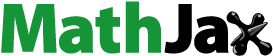
Abstract
The present experimental study aimed at investigating the effect of certain calcination temperature zones on the corrosion prevention performance of biomass. Na2SiO3 was synthesized from rice husk ash (RHA) calcined at 500°C, 550°C, and 600°C. The prepared RHA samples were characterized in terms of surface functional groups, chemical compositions, and crystallinity confirming the presence of the Si-O-Si (Silanol) group, an abundant constituent of SiO2, and the amorphous nature of the silica, respectively. The major step in the synthesis of Na2SiO3 involves a reaction of sodium hydroxide with RHA (SiO2). The effect of inhibitor dose and immersion time on corrosion rate and inhibition efficiency was investigated on mild steel prepared strips. The interaction of the parameter that results in maximum corrosion efficiency of 95.41% was recorded at 30 ml of inhibition dose and 144 hours of immersion time in a sample prepared from 600°C calcined RHA. Isotherm study resulted in best fit on Temkin isotherm model for all samples, a kinetic model was best represented by pseudo-second-order for 500°C and 600°C thermally treated samples and pseudo-zero-order kinetic model for 550°C. The thermodynamic model revealed important parameters including activation energy, enthalpy, and entropy confirming the endothermic process, feasibility, and energy barrier formed on the metal surface. Generally, the effect of low-temperature extracted Na2SiO3 showed a significant effect on the buildup of protective layer which is determined by the amount of silica content and reactivity.
1. Introduction
Corrosion is the spontaneous and irreversible deterioration of metals and alloys by chemical or electrochemical reactions in the presence of moisture in their environment. It is a natural situation and it has a huge outcome on maintenance and repair costs in the industry (Anaee, Citation2014; Karekar et al., Citation2018; Pramudita et al., Citation2019). It is very difficult to eliminate completely on the metal surfaces and it is considered one of the major industrial problems, mainly in the systems involving water such as cooling systems, heating systems (boilers), steel pipelines, etc. (Asra et al., Citation2017; Awizar et al., Citation2013; Govardhane & Shende, Citation2021). A study by the National Association of Corrosion Engineers (NACE) conducted in 2016 G.C. estimated that the global cost of corrosion to be $ 2.5 trillion annually, equivalent to about 3.4% of the global Gross Domestic Product (GDP; Hou et al., Citation2017; Skovhus et al., Citation2017). However, the cost can be reduced enormously in the range of $375-875 billion annually by applying highly efficient corrosion inhibiting technologies (Koch, Citation2017; Tang, Citation2019; Xavier Stango & Vijayalakshmi, Citation2018). Due to the high costs and problems associated with corrosion-resistant materials, the use of corrosion inhibitors is a realistic and cost-effective alternative that can be designed.
Mild steel is the foremost broadly utilized alloy in the world and its appreciable mechanical strength and low cost make it a very crucial material for the manufacturing of metallic items. It is a low-carbon alloy of iron with small additions of elements such as manganese and silicon (Ismail, Citation2016; Sunday Isaac Fayomi & Patricia Idowu Popoola, Citation2019; Zeino et al., Citation2018). Mild steel has versatile applications and it is commonly used in marine industries, chemical processing, refineries, metal processing, construction works, petroleum production, structural beams, car bodies, kitchen appliances, cans, cooling structures of network piping, heat exchangers, and water and wastewater systems (Astuti et al., Citation2022; Murmu et al., Citation2019; Panchenko et al., Citation2020; Pithawala & Jain, Citation2012; Zeino et al., Citation2018). But, the service year and performance of mild steel made structures and equipment significantly reduced due to this material is highly susceptible to corrosion. As a result, mild steel, in general, cannot be used without corrosion inhibitors (Panchenko et al., Citation2020; Xavier Stango & Vijayalakshmi, Citation2018). Mild steel corrosion happens when this metal surface is in contact with water, acids, bases, salts, oil, and other solid and liquid chemicals (Fayomi et al., Citation2018). The main factors that increase the corrosion rates are temperature, solution concentration, pH, flow rate, and so on (Pramudita et al., Citation2019; Singh et al., Citation2020).
Prevention of corrosion is a vital aspect of controlling and maintaining the preservation of metals and alloys. There are different ways to reduce the degradation of mild steel, such as modifying its composition, and surface properties and avoiding the corrosive environment. The use of corrosion inhibitors is a pivotal method to immensely protect mild steel from corrosion by reducing the corrosion rate on its surfaces from corrosive environments. Corrosion inhibitors are chemicals that react with a metallic surface, and it gives the surface a certain level of protection. Inhibitors prevent the corrosion of a metallic surface by adsorbing themselves on the metallic surface to form a protective film. Inhibitors are normally distributed from a solution to trim down the attack of corrosive ions in the solution which is in contact with the metal substrate (Xavier Stango & Vijayalakshmi, Citation2018). The corrosion process of mild steel can be slowdown using inhibitors by increasing the anodic or cathodic polarization behavior, minimizing the movement or diffusion of ions to the metallic surface, and enhancing the electrical resistance of the metallic surface (Emregül et al., Citation2006). Corrosion inhibitors are classified according to their chemical structure, method of action, etc. Generally, corrosion inhibitors are classified as inorganic and organic corrosion inhibitors. Inorganic, organic, and synthetic inhibitors are mostly used inhibitors in various industries and they have proven practically and theoretically as efficient corrosion inhibitors in a wide range of corrosive environments. However, these inhibitors have serious environmental deterioration and a dreadful impact on marine life as excessive usage of the inhibitor has exposed to the environment. These inhibitors have also major limitations such as low efficiency, high cost, and release of toxic chemicals as by-products during their synthesis and they have serious adverse effects on human health and the environment which restricts their practical usage (Emregül et al., Citation2006; Umoren & Eduok, Citation2016). With the increase in concerns for the environment among scientists and researchers, they continue to develop green corrosion inhibitors because it is biodegradable, inexpensive, abundantly available, and environmentally sound which do not contain heavy metals or other toxic compounds and provide end products with improved properties (Gupta et al., Citation2021).
