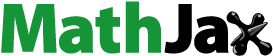
ABSTRACT
Co-formulation of multiple drug products is an efficient and convenient approach to simultaneously deliver multiple biotherapeutics with the potentially added benefit of a synergistic therapeutic effect. However, co-formulation also increases the risk of heteromeric interactions, giving rise to unique impurities with unknown efficacy and immunogenicity. Therefore, it is critical to develop methods to evaluate the risk of heteromers as an impurity that could affect potency, efficacy, and/or immunogenicity. The most direct strategy to evaluate antibody heteromers is via specific enrichment. However, the fact that antibody heterodimers generated from the co-formulated cocktail share highly similar molar mass and size properties as homodimers natively present in each individual antibody drug product poses a unique purification challenge. Here, we report the path to successful enrichment of heterodimers from co-formulated REGEN-COVⓇ and discuss its potential impacts on drug quality.
Introduction
Co-formulation of multiple drug products is an emerging approach to simultaneously deliver multiple biotherapeutics, which may previously have been administered sequentially or concurrently. This approach not only provides a potential for synergistic therapeutic effect, but also enables improved convenience for both patients and health care professionals, with fewer administration events and potentially decreased risk of medical errors.Citation1
However, co-formulation of multiple drug products increases the potential for heteromeric interactions, which have unknown efficacy and immunogenicity. Citation1–4 As pharmaceutical companies expand their pipelines with co-administered and potentially co-formulated therapeutic products to address allergy, oncology, and infectious disease indications,Citation2,Citation5,Citation6 it is critical to develop methods to evaluate potential heteromeric interactions for risks that may affect potency, efficacy, or immunogenicity.Citation1,Citation3,Citation4
During the severe acute respiratory syndrome coronavirus 2 (SARS-CoV-2) pandemic, REGEN-COVⓇ was developed as an important treatment for mild-to-moderate coronavirus disease 2019 (COVID-19) in both adults and pediatric patients.Citation7 REGEN-COVⓇ is a cocktail of two therapeutic IgG1 monoclonal antibodies (mAbs), casirivimab and imdevimab, that simultaneously engage different epitopes of the receptor-binding domain of the SARS-CoV-2 trimeric spike (S) protein.Citation8–11 These properties enable therapeutic efficacy either directly through viral neutralization or indirectly by antibody-dependent cellular cytotoxicity (ADCC).Citation10 Throughout clinical evaluation and COVID-19 emergency use, casirivimab and imdevimab were co-administered, requiring a minimum of four infusions or injections (two per antibody).Citation9,Citation12 Because casirivimab and imdevimab share the same formulation, a 1:1 co-formulation was pursued to potentially improve convenience for patients and healthcare professionals. Simultaneous fill/finishing of co-formulated products could also simplify or accelerate the manufacturing process.
Here, we report our purification strategy for preparative-scale, specific enrichment of heterodimers from co-formulated REGEN-COVⓇ and evaluate their impact on binding and potency based on in vitro and cell-based assays. Overall, the binding parameters and potency of casirivimab-imdevimab heterodimers enriched from co-formulated REGEN-COVⓇ are comparable to that of the individual antibodies. These results suggest that the presence of heterodimers in co-formulated REGEN-COVⓇ would not introduce a risk of loss of efficacy in clinical settings.
Enrichment strategy
A target heterodimer purity of > 50% was selected to ensure that any analytical data obtained from the sample would be representative of the biochemical and biophysical characteristics of the heterodimer. This is particularly important to interpret data from functional assays which measure the average binding kinetics and potency of an ensemble of species within a sample. The >50% purity threshold, representing a simple majority, can provide some assurance that the functional properties exhibited by the enriched sample are representative of the major species – in this case, the heterodimer.
An overall yield of 1 mg was required for additional biophysical and biochemical characterization. The total relative abundance of dimers present in co-formulated samples was ≤0.7% based on analytical SE-UPLC, and heterodimers were only observed at trace amounts (<0.05%) based on two orthogonal mass spectrometric methods (Supplementary Figure S1). Due to the very low abundance of the heterodimeric species of interest, preparative-scale size-exclusion chromatography was performed as a first step to generate a total enriched dimer sample comprising ~88% dimeric species. Within this total dimer sample, the relative abundance of heterodimer was between 3% and 5%, requiring additional purification for downstream in vitro binding and potency evaluations.
Due to the narrow range of predicted masses of the heterodimer (288,764.2 Da) compared to the predicted masses of each homodimer species (casirivimab: 289,889.8 Da; imdevimab: 287,638.5 Da), molecular size alone would not be a viable mode to enrich and purify the heterodimer. Thus, it was critical to consider alternative biophysical properties, such as surface charge and surface hydrophobicity, which may distinguish the various dimeric species and enable separation. Although chromatographic separation based on these properties was met with varying degrees of success, it is valuable to capture the breadth of scientific considerations and empirical results generated throughout the process to support future heterodimer enrichments.
Feasibility of cation exchange chromatography (CEX)
The feasibility of surface-based CEX for heterodimer enrichment was evaluated by subjecting the co-formulated sample to analytical strong cation exchange chromatography coupled to mass spectrometry (SCX-UV-MS)Citation13 using a pH-based gradient of ammonium acetate/acetic acid and sodium bicarbonate. Intact casirivimab was found to elute earlier than imdevimab, suggesting a more acidic surface charge (Supplementary Figure S1(a)); this result is consistent with the predicted isoelectric point (pI)Citation14 for casirivimab (theoretical pI: 7.90) and imdevimab (theoretical pI: 8.35). Furthermore, all homodimers and heterodimers eluted after the monomers, suggesting possible nonspecific interactions between the dimer surfaces and the column resin.Citation15 The casirivimab homodimer co-eluted with basic variants of monomeric imdevimab, and the trace homodimers of imdevimab eluted last. Heterodimer was detected in between the two homodimer peaks.
