10-fold higher compared to BF-588-MonoCAB and 50-fold higher compared to BF-588-WT (tested only at 0.05 mpk). There were no changes in vital signs, clinical pathology, or immunophenotyping parameters (total T cells, total and proliferating CD4+ and CD8+ T cells, and natural killer (NK) cells). In addition, no BF-588-DualCAB-related macroscopic or microscopic histological findings were noted.
IL-6, IL-2, and MCP-1 levels were elevated in animals treated with BF-588-WT or BF-588-MonoCAB. The increase in IL-6 levels in monkeys dosed with BF-588-MonoCAB was dose-dependent and reached similar levels detected in animals treated with 0.05 mpk of BF-588-WT, when BF-588-MonoCAB was dosed at five times higher dose levels of 0.25 mpk (). IL-6 concentrations in animals treated with 0.25 mpk BA588-MonoCAB went back to baseline at 24 h post-dosing, while it remained elevated in animals treated with 0.05 mpk BA588-WT (). Remarkably, minimal elevations in IL-6 were observed at 2 to 6 hours post-dose in animals administered BF-588-DualCAB, which returned to baseline or decreased by 24 h post-dose (). Even though levels of IL-6 and MCP-1 in animals treated with BF-588-MonoCAB at 0.25 mpk reached similar levels in animals treated with BA-588-WT at 0.05 mpk, it did not result in any visible clinically adverse outcome. The levels of IL-6 detected in animals dosed with BF-588-DualCAB were 20-to 50-fold lower than those observed in monkeys treated with BF-588-WT and BF-588-MonoCAB. Minimal to no release of IL-2 and MCP-1 proteins was observed in animals dosed with BF-588-DualCAB. IL-6 levels for all antibody concentrations tested in vivo are shown in Fig.S3.
A repeat-dose toxicity study in cynomolgus monkeys was also conducted to evaluate the safety profile of BF-588-DualCAB and the associated systemic exposure after one month of once-weekly dosing (five total doses). BF-588-DualCAB was administered as an i.v. bolus to male and female monkeys (6 animals/group, 3 males and 3 females) at 0 (vehicle control), 0.5, 1.5, and 5 mg/kg/week. Recovery animals (4 animals/group, 2 males and 2 females; 4-week recovery period) were included in the vehicle control and high-dose groups. BF-588-DualCAB was well tolerated up to the highest dose tested (5 mg/kg/week), with no mortality or morbidity. No BF-588-DualCAB related clinical signs were observed, and no macroscopic or microscopic changes were observed at the end of the 4-week recovery period (). No BF-588-DualCAB-related changes were noted in total T cells, helper and cytotoxic T cells, B cells, NK cells, activated CD69+ T cells, Ki-67+ T cells, or regulatory T cells. Additionally, changes in cytokine levels were present in the vehicle control group, dose independent and not present in both animals in the same group, therefore they were not considered BF-588-DaulCAB related (Figure S4).
Combined data from the single-dose and repeat-dose toxicology studies showed that the tolerability of BF-588-DualCAB (MTD ≥5 mpk) was improved by ≥ 100-fold compared to BF-588-WT (MTD ≤0.05 mpk) (). This synergistic effect demonstrates the versatility and potential to improve the therapeutic index of the CAB technology.
Pharmacokinetics of EpCAM x CD3 bispecific antibodies in cynomolgus monkeys
The pharmacokinetics (PK) profile of EpCAM x CD3 bispecific antibodies in cynomolgus monkeys (2 animals/group) was determined after a single i.v. dose of BF-588-WT at 0.05 mpk; BF-588-MonoCAB at 0.05, 0.125 and 0.25 mpk and BF-588-DualCAB at 0.05, 0.5, and 2.5 mpk. The Cmax, AUClast, AUCinf, t1/2, and Tmax from the non-compartmental analysis of the PK data are presented in (Table S1). No meaningful differences in exposure were observed between male and female monkeys that received the same test article at the same dose. BF-588-WT half-life values were 14.8 and 17.4 hours for male and female monkey, respectively. BF-588-MonoCAB half-life ranged from 5.7–18.6 hours and BF-588-DualCAB half-life ranged from 29.6 to 42.2 hours across the different dose levels. Over the 5-fold and 50-fold dose range (0.05 to 0.25 mpk for BF-588-MonoCAB and 0.05 to 2.5 mpk for BF-588-DualCAB), exposure to BF-588-MonoCAB and BF-588-DualCAB based on Cmax and AUClast generally increased in a dose-proportional manner (Table S1).