Recently, plant-based natural products have been found to exhibit good corrosion inhibition efficiency. Among those plants which can be used as corrosion inhibitors include rice husk, coconut fiber, bamboo, wheat husk, corn straw, sawdust, tea leaves, guava leaves, soybeans, coffee, etc. (Xavier Stango & Vijayalakshmi, Citation2018). The main focus of this study is to develop a corrosion inhibitor from rice husk because rice husk is an excellent source of silica powder. The composition of rice husk is cellulose (50%), lignin (25%-30%), silica (15 %-20%), and moisture (10%-15%) (Dry mass based %; Gupta et al., Citation2021; Sa¸hin et al., Citation2020). Depending on the source of the Rice Husk (RRH) when it incinerated, it generates 17–20% RHA which made up of about 87–93% silica and small amount of other elements (Asra et al., Citation2017; Da Silva Nuernberg et al., Citation2021). 759 million tons of RHA were produced in 2017 and it is rich in silica that is amorphous or crystalline forms, depending on the combustion conditions time, temperature, heating rate, calcination, and cooling atmosphere (Da Silva Nuernberg et al., Citation2021). Silica is one of the inorganic compounds that is used as a potential green corrosion protector for mild metal substrates (Asra et al., Citation2017; Madlangbayan et al., Citation2021; Da Silva Nuernberg et al., Citation2021). Because silicate (SiO2) has good adhesion, a medium for good protection and it can resist the diffusion of water vapor, ions, and oxygen to the metal surface to prevent the metal surface from corrosion (Wang et al., Citation2019). It acts as an anodic and cathodic inhibitor (Ismail, Citation2016), which adsorbs onto the metal surface to form a thin protective layer. Also, silicate corrosion inhibitors are non-toxic, low cost, abundantly available, environmentally friendly, and considered natural corrosion inhibitors (Madlangbayan et al., Citation2021). Silicates are excellent corrosion inhibitors for mild steel (Pramudita et al., Citation2019; Wang et al., Citation2019), carbon steel (Othman et al., Citation2016; Wang et al., Citation2019), zinc (Aramaki, Citation2001), magnesium (Gao et al., Citation2011), galvanized steel (Lan Lin et al., Citation2008), and aluminum (Hamdy, Citation2006; Lan Lin et al., Citation2008). Furthermore, the high surface area and nano size of silica allow it to eliminate corrosion by forming a silica network and this network forms thin layers that can act as a physical barrier that prevents various contaminants potentially initiateing corrosion. Alkali silicate anti-corrosion coating also known as silicate conversion coating is one example of an alkali-treated anti-corrosion coating. The coating structure contains polymeric particles such as silanol groups (Si-OH) and siloxane bridge (Si–O–Si). During the corrosion process, the polymerization proceed with and more siloxane groups transformed to siloxane bridges (Bonetti et al., Citation2019; Cheng et al., Citation2020; Da Silva Nuernberg et al., Citation2021).
The current research work emphasized on the extraction of aqueous Na2SiO3 extracted from RRH to produce an environmentally sound corrosion inhibitor for the application of withstanding corrosion from mild steel immersed in tap water. More than 90 % of the RHA is silica that makes it economical, abundantly available and suitable material to efficiently protect the corrosion of mild steel (Azat et al., Citation2019; H. Bahri et al., Citation2015; Madlangbayan et al., Citation2021; Rajan & Kathirvel, Citation2021; Wang et al., Citation2019). Since silica constituent played an important role in inhibition process by creating a protective film it payed important attention in this particular research work. The amount of silica is dependent on the calcination temperature that determines the surface activity of the inhibition process. A number of previous research work has been done with a focus on the changing of operational parameters in the inhibition process. This research finds the gap that treatment methods are recognized as the most important aspect to increase the efficiency of the inhibitor. Hence, the originality of this paper lies on the effect of low calcination temperature on the corrosion rate and inhibition efficiency of inhibitor applied on mild steel. Through this investigation the effect of other parameters in every calcined treatments were investigated including immersion time and inhibitor dose. To strengthen the analysis and further predict the behavior of the inhibitor, different adsorption models were implemented that include isotherm, kinetics and thermodynamic studies.
2. Methodology
2.1. Metals preparation
Corrosion studies were performed on Mild steel. Prior to the corrosion process, the mild steel was mechanically cut into coupons (2 cm × 4 cm × 0.1 cm). The surface of each coupon was then polished using 800, 1200, and 220 abrasion papers to obtain a smooth area and was then washed with distilled water. They were cleaned again with acetone (99%) and ethanol (70%) and, finally, dried using an oven at 105°C for 12 hours then weighed in the digital balance. The average weight of the metal strips was 4.5 ± 0.1 g which is recorded as an initial weight before corrosion.
2.2. RHA preparation
RRH were collected from local rice husk producers and millers found in South Gondar, Ethiopia. The received rice husk was thoroughly washed using distilled water and the cleaned rice husk was dried in the sun for two days followed by calcination at 500oC 550°C and 600°C for 6 hours using a laboratory scale muffle furnace.
2.3. Characterization of RHA
A number of combined methods were employed to quantitatively determine the silicate and other chemical content (i.e. minor oxide major oxide and loss of ignition) of RRH burnet at different temperatures. These analyses were conducted in the geochemical laboratory, Ethiopian geological survey, Addis Ababa, Ethiopia. LIBO2 fusion, HF attacks, and gravimetric and atomic absorption spectrophotometry were used to analyze the metallic oxide constituent of the RHA T-500, RHA T-550, and RHA T-600. FTIR analysis was carried out on the RHA powder to identify the active functional groups. Two mg of RHA powder was mixed thoroughly with 200 mg of potassium bromide (KBr) and homogenized in an agate mortar. The mixture was then placed in the sample compartment of Infrared Fourier Transform Spectroscopy (FT/IR-6600typeA) to determine the main functional groups . The measurement was conducted between the ranges from 400 to 4000 cm−1 at a resolution of 4 cm−1. The sample crystallinity was evaluated using the X-ray diffraction technique and was performed on an X-ray Diffractometer SHIMADZU-7000 Corporation (Tokyo, Japan) using copper radiation CuKα (λ = 0.154 nm), at an accelerating voltage of 40 kV and an operating current of 30 mA. All patterns are recorded in the range of 2θ (10°–80°). 0.25 g of each sample was pressed under 50 MPa to form pellets having an average of 25 mm in diameter. The final result was presented in terms of 2θ (degrees) vs. intensity (count).
2.4. Formulation of inhibitor
The Na2SiO3 solution was prepared by dissolving 10 g of RHA in 60 ml of 1 M NaOH solutions. The RHA and sodium hydroxide solution was mixed in a 100 ml conical flask for an hour and stirred at a constant agitation speed. The reaction was operated at ambient temperature and atmospheric pressure. The reaction temperature rises to 55 ± 1°C due to heat produced by the exothermic reaction. Then, the solution was filtered by a vacuum filter to separate the insoluble matters like silica and other oxides (K2O, MgO, CaO, Al2O3, Fe2O3, etc.) from the Na2SiO3 solution. Pure Na2SiO3 solution found at the lower layer after centrifugation was taken for a corrosion inhibition experiment. The stoichiometry relation of Na2SiO3 synthesized from rice husk ash is described in Equationequation (1)(1)
(1) . The overall schematic diagram is shown in Figure .
2.5. Inhibition performance
The weight loss method is widely used as a simple and reliable method to measure the inhibition efficiency of Na2SiO3 and corrosion rate. The mild steel samples were pre-cleaned using various grid papers and weighed before and after exposure to the solution. The corrosion performance of mild steel coupons was investigated by immersing coupons in 200 ml of tap water with various Na2SiO3 inhibitor doses (5, 10, 15, 20, 25 and 30ml) extracted from different RAH calcination temperatures (500, 550, and 600°C) at varied immersion time (48, 96 144, 192, 240 and 288 h.). The weight loss measurements were conducted by total immersion of the pre-weighed mild steel coupons. Then, the specimens were washed according to the standard guide for laboratory immersion corrosion testing metals (ASTM G-31) and weighed again. The inhibition efficiency (IE %), degree of surface coverage (ϴ), and corrosion rate (CR) were calculated from the measured data according to Equationequation (2)(2)
(2) , Equationequation (3)
(3)
(3) , and Equationequation (4)
(4)
(4) , respectively (Al-Amiery et al., Citation2020; Behpour et al., Citation2010; Bhawsar et al., Citation2015; Venkatesan & Pazhani, Citation2016):
Where Wo and Wi are the weight in the absence and presence of the inhibitor; A is an area of the mild steel (cm2); ρ is the density of mild steel in g/cm3 (7.85 g/cm3); T is exposure time (h)
2.6. Adsorption isotherm
Adsorption isotherm studies give the descriptive mechanism of how the inorganic inhibitors adsorb to the metal surface. The adsorption isotherm model best describes the adsorption of Na2SiO3 inhibitor on mild steel. It is obtained by fitting the corrosion rate, CR, and the degree of surface coverage of the inhibitor, θ into the various adsorption isotherms models such as Langmuir (Tsoeunyane et al., Citation2019), Freundlich (Alaneme & Olusegun, Citation2012), and Temkin (Nwabanne & Okafor, Citation2012) expressed in linear form as in Equationequation (5(5)
(5) ), Equationequation (6)
(6)
(6) , and Equationequation (7)
(7)
(7) , respectively:
Where Ɵ is the degree of surface coverage, C is the inhibitor concentration, and Kads equilibrium adsorption constant.