Because the separation between heterodimer and homodimers seemed promising, small-scale preparative CEX was pursued as a potential enrichment method for heterodimers. A total dimer pool from co-formulated REGEN-COVⓇ was used as the starting material for this procedure to remove the complexity of the predominant monomeric species. The enriched dimer pool was subjected to a dual salt-pH gradient elution from a strong cation exchange resin, resulting in eight pooled fractions (). Preliminary SE-UPLC analysis indicated that fractions F1 and F2 contained residual monomer, while fractions F3-F8 contained various forms of enriched dimer based on intact SEC-UV-MS analysis ().
Figure 1. (a–b) Preparative CEX chromatogram and deconvoluted masses for homo- and heterodimers of casirivimab and imdevimab from SEC-UV-MS analysis of enriched fractions. Although heterodimers were detected within fractions F3-F6 (gray shaded region), high charge heterogeneity among dimer species complicated the enrichment. (c–d) Preparative MMC chromatogram and deconvoluted masses from SEC-UV-MS analysis of enriched fractions. Heterodimers were detected specifically in MMC fraction F5, which was subjected to additional purification.

The total ion chromatograms corresponding to fraction F3 indicated a possibly higher relative abundance of casirivimab homodimers compared to imdevimab homodimers. Peaks in the mass range corresponding to heterodimer were also observed (). However, the deconvoluted masses exhibited high heterogeneity for deglycosylated casirivimab dimer (289,890 Da) and deglycosylated imdevimab dimer (287,638 Da), suggesting the presence of heterogenous acidic posttranslational modifications, including glycation (+162 Da) and advanced glycation end products. Although the adjacent CEX fraction F4 also contained heterogenous variants of heterodimer and imdevimab homodimer, the deconvoluted mass peaks corresponding to the casirivimab homodimer matched the expected deglycosylated form of casirivimab. Fraction F5 appeared to comprise the unmodified species for all three dimeric species, while casirivimab homodimer was specifically enriched in the late-eluting fraction F7. Overall, these results demonstrated that preparative CEX was capable of enriching for heterodimers to some degree; however, the complexity and convolution of charge variants within the dimer populations precluded enrichment of heterodimer to high purity and would negatively impact heterodimer yield (). Therefore, further enrichment efforts using CEX were not pursued.
Table 1. Summary of strategies for heterodimer enrichment.
Feasibility of HIC
As a result, the feasibility of hydrophobicity-based purification techniques was evaluated using analytical HIC coupled to MS (HIC-UV-MS). HIC is mediated by interactions between the column stationary phase and hydrophobic patches on the protein surface,Citation16 which can be exposed by placing the protein in a high ionic strength kosmotropic salt such as ammonium sulfate/acetate. Using a descending ammonium acetate gradient on an analytical butyl column, two distinct baseline-resolved peaks were observed corresponding to monomeric casirivimab (retention time ~9.9 minutes) and imdevimab (retention time ~14.5 minutes). Importantly, due to the dominance of the hydrophobic interaction between the protein and the stationary phase (compared to size- and/or charge-based chemistry), each homodimer eluted immediately after its corresponding monomer. The heterodimer was detected between the two homodimers and partially co-eluted with monomeric imdevimab ( and S1(d)), potentially leading to scale-up purification issues.
Figure 2. Purification of heterodimers from REGEN-COV®. (a) A three-step enrichment process was required to enrich heterodimers from trace amounts (<0.05%) to > 55% purity. (b) From the initial preparative SEC enrichment, a total dimer abundance of ~ 88% was achieved. (c) By mixed-mode chromatography (MMC), the total dimer sample partitioned into four specific dimeric peaks (shaded blue region). The casirivimab and imdevimab monomers exhibited differential hydrophobicity and could be baseline-resolved using this resin. The final heterodimer sample exhibited a purity of ~ 67% based on this analytical method. (d) The analytical HIC method suggested that heterodimers comprise up to 76% of the total sample; this value may be slightly overestimated due to co-elution of imdevimab monomer.

Although the analytical HIC-UV-MS method is limited to MS-friendly buffer systems, this is not a requirement for purification. The HIC method was reoptimized using ammonium acetate; however, iterative analytical HIC was not a viable option due to a lengthy, labor-intensive process (). To obtain ~1 mg of enriched heterodimer using analytical HIC, approximately 700 injections would need to be performed. In practice, consecutive injections did not overlay, presenting issues with automated, iterative fraction collection into the same vial to increase throughput. This lack of reproducibility was likely exacerbated by the requirement of an elution gradient and emphasizes the impracticality of this approach. Additional efforts using shallow ammonium acetate gradients applied to preparative butyl and octyl columns on an ÄKTA purifier did not afford resolution comparable to the analytical method (data not shown). As a result, alternative purification modes, such as mixed-mode or multi-modal chromatography (MMC) were considered.
MMC combines size-exclusion and hydrophobic interaction chromatography modes, providing an adequate balance between resolution and throughput for heterodimer enrichment
Among MMC resins, the analytical Sepax ZenixⓇ SEC-300 column (3 µm particle size) leverages separation by both size and hydrophobicity.Citation17 This combination of SEC and HIC has been demonstrated to enable separation of antibody fragments and oxidation variants from intact, native antibody as well as bispecific antibodies from parental monospecific impurities.Citation18,Citation19 An additional advantage of an SEC-HIC MMC method is the potential use of isocratic elution, which could mitigate the run-to-run reproducibility issues of a standalone HIC method.
