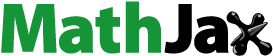
Abstract
Recently, extreme climatic events have been a major concern across the world. Assessments on various climates and geographic regions are needed to understand the uncertainties in extreme climate events’ responses to global warming. Developing countries particularly Ethiopia is highly affected by such extreme climate events due to its low adaptive capacity. The aim of this study was to investigate the trends of daily extreme temperatures indices in Ethiopia. The changes and trends in climate extremes of temperature were evaluated based on 14 indices of extreme temperatures over Ethiopia. The Mann-Kendall (MK) trend test is used to investigate the trends in the selected datasets. In the present study, three zones, which represent 34 stations, were selected based of extreme climate indices. The results of 14 extreme temperature indices showed that warm days (TX90p) and nights (TN90p) increased by 2.65 (P < 0.05) and 2.04 (P < 0.05) days/decade, respectively. The annual maximum and minimum temperatures (TXam and TNam) as well as the diurnal temperature range (DTR) were 2.68/decade, 2.04/decade and 0.62/decade, respectively. 73.5% and 26.5% of the stations show an increasing trend for (TXx and TNx), respectively. The extreme hot temperature indices (TXx, TXn, TX90, TN90 and SU) and cold temperature indices (ID, TNn, TXn, TX10P, TN10P and FD) exhibited contrasting variations over the stations. As far as spatial patterns concerned, most of the stations show significant increasing trends in northern and southeastern parts of Ethiopia. Thus, changes in climate extremes will likely increase the risk of severe extreme events and the society to become more vulnerable to the climate shocks. Generally, the findings of this study could help decision and policymakers to take immediate measures to tackle the climate change impacts.
Public interest statements
Climate change and variability have increasingly become pressing scientific concerns in recent decades. Currently, extreme climate events like flooding, drought and frequent heat waves are becoming more intense than ever before. These significantly pose serious impacts on ecosystems, human wellbeing and cause complex environmental hazards. Thus, this study investigated the long-term trends of extreme temperatures indices in Ethiopia. The 14 indices of extreme temperatures were calculated based on daily maximum and minimum temperatures data using correlation analysis. The findings showed that the trends of temperature have increasing throughout the country during the study period. Furthermore, the increasing trends in the number of warm days and nights were observed at most stations. Despite, the small number of homogenous temperature data series, the study could present a proportion of extreme temperatures in Ethiopia for selected stations.
1. Introduction
Nowadays, climate change and variability have increasingly become pressing scientific concerns in recent decades (Qiaohong Sun et al., Citation2014; Vormoor et al., Citation2016). Climate change is the single biggest threat facing the global food system (Jabal et al., Citation2022). Extreme weather and climatic events have been a worldwide issue due to their severe impacts on human life (W. Sun et al., Citation2015).
Temperature and precipitation are two essential climatic variables in determining the climate of a region; their changes affect different hydro-climate systems directly or indirectly. Changes in climate events in terms of frequency and intensity have a powerful impact on the environment and human beings (Gedefaw et al., Citation2018; Shrestha et al., Citation2016). Currently, extreme climate events like flooding, drought, and frequent heat waves are becoming more intense than ever before (IPCC, Citation2013; Nama et al., Citation2022). Changes in extreme events show large regional variations because of impacts of climate changes on geographically diverse regions (Liu et al., Citation2013; Ren et al., Citation2011; Xu et al., Citation2011).
These significantly pose serious impacts on ecosystems, human wellbeing and cause complex environmental hazards (Fathian et al., Citation2022). The alteration of the Earth’s atmospheric composition has caused a change in global climate system (Mbungu et al., Citation2012). It has been reported that the Earth’s temperature has increased by 0.74°C in the last 100 years. Changes in the frequency and intensity of extreme climate events are likely to have an immediate and intense impact on society and the environment (Nama et al., Citation2022; Shrestha et al., Citation2016). Understanding and give due attention for the spatial and temporal distribution of these events are very essential (Zarrin et al., Citation2022).
Ethiopia is exposed to climate change hazards due to its low adaptive capacity (Gedefaw et al., Citation2018). The implications for the change in temperature are significantly affects particularly arid and semi-arid regions of Ethiopia where temperature extreme is a common event (Aronica & Bonaccorso, Citation2013). Temperature extremes directly or indirectly affect the human health, crop production, environment and energy demand (Jabal et al., Citation2022; Suwarno et al., Citation2021; Wen et al., Citation2017; Yenehun et al., Citation2017). Currently, the number of cold days and nights has decreased globally. On the other hand, the number of warm days and nights has increased on worldwide scale from 1951 to 2010 (IPCC, Citation2013). Thus, climate change poses severe threats to the world (Jabal et al., Citation2022; Saddique et al., Citation2020), but the impacts are not the same globally (Sillmann et al., Citation2013).