3. Result and discussion
3.1. Silicate analysis
According to the silicate analysis results, silica is the primary component among the other compounds present. Table shows the silicate analysis of RHA interms of weight percentage treated at different temperatures along with minor oxides including Al2O3, Fe2O3, K2O, MgO, Na2O, and CaO. From the result, it can be observed that 85.86% of SiO2 was present in an RHA treated at 600°C, which is the highest among the other samples, similar result was reported by recent research work (S. Bahri et al., Citation2019). However, the SiO2 content found in RHA treated at 550°C and 500°C is slightly lower having 84.26% and 80.50% respectively. Other than SiO2 RHA treated at 600°C is composed of 2.91% of Al2O3, 0.95% of Fe2O3, 2.04% of CaO, 2.36 of MgO, and 3.19 of K2O. The sample treated at 550°C is composed of 3.05% of Al2O3, 1.15% of Fe2O3, 1.82 % of CaO, 2.42% of MgO, and 4.17% of K2O. Rice husk burnet at 500°C has high amount of other oxides including Al2O3 (3.15%), Fe2O3 (2.5%), CaO (2.34%), MgO (2.96 %) and K2O (6.2%). The amount of Na2O is lower than 1% in all treated samples. The SiO2 percentages of all samples are comparable to the previous studies (Jembere, Citation2019; Lemessa Jembere, Citation2017). The composition of RHA depends upon many factors including agricultural practice and geographical location; as a result, a range of compositions of RHA has been reported (S. Bahri et al., Citation2019; Nwabanne & Okafor, Citation2012).
Table 1. Chemical composition of different RHA samples
3.2. X-Ray diffraction
A comparative XRD plot for RRH and thermally treated samples is presented to analyze and investigated the change in their crystallinity. The amorphous phase of silica was produced by burning RRH at different temperatures including 500°C, 550°C, and 600°C for 6 hours. Figure shows the X-ray diffraction pattern of different samples. There is a broad peak at 22.32° (RH), 21.46° (RHA-500), 21.72° (RHA-550), and 22.1° (RHA-600). A similar result was obtained by previous research work that reported a broad peak of 22° (Yuvakkumar et al., Citation2014). In all burning temperatures, silica with amorphous properties is portrayed by wide-ranging peaks in XRD analysis. However, the intensity is quite different in each sample. Amorphous silica from RHA was an important phase in this study because it can be easily dissolved and reactive as it contains high porosity and high surface area (Della et al., Citation2002). Additionally, due to the presence of hydroxyl group found on the surface of silica causes the formation of a reactive site. Amorphous characteristics of silica indicate that the silica synthesized is environmentally safe. Silica with a crystalline phase nature is carcinogenic to the environment and hazardous to the living organism (Demadis et al., Citation2007).
3.3. FTIR
Fourier Transform Infrared (FTIR) was employed to examine the functional groups of the different samples of RHA, and the FTIR spectra are shown in Figure . FTIR spectroscopy is used to study the effect of temperature to the surface functional groups of RHA. Besides the porosity, adsorption behavior is influenced by the chemical reactivity of the surface especially in the form of chemisorbed oxygen in various forms of functional groups. The peak at 3470 cm−1 ascribed from organic Si–O-H stretching with contribution from any water might retained in the sample or other mineral derived from hydroxyl group. It is observed that the intensity of the hydroxyl peak decreased for the calcination temperature increment from 500°C to 600°C which indicates that moisture is decreasing as a result of loss of Hydrogen and oxygen atoms due to the breaking bond from hydroxyl group due to increase in temperature. Peaks at 1214 cm−1 are assigned to Si-O asymmetrical stretching. Formation of a new peak was observed when rice husk is subjected to thermal treatment at different temperatures. The characteristic vibrations generally between 2800 and 3750 cm−1 are represented by Silinol hydroxyl (Si–O–H) groups and adsorbed water. This functional group is responsible for the adsorption of a metal particle on the surface of mild steel. The intensity of the corresponding bond for the Si–O–H group was decreasing with the temperature variation. The most dominant peak from the spectrograph was the wavelength between 1000 to1100 cm−1 attributed to Si-O-Si (silanol) reveal the presence of siloxane (Della et al., Citation2002; Kalderis et al., Citation2008; Yuvakkumar et al., Citation2014). From Figure the corresponding silixone group ranges from 1082.83 to 1106.87 cm−1. A new peak was formed for thermally treated samples (500°C—600°C). The new peak around 808 cm−1 is characterized as a symmetric stretching vibration mode of the tetrahedral SiO4 bond and Si-O is shown by the strong bending band vibration around 476 cm−1 (Demadis et al., Citation2007; Javed et al., Citation2010). These show the presence of nano-structured SiO2. Variation of peaks at different temperatures was observed which has a significant effect on the formation. Spectra of RRH in the region 1200–1000 cm−1 is expected to result from superposition of vibrations of the C-OH bond and Si-O bond in the siloxane (Si-O-Si) groups. Intense band at 1082 cm−1 corresponds to the stretching vibrations of SiO4 tetrahedrons. Strong intensity of this peak is expected due to superposition of the stretching vibrations of the C-OH bond in the interval 1200–1000 cm−1 and the stretching vibrations of the Si-O bond. The peak at 485 cm−1 was due to the bending vibration of siloxane bonds (Aktas & Morcali, Citation2011). The wide and intense band with maximum about 3448 cm–1 can be attributed either to the valent vibrations of the O–H bond in water molecules bonded by hydrogen bonds, or to OH groups present in cellulose, hemicellulose and lignin (Nada et al., Citation2002). The band at 1644 cm−1 results from both the deformation vibrations of the water molecules and the presence of C = C bonds in the organic component. The intense absorption band at 2930 cm–1 can be attributed to the asymmetric valent vibrations of the C–H bonds in—CH3 groups in the structures of lignin, cellulose and hemicellulose.
