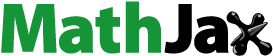
Abstract
Owing to their high strength, high specific modulus, low co-efficient of thermal expansion, and excellent wear resistance, aluminium metal matrix composites reinforced with hard ceramic particles are employed in different domains. The primary heat treatment method employed to enhance the desirable properties of heat-treatable aluminium alloys and their composites is T6 treatment. This study investigated the effects of metallic reinforcement and mechanically mixed layer on the tribological characteristics of Al-Zn-Mg alloy matrix composites. The microstructure, material transfer behavior, tribological characteristics, and wear mechanism of the worn surfaces were examined. A microstructural study revealed that the reinforcements would encourage grain refining, resulting in a 60.7% average size reduction. The wear rates have significantly reduced by 50.2% and 67.2%, respectively, with the introduction of 2.0 wt% of reinforcement and T6 treatment. There is a decreasing trend of wear rate as the weight percentage of reinforcement is increased, both in as-cast and peak-aged conditions. The refractory nature of precipitates formed during T6 treatment improves the hardness of the composite and thereby reduced the wear rate. Mechanically mixed layer (MML) is observed in the worn-out surface of peak-aged composites as revealed by the SEM analysis. Overall, the Al-Zn-Mg 6 wt. % grey cast iron composite showed excellent performance in tribological characteristics.
1. Introduction
Engineering materials such as composites, ceramics, and plastics have dominated the aerospace, transportation, automobile, and construction industries and evolved in the last three decades. Such composites have a high specific strength, which makes them most suitable for many conventional industrial applications. The key importance of composites as an engineering material has been demonstrated since out of thousand six hundred materials, more than 13% of composite materials are available in the market today (Zeng et al., Citation2018).
Applications demanding higher wear resistance and specific strength materials have been on the rise in recent decades (Ren et al., Citation2019). Al alloy matrix composites (AAMCs) with ceramic reinforcements have replaced conventional metals and alloys in weight sensitive automotive applications viz. Cylinder blocks, connecting rods, brake drums, cylinder liners, pistons (Manohar et al., Citation2022). With their counter bodies, these components are frequently subjected to sliding wear. A number of researchers have examined the sliding wear behaviour of AAMCs.
Enhancement in sliding distance increased the wear rate almost linearly for all composites. An increase in grain size also resulted in enhancing in wear rate. Delamination and adhesion wear are dominating according to worn out surface analysis of alloys using SEM, whereas abrasion is the principal wear mechanism in the composite. Improvement in wear characteristics is ascribed to enhancement in hardness and strength as the alloy was reinforced with Gr particles and increase in the time of milling (Prasad Reddy et al., Citation2019). Another researcher considered the role of MML formed on the tribological characteristics of solutionised and aged Al 7075 alloy and Al 7075/SiC composite. It is revealed that the existence of a thin, stable, and hard MML will enhance the wear resistance of alloy and composite even though the coefficient of friction is quite high (Hariharasakthisudhan et al., Citation2019).
Irrespective of speed and load conditions, AAMCs offer higher wear resistance in comparison with virgin alloys. This is mostly because the reinforcement dispersion plastically constrains the matrix alloy and increases the virgin alloy’s high temperature strength. Furthermore, the hard dispersoids that protrude from the composite’s surface prevent the matrix from coming into contact with the counter bodies. This results in significant reduction in wear rate and related properties (Prakash et al., Citation2022). Few researchers found that AAMCs exhibit a higher wear rate than the alloy. They attributed it to harder reinforcement particles penetrating deeper into the counter surface and particle fracture which then becomes entrapped in the contact surface. According to certain study, there is a critical applied load over which alloys and composites wear at comparable rates under similar tribological conditions. They attributed it to the fracture and removal of reinforcement particulate which results in delamination type wear (Lei et al., Citation2021; Moazami et al., Citation2022; Pan et al., Citation2020; Sardar et al., Citation2020). Another disadvantage of ceramic reinforced composites is that, the reinforcement phase has a tendency to behave as rigid abrasive particles, creating additional abrasive action on the counter surface. Additionally, dislodged reinforcement particles move along the contacting surfaces as wear debris, leading to greater wear on both the surfaces of counter bodies by creating three body type abrasion (Lua et al., Citation2018). The negative effects of ceramic reinforcements are overcome by several researchers with the use of hybrid reinforcement made of self-lubricating material such as graphite along with ceramic reinforcements (Jitendra et al., Citation2019; Killic et al., Citation2019; Ramkumar et al., Citation2019). This reduces shear stress, mitigates brittle failure in the subsurface region, hinders metal- to-metal contact, and behaves as a solid lubricant between two sliding surfaces, lowering friction and improving composite wear resistance.