The PK profile of BF-588-DualCAB in cynomolgus monkeys was also determined after weekly i.v. doses of 0.5, 1, and 5 mpk on days 1, 8, 15, 22, and 29 (Fig. S5). There was no significant difference in serum PK exposure to BF-588-DualCAB between male and female cynomolgus monkeys after the first dose or repeat dosing. The PK exposure (both Cmax and AUC) of BF-588-DualCAB increased in a dose-dependent manner from 0.5 mpk to 1.5 mpk and 5 mpk after both, the first dose and four repeat doses. The linear PK characteristics of BF-588-DualCAB were also demonstrated by similar systemic clearance (~1 mL/h/kg) across the 10-fold dose range. The mean terminal phase t1/2 was 53.3, 50.7, and 54.9 hours after the first dose and 51.6, 145.0, and 81.9 hours after repeat doses on Day 22 for BF-588-DualCAB doses of 0.5, 1.5, and 5 mpk, respectively.
The incidence of anti-BF-588-DualCAB antibodies (ADA) was very low in cynomolgus monkeys. Of 22 treated animals, one male monkey in the 1.5 mg/kg group and one female monkey in the 5 mg/kg had preexisting ADA and remained positive throughout the study. PK of BF-588-DualCAB in these monkeys were still within the range of ADA-negative monkeys. Only one female animal in the 1.5 mg/kg group with no preexisting antibodies against BF-588-DualCAB tested ADA positive on Day 29. AUC(0-t) of BF-588-DualCAB in this female animal was approximately 20% lower on Day 22 and 75% lower on Day 29 than the ADA-negative females, while Cmax was not affected on Day 22 but 47% lower on Day 29 in this animal. Overall systemic exposure of BF-588-DualCAB did not appear to consistently be affected by the presence of ADA in cynomolgus monkeys.
Discussion
EpCAM is overexpressed in a wide range of tumors, making it an ideal target for pan-cancer therapies. However, it is also widely expressed in healthy epithelial tissues. EpCAM has been clinically validated using locally administered catumaxomab (EpCAM × CD3 bispecific antibody),Citation17 which has shown good efficacy. However, systemic administration of catumaxomab led to severe CRS and hepatotoxicity. Similarly, clinical trials with solitomab were terminated before reaching efficacious concentrations due to toxicity.Citation12
TCEs are very potent molecules that redirect the cytotoxic activity of T cells in the absence of MHC restriction and are an emerging new class of cancer therapies. TCEs are designed to recruit T cells via a common signaling molecule, such as CD3, against tumor cells bearing a frequently expressed tumor-associated antigen (TAA). To date, twelve TCEs have been approved; nine for hematological cancers targeting BCMA, CD19, CD20, or GPCR5D, and only three for the treatment of solid tumors targeting EpCAM, gp100 and DLL3 (The Antibody Society. Therapeutic monoclonal antibodies approved or in regulatory review. (02/19/2024); www.antibodysociety.org/antibody-therapeutics-product-data).
TCEs targeting solid tumors require much higher peripheral levels to achieve efficacious concentrations inside solid tumor tissueCitation18,Citation19 which can lead to severe on-target off-tumor toxicities. Since TCEs can recognize very low levels of TAAs, it is not uncommon to observe on-target, off-tumor toxicities for TAAs expressed in normal tissues.Citation8, Citation13, Citation20–22 This has hampered the development of bispecific antibodies using CD3-directed TCEs against targets, such as EpCAM, which are widely expressed in normal tissues. The only approved TCE targeting EpCAM, Catumaxomab, has limited application due to severe toxicityCitation10 and it has been withdrawn for the market for commercial reasons.
We used our CAB technologyCitation14 to develop conditionally active EpCAM-specific TCEs that bind to their respective targets only under TME conditions. CAB antibodies and CAB bispecifics have optimized binding domains that have no or greatly reduced binding in normal tissue and preserve high-affinity binding in the TME without covalent modification of the antibody requiring enzymatic activity for activation. Since CAB antibody binding is controlled by extracellular pH and the respective conditional binding of the PaCS molecules, it becomes inactive when leaving the TME for the normal alkaline microenvironment and active once it reenters the acidic TME again.Citation14
A wide range of bispecific antibody formats have been described in the literature.Citation23 These formats vary in size from 25 kDa (nanobodies) to 300 kDa (chemically linked IgGs) and can generally be grouped into hetero-dimeric and homo-dimeric molecules. We elected to use a homo-dimeric format based on an IgG1 backbone (targeting the TAA) and an scFv domain fused to the C-terminus of the light chain for binding to CD3. The presence of an Fc allows purification of bispecific antibodies using protein A as the initial step, similar to a standard IgG molecule. The addition of the CAB-CD3 binding domain to the C-terminus of the light chain allows for the easy conversion of any antibody into a MonoCAB (CAB function at CD3 binding domain) TCE without additional engineering, thus creating a platform for the rapid generation of MonoCAB TCE against any tumor target.