3.4. The effect of inhibitor dose
The inhibition efficiency of Na2SiO3 at a dose of 5, 10, 15, 20, 25, and 30 ml, and burning temperature of the rice husk at 500, 550, and 600°C, at an exposure time of 144 min are presented in Figure . Overall, it is observed that the inhibition efficiency of the mild steel metal increased with increasing concentrations of inhibitors. The addition of inhibiter treated at 500°C with a concentration of 5 ml results in 54.16% of inhibition efficiency. The inhibition efficiency of the inhibitor-treated at 550°C and 600°C in the same dosage concentration was 58.33% and 60.41% respectively. Further increase in the dosage from 5 ml to 25 ml increases the efficiency to 89.58%, 94.58% and 95.41% for 500°C, 550°C and 600°C respectively. This behavior could be attributed to the increase in adsorption of inhibitors on the metal or at the solution interface as the concentration increases. It is known that the portion of the metal surface covered by the adsorbed molecule increases with increased inhibitor concentration. However, when the dosage increases to 30 ml, the performance of inhibition on the mild steel stayed steady. At the final dosage, the thermally treated samples at 600°C caused maximum inhibition efficiency. This is due to the enrichment of amorphous SiO2 as the burning temperature increase. A sufficient amount of SiO2 present in the inhibitor could have a better chance of reacting with mild steel. Treatment samples at 550°C were also a good inhibitor that brought a close proximity value of inhibition efficiency with 600°C. Insufficient inhibition might be caused either by less amount of SiO2 or less amount of dosage of the inhibitor in the solution. The formation of a less harmful surface to the mild steel could be due to inadequate concentration of inhibitor to stabilize the degradation effect of the corrosive anions. According to the early research, it was depicted that the reduction in the free surface for anodic action (reduction in surface available for cationic action) increases the current density at any place where there is no protective film. This results in accelerated corrosion and pitting (Schneider & Stumm, Citation1964). Therefore, a high amount of dosage is capable to preserve the film throughout the entire system.
Figure 4. Effect of inhibitor dose and calcination temperature on (a) inhibitor efficiency and (b) corrosion rate of the mild steel immersed in tap water for exposure time of 144 min and at room temperature.

The corrosion rate drastically decreased as the inhibitor concentration increased. At 5 ml of inhibitor dosage, the corrosion rate was 0.00122, 0.00111 and 0.00159 mm/year at 500, 550, and 600°C, respectively. It is clear to notice that, the initial inhibitor dosage of the sample treated at 600°C experienced a higher corrosion rate. This is due to the degree of reactivity varied with incineration or crystallization temperature. The reactivity of silica with ions will be low as it is transformed partially into crystalline. In another word, Si–O bonds are liberated from the long polymeric chains (Si–O–C/Si–O–Si) at elevated temperatures (below the crystallization point of silica). When the pyrolysis temperatures are increased, more and more Si–O and C–O/C–C are released, which gradually transform into crystalline silica (cristobalite) and crystalline carbon (graphite). At higher inhibitor dose the corrosion rate drops to 0.00029, 0.00014 and 0.00020 mm/year for sample calcined at 500°C, 550°C and 600°C, respectively.
3.5. The effect of immersion time
The effect of immersion time was investigated at inhibition dose of 15 ml and calcination temperature at 500°C, 550°C and 600°C. Figure depicts the effect of immersion time and burning temperature on the inhibition efficiency and corrosion rate of mild steel. Inhibition efficiency reduced gradually in every treatment temperature from 77.08 to 72.08% at 500°C, 91.66% to 83.75% at 550°C and 87.97% to 77.08% at 600°C. There was a slight increment at the onset for the sample treated at 600°C from 87.92% to 88.33% when increasing the impression period from 48 to 96 h which then followed by a rapid decline. Highest inhibition efficiency was recorded for sample treated at 550°C throughout the immersion period. At the beginning, 91.67% inhibition efficiency was recorded which then declined to 89.58% at 96 h of immersion period then remained steady for the next 48 h followed by a rapid decline to 84.17% and remained constant till the end of the immersion period. When sufficient amount of inhibitor are adsorbed on the mild steel surface the adsorption density tends to be high which in turn decreases the interaction between inhibitor molecules through Van der Waals force. Consequently, there might be inhibitor molecules leaving the surface triggering a reduction in the effective area occupied by the inhibitor and thus causing decrease inhibition efficiency. Sample treated at 550°C was able to maintain the stability of the inhibition efficiency after 192 h of exposure that might be attributed to a reactive amorphous silica at the given calcination temperature.
Figure 5. Effect of exposure time at different burning temperature on (a) inhibition efficiency and (b) corrosion rate of the mild steel immersed in tap water with inhibition dose of 15 ml and room temperature.

In contrast, lower corrosion rate was recorded by T-550°C throughout the duration of immersion time followed by 600°C and 500°C. This is directly associated to the inhibition efficiency because efficient inhibitor was able to reduce the rate of corrosion. Mild steel exposed for 48 hours registered 0.000668 mm/year and it was dropped to 0.000217 during 288 h. Overall, the corrosion rate of the metal decreased with the increment of immersion time. The observation is accurate because reaction rates generally decrease with time. The inhibitor inhibition capability increases instantly at the onset. This is because the inhibitor coated the metal surface in a good manner and it prevents the attack of corrosion as immersion time increases. Silicate prevents by creating a thin silicate film on the surface of a metal as the silicate is introduced and the thickness is not increasing with time. The protection is sustained once the film is formed. When the silicate treatment is stopped, the protection is progressively lost. The formed silicate film is self-healing in case of damage as long as a continual feed of the inhibitor is carried out. As the burning temperature increase from 500 to 550°C the corrosion rate decreases slightly but as temperature increases from 550 to 600°C the corrosion rate increase. As the result, the corrosion rate at 550°C is low compared to other samples. This may be due to a good amorphous phase was emerged at this burning temperature.
3.6. Adsorption isotherms
The mechanism of corrosion inhibition can be well understood through isotherm. The isotherm was developed by taking different inhibition dose and calculating the corresponding ɵ. Figure shows the various adsorption isotherm models considered in this study. The R2 values for each isotherm model are presented in Table . This is important to determine the most suitable model that can represent the experimental data. The experimental data were able to fit into Langmuir (Figure ), Freundlich (Figure ) and Temkin isotherms (Figure ). Temkin isotherm with R2 values of about 0.99 best describes the adsorption mechanism of RHA inhibitor on mild steel. Therefore, Temkin adsorption isotherm is appropriate for evaluating the adsorption equilibrium constant, Kads. The Kads values may be taken as an indication of the strength of the adsorption forces between the inhibitor molecules and the mild steel surface (Ameer & Fekry, Citation2010). Tumkin adsorption mechanism confirms a monolayer adsorption of inhibitor on a uniform mild steel.
Table 2. Langmuir, Freundlich and Temkin adsorption isotherm parameters
3.7. Anodic adsorption by SiO3−2
Na2SiO3 extracted from RHA as inhibitors act as cathodic and anodic inhibitors, nevertheless, as a general rule, acts through a process of surface adsorption, designated as a film-forming. Certainly, the existence of molecules exhibiting a high affinity for metal surface compounds shows high inhibition efficiency and of course low environmental risk. These inhibitors during the inhibition process develop a protective hydrophobic film adsorbed molecules on the metal surface, that offers a barrier to the dissolution of the metal and they solubilize in the medium neighboring the metal surface. The silicates are considered anodic inhibitors, initially forming a film on anodic areas. In the anodic areas, the oxidation of a metal occurs as shown in Equationequation (8)(8)
(8) :
The negatively charged siliceous species can react with the metallic cations to form the protective film. However, the most important process in the inhibition, which is the silicate film on the metal, is formed by the adsorption mechanism as shown in Figure .