The use of two reinforcements with different densities poses processing challenges for composites manufactured via liquid metallurgy techniques. This demands the need to investigate the effect of single reinforcement possessing both ceramic and self-lubricating properties.
We were unable to find any published research on the combined effects of precipitation hardening and metallic reinforcement on the Al 7075 matrix composites based on the literature review. The novelty of the work is the precipitation of Aluminium composites by the machined cast iron chips (scrap) and the possibility of solid solution strengthening by precipitation hardening treatment. The primary goal of this research project is to create composites using novel reinforcement materials that have good properties and could be a less expensive alternative to traditional ceramic reinforced metal matrix composites.
2. Materials and methods
2.1. Composition of matrix and reinforcement materials
Al 7075 rods in T6 condition were purchased from Perfect Metal Works in Bengaluru. The nominal composition of the matrix material is shown in Table . (Al 7075). In Servell Engineers, Manglore, GCI rods were cast using the compositions shown in Table .
Table 1. Comparison of actual and standard composition (wt.%) of Al 7075
Table 2. Comparison of actual and standard composition (wt.%) of GCI
2.2. Fabrication of composites (Al 7075-GCI/WCI MMCs) –Two step stir casting technique
In composite production, the stir casting technique was used. Pieces of sized Al 7075 rods are initially stored in a graphite crucible. It’s melted in a 5-kilowatt electric resistance furnace. Continued heating is carried out until a uniform temperature of 750°C is reached. By adding a small amount of scum powder to the molten slurry, slag or flux is removed. For degasification, dry hexa chloroethane (C2Cl6, 0.3 wt. %) is added to the slurry. To improve the wettability of the matrix and reinforcement phase, Mg pieces (1 wt. %) are added to the melt (Ramkumar et al., Citation2019).
GCI particles are preheated for 2 hours at 500°C. This method eliminates all volatile components while keeping the particle temperature close to melt. The melt is cooled to a semi-solid state in the air at 600°C. A mild steel stirrer is employed at 200–250 rpm for 15 minutes to create vortex in the melt (Jitendra et al., Citation2019). A vortex forms during stirring, and GCI powder (2, 4, and 6 wt. %) is introduced into the melt. Maintaining a stirrer speed of 200–250 rpm for 15 minutes results in the best dispersion of GCI.
A temperature probe measures the temperature of the furnace. After the reinforcement has been mixed in a semisolid state, the furnace is reheated to 750°C, which is above the liquidus temperature of Al 7075. Stirring is commenced again at 400 rpm. Once the stirring is complete, the slurry is poured into the preheated (500°C for 1 h) cast iron mold and air cooled.
The two-stage stir casting technique produces composites with low porosity, homogeneous reinforcement distribution, and minimal casting defects. Initially, the molten alloy comprising reinforcement particles is stirred in the semisolid state. The melt’s viscosity is increased in semi-solid form, which reduces the floating of GCI/WCI particles. The gas layers around the particle liquid interface are disrupted by the stirring motion at this stage, allowing for a greater spread of molten metal onto the particle surface. As a result, wettability has improved. By minimising the effect of sedimentation, the combined action of heating the composite slurry above its liquidus temperature and the agitations formed during stirring improves particle distribution.