In this report, we describe the development of a dual CAB EpCAM × CD3 bispecific antibody, BF-588-DualCAB, and compare its properties with those of a MonoCAB version (BF-588-MonoCAB) that has conditionally active binding only on the CD3-binding domains, and the parental non-CAB molecule, BF-588-WT.
Clones BF-588-DualCAB and BF-588-MonoCAB showed tumor-selective binding to human and cynomolgus EpCAM and CD3 in vitro, with a pH inflection point at pH6.6 ( = 50% binding signal compared to pH6.0) and very little binding at normal physiological alkaline pH (≥pH7.4) in an affinity ELISA assay. The binding of BF-588-WT was similar under all the pH conditions tested. Binding kinetics analyzed by SPR showed a decrease in both affinity from pH6.0 to pH7.4, and a concomitant decrease in the maximum signal, similar to other CABs described previously.Citation14 BF-588-DualCAB and BF-588-MonoCAB demonstrated the ability to induce T cell activation and killing of cancer cells in vitro in a pH-dependent manner, while the non-CAB parental clone showed activity independent of the pH conditions.
All three clones were tested in a cell line-derived xenograft (CDX) humanized mouse model using HCT116 human colon cancer cells in NOG mice engrafted with human PBMCs. Treatment with BF-588-WT, BF-588-MonoCAB, or BF-588-DualCAB at 1 mpk (twice weekly for four weeks) induced 100% tumor regression in this model. BF-588-DualCAB also inhibited the tumor growth of a BT474 human breast cancer cell line in NCG mice humanized with human PBMCs at 0.5 mpk (twice weekly for four weeks).
The immunotoxicity and tolerability of these molecules were tested in a single-dose toxicity study in cynomolgus monkeys. As expected, the non-CAB clone BF-588-WT proved to be very toxic and was not tolerated at 0.05 mpk (only dose tested). Cytokine levels increased dramatically within six hours of administration, and the animals were euthanized. BF-588-MonoCAB showed an improved toxicity profile and was well-tolerated up to 0.25 mpk. At this dose cytokine levels increased to similar levels as observed with the non-CAB molecule at 0.05 mpk, but the animals showed only minimal elevation of liver enzymes and had no clinical symptoms. Clone BF-588-DualCAB was tested up to 2.5 mpk and later, in a repeat dose study up to five mpk. Despite its robust ability to activate T cells and eliminate EpCAM-expressing target cells at a similar minimal efficacious dose as the non-CAB and MonoCAB clones, BF-588-DualCAB had an extremely low immunotoxicity profile. Cytokine levels were slightly elevated at 2.5 mpk (IL-6) or barely above the baseline (IL-2 and MCP-1). In the repeat dose study animals were dosed with BF-588-DualCAB up to 5 mg/kg/week for 4 weeks and no mortality or morbidity, no macroscopic or microscopic changes were observed, and no cytokine-related AEs were observed, indicating that the maximum tolerated dose for BF-588-DualCAB was greater than five mpk.
Our data show that the tolerability of EpCAM-specific TCE is improved by more than five-fold by using a conditionally active CD3-binding domain in combination with a non-CAB EpCAM-binding domain. The full potential improvement in safety and tolerability, however, is reached in the DualCAB format, which can be dosed at > 20-fold higher concentrations compared to MonoCAB and > 100-fold higher concentrations compared to the non-CAB parent.
The current results clearly demonstrate that BF-588-DualCAB represents a novel, potentially highly effective targeted agent for the treatment of cancer patients with EpCAM-overexpressing tumors. Recently, our team initiated a Phase 1 clinical trial using this synergistic, DualCAB TCE (named BA3182) to evaluate the potential of this therapeutic candidate as a pan-cancer therapy.
