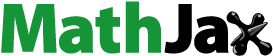
Abstract
The hip joint is one of the most essential joints for transmitting weights to the lower abdomen during day-to-day activities. Loosening of hip implants is mostly caused by wear. The wear assessment during the design stage offers a clear sense of the implant’s life expectancy, and modest adjustments in the design may also greatly enhance the implant’s life expectancy, lowering the probability of revision surgery. Linear wear is estimated at the contact surfaces of the femoral head to the acetabular cup and the acetabular cup to the backing cup in this study. In this work, oval-shaped hip implant is considered with a femoral head diameter of 28 mm, an acetabular cup thickness of 4 mm, and a backing cup thickness of 2 mm. The Archard’s law used to estimate the linear wear rate. It is observed that when the acetabular cup is made of UHWMPE and the stem, femoral head, and backing cup are made of CoCr, the least overall deformation is 0.394 mm during walking loads. When the stem is considered Ti−6Al−4 V and the acetabular cup of UHWMPE, the minimum wear between the femoral head and acetabular cup is 0.063 mm/year. During a typical standing posture, an acetabular cup-to-backing cup wear rate of 0.007 mm/year is estimated. Overall, the CoCr material combination had the lowest wear rate in the four activities considered for this work. These implants designs can be 3D-printed and further can be tested in a hip simulator under the same loading conditions.
1. Introduction
The hip joint is among the most essential load-bearing joint surfaces, enabling both strength and multiaxial movement while transferring multiple times body weight (BW) during daily activities like walking, standing, bending and sprinting (Hua et al., Citation2022). The human body is composed of roughly 270 bones at the moment of birth, and this number will be lowered to 206 bones by the time of maturity when some of the bones are merged together (Clarke, Citation2008). When it comes to the significance of the hip, it may be characterised by the fact that it allows human mobility and sustains the full body weight without causing distress (Hampton et al., Citation1980). The inability of the artificial joint to function properly is among the most prevalent reasons for complete joint revision. Any severe relative movement involving joint partners is classified as joint instability, which is often characterised by harm to implant components or adjacent soft tissue (Herrmann et al., Citation2012). However, because of the joint’s load-carrying capabilities in six degrees of freedom (flexion, extension, abduction, adduction, internal rotation, and external rotation), it is susceptible to degeneration and local loading, resulting in discomfort (K. N. Chethan et al., Citation2014). Artificial joints are frequently used to replace degraded hip joints, also known as total hip arthroplasty to avoid such outcomes. Total hip joint replacement implant consists of stem, femoral head, acetabular cup and backing cup. Figure shows the components associated in THR. Total hip replacements are often patient-specific and reliant on the requirements of the individual patient in terms of shape and sizes. The range of motion and physical function are critical considerations, particularly in younger patients who have high expectations for their life quality after THA surgery (Morlock et al., Citation2011). When a wide-ranging series of patients from various experimental studies were analysed, the findings revealed that younger individuals are more prevalent among older patients undergoing THR to restore function to a clinically relevant level (Foucher, Citation2016; Lunn et al., Citation2019). Furthermore, a substantial reduction in the average age of patients who need hip implants has prompted studies to prolong the durability of THRs in attempt to improve quality of life for active younger patients (K. N. Chethan, Ogulcan, et al., Citation2020).
Figure 1. Components used in hip implants (Bhawe et al., Citation2022).

Implants were traditionally held in place by pressure, friction or screws. Due to the insufficiency of these methods, new and improved methods have been developed. Now, there are two common types of femoral stem fixation: cemented femoral fixation and cementless femoral fixation (Babaniamansour, Citation2017) Designing an Optimized Novel Femoral Stem. A femoral head is attached by press fitting, then an acetabular cup and backing cup are attached (Bhawe et al., Citation2022). Although a press-fit fixation approach eliminates the need for screws or cups with screw holes, it can result in acetabular fractures or incompetence. However, for large-diameter metal-on-metal arthroplasty procedures, using an implant with no screw holes provides a wide surface area for liner support and prevents debris from entering through screw holes (K. N. Chethan et al., Citation2021; Pickering et al., Citation2009). The acetabulum and the lateral condyle of the knee joint are connected by the femoral head (K. N. Chethan, Zuber, et al., Citation2019). The femur bone is composed of two layers: cortical bone, which is tougher and more rigid on the outside, and cancellous bone, which is soft and spongy on the inside. An implant’s femoral component experiences bending and torsional loads due to the increasing strain on the femur caused by an individual’s increased weight and physical activity. If these loads are applied repeatedly and in a variety of ways, fatigue fractures or deformations in hip joint implant can occur (Delikanli & Kayacan, Citation2019). The finite element approach is commonly applied to analyse the numerous biomedical implants (K. N. Chethan et al., Citation2021).
