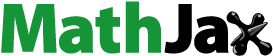
Abstract
Evaluating the rated MVA sizes of the Circuit Breaker (CB) requires calculating reactance parameters from fault analysis and synchronizing these parameters with the reference re- striking voltage. In this paper two ways are used in controlling X/R ratio of the system to reduce CB size in order to save cost on system configuration. PSS/E program was introduced to facilitate and illustrate the solution. X/R ratio and subtransient reactance were calculated to provide an approach to assess the current and duty interruption of generator buses with contributions from all other buses. The effect of reduction factors and NACD for the symmetrical component of the short circuit current is also applied in our proposed method. The obtained results proved that applying the reduction factor technique in New England system reduces the rated interruptions of C.Bs, and CB rate is also minimized in Bismayah132kV power plant from Iraqi grid utilizing effective switching which economizes choosing CB. It is concluded that the two techniques can be used successfully for designing CB ratings with a minimum cost.
PUBLIC INTEREST STATEMENT
Protection devices are essential for the security of electrical grids. Circuit breakers are vital protection strategies. Thus, several standards have studied circuit breakers’ specifications like IEC, ANSI, and IEEE standard. These devices interrupt high currents due to fault, so choosing circuit breaker specifications is of great importance to prevent currents beyond grid equipment tolerance. So short circuit current must be calculated using simulation programs to decide circuit breakers ratings; PSS®E is the most convenient one to find these ratings. For circuit breakers’ high cost, this article focusing light on the techniques that reduce their ratings with precise grid conservation as the price is proportional to ratings. The study applied two approaches, one to New England grid generator busbars and the other method is used to 400/132 kV Bismayah plant as part of the Iraqi electrical power grid. The results show a suitable reduction in ratings. Thereby, cost reduction is valuable.
1. Introduction
Short circuit (SC) arises in power system because of overloading an equipment, transmission line breakdown, lightning, switching buzz, insulation breakdown and several other causes that are entitled faults in power systems. Usually, fault causes high current to flow through lines and may result in power equipment damages if protection is not sufficient. However, this fault might cause equipment like (CBs) to expertise (SC) current which exceeds their rated capacities. To reduce SC currents, current-limiting methods are used, eliminating immediate CB necessity. CBs classification is to their rated: interrupting capacity, frequency, pressure and current; while systems are classified by their normal: frequency, operating pressure, current and current transients. Under certain disorders, the primary function of CB is to interrupt a definite current characteristic. Approximately simple and more complex methods are used to interrupt current; though useful properties categorization of CBs is due to the mixture of their application and design (Ito, Citation2019; Citation1969).
Well-known passive elements that are used to reduce fault current such as current-limiting reactors are practiced particularly in low-voltage (LV) systems. Though, in high-voltage (HV) networks some drawbacks are observed, such as affecting power flow, under normal operation, producing a voltage drop that may cause voltage collapse, so transient stability of power systems is influenced adversely (CIGRE WG A3.10, Citation2003). Thus, new technologies developed fault current limiters (FCL) to improve the aforementioned matters. These FCLs with normal operation have low impedance, while having large impedance at the beginning of a fault; thereby limiting the instantaneous first current peak below the equipment’ current capabilities, and successive current peaks to an extent allowing protection relays to operate correctly, although ranging within CBs’ interrupting capabilities.
A 3-phase power system under disturbance undergoes symmetrical and unsymmetrical faults. Generally, ungrounded 3-phase faults enforce extremely severe circuit breaker commitment, as the first interrupting phase has recovery voltage; with normal frequency; about 87% of phase-to-phase voltage. Transient stability studies the worst condition i.e. 3-phase fault; and if the system endures that fault, then the system is stable in other fault types with similar time duration of clearance as 3-phase fault. Co-coordination of overcurrent devices on time-current requires 30- cycle (SC) current. Subtransient reactance of the generator is replaced with a value greater than it or with transient reactance value (Das, Citation2012).
When SC occurs and the current gets high value, protective relay operates immediately, but separation of CB contact is achieved in a transient state. At this instant, the r.m.s. current value is the CB’ breaking current which is measured in kA.
During the first half cycle, subtransient state, the maximum peak of the current value is reached. This peak value is the current rating of CB which is definitely higher than breaking current.