The age hardening treatment consists of solutionizing and artificial aging. After two hours of solutionizing at 570°C in a muffle furnace, the material is quenched in a demineralized water bath that is kept at room temperature. On a solutionized specimen, artificial ageing (T6 temper) is applied in a hot air oven at temperatures of 100, 150, and 200°C for predetermined times until the material is over aged. Hardness variation with respect to aging time at constant temperature (aging curves) is plotted. Solutionizing at 570°C results in the dissolution of MgZn2 intermetallic phase in the matrix to achieve a single phase solid solution. Artificial aging at elevated temperatures results in precipitation of the intermetallic uniformly throughout the matrix phase, leading to strengthening of the matrix. These secondary precipitated phases enhance the matrix strength while retaining the precipitate’s and matrix’s crystal structure coherency
2.3. Measurement of wear parameters of Al 7075 alloy and composites
Pin on disc type wear tester is used in wear testing, and a data gathering system is established to assess how the Al 7075 alloy and composites respond to sliding wear in both untreated and heat-treated conditions. The wear tester used for the present work is suitable for investigating fundamental wear and friction characteristics.
The ASTM G-99 standard was used to conduct the wear test in a pin on disc apparatus. The disc on the machine and the test specimen were subjected to polishing. To achieve an even contact between the disc and specimen, it is polished using silicon carbide emery paper of 600 grit size and cleaned using an ethanol solution prior to actual run of the specimen. The applied load and speed of rotation of the disc are the major variables. Each trial is carried out with a track radius of 60 mm and for a duration of 60 minutes. Load is varied in 10, 20 and 30 N through a gravity loading system.
The speed of rotation of the disc is varied between 100, 200, and 300 rpm. Material loss was measured by taking the difference between the final and initial weight after every 15 minutes during the course of test. Before carrying out the test, the sample’s end surface is ground and polished to make the surface flat. The sample for the wear test has a 30 mm height and an 8 mm diameter. Wear rate is defined as the mass of material lost per unit sliding distance for a given force. The mass loss m, which is determined after every hour under a particular load, speed, and sliding distance, is used to compute the wear rate. The rate of wear is calculated using the formula
Weight loss, ,
Where, m1 = Initial weight (mg), m2 = Final weight (mg),
, where d= Diameter of track (mm), N= Disc rotation speed (rpm)
And t = Duration of the test (minutes).
3. Results and discussion
3.1. Characterization of tribological behaviour
Figures show the variation in mass loss with the incorporation of reinforcements at various applied loads and sliding speeds on Al 7075 alloy and its composites. It is inferred that, wear rate is significantly reduced with the reinforcements dispersed throughout the alloy matrix as the mass loss is continuously decreasing. The reduction of wear rate of Al 7075–6 wt.% GCI and Al 7075–6 wt.% WCI composites due to lower mass loss even at higher loads can be attributed to the fact that GCI being hard refractory can overcome the abrasion, thus causing lesser mass loss and seizure resistance of materials. The resistance offered to the delamination process of test specimen due to the reinforcement’s presence in Al7075 alloy contributes positively to the reduction in MRR. If the reinforcement particle possesses self-lubricating properties there will be a significant improvement in the dry sliding wear behaviour of AMCs (Deore et al., Citation2019).
Al 7075- GCI composites exhibit lesser mass loss even at peak loads as compared to Al 7075-WCI composites. This improvement can be attributed to the fact that GCI itself is a hard material and contains traces of beneficial free carbon in the form of graphite, which will serve as a self-lubricating agent to provide a cushioning effect between the test specimens and counter disc, thereby resulting in lesser wear.
As the applied normal load increases, the Al 7075 alloy exhibits an unceasing trend of mass loss (Figure ) and, consequently, wear rate due to direct metal-to-metal contact between the rubbing surfaces as a result of significant plastic deformation in the dry sliding condition. Unlike alloys, the depth of penetration by the harder asperities of the counter disc comprised of hardened steel is primarily controlled by the protruded tougher reinforcements of ceramic nature in the case of AMC’s (Figure ). Thus, most of the applied load is carried by the existence of reinforcements in the base matrix alloy. The primary function of reinforcement phase is to support the contact stresses thereby averting considerable plastic deformation and abrasion at the material and disc interface. This in turn reduces the amount of material removal rate (MRR).
Figure 4. Mass loss vs. applied load for as-cast Al 7075-GCI composites with varying wt. % of reinforcement.