Injection of co-formulated REGEN-COVⓇ onto the analytical ZenixⓇ column using a buffer containing sulfate, acetate, phosphate, and chloride salts revealed baseline separation between the individual monomers () consistent with the high resolution afforded by HIC. Interestingly, the enriched total dimer pool demonstrated high heterogeneity for peaks larger than monomer, suggesting that the heterodimer and enriched homodimers may exhibit unique hydrophobic patches which may be chromatographically distinct and separable.
Results
Enrichment of heterodimers using preparative hydrophobicity-based mixed-mode chromatography
A larger particle size (5 µm) SEC-300 MMC column using the same chemically bonded monolayer surface structure as the analytical ZenixⓇ column was evaluated for potential scalability. Using the enriched dimer as starting material for the preparative MMC column, partial enrichment of heterodimer was achieved, despite lower resolution between the putative dimers and the monomers () compared to the analytical MMC column. Intact mass spectrometric analysis of the dimer populations enriched by MMC revealed that the first two predominant peaks present in fractions F3-F4 were specifically enriched for the casirivimab homodimer, while the later-eluting peak present in fraction F6 was specifically enriched for the imdevimab homodimer . The elution order of the dimers was consistent with previous analytical HIC results and independently confirmed that casirivimab was less hydrophobic overall than imdevimab under these experimental conditions. Fraction F5, in the valley between the two dominant peaks, contained the highest abundance of heterodimer, although this sample was only partially enriched for the species of interest (≤14%).
By combining preparative MMC with an additional analytical HIC separation (; ), it was possible to enrich for heterodimer above the target thresholds for purity (≥50%) and yield (~1 mg). Indeed, by adding the preparative MMC step to the purification protocol, the number of injections required for analytical HIC was significantly reduced from ~700 to a manageable ~100. This also corresponded to a 25–50% reduction in overall processing time from ~28 continuous days to ~14–21 days. The increased efficiency could substantially improve final heterodimer yield by mitigating the potential risk of antibody dimers reversing to their component monomers over time and/or at low concentrations.
The two-step enrichment of heterodimer from enriched total dimer resulted in a final estimated purity between 50% and 60% (; ). Analytical HIC resulted in a peak purity of 84%, but this value may overestimate the level of heterodimer due to co-elution with the imdevimab monomer. Analytical SE-UPLC and MMC-UPLC both consistently revealed that the total abundance of dimer including homodimer impurities in the enriched heterodimer sample was ≤67%. Because the analytical MMC-UPLC method could distinguish between each individual monomer (casirivimab: 10.8%; imdevimab: 23.1%), simple arithmetic could be used to estimate the abundance of heterodimer in the final sample (~60%). These results were corroborated by quantitation using mass spectrometry (MS) performed on a deglycosylated enriched heterodimer sample (). Based on deconvoluted mass results (), the heterodimer comprises ~75% of the total dimer population within the enriched sample. Assuming the aforementioned ~67% total abundance of dimer based on analytical SE-UPLC, the heterodimer would comprise ~50% within the enriched sample. The slight discrepancy in heterodimer quantitation between native chromatographic methods and the MS-based result may reflect differences in sensitivity, resolution, buffer condition, and/or sample handling.
Figure 3. Analysis of the dimer peak by native SEC-UV-MS reveals successful enrichment of heterodimer. (a) UV spectrum for deglycosylated enriched heterodimer. (b) Deconvoluted masses corresponding to the dimer region of (a) demonstrates that ~ 75% of the deconvoluted MS signal corresponds to heterodimer with ~ 12–13% each contribution from each homodimer.

The residual monomer present in the enriched heterodimer sample from the SEC-UV-MS data was also characterized as an enrichment method control (Supplementary Figure S2(a–b)). The monomer present in this sample comprises both co-purified imdevimab monomer and dissociated monomer. The deconvoluted masses corresponding to the monomer region were highly similar to the intact masses observed in the co-formulated control sample (Supplementary Figure 2(c)), suggesting that the enrichment process did not induce formation of novel post-translational modifications (PTMs).
Potency evaluation of enriched heterodimer
The final enriched heterodimer fraction was evaluated for potency using pseudovirus neutralization and ADCC surrogate assays. A co-formulated reference standard lot was used as the control for the enriched total dimer and enriched heterodimer samples derived from the co-formulated product, and the corresponding reference standards for casirivimab and imdevimab were used to evaluate potency for the individual antibody products. The enriched heterodimer sample was capable of both neutralization activity (71%) and activation of ADCC (69%), although these values trended slightly lower than those observed in the co-formulated control sample and for casirivimab or imdevimab evaluated individually (; ). Interestingly, homodimers enriched from either casirivimab or imdevimab exhibited higher relative potencies for both neutralization (125–178%) and ADCC (147–162%) compared to the control samples (), indicating that the homodimer configurations may enable a more favorable Fab and Fc orientation for bridging the virus to effector cells (ADCC) or may promote inhibition of viral entry (neutralization) by blocking multiple spike proteins more efficiently. Since the potency activities observed for heterodimer were slightly lower than for the homodimer samples, it is possible that one or both Fab domains may be partially occluded in the heterodimer conformation.
Figure 4. Relative potencies of REGEN-COVⓇ samples by (a–b) pseudovirus neutralization and (c–d) ADCC assays from a single representative data set. Values represent the average relative potency calculated from four separate data sets.

Table 2. Relative potencies of individual and co-formulated REGEN-COVⓇ antibodies by pseudovirus neutralization and ADCC assays.