Figure represents typical CB operating time. Once fault occurs, protective relays are activated, but require elapsing a definite time; detection time; to define overcurrent and initiating trip command (Bulucea et al., Citation2013). The spring mechanism of CBs’ contacts releases them. Instantly a high voltage gradient appears at the two contacts of CB, ionizing the medium, thus keeping the current to flow. This flow generates excessive heat and light forming electric arc. It is useful to elongate the arc in order to be cooled or defused thus different mechanisms are employed. Therefore CB should endure the SC current from being initiating instant to extinguishing instant (Citation1969).
Maximum instantaneous current to be tolerated by CB and total current when breaker contacts separation are the two important factors for circuit breaker selection (Garzon, Citation1997).
In actual load flow subtransient or transient reactance values are entered according to the user’s anticipation, i.e. not using the actual value, for fault studies. The transient reactance value of the generator is usually used in case of oil CB to calculate breaking currents, and in the case of the electromechanical protection relay to calculate operating currents because the effects of subtransient reactance will disappear in the early few cycles and pre CB operation. Theoretically, the subtransient reactance value of the generator should be used to calculate maximum CB making current, also for fast CBs (approximately 2 cycles; C37.04-Citation1999, 2006).
The first-cycle currents are supposed to possess no ac decrement (NACD). “First-cycle” duty sometimes may be stated as momentary or close and latch duty. Close and latch duty statement is related to CBs with the high-voltage rate, whereas (first-cycle) nominate CBs with low -voltage rate. Regardless of the calculation way for fault current; after source, the power system is represented by equivalent Thevénin impedance. For breaker selection, it is vital at the fault point to adjust the peak value to the real X/R ratio, as CB rate relies on the X/R ratio (Kasztenny & Rostron, Citation2018). For old high voltage CBs, rms calculation of asymmetrical current is built on a half cycle peak. 1.6 multiplier is used corresponding to X/R = 25 and for higher X/R, higher values are obtained. As E/X computation is done and X/R is recognized, then multiplying calculated SC current by a proper multiplying factor to complete interrupting duty calculation of high-voltage CBs. Multiplying factor values depend on: CB’ Contact parting time, X/R ratio, and finally the effects of DC and (AC and DC) decaying for remote sources and local sources respectively (Das, Citation2011; Sunil, Citation2010; Citation2019).
As fault occurs the instantaneous current contains DC component so the breaker should break SC current close to its rated SC breaking current comprising any AC component, also, any percentage DC component close to the definite rating (Avni & Qendrim, Citation2020; Lafta et al., Citation2019). The symmetrical current is multiplied by the factor 1.6 is to determine momentary SC current that CB must withstand, thereby selecting high power CB. CB interrupting current is lower than momentary current and dependent on CB speed. Some dc component may keep decaying for that interrupting current could be asymmetrical. CBs can be categorized according to their rated voltage, nominal voltage, voltage range factor (K), continuous current rating and SC current rating at operating time with highest voltage value (Ram & Vishwa Kharma, Citation2011; Citation2019).
It is convenient to present a literature review for some worked researches related to CB rating: Cornelia A. Bulucea et.al (Bulucea et al., Citation2013) determined transient recovery voltage (TRV) thru generator CB-based symmetrical components technique when SC current fed via step up transformer as they revealed that the determined value is after breaking operation. To modulate TRV characteristics and peaks occurring in an unbalanced short circuit, M. R. Barzegar and M. Niasati (Barzegar & Niasati, Citation2015) used oscillation frequency based on surge arrester and capacitor between CB terminals compared to other methods of limiting RRRV. Theoretical analysis for an out-of-phase fault was obtained by H. Liu et al. (H. Liu et al., Citation2009) on the relationship between the FCL and the RRRV and maximum TRV of a CB. Moreover, the optimum design of inductive FCL for significant effect on the maximum TRV and the RRRV of the CB was mathematically presented and calculated by Q. Li et.al (Li et al., Citation2008).
Bogdan Kasztenny and Joe Rostron (Kasztenny & Rostron, Citation2018) explained the factors influencing the asymmetrical breaker rating and calculated rating loss caused by fast CB trip also the researchers suggested custom margins for CB selection.
Avni Alidemaj and Qendrim Nika (Avni & Qendrim, Citation2020) analyzed the quick generator blackout effect on power system and studied the effect of time delay of CB operation to eliminate dc component thus the SC current component can reach zero crossing. A. Norouzi (Norouzi, Citation2020) investigates the problem of estimating a system’s X/R when parallel circuits are at the time of circuit breaker opening. Illustrative examples are provided, and the concept of changing X/R ratio is proposed to estimate the actual impact of transient currents compared with the Thévenin method.