The mass loss at lower loads is smaller compared to higher loads for alloy as well as composites. At smaller loads, the load on the specimen is the least which does not result in any strain hardening effect. This is the reason for lower mass loss, irrespective of sliding speeds and sliding distances. A notable increase in mass loss is observed as the applied load is increased. This phenomenon is common among all the sliding speeds (Figure ). The increase in temperature due to the higher coefficient of friction enhances adhesion of the material and, in turn, deformation at the surface. Large material removal because of subsurface cracking can be ascribed to the extent of plastic deformation (Xiao-Feng et al., Citation2013).
Reinforcing the alloy with particles of higher hardness enhances the effective area of contact between the asperities on the surface of counter disc and wear specimen of the composite, thus reducing the wear rate. At lesser load and speed, both alloy and composite exhibit a lesser wear rate. Irrespective of the aging condition, the wear rate of alloy and composite is lowest at 15 N load, since the load on the pin (wear specimen) is minimum. Even though sliding distance is increased and aging conditions changed, at lower loads the wear rate will still be low due to the lesser intensity of strain hardening effect. At a particular sliding distance and speed, wear rate is enhanced as the applied load increases, which reaches a maximum at a peak load of 45 N[18.
The increasing trend of wear rate at higher loads can be attributed to the fact that there is a higher frictional force, which further leads to de-bonding of surface layer and fracture. At an early stage of experiment, the pin surface in contact with the disc is broken thus resulting in smoothening of the pin surface there by resulting in enhanced strength due to strain hardening effect. Due to the tillage effect between mating surfaces, the surface temperature increases resulting from enhanced coefficient of friction. A rise in temperature leads to increase in adhesion, thereby enhancing the deformation intensity at the surface layers and further increasing material loss (Hindi et al., Citation2022).
Precipitation hardening treatment reduces the wear rate of Al 7075 alloy and its composites. The peak aged specimen at a lower temperature (100°C) had greater wear resistance in all conditions. The wear rate of the alloy or composites is also observed to increase as the aging temperature rises. When compared to specimens aged at a higher temperature, those aged at a lower temperature (100 °C) are more sensitive to reducing wear rate. Because finer intermetallics are precipitated as a result of more intermediate stages with smaller average interparticle distances, lowering the ageing temperature improves the coherency strain in the matrix. Higher aging temperatures(200°C) result in lower hardness because there are fewer intermediate zones formed during the development of coherent precipitates, resulting in less matrix strain (M et al., Citation2021). Figure illustrates mass loss graphs that clearly demonstrate this phenomenon under different load and speed conditions. The precipitates generated during the ageing process are thought to promote interfacial bonding between reinforcements and the matrix, act as a refractory material, and so increase hardness, which reduces wear rate. The refractory nature of precipitates formed during age hardening improves hardness of the composite and thereby reduces the wear rate. The precipitation phenomenon improves the interfacial bonding existing between the matrix & reinforcement phase, thus reducing the specific wear rate (Gopalakrishnan & Murugan, Citation2012). Wear rate is established for Al 7075 alloy, Al 7075-GCI composites under untreated and heat treated conditions. Under all testing conditions (applied load, sliding distance and sliding speed), it is observed that the wear rate of an unreinforced alloy is maximum.