Most studies have done so far have mainly focused on changes in mean observations of temperature and precipitation datasets across the globe (Zhao et al., Citation2015; Badjana et al., Citation2017; Randell & Gray, Citation2016; Gong, Citation2018; Bring & Destouni, Citation2013; Gocic & Trajkovic, Citation2013; Zhang et al., Citation2013; Ahmedou et al., Citation2008). Recent, similar studies on hydro climatic trends conducted in Ethiopia (Gedefaw et al., Citation2018; Tekleab et al., Citation2013; Yenehun et al., Citation2017; Sawan, Citation2017; Seleshi & Zanke, Citation2004; Asfaw et al., Citation2017; Behailu et al., Citation2014; Girma et al., Citation2016). However, no comprehensive study has yet been done to identify the trends and changes in its climate extremes in Ethiopia. Some studies in the world like You et al. (Citation2008) studied the changes in temperature extremes for the Tibetan Plateau and found decreasing trends in cold days/nights and increasing warm days/nights. Klein Tank et al. (Citation2006) also reported increasing number of warm days/nights and decreasing trends of cold days/nights. Thus, based on the literature review and state of the art indicated above, high spatiotemporal variability of climate extreme events are revealed which needs to improve the datasets for accurate simulation and projection. Hence, the innovation of the present study is to conduct a comprehensive study and investigate the trends associated with climate extremes with respect to climate change in Ethiopia. Therefore, the objectives of the present study are (i) to quantify the changes in temperature extremes and (ii) to identify the trends and changes associated with 14 extreme climate indices in Ethiopia.
2. Materials and methods
2.1. Description of study area
Ethiopia is found between 3° −15° North latitude and 33°-48° East longitude. It has a total area of about 1.13 million km2. The Great Rift Valley of the eastern Africa divides the country into two plateaus and stretches from north-east to south-west with 40–60 km wide flat-lying plain in the east, south and west borders of the country that has an elevation of around 600 m above mean sea level (Asfaw et al., Citation2017). The annual average precipitation of the country is 848 mm with 24.5°C annual mean temperature. Figure shows location map of meteorological stations of Ethiopia. The stations were purposely selected to represent the upper zone, middle zone and lower zones of Ethiopia based of extreme climate indices. The stations have different climate variation in different seasons. Thus, all parts of the country were included in this analysis.
2.2. Data sources
Historical records of daily minimum and maximum temperatures of Ethiopia for the period (1980–2016) were collected from the National Meteorological Services Agency of Ethiopia (NMSA) for the present study. Location of gauging stations in the map of Ethiopia is shown in Figure and description of all stations is given in Table . The stations were divided into three zones (Upper, Middle and Lower), which represent the assigned region.
Table 1. Description of temperature gauge stations and time period of data
Daily temperature data from 34 meteorological stations for the period of 1980–2016 was used for this study. There is no specific guideline on the appropriate number of decimal places to use data when presenting climate change. This would have an impact on the interpretation of results and conclusion. The temperature readings are usually taken one decimal place and therefore, it is appropriate to consider only one significant decimal to present temperature data. However, scientists used different decimal digits to present yearly temperature trends. Intergovernmental Panel for Climate Change (IPCC) uses one decimal place to show the change in temperature over a century and two decimal places to show the change over a decade (IPCC, Citation2013). Other recent studies also used two decimal digits. Therefore, one decimal digit is used in this study to show changes in temperature. On the other hand, to analyze the temperature extremes, the following preconditions are applied to select the meteorological stations. (a) All months should contain complete data unless 5 or less days; (b) complete yearly data; and (c) stations have complete data except upto 5 years missing data. Therefore, based on these parameters, 34 stations are selected (Table ).
2.3. Indicators
Various investigation methods were carried out for quality controls of data. Investigating the quality of data is a necessary step before calculations of indices. This is because error outliers can seriously affect the trends (Wang et al., Citation2012). In line with this, the maximum temperature less than 0°C, maximum temperature less than the minimum temperature, minimum temperature greater than 20°C is not accepted. After controlling the quality of data and homogeneity assessment, extreme temperature indices were computed from the daily data. In addition, annual and seasonal trends of minimum and maximum temperatures and indicators were analyzed in this study.