To further investigate this potential trend, in vitro binding to the SARS-CoV-2 trimeric spike protein was evaluated using a standard surface plasmon resonance assay. The assay format involved initial capture of each monomeric antibody, co-formulated mixture, or enriched sample to the carboxymethylated CM4 sensor chip, followed by binding of recombinant SARS-CoV-2 trimeric spike protein at different concentrations. Kinetic rate constants for association (ka) and dissociation (kd), equilibrium dissociation constants (KD), and calculated dissociation half-lives (t1/2) were determined by global fitting ().
Table 3. In vitro binding parameters of enriched individual REGEN-COVⓇ monomers and co-formulated REGEN-COVⓇ samples using surface plasmon resonance (SPR) spectroscopy.
The kinetic binding parameters and equilibrium dissociation constant of the 1:1 co-formulated control sample (KD ~1.26) are more similar to monomeric casirivimab (KD ~1.86) than the average of the two monoclonal antibodies (KD ~7.68), suggesting that the binding affinity and potency of co-formulated REGEN-COVⓇ is dominated by casirivimab. The kinetic binding parameters of the enriched total dimer sample (KD ~1.76) were also comparable to monomeric casirivimab; this result may be due to the enriched total dimer sample containing more casirivimab dimers (~51.3%) and residual monomer (~8.9%) compared to imdevimab dimers (~30.5%) and residual monomer (~4.6%).
In contrast, the enriched heterodimer sample exhibited the weakest equilibrium dissociation constant (KD ~ 21.1) due to a relatively slower rate of association (ka ~ 14.5 1/Ms) and a relatively faster dissociation rate (kd ~ 0.69 1/µs). Overall, this binding behavior suggests that a casirivimab binding site may be partially occluded in the heterodimer, thereby leading to the slight reduction in potency observed in the pseudovirus neutralization and ADCC assays. Additional structural and analytical studies to evaluate heterodimer interfaces at the subunit level suggest that the casirivimab and imdevimab Fab domains are involved in a majority of heterodimer contacts (data not shown).
Enriched heterodimers dissociate under physiological conditions
In addition to potency, the stability of the enriched heterodimer sample was evaluated following extended incubation under physiologically relevant conditions by MMC analysis. Heterodimers that can reversibly dissociate into individual monomeric species either following dilution upon administration, or following extended incubation at physiological temperatures, are less likely to affect the safety and efficacy of the co-formulated product.Citation20 It is important to note that the heterodimers enriched in these samples likely represent the most stable form of heterodimer present in co-formulated REGEN-COVⓇ, having persisted throughout a purification process that included various dilution steps and high ionic strength conditions, which can be disruptive to weakly associated macromolecules. Incubation of the enriched total dimer and enriched heterodimer samples at 37°C demonstrated that a significant proportion of the dimers in each sample were dissociable up to 14 days (). The dimer dissociation rate for each sample across the 14-day evaluation period were modeled using an exponential decay function (Equation 2). The rates of dimer dissociation (k) and extent of dissociation (span) for each sample were generally comparable between the enriched dimer samples (). Given that the enriched total dimer sample is predominantly composed of casirivimab and imdevimab homodimers, these results suggest that the stability/reversibility of heterodimers present in co-formulated REGEN-COVⓇ samples is comparable to homodimers of the individual drug components.
Figure 5. Stability assessment of enriched total dimer and heterodimers from REGEN-COV®. (a) Co-formulated REGEN-COVⓇ, enriched total dimers, and enriched heterodimer were incubated at 37°C and subjected to analytical MMC-FLR-UPLC. (b) Example MMC chromatograms at t = 0 and t = 14 days for each sample. Monomer and dimer peaks are shaded. (c) Peak area quantitation of monomer, dimer, very high molecular weight (vHMW) species, and low molecular weight (LMW) species for each sample throughout the time course based on MMC-FLR-UPLC. Each data point represents the average of three replicate measurements. Dimer peaks were below quantitation in the co-formulated control sample due to heterogeneity of the dimer subpopulations. Results indicate that most enriched dimer forms are partially dissociable, including the heterodimer (right).

Table 4. Comparison of dimer stability parameters for enriched total dimer and enriched heterodimer samples during prolonged 37°C storage.
Discussion
Heterodimers represent a novel impurity and therefore potential quality attribute of co-formulated therapeutic antibody products. To evaluate potential risks associated with potency, efficacy, and immunogenicity of heteromeric species in co-formulated products, a three-step purification protocol was developed to specifically enrich trace heterodimers from co-formulated REGEN-COVⓇ. Initial SEC enrichment to generate a total dimer pool increased the relative abundance of heterodimers from <0.7% to ~3–5%, and additional preparative MMC and analytical HIC steps boosted heterodimer purity to ~14% and >50%, respectively. The enriched heterodimer sample exhibited ADCC activity and neutralized SARS-CoV-2-pseudotyped virions. However, the relative potencies of the enriched heterodimer were slightly lower compared to the co-formulated control and enriched total dimer samples. Additional in vitro binding results suggested that the enriched heterodimer is more similar to the weaker-binding imdevimab molecule, suggesting that a casirivimab binding site may be partially occluded in the heterodimer. Moreover, the heterodimer was able to dissociate under physiological conditions at low concentrations, comparable to homodimers typically observed in antibody drug products.