This article studied two techniques for minimizing CB rate and TRV in order to reduce the cost. The first is by reducing X/R ratio using reduction factor and this way is applied to New England grid. The second one reduces X/R value using proper effective switching of transformers to ground thereby reducing RRRV and maximum TRV and thus reducing CB rating and consequently the cost; this approach is applied to Bismayah plant as it represents part of Iraq grid.
When designing power system, circuit breakers of generator buses should be cautiously selected and implemented; thus SC breaking current is to be calculated to determine the breaking capacity (De Metz-Noblat et al., Citation2005; Shalash & Zaharin, Citation2014). In this paper, the breakers of generator buses are mounted and experienced to interrupt short circuit currents with high asymmetries. The actual asymmetries of currents can be analytically studied for the New England system as it represents the first part of case study. Moreover, before and after applying reduction factor for each faulted generator bus: X/R ratio, complex impedance, NACD ratios, multiplying factors for breakers magnitude and phase angles of all the contributions from generator buses are calculated using PSS/E program.
The second part of case study is concerned to Bismayah power plant. Since Baghdad represents the major load center in Iraq and the most important generating station in Baghdad region is Bismayah power plant because of its generating capacity, its connections to 400 KV system and 132 KV network which results in high short circuit levels, and because its 132 KV transformers are effectively switched to ground that influences the network X/R ratio as it can be altered by opening and closing the grounding switch of those transformers and consequently multiplying factor of symmetrical current is altered.
The paper is arranged as follows; section 2 describes an overview of power circuit breakers requirements. Asymmetric and symmetric current effect on CB capacity is explained in section 3; whereas the case study is introduced in section 4. Section 5 represents the results, and conclusions are summarized in section 6.
2. Power circuit breakers requirements
Specifications of circuit breakers and switches were introduced by a number of standards comprising (C37.04-Citation1999, 2006):
IEEE Std C37.04, ANSI C37.06, IEEE Std C37.09.
IEC counterpart, IEC 62271 part 100.
C37.016- IEEE Std for high voltage switchers.
According to the standards which are used in PSS/E (e.g., ASCC, ANSI Standard, or IEC60909; Lafta et al., Citation2019), the C.B interrupting duty needs the calculation of these values:
A- Instantaneous peak current value is important with respect to the IEC standards when the CB contacts trip (PSS/E 32.0, Citation2009).
B- The equivalent root-mean-square current is used by ANSI C37 standards.
In ANSI/IEEE standards of C37.010 and C37.04, CB rating calculations are based on the objectives of the new rating structures to simplify the rating and bring the American standards closer to their IEC counterparts by including the X/R ratio which is altered accordingly to that close and open with contact parting time in the rating calculations (Huening, Citation1969). Also, E/X simplified method in C37.010–1964; as the steady-state current is calculated by dividing the voltage E by the reactance X (Norouzi, Citation2020), the symmetrical E/X current is adjusted with multipliers and compared to a breaker’s balanced rating, applies Thevenin equivalent in PSS/E.
Some specifications are to be briefly summarized explaining their objective and application.
2.1. Normal operating conditions
These specifications take into account environmental conditions, especially temperature and height. Temperature range specified in ANSI standards (−30°C to +40°C) and height below 1,000 m.
As in case of 138–13.8 kV transformer, its rated values according to temperature rise are: 40 MVA, 44.8 MVA, 60 MVA, 75 MVA.
Previous ratings are usually suitable for temperature of 40°C (annual average of 30°C), and period of 24 h. In case of (40 or 44.8) MVA based the percentage impedance value is usually specified.
2.2. Maximum operating voltage
It identifies the breaker maximum rate which is characterized by the rms value of the (line—line) voltage. IEC and ANSI standards may differ a little on the recommended nominal values, i.e. may IEC margin be 525 kV whereas ANSI is 550 kV. This variance is due to network rated voltage that is practiced in several parts in the world (Kasztenny & Rostron, Citation2018). At a given standard operating voltage the utmost CB symmetrical current value of SC is at the moment of contact parting regardless of DC component (Das, Citation2012).
2.3. Voltage Range Factor -K
The (maximum rated voltage/minimum operating voltage) ratio represents a K factor which is firstly used in old CB; where the interrupting current has an inverse relationship with operating voltage. Altering interrupting capability, according to operating voltage, is limited by a K-factor (Kasztenny & Rostron, Citation2018).