The Expert Team on Climate Change Detection and Indices (ETCCDI) defined 27 daily rainfall and temperature extreme indices, which covers a wide range of climates (Wagesho et al., Citation2012). In this study, 14 extreme temperature indices were selected from the list of ETCCDI considering their importance in Ethiopia (Table ). Some indices were based on threshold defined as percentiles. The percentiles were calculated from the reference period 1971–2000, which is a climate normal period defined by World Meteorological Organization (Theobald et al., Citation2016).
Table 2. Temperature indices selected for this study
The extremes indices used in this study are categorized into three classes. The warm temperature extreme indices are the number of summer days (SU), the highest daily maximum and minimum temperatures (TXx and TNx), the numbers of warm days and nights (TX90p and TN90p). The cold temperature extreme indices are the number of ice days (ID), the lowest daily maximum and minimum temperatures (TXn and TNn), the numbers of cold days and nights (TX10p and TN10p) and the number of frost days (FD). The third type includes the annual mean maximum and minimum temperatures (TXam and TNam) and the diurnal temperature range (DTR).
2.4. Methods
2.4.1. Mann—Kendall Test for Monotonic trends
The non-parametric Mann-Kendall (MK) test is commonly employed to detect monotonic trends in series of climate data or hydrological data which was first developed by Kendall, Citation1975 and Mann, Citation1945 (Pingale et al., Citation2014). It is used to detect the trends in time series data of precipitation and temperature. The flowchart of the methods of this study is shown (Figure ). A positive or negative value of MK (Z) value indicates an upward or downward trend, respectively. At 95% significance level, the null hypothesis of no trend is rejected if |Z| > 1.96. Mann—Kendall statistic (S) for a time series X1, X2, X3 …, and Xn is calculated as (Gedefaw et al., Citation2018):
The probability associated with S and the sample size, n, is then computed to statistically quantify the significance of the trend using normalized test statistic Z as follows:
The variance is computed as (Gedefaw et al., Citation2018):
where n is the number of data points, q is the number of tied groups and tp is the number of data values in the pth group.
2.4.2. Analysis of the Extreme temperature
The level of significance was taken at 95% confidence interval for the statistical significance of the correlation coefficient.
3. Results and discussion
3.1. Trends in annual mean temperature
This study revealed that the maximum-recorded temperature was found to be 36.7°C at Degahabur station and the minimum was 20.4oC in Fitche station. The maximum temperature was increased by (0.3–6.4 °C) during 1980–2016. On the other hand, the hot temperature extreme was observed in Degahabur station with a rate of 9.9°C. Figure shows the trends of maximum temperature from 1980 to 2016. It is clearly observed that the MK test shows a decreasing trend of temperature (1982–1993) and increasing trend (1994–2016). A significant decreasing trend of annual mean temperature was observed (1987–1999). However, the significant increasing trends of annual temperature have been seen (1982–1984). On the other hand, the annual maximum and minimum temperatures (TXam and TNam) and the diurnal temperature range (DTR) in the country during the study period (1980–2016) were 2.68/decade, 2.04/decade and 0.62/decade, respectively.
The results of the present study are comparable with the findings such as (Asfaw et al., Citation2017; Behailu et al., Citation2014; Tekleab et al., Citation2013).
3.2. Trends in seasonal temperature
Average daily seasonal temperature data were used to assess the trends of temperature. There is seasonal variation of temperature from 1980 to 2016. The results indicated that the calculated temperature in each season is as follows: Summer (25.0°C), Winter (27.7°C), Spring (28.5°C) and Autumn (25.9°C). There is also significant spatial variation in the mean range of temperature. This change may express the diurnal variation and demanding atmospheric moisture (Subash & Sikka, Citation2013). From the trend of maximum and minimum temperature, it was observed that the magnitude of the mean value change reached by 4.9°C/year and 3.8°C/year, respectively. The results revealed that there were high fluctuations of seasonal temperature across the stations. The same results were reported in (Asfaw et al., Citation2017; Behailu et al., Citation2014; Gedefaw et al., Citation2018).
Time series of maximum and minimum temperatures at three zones for summer and winter season are shown in Figures .
3.3. Trends in extreme temperature indices
3.3.1. Hot temperature extremes (TXx, TNx, TX90p and TN90p and SU)
The highest daily maximum and minimum temperatures (TXx and TNx) have increased from 1980 to 2016. The monthly maximum value of daily maximum temperature (TXx) trend increased at about 73.5% of stations for the period 1980–2016 and 26.5% of the stations show an increasing trend for (TNx). Most of these stations are found in northern and southeastern parts of the country. The average hot days (TX90p) and nights (TN90p) increased by 2.65 (P < 0.05) and 2.04 (P < 0.05) days/decade, respectively (Table ). This result is consistent with the results of Soltani et al. (Citation2016) who reported an increasing trend with the same indices in Iran. Similar findings have been also reported by (IPCC, Citation2013; Rahimzadeh et al., Citation2009) for most regions in the world.