Materials and methods
Antibody engineering and screening
Antibodies against human EpCAM (huEpCAM) were generated by immunizing mice with a huEpCAM hexa-histidine-tagged ECD (huEpCAM ECD-His, Sinobiological catalog no. 10694-H08H). The hybridoma clones were screened for binding to recombinant huEpCAM ECD-His and CHO cells (RRID:CVCL_0213) expressing human EpCAM (BioAtla). Variable domains of selected hybridoma clones were sequenced, cloned into a human IgG1/kappa backbone containing the N297Q mutation to generate non-glycosylated chimeric antibodiesCitation24,Citation25 and screened again to confirm their binding activities. Chimeric clone BA-105-12C10F12 was selected for further engineering. Single amino acid variant libraries of BA-105-12C10F12 were constructed, expressed as full-length IgGs in CHO cells, and screened for conditional binding activity under TME conditions as previously described for CAB antibody generation.Citation14 One of the chimeric CAB variants was humanized using BioAtla’s proprietary Express HumanizationTM protocol. A library of conditionally active anti-CD3-scFv (BioAtla) was then fused to the C-terminus of the kappa light-chain domain of the humanized CAB variant. The resulting library of DualCAB TCE molecules was screened for binding to human and cynomolgus (cyno) EpCAM (BioAtla) and human CD3ε/δ heterodimer (BioVision catalog no. P1183) using pH affinity ELISA and T-cell activation bioassays (see below for the methods). The clones used in this study were BF-588-WT (non-CAB TCE binding to EpCAM and CD3), BF-588-MonoCAB (conditional binding to CD3), and BF-588-DualCAB (conditional binding to EpCAM and CD3).
Cell lines and cell culture
HCT116 (ADCC, catalog no. CCL-247), a human colorectal cancer cell line, was cultured in McCoy’s 5A medium (catalog no.16600–082) supplemented with 10% FBS (Gibco, catalog no. 16140–071). CHO-S (Gibco, catalog no. R80007) was cultured in DMEM (Gibco, catalog no. 11965–084) supplemented with 10% fetal bovine serum (FBS). CHO cells expressing human EpCAM ECD (CHO huEpCAM) or cynomolgus EpCAM ECD (CHO cynoEpCAM) on the cell surface were created at BioAtla and cultured in DMEM supplemented with 10% FBS and 1 mg/mL G418 (Invitrogen, catalog no ant-gn-5). Human frozen PBMC (Precision for Medicine, catalog no. 93000-10 M). All cells were maintained at 30°C and 5% CO2 in a humidified atmosphere.
pH affinity ELISA
The human CD3ε/δ heterodimer (Biovision, catalog no. P1183) was immobilized in the wells at 0.5 µg/mL overnight at 4°C (100 µL/well). Plates were blocked with either pH6.0 ELISA assay solution (phosphate-buffered saline (PBS) with 2.5 g/L sodium bicarbonate, 10 g/L bovine serum albumin (BSA), pH6.0) or pH7.4 ELISA assay solution (PBS with 2.5 g/L sodium bicarbonate, 10 g/L BSA, pH7.4) at room temperature for one hour, and then washed with the corresponding pH ELISA assay solution. BF-588-WT, BF-588-MonoCAB, and BF-588-DualCAB antibodies were serially diluted in the corresponding pH ELISA assay solutions and added to the wells. ELISA plates containing diluted antibodies were sealed and incubated at room temperature for one hour with shaking. The plates were then washed three times with the corresponding pH ELISA solution. Next, 100 µL of human EpCAM ECD fused to mouse Fc protein (BioAtla) was added to each well at a concentration of 1 µg/mL, diluted in the corresponding pH ELISA assay solution. The plates were sealed and incubated at room temperature for one hour with constant shaking. Following incubation, the plates were washed three times with the corresponding pH ELISA assay solution, and 100 µL of anti-mouse IgG antibody horseradish peroxidase (HRP) secondary antibody (Promega, catalog no. W402B, diluted 1:2,500 in the corresponding pH ELISA solution) was added to each well. The plates were then sealed and incubated at room temperature for one hour with shaking. Following incubation, the plates were washed thrice with the corresponding pH ELISA assay solution. TMB Peroxidase substrate solution (50 µL/well, Life Technology, catalog no. 002023) was added to each well and the reaction was stopped after 5 min with 50 µL of 0.1N HCl (Beijing Reagent, catalog no. G81788B). The OD at 450 nm was measured using a Microplate Spectrophotometer (ThermoFisher, Multiska™ Sky 51119770DP). EC50 values for binding to huCD3/huEpCAM-mFc or huCD3/cynoEpCAM-mFc at pH6.0 and pH7.4 were determined using the nonlinear fit model (variable slope, four parameters) of GraphPad Prism version 7.03.