K times rated SC current represents the maximum symmetrical value of CB’ interrupting current, i.e.:
Symmetrical interrupting capacity of CB = (maximum rated voltage × Rated SC current)/Operating voltage
= K× Rated SC current
2.4. Transient recovery voltage (TRV)
The voltage appearing across circuit breaker pole after interruption is TRV. This rating is specified within the inherent TRV.
When TRV through contacts go beyond the dielectric strength, prestrikes occur (Gang Liu et al., Citation2018; Obi & Emeghara, Citation2017).
The frequency of the (TRV) is given in (Soni et al., Citation1987):
Where
fn: the normal frequency,
L: inductance of the circuit, and
C: capacitance of the circuit.
If the breakdown arises within 1/4 cycle of primary arc destruction, the event is called re-ignition and if it occurs after that period it is called restrike.
Rate of rise of re-striking voltage (R.R.R.V.) infers whether re-striking of arc occurs or not. When dielectric strength concerning the breaker contacts is less than R.R.R.V value, then the arc would re-strikes again; and vice versa (Kasztenny & Rostron, Citation2018). R.R.R.V. is influenced by recovery voltage and natural frequency of oscillations as obvious in EquationEquation (2)(2)
(2) :
The higher the natural frequency the more severe is the re-striking voltage. Thus if a fault occurs near power station busbars, then CB will be in the worst condition because when SC occurs near the bus-bars, the circuit capacitance is small, thus fn will be higher resulting in R.R.R.V to be high (Wadhwa, Citation2012). The oscillation frequency significantly influences the RRRV value, but in PSS/E, the distance (Barzegar & Niasati, Citation2015) is taken into account using SCMU activity to apply and solve the unbalanced fault (PSS/E 32.0, Citation2009).
2.5. Grid frequency
Nominal frequency decrees the rate of current change to be close to the current zero crossing, thus frequency influences CB interrupting capability. Breakers specification must be rated according to operating frequency, i.e 50 HZ or 60 HZ.
2.6. Dielectric strength
This rate is identified by successive experiments in which CB should withstand. Each experiment study overvoltage as it is inversely proportional to fault impedance (Velmurugan & Baran Chattopadhayay, Citation2020), also study transients’ aspects, including chopped wave, nominal frequency overvoltage, lightning and switching impulses.
2.7. Rated SC current
It pertains to maximum rms value of symmetrical SC current; disregarding a decayed dc component which is securely interrupted by CB. This feature was used; historically to specify the CB’s overall interrupting capacity neglecting the decayed DC component and the asymmetrical SC current is derated by the user. With MVA rating also the symmetrical SC current rate is regularly considered, allowing lower voltages operation because as MVA rate is constant, then current capability will be greater at lower voltage (Kasztenny & Rostron, Citation2018; Ram & Vishwa Kharma, Citation2011).
Re-ignition is correlated to the rate of current change thus rated continuous current value depends on CB design and its permissible temperature increases due to losses that are dissipated through contact of CB and joining resistances (Qin et al., Citation2018).
2.8. Duty cycles
Duty Cycles are related to breaker operation conditions; these cycles effect persists after interruption decreasing breaker clearing ability thru repeated interrupting and closing in quick sequence. For multi-auto-reclosing a substantial rating is needed.
In ANSI- CB have the specified interrupting below (Das, Citation2012):
(85 − 100) % of momentary interruption at operating voltage with, 3 duty cycles.
(Rated current—85% of desired momentary ability), operations that sum interrupting currents not less than 800% of desired momentary interruption ability of the CB at rated voltage.
3. Asymmetric And Symmetric Current Effect On CB capacity
Their difference is dependent on the way of addressing the asymmetry in SC current. CB’s symmetrical rating takes into account asymmetry. SC current asymmetry includes peak asymmetrical closing current, i.e. condition of closing CB on fault; decayed DC SC current in fault period with a closed breaker; and short time current.
Asymmetrical rate (S) is the necessary asymmetrical interrupting ability of each unit of symmetrical interrupting ability can be expressed by EquationEquation (3)(3)
(3) :
Where tpart is the time constant for symmetrical current and TDC is the time constant for DC current.
S = Symmetrical CB capability (at operating voltage) × suitable factor
S value is quantified as 1.0 or (1.1, 1.2, 1.3, 1.4) for CBs with time of contact separation about (4 or more cycles) or (3, 2, 1.5, 1) respectively.