Na2SiO3 prevents the formation of corrosion by going through an anodic reaction on mild steel due to the interaction of SiO32- and Fe2+ ions and forming a corrosion-resistant protective film-based iron silicate (FeSiO3) and deposited on the anodic region of the mild steel.
3.8. Cathodic adsorption by Na+
During the inhibition process, the cathodic corrosion inhibitors inhibit the occurrence of the cathodic reaction on the metal surface. These inhibitors have metal ions that are capable to produce a cathodic reaction due to their alkalinity nature, consequently forming insoluble compounds that precipitate selectively on cathodic sites.
As can be seen from Figure , the medium contains cations of water molecule reacting with oxygen as shown in EquationEquation (9)(9)
(9) to give rise to OH− as given in EquationEquation (10)
(10)
(10) , the cation constituent of the inhibitor contains Na+, then interact with OH− to form a basic solution NaOH which deposit over the metal. This protective film is often characterized as a compact and adherent film, restricting the dispersion of reducible species around the metal areas. Increasing the diffusion restriction of the reducible species will cause high cathodic inhibition. Furthermore, a Cathodic reaction is responsible for increasing the pH value.
3.9. Kinetics
A kinetic model including Zero order, Pseudo-first-order, and second-order kinetic models was investigated for the corrosion inhibition of mild steel by using Equationequation (11)(11)
(11) , Equationequation (12)
(12)
(12) , and Equationequation (13)
(13)
(13) , respectively. Figure shows the kinetic study of the adsorption in corrosion inhibition by different samples of Na2SiO3. Different kinetic parameters were calculated from the plot as recorded in Table , these are kinetic constant (K) and half-life (t1/2), and the values of correlation coefficient R2 were obtained from the analysis of different kinetic models to determine the fitness of the samples to the model.
Table 3. Kinetic parameters for adsorption by different treated samples
The half-life of the process can be calculated as per Equationequation (14(14)
(14) ; Eddy et al., Citation2009).
The sample treated at 500°C resulted in the best fit for a pseudo-second-order kinetic model with the determination of coefficient of R2 = 0.9835, based on this the rate constant, K is 0.0004 h−1 and the half-life is around 17,325 h. With respect to the corrosion inhibition performed by the sample treated at 550°C, clearly notify that the zero-order kinetic model can best represent the experimental results with R2 = 0.9047. Sample treated at 550°C resulted zero order that indicates the rate is independent of inhibitor dose or concentration. This might be associated with 550°C, which might have created a reactive amorphous surface. The corresponding value of K is 0.0049 h−1 and the half-life is 141.4 h. The corrosion inhibition with a Na2SiO3 prepared from a sample treated at 600°C resulted in the highest fitness to pseudo-second-order kinetic model with 0.9967. The corresponding kinetic parameters are 0.0001 h−1 and 6930 h.’ of K and half-life respectively.
3.10. Thermodynamics study
Thermodynamic study was performed by varying operational temperature from 303 to 363 K at 15 ml and 144 hr inhibition dose and immersion time, respectively. The linearized form of the Arrhenius equation defined the correlation between the corrosion rate (CR) and temperature (T) as can be expressed in Equationequation (15)(15)
(15) .
Where Ea is the activation energy, R is the gas constant, T is the temperature (oK) and A is the Arrhenius pre-exponential factor. The activation energy was determined from the slope of a plot CR against 1/T as shown in Figure ). Enthalpy change and entropy change were calculated through EquationEquation (16)(16)
(16) , which is an alternative formula for the Arrhenius equation in the transition state (Ebenso, Citation2003).
Figure 9. Thermodynamic plot for determination of (a) activation energy and (b) enthalpy and entropy.

Linearizing the above equation yields EquationEquation (17)(17)
(17) :
Simplifying the equation yields Equationequation (18)(18)
(18) :
Where h is Planck’s constant, N is the Avogadro’s number equivalent to 6.0221408e+23 mol−1, ΔS is the entropy change, ΔH is the enthalpy change and R is the universal gas constant equal to 8.314 J/K.mol.
The enthalpy change, ΔH was evaluated from the slope of the plot (Figure ) of
against and
the entropy change, ΔS was evaluated from the intercept
of the same plot. The activation energy, Ea, evaluated at different sample treatments for T-500°C, T-550°C, and T-600°C calcination temperature is presented in Table . The higher value of Ea in the presence of an inhibitor was due to the increased energy barrier. This occurrence indicates the development of a complex compound between the inhibitor and mild steel (Erna et al., Citation2019). From the result, it is important to note that the corrosion inhibitor is feasible as the increase in energy barrier for the metal dissolution. The reason for the increased activation energy with increasing the calcination temperature is due to higher SiO2 content forming a strong film on mild steel metal surface, which creates a barrier at the anodic inhibition. The values of enthalpy change (ΔH) and entropy change (ΔS) obtained at different samples are also given in Table . The calculated ΔH values are positive for all samples indicating an endothermic corrosion inhibition process. This phenomenon indicates that high temperature accelerates the corrosion process of mild steel, and thus is ascribed to chemical adsorption. However, the value of entropy change on the other hand was negative which indicates a decrease in disorderliness that indicate the effectivness of the inhibitor to prevent wearing out of mild steel.
Table 4. Thermodynamics and activation energy parameters
3.11. Comparison with related studies
RHA extract inhibitor treated at different temperature is compared to different extract inhibitors as can be depicted from Table . Sample T-550°C and T-600°C are comparable with most of high efficient inhibitors as shown from Table .
Table 5. Comparison of various extract inhibitors performance
4. Conclusion
Generally, low temperature calcined RHA granted a reactive silicate solution that potentially offers an advantage of minimizing the corrosion rate and increases the inhibition efficiency of mild steel. Advanced characterization was able to describe the RHA sample surface consists a functional group responsible for inhibition such as Si-O-Si, XRD analysis confirmed that the samples calcined at different temperatures consist amorphous phase of silica. It was proved that the amount of silicon content resulting from different calcination temperature determines the degree of reactivity with the metal surface. Due to this, a RHA-600°C inhibits the corrosion by 95.41% with corresponding value 0.000184 mm/year of corrosion rate. Sample treated at 550°C also brought in a good agreement with 600°C. Effect of immersion time and inhibitor dose significantly affected inhibition efficiency and corrosion rate. The interaction of the parameter that results in maximum corrosion efficiency was 30 ml of inhibition dose and 144 hours of immersion time in a sample prepared from 600°C calcined RHA. Corrosion inhibition was further analyzed using adsorption isotherm models, and all samples are best fitted in the Temkin model. The kinetic model revealed RHA calcined at 500°C and 600°C best fitted to second order kinetic model while sample calcined at 550°C best fitted to zero order kinetic model. The thermodynamic study resulted in important thermodynamic parameters and generally indicated the feasibility of the process.
Disclosure Statement
No potential conflict of interest was reported by the author(s).