The risk of immunogenicity for a therapeutic antibody is dependent on its format, isotype, and physiochemical properties, including size, morphology, conformation, surface charge, and hydrophobicity.Citation21–25 Based on the observation that the heterodimer formed from casirivimab and imdevimab could be separated from the respective homodimeric forms by exploiting differences in their electrostatic properties suggests that the heterodimer may represent a novel impurity with a potential risk for immunogenicity. Although the immunogenic potential of the enriched heterodimer was not directly evaluated in this study, intact mass analysis of the enriched heterodimer sample revealed no novel PTMs that might contribute to immunogenicity. Furthermore, high intravenous (IV) doses of casirivimab and imdevimab have been administered in Phase 2/3 clinical trials, with positive clinical outcomes and no indication of the development of anti-drug antibodies.Citation9 Given that during IV coadministration of REGEN-COVⓇ, both molecules were mixed 1:1 in the same IV administration bag, it is possible that there was clinical exposure to trace levels of heterodimer in these trials. Taken together, these results may suggest that the risk of immunogenicity incurred from REGEN-COVⓇ heterodimers is relatively low; however, further investigation into the immunogenicity risk of antibody heterodimers is warranted and will likely need to be conducted on a case-by-case basis.
Overall, the results presented in this study present an assessment of heterodimer risk to potency from a purely biophysical and biochemical standpoint. This workflow for enrichment and limited characterization could be used to support and/or contextualize results from co-administrated and co-formulated clinical studies to assess potency and immunogenicity risk from heterodimers in a broader context. Future studies to characterize the structural organization of the enriched heterodimer from REGEN-COVⓇ compared to casirivimab and imdevimab homodimers may also provide additional clarity with respect to the stability profile and target binding mechanism for this product.
Materials and methods
All mAbs used in this experiment were expressed in Chinese hamster ovary cells and produced at Regeneron Pharmaceuticals, Inc.
Enrichment of total dimers and heterodimers
Heterodimer enrichment was performed using preparative size-exclusion chromatography (SEC), followed by semi-preparative mixed-mode chromatography (MMC) and analytical hydrophobic interaction chromatography (HIC). For preparative SEC, co-formulated REGEN-COVⓇ was initially diluted with 10 mM histidine, pH 6.0 to ~50 mg/mL and subsequently loaded (~180 mg) onto a preparative grade Superdex 200 (26/1000) column (Cytiva) pre-equilibrated in 1X Dulbecco’s phosphate-buffered saline (DPBS), pH ~ 7.2 for isocratic elution at 2.6 mL/minute with fractionation. Fractions containing high molecular weight (HMW) species were subsequently pooled and concentrated using centrifugal filtration units (Vivacell 100; 30 kDa molecular weight cutoff; Sartorius) to increase the protein concentration to ≥1.0 mg/mL, resulting in the enriched total dimer sample. The enriched dimer sample was then subjected to semi-preparative MMC using a ZenixⓇ 300 columns (21.5 × 300 mm) pre-equilibrated with 20 mM sodium phosphate, 10 mM sodium acetate, 300 mM sodium sulfate, and 150 mM NaCl. Fractions were analyzed using mass spectrometry (MS) to assess heterodimer abundance; fractions containing heterodimer were pooled, concentrated, and subjected to a final analytical HIC enrichment step. Analytical HIC fractionation was performed using an Agilent 1260 HPLC equipped with a YMC BioPro HIC BF column (100 × 4.6 mm, 4 µm) and a Photodiode Array detector for UV detection at 280 nm. Mobile phase A consisted of 3 M ammonium acetate, and mobile phase B consisted of 100% water. Prior to sample injection, the HIC column was pre-equilibrated with 100% mobile phase A at a flow rate of 0.5 mL/minute and was maintained at 30°C throughout the experiment. Samples containing heterodimer (~100 µg) were injected onto the column and initially held at 100% mobile phase A at a flow rate of 0.5 mL/minute for 2 minutes. A two-step linear gradient was then applied from 0% to 22% mobile phase B for 1 minute and then from 22% to 90% mobile phase B for 15 minutes, followed by a 4-minute isocratic hold at the end of the gradient to ensure complete elution of the sample. Fractions containing heterodimer, based on MS analysis, were combined, concentrated, and buffer exchanged into 1X× DPBS, pH ~ 7.2.
Analytical size-exclusion ultra-performance liquid chromatography (SE-UPLC)
Analytical SE-UPLC was performed on each sample to assess the level of dimer enrichment. Samples (10 μg) were injected into two tandem Waters™ ACQUITY™ BEH200 SEC columns (1.7 μm particle size, 200 Å porosity, 2.6 mm internal diameter, 150 mm length), pre-equilibrated in 10 mM sodium phosphate, 500 mM sodium chloride, pH 7.0 at a flow rate of 0.3 mL/min. UV absorbance at both 215 and 280 nm was monitored. Data integration and analysis were performed using Empower 3 software (Waters Corporation).
Mixed-mode ultra-performance liquid chromatography (MMC-UPLC)
Each sample (10 μg) was injected onto a ZenixⓇ SEC-300 size-exclusion column (7.8 mm × 300 mm, 3 μm particle size; Sepax Technologies) pre-equilibrated in 20 mM sodium phosphate, 10 mM sodium acetate, 300 mM sodium sulfate, and 150 mM NaCl at a flow rate of 0.25 mL/minute. The absorbance signal was monitored at 215 nm and 280 nm, and chromatograms were integrated to determine relative abundance of each peak. Data integration and analysis were performed using Empower 3 software (Waters Corporation).