Switching capacitive loads are concerned to long cables and capacitor banks. Though switched back -to- back capacitor can represent shunt capacitor banks if the maximum current changes increase; at closing; to go beyond that value by which the shunt capacitor or cable could be identified as isolated equipment. Reduction factor of AC decrement could be obtained from ; Das, Citation2012; Ram & Vishwa Kharma, Citation2011):
A system of multi SC sources, despite including local or remote generators, local or remote multiplying factors are not applied considering interconnecting impedances. At fault location, further calculations of total current provided by a number of sources are required for the calculation of the NACD (No AC Decrement) ratio that is shown in ; Citation2019).
The main terms used in CB analysis are (Ravindranath & Chander, Citation1977):
Re-striking voltage: the transient voltage appearing across contacts when the current is at or near zero during arc period.
Recovery voltage: the rms. voltage appearing across CB contacts after arc destruction. It is about the system voltage.
Arc Voltage: during arcing period, the voltage is to be raised across CB contacts. As CB contacts separate arc is formed.
The rate of decaying DC component to zero is inversely proportional to X/R ratio at fault point; as X/R ratio is low then the decay period is short, also as X/R is high then the resultant peak current is high and decay period is long exposing the breaker to high current along that period. And to solve this defect either derate the breaker rating or multiplying the symmetrical fault current by a factor.
Hence X/R ratio is utilized to find the multiplying factor (MF) in order to consider the DC offset. A proper multiplying factor is applied to find the asymmetrical value of the first cycle current and the second to fifth cycle current. Actual Current multiplying factor (AMF) can be calculated using NACD ratio as in EquationEquation (6)(6)
(6) and the resultant value is used to find the rms of the interrupting fault current (Iint.rms.; Citation2019).
Where:
MFr: remote contributions multiplying factor
MFl: local contributions multiplying factor.
The symmetrical rms. current of the interrupting fault (Iint.rms.sym) is to be found, and then evaluate the current contributions at fault location of associated buses which can be classified into remote and local buses. The local and remote current contributions are essentially dependent on X/R ratio and their values can be changed by closing or opening the effective switch that is connected to the ground. EquationEquation (7)(7)
(7) is used to determine the (rms) interrupting short circuit current (Iint.rms) which represents the circuit breaker interrupting duty.
4. Case study
This article consists of two case studies, the first case is concerned with New England system; and the second case is Bismayah plant.
1- The New England system has 34 transmission lines and 39 busbars: 10 generator buses and 29 load buses as shown in Figure .
SC level for this test system was studied to find time to reach the peak re-striking voltage and consequently the average (R.R.R.V.). As recovery voltage is 12.25 kV and time to reach maximum re-striking voltage for half cycle can be calculated from frequency of oscillation, so the average (R.R.R.V.) would be calculated using EquationEquation (2)(2)
(2) . Since L and C are used in normal frequency calculation using EquationEquation (1)
(1)
(1) , the time to reach the re- striking voltage is calculated. Circuit breakers interrupting duties are also calculated.
2- Bismayah gas power plant of 3000 MW capacity is 25 Km southeast of Baghdad and consists of 11 busbars, 8 generator buses, and two transformers for each generator. The produced power is transferred by secondary stations of 400KV and 132KV (Citation2020). Bismayah generation plant is expanded to have combined cycle gas turbine plant (Wheeb Mashi, Citation2016) as part of it. Figure shows the single line diagram of Bismayah plant and the connection of 132/33 KV substations T1&T2.
Figure 3. (A): Bismayah single line diagram. (B): Bismayah connection of 132/33 KV substations T1&T2

The SC level of Bismayah plant has been studied for its maximum and minimum value depending on X/R ratio value which is altered by transformer effective switching. Then X/R with NACD is utilized to find the multiplying factor which in turn is used to calculate circuit breakers rms interrupting SC current.
5. Results
The results are divided into two parts the first is of the New England system test and the second is of Bismayah plant assessment.
The New England grid is examined by applying SC test for each generator busbar as each machine of the ten generates 15kV, and the Maximum value of recovery voltage is 12.25 kV and peak re-striking voltage is 24.49 kV. The time to reach the peak re-striking voltage and the average (R.R.R.V.) result values are illustrated in Table .