Table 4. Decadal change of extreme temperature indices
As far as seasonal variation concerned, all seasons show marked increasing trend except TX90p in spring season. 70.59% of the stations show an increasing trend in summer days (SU), 6 days/decade.
3.3.2. Cold temperature extremes (ID, TNn, TXn, TX10p, TN10p and FD)
The lowest daily minimum temperature (TNn) and the lowest daily maximum temperature (TXn) increased from 1980 to 2016.
Regarding the number of cold days, about 26.5% of the stations show an increasing trend of (TXn). The occurrence of cold days (TX10p) and nights (TN10p) decreased by 1.84 (P < 0.05) and 0.79 (P < 0.05) days/decade, respectively (Table ). The present study agrees with the findings of (Rahimzadeh et al., Citation2009) where (TN10p) and (TX10p) was decreased in many parts of Iran. Erfanian et al. (Citation2014) also reported a general decreasing of (TX10p) and nights (TN10p) in northeastern Iran.
Although all seasons show a marked increasing trend, the variation in TXn is greater than TNn in all seasons of the country. This variation is observed especially in autumn and winter which are larger than summer and spring seasons.
3.3.3. Correlation coefficients of temperature indices
The warm and cold extremes temperature indices were highly correlated with the mean annual temperature, with correlation coefficients higher than 0.5 (P < 0.05). The warm temperature indices were positively correlated with the mean temperature and the correlation coefficients ranged from 0.74 to 0.95 (Table ). This result is consistent with (W. Sun et al., Citation2015).
Table 3. The correlation matrix of extreme temperature indices in Ethiopia
The results showed that 70.59% of the stations have greater trend magnitudes for summer days (SU). This implies that the summer temperatures increase faster than winter temperatures. The trend of TX90p (2.65 days/decade) is much higher than TN10p (1.84 days/decade). On the other hand, the trend of TN90p (2.04 days/decade) is much higher than TX10p (0.79 days/decade). Thus, there is an increased inter-diurnal variability during daytime. The variations in the extreme climate indices of the daily maximum temperature (TX10p and TX90p) are generally higher than changes in the extreme indices of daily minimum temperature (TN10p and TN90p).
Correlation coefficients were calculated to further clarify the relationships of extreme temperature indices (Table ). The hot indices versus hot indices (TXx, TXn, TX90, TN90 and SU) and cold indices versus cold indices (ID, TNn, TXn, TX10P, TN10P and FD) showed positive correlations. However, hot indices (TXx, TXn, TX90, TN90 and SU) versus cold indices (ID, TNn, TXn, TX10P, TN10P and FD) showed negative correlations. These indicate that hot indices experienced an upward trend than cold indices over the past 37 years.
3.3.4. Extreme temperature‐related indices
The results showed that the intensity and frequency of warm temperature extremes have increased, while cold temperature extremes decreased in most stations. The decadal changes of extreme temperature indices are shown in (Table ). The monthly maximum value of daily maximum temperature (TXx) trend increased at 73.5% stations while the trends in (TNx) has increased for 26.5% of the stations during 1980–2016. Most of the stations showing a significant positive trend in (TXx) are found in northern and southeastern parts of the Ethiopia. The monthly minimum value of daily maximum temperature (TXn) also showed positive trends, which are located in the same part of the country.
4. Discussion
Global warming is triggering particular climate extremes, including decreasing the frequency of cold days and cold nights and increasing the frequency of warm days and warm nights (IPCC, Citation2007). Ethiopia is characterized with inter-annual variability of rainfall and temperature. The rainfall variability affecting crop production over most parts of the country. All the weather stations exhibited a coefficient of variation (CV> 0.1) except Dembi dolo, Gode, Alem Ketema and Jinka stations. This variation may influence the regional climate systems and the hydrological cycle (Asfaw et al. (Citation2017). The increasing dry days and downward trends in precipitation in winter season support the occurrence of droughts, while opposite trends in summer contributes flood risks (Gedefaw et al., Citation2018; Tekleab et al., Citation2013). The annual maximum and minimum temperatures (TXam and TNam) and the diurnal temperature range (DTR) in the country were 2.68/decade, 2.04/decade and 0.62/decade, respectively, during the study period. There is also significant spatial variation in the mean range of temperature. This change may express the diurnal variation and demanding atmospheric moisture (Subash & Sikka, Citation2013). On the other hand, (Mohan et al., Citation2020) has been reported that the DTR showed significant decreasing trends since the nighttime temperatures have risen.