pH range ELISA
The binding activity of BF-588-WT, BF-588-MonoCAB, and BF-588-DualCAB was tested in a range of pH ELISA assay conditions mimicking a TME pH (pH6.0, pH6.7) and the normal alkaline physiological pH (≥pH7.4). The human CD3ε/δ heterodimer (Biovision, catalog no. P1183) was immobilized in the wells at 0.5 µg/mL overnight at 4°C (100 µL/well). Plates were blocked with various pH ELISA assay solutions (PBS with 2.5 g/L sodium bicarbonate and 10 g/L BSA at pH6.0, 6.2, 6.5, 6.7, 7.0, and 7.4) at room temperature for one hour, and then washed with the corresponding pH ELISA assay solutions. BF-588-WT, BF-588-MonoCAB, and BF-588-DualCAB antibodies were diluted in the corresponding pH ELSA assay solutions (0.125 nM final concentration) and added to the wells. ELISA plates were sealed and incubated at room temperature for one hour with shaking. The plates were washed thrice with the corresponding pH ELISA assay solutions. Binding of EpCAM × CD3 bispecific antibodies was detected as described in the pH affinity ELISA protocol using human EpCAM ECD fused to the mouse Fc protein and anti-mouse IgG HRP secondary antibody (Promega, catalog no. W402B). TMB Peroxidase substrate was added to the wells and the enzymatic reaction was stopped after 5 min by addition of 0.1 N HCL. The optical density (OD) at 450 nM was measured using a Microplate Spectrophotometer. The amount of bispecific antibodies bound to both the human CD3 complex and the human EpCAM antigen under the different pH assay conditions was proportional to the OD at 450 nm. The pH inflection points of BF-588-MonoCAB and BF-588-DualCAB, which demonstrated 50% binding activity at varying pH compared to pH6.0 (set as 100%) were determined using the nonlinear fit model (variable slope, four parameters) of GraphPad Prism (RRID:SCR_002798)
Kinetic analysis of pH-dependent binding of BF-588-DualCAB
The binding kinetics of clone BF-588-DualCAB to EpCAM and CD3 were analyzed using SPR on an SPR32 Pro instrument (Bruker). Briefly, varying antibody concentrations were injected over a sensor surface with immobilized huEpCAM, cynoEpCAM, human CD3ε/δ heterodimer, or cynomolgus CD3ε/δ heterodimer. Binding kinetics were analyzed using a 1:1 model with analysis software (Sierra Analyzer R3, Bruker).
T-cell activation bioassay
A T-cell Activation Bioassay (Promega, catalog no. J1621) was used to evaluate the functional activity of BF-588-WT, BF-588-MonoCAB and BF-588-DualCAB antibodies. To this end, target cell lines, HCT116, CHO cells expressing human EpCAM, and naive CHO cells were seeded at a density of 4 × 104 cells/well in culture media overnight at 37°C with 5% CO2. The next day, BF-588-WT, BF-588-MonoCAB, BF-588-DualCAB and Isotype x WT CD3 control antibodies (anti-hen egg lysozyme x anti-CD3 wild type antibody, Evitria) were serially diluted in DMEM 10% FBS at pH6.0 and pH7.4. Engineered Jurkat TCR/CD3 cells that express a luciferase reporter driven by NFAT-response element were thawed and then resuspended to 4 × 106 cells/mL in DMEM 10% FBS at pH6.0 and pH7.4. Media of the cells was removed; 25 µL of diluted antibodies at each respective pH were added to the cells, and 25 µL of TCR/CD3 effector cell suspension at pH6.0 and pH7.4 was added to the same wells at each respective pH. Co-cultures of target and effector cells were incubated with BF-588-WT, BF-588-MonoCAB or BF-588-DualCAB antibodies at 37°C 5% CO2 for 6 hours. After this period, 50 µL/well of Bio-Glo reagent was added to each well, cultures were incubated for 5 min at room temperature. Luminescence signal was quantified using SpectraMax-i3X plate reader (Molecular Devices). Data was analyzed using GraphPad Prism Software 7.03
Peripheral blood mononuclear cell-mediated cytolysis
Cytotoxicity of target cells mediated by huPBMCs in the presence of BF-588-WT, BF-588-MonoCAB and BF-588-DualCAB antibodies were determined using the XCELLigence Real-Time Cell Analysis (RTCA) technology (ACEA Biosciences). All steps of the killing assay were conducted in a E-Plate 96 PE (ACEA Biosciences, catalog no. 00300600910). Plates were first hydrated with 100 µL cell culture media. One hundred microliters of target cells (HCT116) resuspended in their growth media were added to the wells at a density of 4 × 104 cells/mL (4×103 cells/well). Plates were added to the xCELLigence RTCA MP instrument, and cell proliferation was monitored overnight at 37C with 5% CO2. huPBMCs were thawed, resuspended in PBMC media (RPMI, Gibco, catalog no.11875–085 + 10% FBS, Sigma, catalog no.12306C) and incubated overnight at 37C with 5% CO2. Next day, BF-588-WT, BF-588-MonoCAB, BF-588-DualCAB and isotype control antibodies were serially diluted in PBMC media at pH6.5 and pH7.4. The RTCA experiment was paused and media from the cells in culture was removed and 100 µL of serially diluted antibodies at each respective pH was added to the wells. huPBMCs were resuspended at 4 × 105 cells/mL in PBMC media at pH6.5 and pH7.4, and 100 µL of cell suspension was added to each pH well, respectively Plates were returned to the RTCA MP instrument, and the incubation was resumed. Cellular cytotoxicity was then monitored for 80 hr at 37C 5% CO2. Data was analyzed using RTCA PRO software and 4-parameter curves generated using Graph Pad Prism software 7.03.