Table 1. The time to reach the peak re-striking voltage and the average (R.R.R.V.) results
In this table frequency of oscillations when a short-circuit occurs near the generator buses, the time to reach the peak re-striking voltage and the average (R.R.R.V.) values are suddenly applied to the inductance and capacitance forming an oscillatory circuit. For example, in Table , at bus 30, the 8.8532 H is the inductance per phase of the network from the generator at bus 30 up to the point of SC also the capacitance value is 0.3815 × 10–6 F, whereas the resistance of the network is neglected as it is very small. The inductance and capacitance composition forms the network oscillatory surges producing a transient frequency of 86.6035 Hz which appears across the contacts of the CB.
Busbar 19 is tested with line to ground fault using PSSE package (Lafta et al., Citation2018). Figure shows the connections of that bus and contributions of busbars 33, 20, and 16 during the fault, as current magnitude and angle of interrupting duties are due to the current at the fault location.
Figure shows busbars 34 and 19 connected to busbar 20; which is also examined with line to ground fault showing buses contributions as vector value.
The symmetrical root mean square of a rated breaker interruption is 31.5 KA with each machine generates 15kV. To calculate the maximum CB making currents value; at fault the value of the subtransient reactance at each generator bus is used; the generated voltage is divided by the subtransient current.
The close and latch duties of the first-cycle and the interrupting duties for the rms symmetrical current were calculated in Table ; new breakers rates like X/R ratio and NACD ratio also have been found.
Table 2. First-cycle duties and interrupting duties results
Obviously all rated breakers interruption, after applying the reduction factor, are below the value of 31.5 KA. For example, bus 30 in the first-cycle duty, the ratio of X/R reduced to 35.75 as the multiplying factor is 1.3884 and the reduction factor is 0.5839, leading the first cycle close and latch duty to be 27.8 kA rms symmetrical which is the highest value compared to other buses that are listed in Table . This value is less than the breaker interrupting rating (31.5 KA). On the other hand the least value is at bus 38, rms symmetrical duty is 21.97 KA and this value represents a much more reduction as compared to the designed symmetrical root mean square of the rated breaker interruption.
Before and after applying the reduction factor, NACD ratio versus X/R ratio for various contact parting times are illustrated in Figure and Figure shows the curves of duty symmetrical current and the amplitude interrupting duty of fault current E/Z at each bus.
2-Bismayah power plant is examined by applying fault in several tests with several effective switching of transformers to ground in order to minimize circuit breaker size (rating), also X/R ratio is altered according to that close and open. This ratio is at fault location where the fault current is limited by SC impedance (De Metz-Noblat et al., Citation2005). PSSE applies Thevenin equivalent resistance and equivalent reactance to find X/R ratio (Lafta et al., Citation2019), noting that the effective switching would influence the impedance value of the zero sequence and thereby the X/R value.
shows the connections of the eight generators to plant buses and the plant connection to Baghdad south bus through the bus BSMA (Citation2020).
The minimum and maximum interrupting SC currents; utilizing X/R ratio and multiplying factor at each bus; are calculated as shown in Table and Table respectively.
Table 3. Calculating interrupting current utilizing X/R ratio and multiplying factor for minimum SC current
Table 4. Calculating interrupting current utilizing X/R ratio and multiplying factor for maximum SC current
The minimum SC current that realizes the highest reduction in (rms) interrupting current were in BSMD and BSME buses and this result is clear in Table .
Table shows the maximum SC current showing how the contributions of other buses raise the fault current.
It is interesting to know that CB ratings- rms interrupting current- are chosen depending on the minimum SC current.
illustrates the local multiplying factor versus (rms) interrupting short circuit current for both minimum and maximum SC currents.
The utilized parameter to find CB rating in New England network is the Average (R.R.R.V.) while the rated SC current in Bismayah is utilized to determine the proper CB capacity and also the effective switching of the transformer is used to minimize CB rating.
6. Conclusion
This paper explains the evaluation of circuit breakers performance criteria during fault; the presented results include the contributions of the buses in SC current regardless of its type and fault location, so remote busbars contribute the fault current whatever their distance from SC location. This is conventional from the SC computation point of view causing to ascend the average (R.R.R.V.) value.
At fault, AC degradation incorporated with impedance, factors and time to reach the peak re-striking voltage were surveyed in New England part of result to find the CB rating.
CBs ratings in the New England part are reduced using the reduction factor to control the X/R ratio in order to minimize the CB interrupting current, and the results show that the applied technique has an effective rms interrupting current margin reduction.
The study also presented a reduction in the X/R ratio of generator buses of the Iraqi national network system; Bismayah plant which is characterized by a high short circuit current and a large number of bottlenecks. But in this part of research results it is obvious that X/R is lowered by proper effective switching of transformers to ground and this technique had an explicit effect on decreasing CBs interrupting duties in 132kv side and subsequently minimizing the multiplying factor of the maximum SC current.