Heterodimer quantitation by mass spectrometry
Native SEC-UV-MS was performed to assess the purity of each fraction. Prior to analysis, each sample fraction was treated with PNGase F (1 IUB milliunit per 10 µg of protein) at 45°C in 50 mM Tris-HCl (pH 7.0) for 1 hour to remove the N-glycan chains from each heavy chain CH2 domain. Native SEC chromatography was performed on an UltiMate™ 3000 UHPLC System (Thermo Fisher Scientific) equipped with an ACQUITY™ BEH200 SEC column (4.6 × 300 mm, 1.7 µm, 200 Å; Waters™, Milford, MA) with the column compartment set to 30°C. An isocratic flow of 150 mM ammonium acetate, pH ~6.8, at 0.2 mL/minute was applied to separate and elute protein size variants prior to native MS analysis. To enable online native MS analysis, the analytical flow was split into a microflow (<10 µL/minute) for nano-electrospray ionization-MS detection and a remaining high flow for UV detection. A Thermo Scientific™ Q Exactive™ UHMR (Thermo Fisher Scientific) equipped with a microflow-nanospray electrospray ionization (MnESI) source and a microfabricated monolithic nulti-nozzle (M3) emitter (NewomicsⓇ) was used for native MS analysis. A detailed experimental setup and instrument parameters can be found in a previous publication.Citation26
To enable heterodimer quantitation, total dimer was first quantified using UV measurements of the SEC-resolved dimer and monomer peaks. Deconvoluted mass spectra of the dimer peak from native SEC-MS analysis were then generated using Intact Mass™ software from Protein Metrics. The relative distribution of homodimers and heterodimer in the dimer peak was calculated using the corresponding deconvoluted mass area. Lastly, heterodimer abundance was estimated by multiplying the measured total dimer abundance in the sample by the calculated heterodimer distribution in the dimer region.
Pseudovirus neutralization assay
Pseudotyped virus-like particles (VLPs) were generated using a VSVΔG system in which the vesicular stomatitis virus (VSV) glycoprotein was deleted from the genome and replaced with SARS-CoV-2 S protein (aa 14–1255). The pseudotyped VLPs were also engineered to express a firefly luciferase reporter. Non-replicating pVSV-SARS-CoV-2-S VLPs (~1,000 fluorescent focus units) were pre-incubated for 30 minutes with either casirivimab or imdevimab at varying concentrations ranging from 0.3 pM to 20 nM. Following pre-incubation, pVSV-SARS-CoV-2-S VLPs/antibody mixtures were added to 96-well plates containing Vero cells (2 × 10Citation4 cells/well) and incubated for 24 hours at 37°C with 5% CO2.
Plates were then equilibrated at ambient temperature for 15 minutes, followed by addition of ONE-Glo™ (Promega) firefly luciferase substrate and a subsequent 5–7 minute incubation at ambient temperature with gentle shaking. Luciferase activity (as a measure of Vero cells infectivity) was captured as relative light units using an EnVision™ multilabel plate reader, and values were analyzed using a four-parameter logistic equation over an 11-point dose–response curve to obtain EC50 values (GraphPad Prism). Relative potency of each test article was determined using EquationEquation (1)(1)
(1) :
ADCC assay
IgG receptor IIIA (FCGR3A) receptors expressed on natural killer (NK) cells are activated by interaction with Fc domains of antibodies bound to target cells. Activated NK cells then secrete cytokines, perforin and granzymes, which trigger target cell lysis. Here, REGEN-COVⓇ antibodies targeting the S protein of SARS-CoV-2 were evaluated in an ADCC reporter bioassay using engineered Jurkat effector cells expressing the high affinity human FCGR3A F176V allotype receptor and luciferase as a reporter gene (Jurkat/nuclear factor of activated T cells-luciferase [NFAT-Luc]/FCGR3A F176V) and target cells expressing the SARS-CoV-2 S protein (Jurkat/hCD20/SARS-CoV-2 S protein). In effector cells, engagement of FCGR3A F176V receptors via the Fc domain of human IgG1 antibodies bound to target cells triggers activation of the transcription factor NFAT. Transcriptional activation drives the expression of luciferase, which is then measured via a luminescence readout.
Briefly, Jurkat/NFAT-Luc/FCGR3A F176V effector cells and Jurkat/hCD20/SARS-CoV-2 S protein target cells were plated at a 1:1 ratio (50,000 cells each/well) in a 96-well plate. Casirivimab and imdevimab samples were evaluated separately at varying concentrations ranging from 4.88 pM to 5 nM and 338 fM to 20 nM, respectively. The final concentration of enriched heterodimer ranged from 1.05 pM to 10 nM. Plates were incubated at 37°C, 5.0% CO2 for 6 hours, followed by equilibration at ambient temperature for 10 minutes. An equal volume of ONE-Glo™ luciferase substrate was added to each well and the plate was incubated at room temperature for 3–5 minutes with shaking. Luciferase activity was captured as relative light units using an Envision multilabel plate reader and values were analyzed using a four-parameter logistic equation over an 11-point dose–response curve to obtain EC50 values (GraphPad Prism). Relative potency of each test article was determined using Equation 1.
Biacore assay
The kinetic binding parameters of the casirivimab, imdevimab, and enriched dimer samples to recombinant SARS-CoV-2 S protein were determined using surface plasmon resonance technology (Biacore™ T200). In brief, anti-human Fc was immobilized on the surface of a carboxymethyl dextran-coated (CM4) chip at 25°C following an amine-coupling procedure recommended by the manufacturer. After stabilization with running buffer (1X HBS-EP+ [10 mM HEPES, 150 mM NaCl, 3 mM EDTA, 0.05% (v/v) Surfactant P20, pH 7.4]), unfractionated and enriched dimer samples were captured separately on the immobilized chip surfaces. Subsequently, solutions of SARS-CoV-2 S protein at varying concentrations ranging from 1.25 nM to 80 nM were injected over each of the surfaces for 3 minutes at a flow rate of 50 μL/minute. Dissociation of bound SARS-CoV-2 S protein was monitored for 2 minutes. The specific SARS-CoV-2 S protein binding kinetic sensorgrams were obtained using a double referencing procedure to remove the refractive index change resulting from any nonspecific binding of SARS-CoV-2 S protein solutions as well as to remove binding signal changes due to the natural dissociation of the captured casirivimab or imdevimab from the chip surfaces. The resultant sensorgrams were then subjected to kinetic data analysis. The binding parameters were obtained by globally fitting the data to a 1:1 binding model with mass transport limitation, using the Biacore T200 evaluation software (version 3.0). The equilibrium dissociation constant (KD) was calculated by dividing the dissociation rate constant (kd) by the association rate constant (ka). The dissociation half-life (t1/2) was calculated by dividing the natural logarithm of 2 by the experimentally determined kd value.