Additional information
Funding
References
- Aktas, S., & Morcali, M. H. (2011). Gold uptake from dilute chloride solutions by a Lewatit TP 214 and activated rice husk. International Journal of Mineral Processing, 101(1–4), 63–21. https://doi.org/10.1016/j.minpro.2011.07.007
- Al-Amiery, A., Salman, T. A., Alazawi, K. F., Shaker, L. M., Kadhum, A. A. H., & Takriff, M. S. (2020). Quantum chemical elucidation on corrosion inhibition efficiency of Schiff base: DFT investigations supported by weight loss and SEM techniques. International Journal of Low-Carbon Technologies, 15(2), 202–209. https://doi.org/10.1093/ijlct/ctz074
- Alaneme, K. K., & Olusegun, S. J. (2012). Corrosion inhibition performance of lignin extract of sun flower (Tithonia Diversifolia) on medium carbon low alloy steel immersed in H2SO4 solution. Leonardo Journal of Science, 20(20), 59–70.
- Ameer, M. A., & Fekry, A. M. (2010). Inhibition effect of newly synthesized heterocyclic organic molecules on corrosion of steel in alkaline medium containing chloride. International Journal of Hydrogen Energy, 35(20), 11387–11396. https://doi.org/10.1016/j.ijhydene.2010.07.071
- Anaee, R. A. M. (2014). Sodium silicate and phosphate as corrosion inhibitors for mild steel in simulated cooling water system. Arabian Journal for Science and Engineering, 39(1), 153–162. https://doi.org/10.1007/s13369-013-0865-x
- Aramaki, K. (2001). Inhibition effects of chromate-free, anion inhibitors on corrosion of zinc in aerated 0.5 M NaCl. Corrosion Science, 43(3), 591–604. https://doi.org/10.1016/S0010-938X(00)00085-8
- Arockiasamy, P., Sheela, X. Q. R., Thenmozhi, G., Franco, M., Sahayaraj, J. W., & Santhi, R. J. (2014). Evaluation of corrosion inhibition of mild steel in 1 M hydrochloric acid solution by Mollugo cerviana. International Journal of Corrosion, 2014, 1–7. https://doi.org/10.1155/2014/679192
- Asra, D., Othman, N. K., & Dasuki, Z. (2017). Nano-silicate inhibitor derived from rice husk for corrosion mitigation of steel in distilled water. AIP Conference Proceedings, 1838. https://doi.org/10.1063/1.4982176
- Astuti, P., Rafdinal, R. S., Yamamoto, D., Andriamisaharimanana, V., & Hamada, H. (2022). Effective Use of sacrificial zinc anode as a suitable repair method for severely damaged RC members due to chloride attack. Civil Engineering Journal, 8(7), 1535–1548. https://doi.org/10.28991/CEJ-2022-08-07-015
- Awizar, D. A., Othman, N. K., Jalar, A., Daud, A. R., Rahman, I. A., & Al-Hardan, N. H. (2013). Nanosilicate extraction from rice husk ash as green corrosion inhibitor. International Journal of Electrochemical Science, 8(2), 1759–1769. http://electrochemsci.org/papers/vol8/80201759.pdf
- Azat, S., Sartova, Z., Bekseitova, K., & Askaruly, K. (2019). Extraction of high-purity silica from rice husk via hydrochloric acid leaching treatment. Turkish Journal of Chemistry, 43(5), 1258–1269. https://doi.org/10.3906/kim-1903-53
- Bahri, H., Danaee, I., Rashed, G. R., & Zaarei, D. (2015). Effect of silica ratio on the corrosion behavior of nano-silica potassium silicate coatings on aluminum alloy 2024. Journal of Materials Engineering and Performance, 24(2), 839–847. https://doi.org/10.1007/s11665-014-1325-9
- Bahri, S., Mahmud, H. B., Shafigh, P., & Majuar, E. (2019). Mechanical and durability properties of high strength high performance concrete incorporating rice husk ash. IOP Conference Series: Materials Science and Engineering, 536(1), 012028. https://doi.org/10.1088/1757-899X/536/1/012028
- Behpour, M., Ghoreishi, S. M., Mohammadi, N., Soltani, N., & Salavati-Niasari, M. (2010). Investigation of some Schiff base compounds containing disulfide bond as HCl corrosion inhibitors for mild steel. Corrosion Science, 52(12), 4046–4057. https://doi.org/10.1016/j.corsci.2010.08.020
- Bhawsar, J., Jain, P. K., & Jain, P. (2015). Experimental and computational studies of Nicotiana tabacum leaves extract as green corrosion inhibitor for mild steel in acidic medium. Alexandria Engineering Journal, 54(3), 769–775. https://doi.org/10.1016/j.aej.2015.03.022
- Bhuvaneswari, T. K., Jeyaprabha, C., & Arulmathi, P. (2020). Corrosion inhibition of mild steel in hydrochloric acid by leaves extract of Tephrosia purpurea. Journal of Adhesion Science and Technology, 34(22), 2424–2447. https://doi.org/10.1080/01694243.2020.1766395
- Bonetti, S., Spengler, R., Petersen, A., Aleixo, L. S., Merlo, A. A., & Tamborim, S. M. (2019). Surface-decorated silica with Schiff base as an anticorrosive coating for aluminium alloy 2024-T3. Applied Surface Science, 475(January), 684–694. https://doi.org/10.1016/j.apsusc.2018.12.298
- Cheng, L., Lou, F., & Guo, W. (2020). Corrosion protection of the potassium silicate conversion coating. Vacuum, 176(March), 109325. https://doi.org/10.1016/j.vacuum.2020.109325
- da Silva Nuernberg, N. B., Niero, D. F., & Bernardin, A. M. (2021). Valorization of rice husk ash and aluminum anodizing sludge as precursors for the synthesis of geopolymers. Journal of Cleaner Production, 298, 126770. https://doi.org/10.1016/j.jclepro.2021.126770
- Della, V. P., Kühn, I., & Hotza, D. (2002). Rice husk ash as an alternate source for active silica production. Materials Letters, 57(4), 818–821. https://doi.org/10.1016/S0167-577X(02)00879-0