From the results of the two techniques used it is clear that they represent a valuable cost- reducing approach for designing CB ratings.
Disclosure statement
No potential conflict of interest was reported by the authors2021
Additional information
Funding
Notes on contributors

Nadheer A. Shalash
Nadheer A. Shalash: received the B.S. degree (2003), the M.Sc. degree (2008)/University of Technology (UOT). The Ph.D. degree in Electrical Power Engineering / UMP (Malaysia) in 2015. Assistant Professor at Faculty of Engineering of Electrical Power Techniques, Al–M’amoon University College. His current research highlights critical studies for two methods to choose circuit breaker ratings with fewer prices that can be utilized as an effective cost for power system protection modeling
Yasar N. Lafta
Yasar N. Lafta: received the B.S. degree / UOT in 1990, M.Sc. degree in Electrical engineering /Electrical & Electronic Techniques in 2011. She experienced 14 years of work as a researcher, Assistant Professor in Mechatronics Engineering department/ University of Baghdad.
Mafaza N. Lafta
Mafaza N. Lafta: received the B.S. degree in Systems and Control engineering / UOT in 1986. She started her work at the scientific research council. Now she works in supervisory control & data acquisition systems in generation, transmission &distribution networks
References
- https://www.electrical4u.com/rating-of-circuit-breaker-short-circuit-breaking-making-current/
- Avni, A., & Qendrim, N. (July 2020). Important factors for consideration during the specification of SF6 circuit breakers for high voltage generators. MDPI Publication, Energies, 13(14, 13).https://doi.org/10.3390/en13143608.
- Barzegar, M. R., & Niasati, M., “Fusion TRV limiter a solution to modify interrupting characteristics of CBs with presence of resonance type SFCL,” 2015 30th international power system conference (PSC), 2015, pp. 92–17, https://doi.org/10.1109/IPSC.2015.7827732.
- Bulucea, C. A., Rosen, M. A., Nicola, D. A., Mastorakis, N. E., & Bulucea, C. A. (2013). Approaching the processes in the generator circuit breaker at disconnection through sustainability concepts. Sustainability, 5(3), 1161–1176. https://doi.org/10.3390/su5031161
- C37.04-1999. IEEE standard rating structure for AC high voltage circuit breakers, reaffirmed 2006.
- CIGRE WG A3.10. (2003). Fault current limiters in electrical medium and high voltage systems. CIGRE Technical Brochure, 239. https://cigreindia.org/CIGRE%20Lib/Tech.%20Brochure/239%20FCL%20in%20Electrical%20MV%20and%20HV%20systems.pdf
- Committee Report on “500 kV A/C substation design criteria-summary of industry practices,” IEEE transaction on power apparatus and systems, June 1969.
- Das. (2011). Short-circuit calculations according to ANSI standards. Power Engineering (Willis).
- Das, J. C. (2012). Power system analysis, short circuit load flow and harmonics. CRC press- Taylor & Francis group.
- de Metz-Noblat, B., Dumas, F., & Poulain, C. (2005). Calculation of short-circuit current. Schneider Electric’s, Cahier Technique, (158). https://www.studiecd.dk/cahiers_techniques/Calculation_of_short_circuit_currents.pdf
- Garzon, R. D. (1997) Design and Application. High voltage circuit breakers. Marcel Dekker.
- Huening, W. C., Jr. (1969, September). Interpretation of New American national standards for power circuit breaker applications. IEEE Transactions on Industry and General Applications, IGA-5(5), 501–523. https://doi.org/10.1109/TIGA.1969.4181065
- (29 March 2019). In: IEEE Std 3002.3™, IEEE recommended practice for conducting short-circuit studies and analysis of industrial and commercial power systems. IEEE. https://doi.org/10.1109/IEEESTD.2019.8672198.
- Ito, H. (2019). Switching equipment, national council on large electric systems (CIGRE) study committee A3: High voltage equipment. Springer International Publishing AG.