3.2. Microstructural investigation of worn out surface using SEM
Figures show the surface morphology of Al 7075 alloy and composite worn out surfaces at both as-cast and 100°C peak aged condition at an applied load of 45 N and a sliding speed of 450 rpm. Applied load and sliding speed are the major contributors in deciding the nature of worn out surface (Gowri Shankar et al., Citation2016). Al 7075 alloy is subjected to severe erosion wear (Figure ) due to direct metal to metal contact between the matrix and wear tester disc. High load and sliding speed result in high stress and frictional force, leading to softening of the material and higher material loss. Al 7075 alloy WS shows extensive plastic grooving and ploughing. Under the as-cast condition, (Figure ) heavy surface damage from the matrix material smearing is observed at many points. The grooves on the peak aged samples (Figure b-c) are finer and minimum plastic deformation is observed at the edge of the grooves. Al 7075 alloy’s wear rate is reduced due to the incorporation of reinforcing particles at all loading conditions. Worn surface of GCI reinforced composites(Figures ) in as-cast condition is relatively smooth compared to the alloy. In peak aged samples worn out surface, presence of fine grooves is an indication of lesser damage to the surface. Furthermore, grooves are aligned in the direction of sliding, having minimum number of cracks at the surface. During sliding some particles are cut off and in some cases, smaller particles have emerged from the composite matrix. The accumulation of hard particles in damaged groove regions is an indication of existence of scars. Wear mechanisms vary for alloy and composites with various percentages of reinforcement as observed in SEM images of worn out surfaces (Kanayo & Kazeem, Citation2015). Delamination and adhesion are the major contributors to wear in case of base alloy. In few locations, minor ploughing can also be seen. Higher mass loss can be attributed to the extensive plastic deformation in the sliding direction. For 2 and 4 wt.% of reinforcement, the density of adhesion and delamination regions are lower compared to the base alloy. Delamination phenomena is almost absent for 6 wt.% of reinforcement. The dominant wear mechanism is abrasive in nature in the form of ploughing. Plastic deformation has significantly reduced due to the presence of reinforcement phase, which is ceramic in nature (Ul Haq & Anand, Citation2018).
Figure 6. SEM micrographs of Al 7075 alloy worn surface a) as-cast, b) Aged at100°C andc)Aged at 200°C.

3.3. Analysis of mechanically mixed layer (MML)
A mechanically mixed layer (MML) is observed in the worn out surface of both composites (Figures ). MML is formed mainly due to the transfer of material from the counterface to the worn surface. EDAX analysis of composites reveals a higher concentration of iron (Fe) which strengthens the argument of formation of MML. An increase in the concentration of Fe can only be attributed to the transfer of material from counterface to worn surface due to abrasion of the former. The reinforcement of hard ceramic particulates increases the capacity of the alloy to withstand applied load. Hence the wear specimen when in contact with the counterface results in heavy scratches on it. The disc material further undergoes a churning process and hence removes Fe from the counterface. This results in the formation of MML on the specimen surface. The transfer of material from the counterface to specimen surface and further formation of MML convert two body abrasion into a three body wear pattern. The MML acts as a solid lubricant thereby reducing the wear rate of composite (Miranda et al., Citation2015). EDAX analysis of worn out surface of alloy does not show any concentration of Fe (Figure ), thereby confirming the absence of material transfer from the counter face and formation of MML. The analysis of worn out surface of peak aged sample at 100°C reveals minimal surface damage. The grooves are finer and aligned in the direction of sliding with no surface cracks when the samples are peak aged at 100°C. The presence of MML in the composite also results in lesser surface damage, resulting in smoother worn out surface compared to the alloy.
Figure 9. Worn surface SEM image and EDAX of Al 7075–6 wt. % GCI composite with quantitative analysis of elements present.

4. Conclusions
It is inferred from the study of wear behaviour that, sliding wear rate is significantly reduced with the dispersion of reinforcements in the alloy matrix. There is a decreasing trend of sliding wear rate as the weight percentage of reinforcement is increased, both in as-cast and peak aged conditions. A notable increase in wear rate is observed as the applied load increases in all materials (alloy & composites) considered. The reduced sliding wear rate of Al 7075–6 wt.% GCI composites even at higher loads can be credited to the fact that GCI being hard refractories can overcome the abrasion, thus causing lower mass loss & wear rate. In all the cases, the peak aged specimen at lower temperature (100°C) shows significant reduction in wear rate. As the aging temperature increases, the wear rate of the alloy and composites increases. When compared to specimens aged at a higher temperature, those aged at a lower temperature (100 °C) are more sensitive to reducing the wear rate. The refractory nature of precipitates formed during age hardening improves hardness of the composite and thereby WR. 15–55% reduction in mass loss forAl 7075-GCI composite when aged at 200°C and 30–90% reduction in mass loss when aged at 100°C. 10–40% reduction in mass loss forAl 7075- WCI composite when aged at 200°C and 20–80% reduction in mass loss when aged at 100°C. Al 7075 alloy is subjected to severe erosion wear as compared to composites due to direct metal to metal contact between the matrix and wear tester disc. The grooves on the peak aged samples of Al 7075 alloy are finer and minimum plastic deformation is observed at the edge of the grooves. In peak aged samples WS of Al 7075 GCI/WCI composites, presence of fine grooves is an indication of lesser damage in the surface. Furthermore, grooves are aligned in the direction of sliding having minimum number of cracks at the surface. A mechanically mixed layer (MML) is observed in the worn out surface of both Al 7075 GCI/WCI composites. MML is formed mainly due to the transfer of material from the counter face to wear surface. EDAX analysis of the worn out surface of both composites reveals higher concentration of iron which supports the formation of MML. EDAX analysis of worn out suface of alloy does not show any concentration of iron, thereby confirming the absence of material tranfer from the counterface and formation of MML.