- Demadis, K. D., Mavredaki, E., Stathoulopoulou, A., Neofotistou, E., & Mantzaridis, C. (2007). Industrial water systems: Problems, challenges and solutions for the process industries. Desalination, 213(1–3), 38–46. https://doi.org/10.1016/j.desal.2006.01.042
- Ebenso, E. (2003). Effect of halide ions on the corrosion inhibition of mild steel in H2SO4 using methyl red. Bulletin of Electrochemistry, 19, 209–216. https://www.researchgate.net/publication/279892720_Effect_of_halide_ions_on_the_corrosion_inhibition_of_mild_steel_in_H_2SO4_using_methyl_red_-_Part_1
- Eddy, N. O., Ekwumemgbo, P. A., & Mamza, P. A. P. (2009). Ethanol extract of Terminalia catappa as a green inhibitor for the corrosion of mild steel in H 2 SO 4. Green Chemistry Letters and Reviews, 2(4), 223–231. https://doi.org/10.1080/17518250903359941
- El-Haddad, M. A. M., Bahgat Radwan, A., Sliem, M. H., Hassan, W. M. I., & Abdullah, A. M. (2019). Highly efficient eco-friendly corrosion inhibitor for mild steel in 5 M HCl at elevated temperatures: Experimental & molecular dynamics study. Scientific Reports, 9(1), 1–15. https://doi.org/10.1038/s41598-019-40149-w
- Emregül, K. C., Düzgün, E., & Atakol, O. (2006). The application of some polydentate Schiff base compounds containing aminic nitrogens as corrosion inhibitors for mild steel in acidic media. Corrosion Science, 48(10), 3243–3260. https://doi.org/10.1016/j.corsci.2005.11.016
- Erna, M., Herdini, H., & Futra, D. (2019). Corrosion Inhibition mechanism of mild steel by amylose-acetate/carboxymethyl chitosan composites in acidic media. International Journal of Chemical Engineering, 2019, 1–12. https://doi.org/10.1155/2019/8514132
- Faiz, M., Zahari, A., Awang, K., & Hussin, H. (2020). Corrosion inhibition on mild steel in 1 M HCl solution by Cryptocarya nigra extracts and three of its constituents (alkaloids). RSC Advances, 10(11), 6547–6562. https://doi.org/10.1039/c9ra05654h
- Fayomi, O. S. I., Akande, I. G., Oluwole, O. O., & Daramola, D. (2018). Effect of water-soluble chitosan on the electrochemical corrosion behaviour of mild steel. Chemical Data Collections, 17–18, 321–326. https://doi.org/10.1016/j.cdc.2018.10.006
- Gao, H., Li, Q., Chen, F. N., Dai, Y., Luo, F., & Li, L. Q. (2011). Study of the corrosion inhibition effect of sodium silicate on AZ91D magnesium alloy. Corrosion Science, 53(4), 1401–1407. https://doi.org/10.1016/j.corsci.2011.01.008
- Govardhane, S., & Shende, P. (2021). Integration of green nanotechnology with silica for corrosion inhibition. Corrosion Reviews, 39(3), 211–218. https://doi.org/10.1515/corrrev-2020-0115
- Gupta, R., Bhardwaj, P., Mishra, D., Sanghi, S. K., & Amritphale, S. S. (2021). Novel non-hydroxyl synthesis and fabrication of advanced hybrid inorganic-organic geopolymeric coating material for corrosion protection. International Journal of Adhesion and Adhesives, 110, 102951. https://doi.org/10.1016/j.ijadhadh.2021.102951
- Hamdy, A. S. (2006). Corrosion protection of aluminum composites by silicate/cerate conversion coating. Surface and Coatings Technology, 200(12–13), 3786–3792. https://doi.org/10.1016/j.surfcoat.2005.03.012
- Hou, B., Li, X., Ma, X., Du, C., Zhang, D., Zheng, M., Xu, W., Lu, D., & Ma, F. (2017). The cost of corrosion in China. Npj Materials Degradation, 1(1). https://doi.org/10.1038/s41529-017-0005-2
- Ismail, A. (2016). A review of green corrosion inhibitor for mild steel in seawater. ARPN Journal of Engineering and Applied Sciences, 11(14), 8710–8714. http://www.arpnjournals.org/jeas/research_papers/rp_2016/jeas_0716_4665.pdf
- Javed, S. H., Naveed, S., Feroze, N., Zafar, M., & Shafaq, M. (2010). Crystal and amorphous silica from KMnO4 treated and untreated rice husk. Journal of Quality Technology and Management, VI(1), 81–90. http://pu.edu.pk/images/journal/iqtm/PDF-FILES/paper-5-Volume_VI_Issue-1-June-2010.pdf
- Jayakumar, S., Nandakumar, T., Vadivel, M., Thinaharan, C., George, R. P., & Philip, J. (2020). Corrosion inhibition of mild steel in 1 M HCl using Tamarindus indica extract: Electrochemical, surface and spectroscopic studies. Journal of Adhesion Science and Technology, 34(7), 713–743. https://doi.org/10.1080/01694243.2019.1681156
- Jembere, A. L. (2019). Extraction of silica gel from rice husk ash for promising sustainable industrialization: Statistical analysis. Journal of Materials Science and Engineering with Advanced Technology, 18(1–2), 21–42. https://doi.org/10.18642/jmseat_7100122004
- Kalderis, D., Bethanis, S., Paraskeva, P., & Diamadopoulos, E. (2008). Production of activated carbon from bagasse and rice husk by a single-stage chemical activation method at low retention times. Bioresource Technology, 99(15), 6809–6816. https://doi.org/10.1016/j.biortech.2008.01.041
- Karekar, S. E., Bagale, U. D., Sonawane, S. H., Bhanvase, B. A., & Pinjari, D. V. (2018). A smart coating established with encapsulation of Zinc Molybdate centred nanocontainer for active corrosion protection of mild steel: Release kinetics of corrosion inhibitor. Composite Interfaces, 25(9), 785–808. https://doi.org/10.1080/09276440.2018.1439631
- Khadom, A. A., Abd, A. N., & Ahmed, N. A. (2018). Xanthium strumarium leaves extracts as a friendly corrosion inhibitor of low carbon steel in hydrochloric acid: Kinetics and mathematical studies. South African Journal of Chemical Engineering, 25, 13–21. https://doi.org/10.1016/j.sajce.2017.11.002
- Koch, G. (2017). Cost of corrosion. Elsevier Ltd.