The monthly maximum value of daily maximum temperature (TXx) trend increased at about 73.5% of stations and 26.5% of the stations show an increasing trend for TNx. The findings of this study showed that the frequency and intensity of warm temperature extremes have increased, while cold temperature extremes decreased in most stations.
The average hot days (TX90p) and nights (TN90p) increased by 2.65 (P < 0.05) and 2.04 (P < 0.05) days/decade, respectively. However, cold days (TX10p) and nights (TN10p) decreased by 1.84 (P < 0.05) and 0.79 (P < 0.05) days/decade, respectively. Alexander et al. (Citation2006) found that cold nights decreased and warm nights increased at approximately 70% of locations worldwide. Similar study carried out by Rahimzadeh et al. (Citation2009) found an increase in TN90p and TX90p changes and a decrease in the TN10p and TX10p changes. Another study conducted by Erfanian et al. (Citation2014) in Iran also showed a decreasing trend in TX10p and TN10p and an increasing trend in TX90p and TN90p indices. The findings of this study are inconsistent with these findings. Overall, the increase in the TN90p and TX90p indices is most likely due to global warming (Kiktev et al., Citation2003).
The changes in water vapor divergence for the rainy season (June to September) exhibited a regional increasing drying trend in the country from 1980 to 2016, which was consistent with the variation in consecutive dry days (Gedefaw et al., Citation2018).
5. Conclusion
The present study was conducted to identify the changes and trends in climate extremes in Ethiopia using temperature-related extreme indices for the period 1980–2016. Thereby, the trends of climate change on climate extremes were analyzed using the trend detection methods using observed datasets. Assessment of temperature-related extreme indices showed that the trends of temperature have increased throughout the country during the study period. The maximum and minimum values of daily maximum and minimum temperatures demonstrated an increasing trend in most regions. Furthermore, the increasing trends in the number of warm days and nights were observed at most stations. The increasing trends of temperature at most stations are observed in northern and southeastern parts of the country. Hot temperature extremes are increasing at most stations as compared to cold temperature extremes.
Most of the hot extremes are found to increase in northern and southeastern stations (Zone I & II). On the other hand, cold extremes are found to decrease in the southwestern part (zone III). The hot temperature extremes (TXX, TNX) and the cold temperature extremes (TXN, and TNN) show variation with respect to spatial distributions. The extreme hot temperature indices (TXx, TXn, TX90, TN90 and SU) and cold temperature indices (ID, TNn, TXn, TX10P, TN10P and FD) during 1980–2016 exhibited contrasting variations across the stations. Overall, assessment of the impacts of extremes climate events on agriculture, water resources as well as on human well beings will give insights for policy- and decision makers to take proactive measures to the risks. Therefore, the results of this study could help to understand the current conditions of extreme climate variations over Ethiopia.
Authorship statement
I submitted a manuscript entitled: “Assessment of changes in climate extremes of temperature over Ethiopia” which will be published in Cogent Engineering journal. The author certifies that he has participated sufficiently in the work to take public responsibility for the content, including participation in the concept, design, analysis, writing or revision of the manuscript. The final manuscript before submission was checked and approved by the author.
Acknowledgements
The author thanks the editor and the four reviewers for their constructive comments and suggestions to improve the quality this article.
Disclosure statement
No potential conflict of interest was reported by the author(s).
Data Availability Statement
The Authors confirm that the data supporting the findings of this study are available within this article. The raw data that supports the findings of this study are available upon the request from the corresponding author.