In vivo antitumor and toxicity studies
All animal studies were conducted following Institutional Animal Care and Use Committee protocols. Efficacy of the bispecific antibodies with conditional binding to EpCAM and CD3 was evaluated in vivo in humanized mouse xenograft models of human colon (HCT116) and breast cancers (BT474) for tumor killing activity with human T cells.Citation26,Citation27 For the HCT116 human colon carcinoma model, female NOG mice (6–9 weeks old, 15–20 g) were inoculated with 5 × 106 HCT116 cells subcutaneously in the right front flank. Two hours after tumor cells inoculation, mice were engrafted intraperitoneally with non-activated 5 × 106 huPBMCs from healthy donors (Allcells, Beijing, China). When tumor volume was approximately 130 mm3, mice were randomized in different study groups and treatment with EpCAM x CD3 bispecific antibodies was initiated. For the BT474 human breast cancer model, NCG mice (6–9 weeks old, 15–20 g) were implanted with estrogen pellets (17β-estradiol, 0.36 mg/60 days release) at the right flank one day before the tumor inoculation (day 0). On day one, a mixture of 8 × 106 BT474 tumor cells and 8 × 106 nonactivated huPBMCs from healthy subjects was inoculated subcutaneously in PBS-Matrigel at the right mammary fat pad. Animals were randomized, and treatment was initiated when tumor volume was approximately 95 mm3. Two or more efficacy studies were carried out to confirm BF-588-DualCAB’s anti-tumor activity using 8 mice/group.
The potential toxicity and associated exposure of BF-588-DualCAB was evaluated in vivo in cynomolgus monkeys and compared to both, BF-588-MonoCAB (CAB function only in the CD3 binding domain) and to BF-588-WT (Wild Type, non-CAB) antibody. For the dose-range finding study, cynomolgus monkeys (2 animals/group, 1 male and 1 female) were administered with a single dose of BF-588-WT, BF-588-MonoCAB at and BF-588-DualCAB at specified doses by i.v. bolus. In the repeat-dose toxicity study, BF-588-DualCAB was administered by i.v. bolus to cynomolgus monkeys (6 animals/group, 3 males and 3 females) at 0 (vehicle control), 0.5, 1.5, and 5 mg/kg/week. Recovery animals (4 animals/group, 2 males and 2 females; 4-week recovery period) were included in the vehicle control and high-dose groups. Safety endpoints for these studies included clinical observations, food evaluation/consumption, body weight, hematology, coagulation, serum chemistry, urinalysis, organ weights, and macroscopic and microscopic examinations. Cytokine analysis and Immunophenotyping were conducted to assess total T cells, activated and proliferating CD8+ and CD4+ T cells, B cells, NK cells, and T regulatory cell populations. Blood samples were collected to evaluate systemic exposure to each test article.
Abbreviations
ADA | = | anti-drug antibodies |
CAB | = | conditionally active biologic |
CDX | = | cell line derived xenograft |
CRS | = | cytokine release syndrome |
cyno | = | cynomolgus |
ECD | = | extracellular domain |
EpCAM | = | epithelial cell adhesion molecule |
GLP | = | good laboratory practice |
hu | = | human |
i.v. | = | intravenously |
MTD | = | maximum tolerated dose |
mpk | = | milligram per kilogram |
PaCS | = | protein-associated chemical switches |
PBMC | = | peripheral blood mononuclear cells |
TAA | = | tumor-associated antigen |
TCE | = | T-cell engager |
TGI | = | tumor growth inhibition |
TME | = | tumor microenvironment |
WT | = | wild type |
Author contributions
Conceptualization: GF, HWC, JMS; Investigation and Data Analysis: GF, APGC, HL, CX, CW, HWC, WJB, and JMS; Visualization: GF, APGC, HL, CX, CW; Writing (original draft), review, and editing): GF, APGC, HL, CX, and HWC; Writing (review and editing): GF, APGC, HL, HWC, WJB, and JMS.
Data availability satement
All data are available in the main text or the supplementary materials.