- Lan Lin, B., Tang Lu, J., & Kong, G. (2008). Synergistic corrosion protection for galvanized steel by phosphating and sodium silicate post-sealing. Surface and Coatings Technology, 202(9), 1831–1838. https://doi.org/10.1016/j.surfcoat.2007.08.001
- Lemessa Jembere, A. (2017). Studies on the synthesis of silica powder from rice husk ash as reinforcement filler in rubber tire tread part: Replacement of commercial precipitated silica. International Journal of Materials Science and Applications, 6(1), 37. https://doi.org/10.11648/j.ijmsa.20170601.16
- Madlangbayan, M. S., Diola, C. N. B., Tapia, A. K. G., Peralta, M. M., Peralta, E. K., Almeda, R. A., Bayhon, M. A. L., & Sundo, M. B. (2021). Corrosion inhibition of sodium silicate with nanosilica as coating in pre-corroded steel. Civil Engineering Journal, 7(11), 1806–1816. https://doi.org/10.28991/cej-2021-03091761
- Manh, T. D., Huynh, T. L., Thi, B. V., Lee, S., Yi, J., & Nguyen Dang, N. (2022). Corrosion inhibition of mild steel in hydrochloric acid environments containing sonneratia caseolaris leaf extract. ACS Omega, 7(10), 8874–8886. https://doi.org/10.1021/acsomega.1c07237
- Murmu, M., Saha, S. K., Murmu, N. C., & Banerjee, P. (2019). Effect of stereochemical conformation into the corrosion inhibitive behaviour of double azomethine based Schiff bases on mild steel surface in 1 mol L−1 HCl medium: An experimental, density functional theory and molecular dynamics simulation study. Corrosion Science, 146, 134–151. https://doi.org/10.1016/j.corsci.2018.10.002
- Muthamma, K., Kumari, P., Lavanya, M., & Rao, S. A. (2021). Corrosion inhibition of mild steel in acidic media by N-[(3,4-Dimethoxyphenyl)Methyleneamino]-4-Hydroxy-Benzamide. Journal of Bio- and Tribo-Corrosion, 7(1), 1–19. https://doi.org/10.1007/s40735-020-00439-7
- Nada, A. M. A., Abou Yousef, H., & El-Gohary, S. (2002). Thermal degradation of hydrolyzed and oxidized lignins. Journal of Thermal Analysis and Calorimetry, 68(1), 265–273. https://doi.org/10.1023/A:1014925803350
- Nwabanne, J. T., & Okafor, V. N. (2012). Adsorption and thermodynamics study of the inhibition of corrosion of mild steel In H2SO4 medium using Vernonia Amygdalina. Journal of Minerals and Materials Characterization and Engineering, 11(9), 885–890. https://doi.org/10.4236/jmmce.2012.119083
- Omran, M. A., Fawzy, M., Mahmoud, A. E. D., & Abdullatef, O. A. (2022). Optimization of mild steel corrosion inhibition by water hyacinth and common reed extracts in acid media using factorial experimental design. Green Chemistry Letters and Reviews, 15(1), 214–230. https://doi.org/10.1080/17518253.2022.2032844
- Othman, N. K., Yahya, S., & Awizar, D. A. (2016). Anticorrosive properties of nano silicate from paddy husk in salt medium. Sains Malaysiana, 45(8), 1253–1258. https://core.ac.uk/download/pdf/84306697.pdf
- Panchenko, Y. M., Marshakov, A. I., Nikolaeva, L. A., & Igonin, T. N. (2020). Evaluating the reliability of predictions of first-year corrosion losses of structural metals calculated using dose-response functions for territories with different categories of atmospheric corrosion aggressiveness. Protection of Metals and Physical Chemistry of Surfaces, 56(7), 1249–1263. https://doi.org/10.1134/S207020512007014X
- Pithawala, N. A., & Jain, B. (2012). Journal of advanced scientific research. Advancement of Science, 1(2), 19–23. [Online]. Available. http://www.sciensage.info/journal/1359303580JASR_3006121.pdf
- Pramudita, M., Sukirno, S., & Nasikin, M. (2019). Synergistic corrosion inhibition effect of rice husk extract and KI for mild steel in H2SO4 solution. Bulletin of Chemical Reaction Engineering & Catalysis, 14(3), 697–704. https://doi.org/10.9767/bcrec.14.3.4249.697-704
- Rajan, H. S., & Kathirvel, P. (2021). Sustainable development of geopolymer binder using sodium silicate synthesized from agricultural waste. Journal of Cleaner Production, 286, 124959. https://doi.org/10.1016/j.jclepro.2020.124959
- Sa¸hin, E. A., Solmaz, R., Gecibesler, I. H., & Kardaş, G. (2020). Adsorption ability, stability and corrosion inhibition mechanism of Phoenix dactylifera extrat on mild steel. Materials Research Express, 7(1), 016585. https://doi.org/10.1088/2053-1591/ab6ad3
- Schneider, C. R., & Stumm, W. (1964). Evaluation of corrosion in distribution systems. International Journal of Mineral Processing, 56(5), 621–632. https://doi.org/10.1002/j.1551-8833.1964.tb01252.x
- Sehmi, A., Ouici, H. B., Guendouzi, A., Ferhat, M., Benali, O., & Boudjellal, F. (2020). Corrosion inhibition of mild steel by newly synthesized pyrazole carboxamide derivatives in HCl acid medium: Experimental and theoretical studies. Journal of the Electrochemical Society, 167(15), 155508. https://doi.org/10.1149/1945-7111/abab25
- Singh, A. K., Chugh, B., Thakur, S., Pani, B., Lgaz, H., Chung, I.-M., Pal, S., & Prakash, R. (2020). Green approach of synthesis of thiazolyl imines and their impeding behavior against corrosion of mild steel in acid medium. Colloids and Surfaces A: Physicochemical and Engineering, 599(April), 124824. https://doi.org/10.1016/j.colsurfa.2020.124824
- Skovhus, T. L., Eckert, R. B., & Rodrigues, E. (2017). Management and control of microbiologically influenced corrosion (MIC) in the oil and gas industry—Overview and a North Sea case study. Journal of Biotechnology, 256(Mic), 31–45. https://doi.org/10.1016/j.jbiotec.2017.07.003
- Sunday Isaac Fayomi, O., & Patricia Idowu Popoola, A. (2019). Corrosion propagation challenges of mild steel in industrial operations and response to problem definition. Journal of Physics: Conference Series, 1378(2), 022006. https://doi.org/10.1088/1742-6596/1378/2/022006
- Tang, Z. (2019). A review of corrosion inhibitors for rust preventative fluids. Current Opinion in Solid State and Materials Science, 23(4), 1–16. https://doi.org/10.1016/j.cossms.2019.06.003
- Tsoeunyane, M. G., Makhatha, M. E., & Arotiba, O. A. (2019). Corrosion inhibition of mild steel by Poly(butylene succinate)-L-histidine Extended with 1,6-diisocynatohexane polymer composite in 1 M HCl. International Journal of Corrosion, 2019, 1–12. https://doi.org/10.1155/2019/7406409
- Umoren, S. A., & Eduok, U. M. (2016). Application of carbohydrate polymers as corrosion inhibitors for metal substrates in different media: A review. Carbohydrate Polymers, 140, 314–341. https://doi.org/10.1016/j.carbpol.2015.12.038
- Umoren, S. A., Solomon, M. M., Obot, I. B., & Suleiman, R. K. (2018). Comparative studies on the corrosion inhibition efficacy of ethanolic extracts of date palm leaves and seeds on carbon steel corrosion in 15% HCl solution. Journal of Adhesion Science and Technology, 32(17), 1934–1951. https://doi.org/10.1080/01694243.2018.1455797
- Venkatesan, R. P., & Pazhani, K. C. (2016). Strength and durability properties of geopolymer concrete made with ground granulated blast furnace slag and black rice husk ash. KSCE Journal of Civil Engineering, 20(6), 2384–2391. https://doi.org/10.1007/s12205-015-0564-0
- Wang, C., Chen, J., Hu, B., Liu, Z., Wang, C., Han, J., Su, M., Li, Y., & Li, C. (2019). Modified chitosan-oligosaccharide and sodium silicate as efficient sustainable inhibitor for carbon steel against chloride-induced corrosion. Journal of Cleaner Production, 238, 117823. https://doi.org/10.1016/j.jclepro.2019.117823
- Xavier Stango, S. A., & Vijayalakshmi, U. (2018). Studies on corrosion inhibitory effect and adsorption behavior of waste materials on mild steel in acidic medium. Journal of Asian Ceramic Societies, 6(1), 20–29. https://doi.org/10.1080/21870764.2018.1439608
- Yuvakkumar, R., Elango, V., Rajendran, V., & Kannan, N. (2014). High-purity nano silica powder from rice husk using a simple chemical method. Journal of Experimental Nanoscience, 9(3), 272–281. https://doi.org/10.1080/17458080.2012.656709
- Zeino, A., Abdulazeez, I., Khaled, M., Jawich, M. W., & Obot, I. B. (2018). Mechanistic study of polyaspartic acid (PASP) as eco-friendly corrosion inhibitor on mild steel in 3% NaCl aerated solution. Journal of Molecular Liquids, 250(November), 50–62. https://doi.org/10.1016/j.molliq.2017.11.160