Additional information
Funding
References
- Ahmedou, O. C. A., Yasuda, H., Wang, K., & Hattori, K. (2008). Characteristics of precipitation in Northern Mauritania and its links with sea surface temperature. 72, 2243–16. https://doi.org/10.1016/j.jaridenv.2008.07.011
- Alexander, L., Zhang, X., Peterson, T., Caesar, J., Gleason, B., Klein Tank, A., Haylock, M., Collins, D., Trewin, B., & Rahimzadeh, F. (2006). Global observed changes in daily climate extremes of temperature and precipitation. Journal Of Geophysical Research-Atmospheres (1984–2012), 111(D5). https://doi.org/10.1029/2005JD006290
- Aronica, G. T., & Bonaccorso, B. (2013). Climate change effects on hydropower potential in the Alcantara River Basin in Sicily (Italy). Earth Interactions, 17(19), 1–22. https://doi.org/10.1175/2012EI000508.1
- Asfaw, A., Simane, B., Hassen, A., & Bantider, A. (2017 Elsevier Ltd). Variability and time series trend analysis of rainfall and temperature in Northcentral Ethiopia: A case study in Woleka Sub-Basin’. Weather and Climate Extremes, June, 1–13. https://doi.org/10.1016/j.wace.2017.12.002
- Badjana, H. M., Renard, B., Helmschrot, J., Edjamé, K. S., Afouda, A., & Wala, K. (2017). Bayesian trend analysis in annual rainfall total, duration and maximum in the Kara River Basin (West Africa). Journal of Hydrology: Regional Studies, 13(August), 255–273. Elsevier. https://doi.org/10.1016/j.ejrh.2017.08.009
- Behailu, S., Melesse, A. M., Bhat, M. G., & Mcclain, M. E. (2014). Catena assessment of water resources availability and demand in the Mara River Basin. Catena, 115, 104–114 Elsevier B.V. https://doi.org/10.1016/j.catena.2013.11.017
- Bring, A., & Destouni, G. (2013). Hydro-climatic changes and their monitoring in the Arctic: Observation-model comparisons and prioritization options for monitoring development. Journal of Hydrology, 492, 273–280 Elsevier B.V. https://doi.org/10.1016/j.jhydrol.2013.04.003
- Erfanian, M., Ansari, H., Alizadeh, A., & Benayan Aval, M. (2014). Assessment of climatic extreme events variations in Khorasan Razavi province. Iran Journal of Irrigation and Drainage, 4, 817–825.
- Fathian, F., Ghadami, M., & Dehghan, Z. (2022). Observed and projected changes in temperature and precipitation extremes based on CORDEX data over Iran. Theoretical and Applied Climatology, 149(1–2), 569–592. https://doi.org/10.1007/s00704-022-04066-9
- Gedefaw, M., Yan, D., Wang, H., Qin, T., Girma, A., Abiyu, A., & Batsuren, D. (2018). Innovative trend analysis of annual and seasonal rainfall variability in Amhara Regional State, Ethiopia. Atmosphere, 9(9), 326. https://doi.org/10.3390/atmos9090326
- Girma, E., Tino, J., & Wayessa, G. (2016). Rainfall trend and variability analysis in Setema-Gatira Area of Jimma, Southwestern Ethiopia. African Journal of Agricultural Research, 11(32), 3037–3045. https://doi.org/10.5897/AJAR2015.10160
- Gocic, M., & Trajkovic, S. (2013). Analysis of changes in meteorological variables using Mann-Kendall and Sen ’ s slope estimator statistical tests in Serbia. Global and Planetary Change, 100, 172–182 Elsevier B.V. https://doi.org/10.1016/j.gloplacha.2012.10.014
- Gong, B. (2018). Variation of hydrothermal conditions under climate change in naqu prefecture, Tibet Plateau, China. International Journal of Environmental Research and Public Health, 15(10), 2271. https://doi.org/10.3390/ijerph15102271
- IPCC. (2007). Climate change- the scientific basis. Intergovernmental Panel on Climate Change. Cambridge University Press, Cambridge.
- IPCC. (2013). Climate change 2013: The physical science basis. In: Contribution of working group i to the fifth assessment report of the intergovernmental panel on climate change. Intergovern- mental Panel on Climate Change, Working Group I Contribution to the IPCC Fifth Assessment Report (AR5). Cambridge Univ Press, New York, 1535. https://doi.org/10.1029/2000JD000115.
- Jabal, Z. K., Khayyun, T. S., & Alwan, I. A. (2022, June). Impact of climate change on crops productivity using MODIS-NDVI time series. Civil Engineering Journal, 8(6), 1136–1156. https://doi.org/10.28991/CEJ-2022-08-06-04
- Kendall, M. G. (1975). Rank correlation Methods. Griffin, London, UK.
- Kiktev, D., Sexton, D. M. H., Alexander, L., & Folland, C. K. (2003). Comparison of modeled and observed trends in indices of daily climate extremes. Journal of Climate, 16(22), 3560–3571. https://doi.org/10.1175/1520-04422003016<3560:COMAOT>2.0.CO;2
- Klein Tank, A., Peterson, T., Quadir, D., Dorji, S., Zou, X., Tang, H., Santhosh, K., Joshi, U., Jaswal, A., & Kolli, R. (2006). Changes in daily temperature and precipitation extremes in central and south Asia. Journal Of Geophysical Research-Atmospheres (1984–2012), 111(D16). https://doi.org/10.1029/2005JD006316
- Liu, W., Zhang, M., Wang, S., Wang, B., Li, F., & Che, Y. (2013). Changes in precipitation extremes over Shaanxi Province, northwestern China, during 1960–2011. Quaternary International, 313-314, 118–129. https://doi.org/10.1016/j.quaint.2013.06.033
- Mann, H. B. (1945). Non-parametric tests against trend. Econometrica, 13(3), 245–259.