Stability evaluation of enriched dimers and heterodimers
Enriched REGEN-COVⓇ dimers and co-formulated casirivimab and imdevimab were diluted to ~0.5 mg/mL in 1X DPBS, pH 7.2 and sub-aliquoted into UPLC vials (Waters™; Stoppers, TWD Trade Winds Inc) and incubated at 37°C. At each time point (0, 1, 3, 7, 14 days), one vial for each sample was removed and analyzed in triplicate by analytical mixed-mode chromatography using an UPLC system (Waters™) equipped with fluorescence detection (MMC-FLR-UPLC). Analytical MMC-FLR-UPLC followed the same protocol as described for MMC-UPLC with a reduced load of 1 µg. Tryptophan fluorescence was monitored by excitation at 295 nm and emission at 350 nm. Data integration and analysis were performed using Empower 3 software (Waters Corporation).
Dissociation rates of the dimer species were evaluated across the 14-day time course using JMPⓇ, (Version 17; SAS Institute Inc). An exponential decay function, EquationEquation (2)(2)
(2) , was used to model the decay rate:
where Days is the length of incubation period, k represents the rate of dimer dissociation, and Plateau is the asymptotic extent of dissociation. Span is defined as the difference between the asymptotic extent of dissociation and the first point measured at time zero.
Abbreviations
ADCC | = | Antibody-dependent cellular cytotoxicity |
CEX | = | Cation exchange chromatography |
Covid-19 | = | Coronavirus disease 2019 |
DPBS | = | Dulbecco’s phosphate-buffered saline |
HIC | = | Hydrophobic interaction chromatography |
HIC-UV-MS | = | Hydrophobic interaction chromatography coupled to mass spectrometry |
HIC-HPLC | = | Hydrophobic interaction high-performance liquid chromatography |
HMW | = | High molecular weight |
mAb | = | Monoclonal antibody |
MMC | = | Mixed-mode chromatography, multi-modal chromatography |
MMC-UPLC | = | Mixed-mode chromatography or multi-modal ultra-performance chromatography |
MS | = | Mass spectrometry |
SARS-CoV-2 | = | Severe acute respiratory syndrome coronavirus 2 |
SCX-UV-MS | = | Strong cation exchange chromatography coupled to mass spectrometry |
SEC | = | Size-exclusion chromatography |
SE-UPLC | = | Size-exclusion ultra-performance liquid chromatography |
VSV | = | Vesicular stomatitis virus |
Supplemental Material
Download MS Word (5.8 MB)Acknowledgments
The authors gratefully acknowledge Polat Abdubek and Glynn O’Grady for providing enriched homodimer from casirivimab and imdevimab. We also thank Yuan Cao for consulting on formulations development for this program and Lauren Salvador for editorial support and a critical reading of the manuscript.
Disclosure statement
No potential conflict of interest was reported by the author(s).
Supplementary material
Supplemental data for this article can be accessed online at https://doi.org/10.1080/19420862.2024.2338301
Additional information
Funding
References
- Kim J, Kim YJ, Cao M, Mel ND, Miller K, Bee JS, Wang J, Wang X, Albarghouthi M. Analytical characterization of coformulated antibodies as combination therapy. mAbs. 2020;12(1):1738691. doi:10.1080/19420862.2020.1738691.
- Chauhan VM, Zhang H, Dalby PA, Aylott JW. Advancements in the co-formulation of biologic therapeutics. J Control Release. 2020;327:397–12. doi:10.1016/j.jconrel.2020.08.013
- Sauna ZE, Lagassé D, Pedras-Vasconcelos J, Golding B, Rosenberg AS. Evaluating and mitigating the immunogenicity of therapeutic proteins. Trends Biotechnol. 2018;36(10):1068–84. doi:10.1016/j.tibtech.2018.05.008
- Yan Y, Xing T, Liu AP, Zhang Z, Wang S, Li N. Post-column denaturation-assisted native size-exclusion chromatography–mass spectrometry for rapid and in-depth characterization of high molecular weight variants in therapeutic monoclonal antibodies. J Am Soc Mass Spectrom. 2021;32(12):2885–94. doi:10.1021/jasms.1c00289
- Melero I, Hervas-Stubbs S, Glennie M, Pardoll DM, Chen L. Immunostimulatory monoclonal antibodies for cancer therapy. Nat Rev Cancer. 2007;7(2):95–106. doi:10.1038/nrc2051
- Krieg D, Winter G, Svilenov HL. It is never too late for a cocktail - development and analytical characterization of fixed-dose antibody combinations. J Pharm Sci. 2022;111(8):2149–57. doi:10.1016/j.xphs.2022.05.014
- Baral PK, Yin J, James MNG. Treatment and prevention strategies for the COVID-19 pandemic: a review of immunotherapeutic approaches for neutralizing SARS-CoV-2. Int J Biol Macromol. 2021;186:490–500. doi:10.1016/j.ijbiomac.2021.07.013
- Wu Y, Wang F, Shen C, Peng W, Li D, Zhao C, Li Z, Li S, Bi Y, Yang Y. et al. A noncompeting pair of human neutralizing antibodies block COVID-19 virus binding to its receptor ACE2. Science. 2020;368(6496):1274–78. doi:10.1126/science.abc2241