Correction
This article has been corrected with minor changes. These changes do not impact the academic content of the article.
Disclosure statement
No potential conflict of interest was reported by the authors.
References
- Deore, H. A., Mishra, J., Rao, A. G., Mehtani, H., & Hiwarkar, V. D. (2019). Effect of filler material and post process ageing treatment on microstructure, mechanical properties and wear behaviour of friction stir processed AA 7075 surface composites. Surface & Coatings Technology, 374, 52–13. https://doi.org/10.1016/j.surfcoat.2019.05.048
- Gopalakrishnan, S., & Murugan, N. (2012). Production and wear characterisation of AA 6061 matrix titanium carbide particulate reinforced composite by enhanced stir casting method. Composites Part B: Engineering, 43(2), 302–308. https://doi.org/10.1016/j.compositesb.2011.08.049
- Gowri Shankar, M. C., Sharma, S. S., Kini, A., Jayashree, P. K., & Gurumurthy, B. M. (2016). Study of wear behaviour and mechanical mixed layer on artificial aged Al6061 composite reinforced with B4C particles. Indian Journal of Science and Technology, 12(12), 1–9. https://doi.org/10.17485/ijst/2016/v9i12/88073
- Hariharasakthisudhan, P., Jose, S., & Manisekar, K., Dry sliding wear behaviour of single and dual ceramic reinforcements premixed with Al powder in AA6061 matrix, Journal of Materials Research and Technology, Volume 8, Issue 1, 2019, 275–283, https://doi.org/10.1016/j.jmrt.2018.01.005.
- Hindi, J., Hegde, A., Bm, G., Sharma, S., Kini, A., Murthy, A. A., & K,M. (2022). ‘TEM analysis and related mechanical characterization of age hardened aluminium 7075-grey cast iron particle reinforced composites. Cogent Engineering, 9(1), 2114208.
- Jitendra, M., Gohil, Mistrya, P. P., & Gohilc, P. (2019). Experimental investigations on wear and friction behaviour of Si3N4 reinforced heat-treated aluminium matrix composites produced using electromagnetic stir casting process. Composites Part B, 161, 190–204. https://doi.org/10.1016/j.compositesb.2018.10.074
- Kanayo, K., & Kazeem, A. (2015). Microstructural characteristics, mechanical and wear behaviour of aluminium matrix hybrid composites reinforced with alumina, rice husk ash and graphite. Engineering Science and Technology, 18(3), 416–422. https://doi.org/10.1016/j.jestch.2015.02.003
- Killic, S., Kacar, I., Sahin, M., Ozturk, F., & Erdem, O., “Effects of aging temperature, time, and pre-strain on mechanical properties of AA7075”, Materials Research, 22, 2019, 1–13, https://doi.org/10.1590/1980-5373-mr-2019-0006
- Lei, Y., Yan, H., & Wei, Z. (2021). Effect of hot extrusion on microstructure and tribological behavior of Al2O3p reinforced 7075 aluminum-matrix composites. Journal of Central South University, 28, 2269–2284. https://doi.org/10.1007/s11771-021-4768-9
- Lua, T., Chena, W., Xua, W., Wang, P., Maoa, M., Liu, Y., & Fu, Z. (2018). Yixiong Liuc and Zhiqiang Fua “The effects of Cr particles addition on the aging behaviour and mechanical properties of SiCp/7075Al composites. Materials Characterization, 136, 264–271. https://doi.org/10.1016/j.matchar.2017.12.030
- Manohar, G., Maity, S. R., & Pandey, K. M. (2022). Microstructural and mechanical properties of microwave sintered AA7075/Graphite/SiC hybrid composite fabricated by powder metallurgy techniques. Silicon, 14(10), 5179–5189. https://doi.org/10.1007/s12633-021-01299-7