- Mbungu, W., Ntegeka, V., Kahimba, F. C., Taye, M., & Willems, P. (2012). temporal and spatial variations in hydro-climatic extremes in the Lake Victoria Basin. Physics and Chemistry of the Earth, 50-52, 24–33 Elsevier Ltd. https://doi.org/10.1016/j.pce.2012.09.002
- Mohan, S., Clarke, R. M., & Chadee, X. T. (2020). Variations in extreme temperature and precipitation for a Caribbean island: Barbados (1969–2017). Theoretical and Applied Climatology, 140(3–4), 1277–1290. https://doi.org/10.1007/s00704-020-03157-9
- Nama, A. H., Abbas, A. S., & Maatooq, J. S. (2022, June). Field and satellite images-based investigation of rivers Morphological Aspects. Civil Engineering Journal, 8(7), 1339–1357. https://doi.org/10.28991/CEJ-2022-08-07-03
- Pingale, S. M., Khare, D., Jat, M. K., & Adamowski, J. (2014). Spatial and temporal trends of mean and extreme rainfall and temperature for the 33 Urban Centers of the Arid and Semi-Arid State of Rajasthan, India. Atmospheric Research, 138, 73–90 Elsevier B.V. https://doi.org/10.1016/j.atmosres.2013.10.024
- Rahimzadeh, F., Asgari, A., & Fattahi, E. (2009). Variability of extreme temperature and precipitation in Iran during recent decades. International Journal of Climatology, 29(3), 329–343. https://doi.org/10.1002/joc.1739
- Randell, H., & Gray, C. (2016). Climate variability and educational attainment: Evidence from Rural Ethiopia. Global Environmental Change, 41, 111–123 Elsevier Ltd. https://doi.org/10.1016/j.gloenvcha.2016.09.006
- Ren, G., Guan, Z., Shao, X., & Gong, D. (2011). Change in climatic extremes over Mainland China. Climate Research, 50(1–2), 105–111. https://doi.org/10.3354/cr01067
- Saddique, N., Khaliq, A., & Bernhofer, C. (2020). Trends in temperature and precipitation extremes in historical (1961–1990) and projected (2061–2090) periods in a data scarce mountain basin, northern Pakistan. Stochastic Environmental Research and Risk Assessment, 34(10), 1441–1455. https://doi.org/10.1007/s00477-020-01829-6
- Sawan, Z. M. 2017. Climatic variables: Evaporation, sunshine, relative humidity, soil and air temperature and its adverse effects on cotton production. Information Processing in Agriculture, China Agricultural University. https://doi.org/10.1016/j.inpa.2017.09.006
- Seleshi, Y., & Zanke, U. (2004). Recent changes in rainfall and rainy days in Ethiopia. International Journal of Climatology, 24(8), 973–983. https://doi.org/10.1002/joc.1052
- Shrestha, A. B., Bajracharya, S. R., Sharma, A. R., Duo, C., & Kulkarni, A. (2016). Observed trends and changes in daily temperature and precipitation extremes over the Koshi river basin 1975–2010. International Journal of Climatology, 2016. https://doi.org/10.1002/joc.4761
- Sillmann, J., Kharin, V. V., Zhang, X., Zwiers, F. W., & Bronaugh, D. (2013). Climate extremes indices in the CMIP5 multimodel ensemble: Part 1. Model evaluation in the present climate. Journal of Geophysical Research: Atmospheres, 118(4), 1716–1733. https://doi.org/10.1002/jgrd.50203
- Soltani, M., Laux, P., Kunstmann, H., Stan, K., Sohrabi, M. M., Molanejad, M., Sabziparvar, A. A., Ranjbar Saadatabadi, A., Ranjbar, F., Rousta, I., Zawar-Reza, P., Khoshakhlagh, F., Soltanzadeh, I., Babu, C. A., Azizi, G. H., & Martin, M. V. (2016). Assessment of climate variations in temperature and precipitation extreme events over Iran. Theoretical and Applied Climatology, 126(3–4), 775–795. https://doi.org/10.1007/s00704-015-1609-5
- Subash, N., & Sikka, A. K. (2013). Trend analysis of rainfall and temperature and its relationship over India. no. 1974. https://doi.org/10.1007/s00704-013-1015-9
- Sun, Q., Kong, D., Miao, C., Duan, Q., Yang, T., & Aizhong, Y. (2014). Variations in global temperature and precipitation for the period of 1948 to 2010. Environmental Monitoring and Assessment, 186(9), 5663–5679. https://doi.org/10.1007/s10661-014-3811-9