- Weinreich DM, Sivapalasingam S, Norton T, Ali S, Gao H, Bhore R, Musser BJ, Soo Y, Rofail D, Im J. et al. REGN-COV2, a neutralizing antibody cocktail, in outpatients with Covid-19. N Engl J Med. 2020;384(3):238–51. doi:10.1056/NEJMoa2035002
- Hansen J, Baum A, Pascal KE, Russo V, Giordano S, Wloga E, Fulton BO, Yan Y, Koon K, Patel K. et al. Studies in humanized mice and convalescent humans yield a SARS-CoV-2 antibody cocktail. Science. 2020;369(6506):1010–14. doi:10.1126/science.abd0827
- Baum A, Fulton BO, Wloga E, Copin R, Pascal KE, Russo V, Giordano S, Lanza K, Negron N, Ni M. et al. Antibody cocktail to SARS-CoV-2 spike protein prevents rapid mutational escape seen with individual antibodies. Science. 2020;369(6506):1014–18. doi:10.1126/science.abd0831
- Weinreich DM, Sivapalasingam S, Norton T, Ali S, Gao H, Bhore R, Xiao J, Hooper AT, Hamilton JD, Musser BJ. et al. REGEN-COV antibody combination and outcomes in outpatients with Covid-19. N Engl J Med. 2021;385(23):e81. doi:10.1056/NEJMoa2108163
- Yan Y, Liu AP, Wang S, Daly TJ, Li N. Ultrasensitive characterization of charge heterogeneity of therapeutic monoclonal antibodies using strong cation exchange chromatography coupled to native mass spectrometry. Anal Chem. 2018;90(21):13013–20. doi:10.1021/acs.analchem.8b03773
- Gasteiger E, Gattiker A, Hoogland C, Ivanyi I, Appel RD, Bairoch A. ExPASy: The proteomics server for in-depth protein knowledge and analysis. Nucleic Acids Res. 2003 Jul 1;31(13):3784–8. doi:10.1093/nar/gkg563. PMID: 12824418; PMCID: PMC168970.
- Joyce JG, Cook JC, Przysiecki CT, Lehman ED. Chromatographic separation of low-molecular-mass recombinant proteins and peptides on superdex 30 prep grade. J Chromatogr B Biomed Appl. 1994;662(2):325–34. doi:10.1016/0378-4347(94)00206-1
- Yan Y, Xing T, Wang S, Daly TJ, Li N. Online coupling of analytical hydrophobic interaction chromatography with native mass spectrometry for the characterization of monoclonal antibodies and related products. J Pharm Biomed Anal. 2020;186:113313. doi:10.1016/j.jpba.2020.113313
- Pavon JA, Li X, Chico S, Kishnani U, Soundararajan S, Cheung J, Li H, Richardson D, Shameem M, Yang X. Analysis of monoclonal antibody oxidation by simple mixed mode chromatography. J Chromatogr A. 2016;1431:154–65. doi:10.1016/j.chroma.2015.12.068
- Jiang H, Xu W, Liu R, Gupta B, Kilgore B, Du Z, Yang X. Characterization of bispecific antibody production in cell cultures by unique mixed mode size exclusion chromatography. Anal Chem. 2020;92(13):9312–21. doi:10.1021/acs.analchem.0c01641
- Yan Y, Xing T, Wang S, Daly TJ, Li N. Coupling mixed-mode size exclusion chromatography with native mass spectrometry for sensitive detection and quantitation of homodimer impurities in bispecific IgG. Anal Chem. 2019;91(17):11417–24. doi:10.1021/acs.analchem.9b02793
- Knight MJ, Floret L, Patel N, O’Hara J, Rodriguez E. The impact of forced degradation conditions on mAb dimer formation and subsequent influence on aggregation propensity. Mabs-austin. 2022;14(1):2127172. doi:10.1080/19420862.2022.2127172
- Lundahl MLE, Fogli S, Colavita PE, Scanlan EM. Aggregation of protein therapeutics enhances their immunogenicity: causes and mitigation strategies. RSC Chem Biol. 2021;2(4):1004–20. doi:10.1039/D1CB00067E
- Swanson MD, Rios S, Mittal S, Soder G, Jawa V. Immunogenicity risk assessment of spontaneously occurring therapeutic monoclonal antibody aggregates. Front Immunol. 2022;13:915412. doi:10.3389/fimmu.2022.915412
- Joubert MK, Hokom M, Eakin C, Zhou L, Deshpande M, Baker MP, Goletz TJ, Kerwin BA, Chirmule N, Narhi LO. et al. Highly aggregated antibody therapeutics can enhance the in vitro innate and late-stage T-cell immune responses. J Biol Chem. 2012;287(30):25266–79. doi:10.1074/jbc.M111.330902
- Bessa J, Boeckle S, Beck H, Buckel T, Schlicht S, Ebeling M, Kiialainen A, Koulov A, Boll B, Weiser T. et al. The immunogenicity of antibody aggregates in a novel transgenic mouse model. Pharm Res. 2015;32(7):2344–59. doi:10.1007/s11095-015-1627-0
- Joubert MK, Deshpande M, Yang J, Reynolds H, Bryson C, Fogg M, Baker MP, Herskovitz J, Goletz TJ, Zhou L. et al. Use of in vitro assays to assess immunogenicity risk of antibody-based biotherapeutics. PloS One. 2016;11(8):e0159328. doi:10.1371/journal.pone.0159328
- Yan Y, Xing T, Wang S, Versatile LN. Versatile, sensitive, and robust native LC–MS platform for intact mass analysis of protein drugs. J Am Soc Mass Spectrom. 2020;31(10):2171–79. doi:10.1021/jasms.0c00277