Frey_et_al_supplemental_material_revised_2.docx
Download MS Word (718.2 KB)Acknowledgments
We thank the BioDuro-Sundia Discovery Biology team for their valuable technical assistance and R&D support and Monica Sullivan and James Butler for critical reading of the manuscript.
Disclosure statement
All authors are shareholders of BioAtla, Inc., which owns intellectual property rights to CABs- and PaCS-related technology. HWC, GF, and JMS are inventors of relevant patents. JMS serve as Director of BioAtla.
Supplementary material
Supplemental data for this article can be accessed online at https://doi.org/10.1080/19420862.2024.2322562
Additional information
Funding
References
- Herreros-Pomares A, Aguilar-Gallardo C, Calabuig-Farinas S, Sirera R, Jantus-Lewintre E, Camps C. EpCAM duality becomes this molecule in a new Dr. Jekyll and Mr. Hyde tale. Crit Rev Oncol Hematol. 2018;126:52–14. doi:10.1016/j.critrevonc.2018.03.006.
- Armstrong A, Eck SL. EpCAM: a new therapeutic target for an old cancer antigen. Cancer Biol Ther. 2003;2:320–26. doi:10.4161/cbt.2.4.451.
- Spizzo G, Fong D, Wurm M, Ensinger C, Obrist P, Hofer C, Mazzoleni G, Gastl G, Went P. EpCAM expression in primary tumour tissues and metastases: an immunohistochemical analysis. J Clin Pathol. 2011;64:415–20. doi:10.1136/jcp.2011.090274.
- Liu Y, Wang Y, Sun S, Chen Z, Xiang S, Ding Z, Huang Z, Zhang B. Understanding the versatile roles and applications of EpCAM in cancers: from bench to bedside. Exp Hematol Oncol. 2022;11:97. doi:10.1186/s40164-022-00352-4.
- Przepiorka D, Ko CW, Deisseroth A, Yancey CL, Candau-Chacon R, Chiu HJ, Gehrke BJ, Gomez-Broughton C, Kane RC, Kirshner S. et al. FDA Approval: Blinatumomab. Clin Cancer Res. 2015;21(18):4035–9. doi:10.1158/1078-0432.CCR-15-0612.
- Thieblemont C, Phillips T, Ghesquieres H, Cheah CY, Clausen MR, Cunningham D, Do YR, Feldman T, Gasiorowski R, Jurczak W. et al. Epcoritamab, a novel, subcutaneous CD3xCD20 bispecific T-Cell–engaging antibody, in relapsed or refractory large B-Cell lymphoma: dose expansion in a phase I/II trial. J Clin Oncol. 2023;41(12):2238–47. doi:10.1200/JCO.22.01725.
- Budde LE, Sehn LH, Matasar M, Schuster SJ, Assouline S, Giri P, Kuruvilla J, Canales M, Dietrich S, Fay K. et al. Safety and efficacy of mosunetuzumab, a bispecific antibody, in patients with relapsed or refractory follicular lymphoma: a single-arm, multicentre, phase 2 study. Lancet Oncol. 2022;23(8):1055–65. doi:10.1016/S1470-2045(22)00335-7.
- Kiewe P, Hasmuller S, Kahlert S, Heinrigs M, Rack B, Marme A, Korfel A, Jäger M, Lindhofer H, Sommer H. et al. Phase I trial of the trifunctional Anti-HER2 × Anti-CD3 antibody ertumaxomab in metastatic breast cancer. Clin Cancer Res. 2006;12:3085–91. doi:10.1158/1078-0432.CCR-05-2436.
- Seimetz D, Lindhofer H, Bokemeyer C. Development and approval of the trifunctional antibody catumaxomab (anti-EpCAM x anti-CD3) as a targeted cancer immunotherapy. Cancer Treat Rev. 2010;36:458–67. doi:10.1016/j.ctrv.2010.03.001.
- Mau-Sørensen M, Dittrich C, Dienstmann R, Lassen U, Büchler W, Martinius H, Tabernero J. A phase I trial of intravenous catumaxomab: a bispecific monoclonal antibody targeting EpCAM and the T cell coreceptor CD3. Cancer Chemother Pharmacol. 2015;75:1065–73. doi:10.1007/s00280-015-2728-5.
- Cioffi M, Dorado J, Baeuerle PA, Heeschen C. EpCAM/CD3-Bispecific T-cell engaging antibody MT110 eliminates primary human pancreatic cancer stem cells. Clin Cancer Res. 2012;18(2):465–74. doi:10.1158/1078-0432.CCR-11-1270.