- Sun, W., Mu, X., Song, X., Wu, D., Cheng, A., & Qiu, B. (2015). Changes in extreme temperature and precipitation events in the Loess Plateau (China) during 1960–2013 under global warming. https://doi.org/10.1016/j.atmosres.2015.09.001
- Suwarno, I., Ma’arif, A., Raharja, N. M., Nurjanah, A., Ikhsan, J., & Mutiarin, D. (2021). IoT-based lava flood early warning system with rainfall intensity monitoring and disaster communication technology. Emerging Science Journal, 4, 154–166 Special Issue “IoT, IoV, and Blockchain”. https://doi.org/10.28991/esj-2021-SP1-011
- Tekleab, S., Mohamed, Y., & Uhlenbrook, S. (2013). Hydro-climatic trends in the Abay/Upper Blue Nile Basin, Ethiopia. Physics and Chemistry of the Earth, 61–62, 32–42 Elsevier Ltd. https://doi.org/10.1016/j.pce.2013.04.017
- Theobald, A., Mcgowan, H., & Speirs, J. (2016). Trends in synoptic circulation and precipitation in the Snowy Mountains Region, Australia, in the period 1958 – 2012. Atmospheric Research, 169, 434–448 Elsevier B.V. https://doi.org/10.1016/j.atmosres.2015.05.007
- Vormoor, K., Lawrence, D., Schlichting, L., Wilson, D., & Kwok, W. (2016). Evidence for changes in the magnitude and frequency of observed rainfall vs. snowmelt driven floods in Norway. Journal Of Hydrology, 538, 33–48 Elsevier B.V. https://doi.org/10.1016/j.jhydrol.2016.03.066
- Wagesho, N., Goel, N. K., & Jain, M. K. (2012). Investigation of non-stationarity in hydro-climatic variables at Rift Valley Lakes Basin of Ethiopia. Journal of Hydrology, 444–445, 113–133 Elsevier B.V. https://doi.org/10.1016/j.jhydrol.2012.04.011
- Wang, H., Zhang, M., Zhu, H., Dang, X., Yang, Z., & Yin, L. (2012). Hydro-climatic trends in the last 50 years in the Lower Reach of the Shiyang River Basin, NW China. Catena, 95, 33–41 Elsevier B.V. https://doi.org/10.1016/j.catena.2012.03.003
- Wen, X., Xiaoqing, W., & Gao, M. (2017). Spatiotemporal variability of temperature and precipitation in Gansu Province (Northwest China) during 1951 – 2015. Atmospheric Research, 197(November 2016), 132–149. Elsevier. https://doi.org/10.1016/j.atmosres.2017.07.001
- Xu, X., Du, Y., Tang, J., & Wang, Y. (2011). Variations of temperature and precipitation extremes in recent two decades over China. Atmospheric Research, 101(1), 143–154. https://doi.org/10.1016/j.atmosres.2011.02.003
- Yenehun, A., Walraevens, K., & Batelaan, O. (2017). Spatial and temporal variability of groundwater recharge in Geba Basin, Northern Ethiopia. Journal of African Earth Sciences, 134, 198–212— Elsevier B.V. https://doi.org/10.1016/j.jafrearsci.2017.06.006
- You, Q., Kang, S., Aguilar, E., & Yan, Y. (2008). Changes in daily climate extremes in the eastern and central Tibetan Plateau during 1961– 2005. Journal of Geophysical Research, 113(D7). https://doi.org/10.1029/2007JD009389
- Zarrin, A., Dadashi-Roudbari, A., & Hassani, S. (2022). Future changes in precipitation extremes over Iran: Insight from a CMIP6 bias corrected multi-model ensemble. Pure Applied Geophysics, 179(1), 441–464. https://doi.org/10.1007/s00024-021-02904-x
- Zhang, Q., Jianfeng, L., Singh, V. P., & Xiao, M. (2013). Spatio-temporal relations between temperature and precipitation regimes: Implications for temperature-induced changes in the hydrological cycle. Global and Planetary Change, 111, 57–76 Elsevier B.V. https://doi.org/10.1016/j.gloplacha.2013.08.012
- Zhao, J., Huang, Q., Chang, J., Liu, D., Huang, S., & Shi, X. (2015). Analysis of temporal and spatial trends of hydro-climatic variables in the Wei River Basin. Environmental Research, 139, 55–64 Elsevier. https://doi.org/10.1016/j.envres.2014.12.028