- Kasztenny, B., & Rostron, J. (2018). “Circuit breaker ratings – A primer for protection engineers”, 71st Annu. Conf. for Protective Relay Engineers (CPRE). https://doi.org/10.1109/CPRE.2018.8349782
- Lafta, Y. N., Shalash, N. A., Abd, Y. N., & Al- Lami, A. A. (2018, May). Power flow control of Iraqi international super grid with two terminal HVDC techniques using PSS/E. International Journal of Control and Automation, 11(5), 201–212. https://doi.org/10.14257/ijca.2018.11.5.18
- Lafta, Y. N., Shalash, N. A., Abd, Y. N., & Al- Lami, A. A. (2019). IEC 60909 and ANSI standards comparison with ASCC based fault calculations of Iraqi power system,” cogent OA, part of Taylor & Francis Group. Journal of Cogent Engineering, 6. https://doi.org/10.1080/23311916.2019.1705654
- Li, Q., Liu, H., Lou, J., & Zou, L. (2008, April). Impact research of inductive FCL on the rate of rise of recovery voltage with circuit breakers. IEEE Transactions on Power Delivery, 23(4), 1978–1985. https://doi.org/10.1109/TPWRD.2008.921119
- Liu, G., Guo, Y., Xin, Y., You, L., Jiang, X., Zheng, M., & Tang, W. (issu 2, Feb 2018). Analysis of switching transients during energization in large offshore wind farms. MDPI Publication, Energies, 11.https://doi.org/10.3390/en11020470.
- Liu, H., Li, Q., Zou, L., & Siew, W. H. (2009, October). Impact of the inductive FCL on the interrupting characteristics of high-voltage CBs during out-of-phase faults. IEEE Transactions on Power Delivery, 24(4), 2177–2185. https://doi.org/10.1109/TPWRD.2009.2028793
- Matar H B, Al-Zubaidi S S and Al-Kindi L A. (2021). Evaluation of the Main Causes of Diesel Engine Injector Failure using Fault Tree Analysis. alkej, 17(4), 23–35. 10.22153/kej.2021.12.006
- Norouzi, A., “Fault currents, circuit breakers, and a new method for X/R calculation in parallel circuits part II: analysis and application,” 2020 IEEE/IAS 56th industrial and commercial power systems technical conference (I&CPS), 2020, pp. 1–9, https://doi.org/10.1109/ICPS48389.2020.9176786.
- Obi, P. I., & Emeghara, M. C. O. “Dynamic modeling of circuit breaker transient recovery voltage”, IEEE 3rd international conference on electro-technology for national development (NIGERCON), 7-10 Nov. 2017, https://doi.org/10.1109/NIGERCON.2017.8281957.
- PSS/E 32.0. (2009June). In: “program application Guid”. Vol. I. Siemens P.T.I:.
- Qin, T., Zhang, Y., Dong, E., & Zou, J. 2018. Characteristics of high frequency interruption for vacuum DC breakers. AIP Publishing, Phys. Plasmas Journal, 25(8), 083515. doi 10.1063/1.5028566. https://doi.org/10.1063/1.5028566.
- Ram, B., & Vishwa Kharma, D. N. (2011). “power system protection & switchgear”. McGraw-Hill Professional Publishers.
- Ravindranath, B., & Chander, M. (1977). “power system protection & switchgear”. New age Publications.
- (2020). Republic of Iraq, ministry of electricity. Planning and Studies Office.
- Soni, M. L., Gupta, P. V., & Bhatnagar, U. S. (1987). “A course in electrical power”. Dhanapatirai Publication.
- Shalash, Nadheer A., and Abu Zaharin Bin Ahmad. “Agents for fuzzy indices of reliability power system with uncerta6inty using Monte Carlo algorithm.” In 2014 IEEE 8th International Power Engineering and Optimization Conference (PEOCO2014), pp. 258–264. IEEE. DOI: 10.1109/PEOCO.2014.681443.
- Sunil, S. R. (2010). Switchgear Protection & power systems. Khanna Publishers.
- Velmurugan, P., & Baran Chattopadhayay, A. (2020). “ Sensitivity analysis of a single phase to ground fault system in connection with high impedance faults: A case study”. Taylor and Francis group, cogent engineering. https://doi.org/10.1080/23311916.2020.1770916
- Wadhwa, C. L. (2012). “electrical power systems”, new academic science limited, the control centre, 11 A Little Mount Sion. https://handoutset.com/wp-content/uploads/2022/02/Electrical-Power-Systems-by-C.-L.-Wadhwa.pdf
- Wheeb Mashi, H. (2016). Performance enhancement of the south Baghdad thermal station. Al- Khwarizmi Engineering Journal, 12(2), 90–99. https://www.iasj.net/iasj/download/a76ee657aad70743