- M, B., Hindi, G., Kini, J., U, A., Sharma, S. S., & Stojanovic, B. (2021). Investigation and analysis of aging behavior and tensile fracture study on precipitation hardened al7075-white cast iron particulate reinforced composites. Cogent Engineering, 8(1), 1920093. https://doi.org/10.1080/23311916.2021.1920093
- Miranda, G., Buciumeanu, M., Madeira, S., Carvalho, O., Soares, D., & Silva, F. S. (2015). Hybrid composites-metallic and ceramic reinforcements influence on mechanical and wear behaviour. Composites Part B, 74, 153–165. https://doi.org/10.1016/j.compositesb.2015.01.007
- Moazami, M. R., Razaghian, A., Mirzadeh, H., Emamy, M., & Moharami, A., Tribological behavior of as-cast and wrought Al –Mg2Si hybrid composites reinforced by Ti-based intermetallics, Journal of Materials Research and Technology, 20, 2022, 1315–1327, https://doi.org/10.1016/j.jmrt.2022.07.142.
- Pan, S., Saso, T., Yu, N., Sokoluk, M., Yao, G., Umehara, N., & Li, X., New study on tribological performance of AA7075- TiB2 nanocomposites, Tribology International, 152, 2020, 106565.
- Prakash, J., Gopalakannan, S., & Chakravarthy, V. K. Mechanical characterization studies of aluminium alloy 7075 based nanocomposites. Silicon 14, 1683–1694. (2022). https://doi.org/10.1007/s12633-021-00979-8
- Prasad Reddy, A., Vamsi Krishna, P., & Rao, R. N. (2019). Tribological Behaviour of Al6061–2SiC-xGr hybrid metal matrix nanocomposites fabricated through ultrasonically assisted stir casting technique. Silicon, 11(6), 2853–2871. https://doi.org/10.1007/s12633-019-0072-9
- Ramkumar, S., Fahad, A., Al-Mufadi, S., Siddharth, Raghu, R., & Siddharth, S. (2019). Investigations on microstructure, mechanical, and tribological behaviour of AA 7075 –x wt.% TiC composites for aerospace applications. Archives of Civil and Mechanical Engineering, 19(2), 428–438. https://doi.org/10.1016/j.acme.2018.12.003
- Ren, J., Wang, R., Feng, Y., Peng, C., & Cai, Z. (2019). Microstructure evolution and mechanical properties of an ultrahigh strength Al-Zn-Mg-Cu-Zr-Sc (7055) alloy processed by modified powder hot extrusion with post aging. Vacuum, 161, 434–442. https://doi.org/10.1016/j.vacuum.2019.01.013
- Sardar, S., Karmakar, S. K., & Das, D. (2020). Identification and modelling of applicable wear conditions for stir cast Al-composite. Friction, 8(5), 847–873. https://doi.org/10.1007/s40544-019-0302-6
- Ul Haq, M. I., & Anand, A. (2018). Mir Irfan Ul Haq and Ankush Anand “dry sliding friction and wear behaviour of AA7075-Si3N4 composite. Silicon, 10(5), 1819–1829. https://doi.org/10.1007/s12633-017-9675-1
- Xiao-Feng, W. U., ZHANG, G.A., & Fu-Fa, W. U. (2013). Influence of Bi addition on microstructure and dry sliding wear behaviors of cast Al-Mg2Si metal matrix composite. Transactions of Nonferrous Metals Society of China, 23(6), 1532–1542. Pages 1532-1542, ISSN 1003-6326. https://doi.org/10.1016/S1003-6326(13)62627-8
- Zeng, X., Yu, J., Fu, D., Zhang, H., & Teng, J. (2018). Wear characteristics of hybrid aluminum-matrix composites reinforced with well-dispersed reduced graphene oxide nanosheets and silicon carbide particulates. Vacuum, 155, 364–375. https://doi.org/10.1016/j.vacuum.2018.06.033