- English DP, Bellone S, Schwab CL, Roque DM, Lopez S, Bortolomai I, Cocco E, Bonazzoli E, Chatterjee S, Ratner E. et al. Solitomab, an epithelial cell adhesion molecule/CD3 bispecific antibody (BiTE), is highly active against primary chemotherapy-resistant ovarian cancer cell lines in vitro and fresh tumor cells ex vivo. Cancer. 2015;121(3):403–12. doi:10.1002/cncr.29062.
- Kebenko M, Goebeler ME, Wolf M, Hasenburg A, Seggewiss-Bernhardt R, Ritter B, Rautenberg B, Atanackovic D, Kratzer A, Rottman JB. et al. A multicenter phase 1 study of solitomab (MT110, AMG 110), a bispecific EpCAM/CD3 T-cell engager (BiTE®) antibody construct, in patients with refractory solid tumors. Oncoimmunology. 2018;7:e1450710. doi:10.1080/2162402X.2018.1450710.
- Chang HW, Frey G, Liu H, Xing C, Steinman L, Boyle WJ, Short JM. Generating tumor-selective conditionally active biologic anti-CTLA4 antibodies via protein-associated chemical switches. Proc Natl Acad Sci U S A. 2021;118. doi:10.1073/pnas.2020606118.
- Chen M, Chen C, Shen Z, Zhang X, Chen Y, Lin F, Ma X, Zhuang C, Mao Y, Gan H. et al. Extracellular pH is a biomarker enabling detection of breast cancer and liver cancer using CEST MRI. Oncotarget. 2017;8(28):45759–67. doi:10.18632/oncotarget.17404.
- Liu X, Deng J, Yuan Y, Chen W, Sun W, Wang Y, Huang H, Liang B, Ming T, Wen J. et al. Advances in Trop2-targeted therapy: novel agents and opportunities beyond breast cancer. Pharmacology & Therapeutics. 2022;239:108296. doi:10.1016/j.pharmthera.2022.108296.
- Linke R, Klein A, Seimetz D. Catumaxomab: clinical development and future directions. Mabs-austin. 2010;2(2):129–36. doi:10.4161/mabs.2.2.11221.
- Baeuerle PA, Wesche H. T-cell-engaging antibodies for the treatment of solid tumors: challenges and opportunities. Curr Opin Oncol. 2022;34(5):552–8. doi:10.1097/CCO.0000000000000869.
- Dreher MR, Liu W, Michelich CR, Dewhirst MW, Yuan F, Chilkoti A. Tumor vascular permeability, accumulation, and penetration of macromolecular drug carriers. J Natl Cancer Inst. 2006;98(5):335–44. doi:10.1093/jnci/djj070.
- Goebeler ME, Bargou RC. T cell-engaging therapies - BiTEs and beyond. Nat Rev Clin Oncol. 2020;17:418–34. doi:10.1038/s41571-020-0347-5.
- Saber H, Del Valle P, Ricks TK, Leighton JK. An FDA oncology analysis of CD3 bispecific constructs and first-in-human dose selection. Regul Toxicol Pharmacol. 2017;90:144–52. doi:10.1016/j.yrtph.2017.09.001.
- Shanshal M, Caimi PF, Adjei AA, Ma WW. T-Cell engagers in solid cancers—Current landscape and future directions. Cancers Basel. 2023;15:15. doi:10.3390/cancers15102824.
- Spiess C, Merchant M, Huang A, Zheng Z, Yang NY, Peng J, Ellerman D, Shatz W, Reilly D, Yansura DG. et al. Bispecific antibodies with natural architecture produced by co-culture of bacteria expressing two distinct half-antibodies. Nat Biotechnol. 2013;31(8):753–8. doi:10.1038/nbt.2621.
- Bolt S, Routledge E, Lloyd I, Chatenoud L, Pope H, Gorman SD, Clark M, Waldmann H. The generation of a humanized, non-mitogenic CD3 monoclonal antibody which retains in vitro immunosuppressive properties. Eur J Immunol. 1993;23:403–11. doi:10.1002/eji.1830230216.
- Wang X, Mathieu M, Brezski RJ. IgG fc engineering to modulate antibody effector functions. Protein Cell. 2018;9(1):63–73. doi:10.1007/s13238-017-0473-8.
- Kumari R, Feuer G, Bourre L. Humanized mouse models for Immuno-oncology drug discovery. Curr Protoc. 2023;3:e852. doi:10.1002/cpz1.852.
- De La Rochere P, Guil-Luna S, Decaudin D, Azar G, Sidhu SS, Piaggio E. Humanized mice for the study of immuno-oncology. Trends Immunol. 2018;39(9):748–63. doi:10.1016/j.it.2018.07.001.