One of the most essential requirements for materials used in THR is they should be biocompatible and have a low wear rate in order to minimise the number of revisions required owing to aseptic loosening of implants (K. N. Chethan, Zuber, et al.,). Nowadays, implants are made up of Ti−6Al−4 V, Co-Cr and UHWMPE (K. N. Chethan et al., Citation2021). Because of differences in individual anatomy, femoral heads can range in size from 22 millimetres to 54 millimetre (K. N. Chethan, Shyamasunder Bhat, et al., ; K. N. Chethan, Zuber, et al., Citation2020). Femoral stems appear in a range of geometrical shapes, including straight, tapered, short length and anatomical. The different stem designs used are circular, ellipse, oval and trapezoidal (K. N. Chethan, Zuber, et al., Citation2020).
Metal-on-polyethylene (MoP), metal-on-metal (MoM), ceramic-on-ceramic (CoC), and ceramic-on-polyethylene (CoP) bearings are the four basic types of bearings evaluated as well as utilised in THA. Metal-on-polyethylene (MoP) and ceramic-on-polyethylene (CoP) bearings are examples of hard-on-soft bearings, whereas hard-on-hard bearings include metal-on-metal (MoM), ceramic-on-ceramic (CoC), and ceramic-on-metal (CoM) (K. N. Chethan, Shyamasunder Bhat, et al.,). Ceramic heads with metallic inserts (CoM) have lately been launched as a mixed composition (Merola & Affatato, Citation2019). MOMs and COCs have garnered increased attention due to their ability to minimise wear rates of arthroplasties, especially in younger patients with an active lifestyle (Agarwal et al., Citation2005).
Delikanli et al (Delikanli & Kayacan, Citation2019)., suggested revascularisation of medullary tissue by lightening the implant. The lightened and roughened implants have asserted to be successful in integrating with the bone in the implantation location, according to animal research using a titanium mesh acetabular cup that had been implanted in a human body for 27 years, Koch et al., investigated the histological discoveries of the bone—implant junction in the cup. The findings revealed that there was adaptive bone remodelling at the interface between the inner solid core of the acetabular cup and the surrounding bone (Koch et al., Citation2018).
In the present study, oval stem profile with femoral head size 28 mm, acetabular cup of 4 mm and backing cup of 2 mm is considered. Different widely used materials are used for the analysis. Deformation, stresses and wear rates at the junction of acetabular cup of femoral head to acetabular cup and acetabular cup to backing cups are calculated for various activities like walking, running, knee bend etc. ANSYS R−19 is used to do finite element analysis on the models. ASTM F2996–13 standards have been used to specify boundary conditions (ASTM, Citation2015). The materials used in the study are Ti−6Al−4 V, CoCr alloy and UHWMPE. These materials are evaluated with different combinations for stem, femoral head, acetabular cup and backing cup to find the best suited materials with least wear rate per million cycles which can be used for hip stems. This work will significantly help to evaluate the life of the current design used for the THR.
2. Materials and methods
Several distinct designs are currently available for usage in THA (Colic & Sedmak, Citation2016; Jun & Choi, Citation2010; Kim & Yoo, Citation2016). They all have their own set of benefits and drawbacks. A stem with an oval cross-section is considered for the analysis in the current work (K. N. Chethan, Zuber, et al., ; K. N. Chethan et al., Citation2021). The femoral head size of 28 mm, acetabular cup of 4 mm and backing cup of 2 mm is considered. Modelling is carried out using CATIA V−6. CoCr alloy, Ti−6Al−4 V, and UHWMPE are the materials that were employed in the current work due to their mechanical properties. The length of stem is considered as 180 mm. Figure shows the cross-sectional frontal view of the assembled hip implant.
Titanium and its alloys are prominent metallic implant biomaterials that are utilised in total hip arthroplasty. In the medical industry, titanium alloys, such as Ti−6Al−4 V, are the most frequently used materials for stems and acetabular cementless hip replacements (Hu & Yoon, Citation2018). Titanium and its alloys have high corrosion resistance, great mechanical strength and a relatively low density (Hu & Yoon, Citation2018). This metal is widely used in many applications in the medical sector because of its outstanding biocompatibility (Shrestha, Citation2017). Because of its strength properties, durability, and chemical inertness, UHMWPE has been adopted for acetabular bearings for nearly 50 years. Recent advances have resulted in a material that is extremely wear-resistant and may last more than 15 years (Wang, Citation2013)(Encyclopedia of Tribology). Alloys of Co-Cr have excellent strength, corrosion, and wear properties, making them a popular implant material choice. Since its Young’s modulus is greater than titanium alloys’ and its wear resistance is greater, it is mainly used as a cement type femoral stem material (Hu & Yoon, Citation2018). The mechanical materials properties are shown in Table .
Table 1. Mechanical properties of the materials used for hip implant (Chen et al., Citation2018; K. N. Chethan, Shyamasunder Bhat, et al.,)
In this work, femoral head and backing cup material is kept constant as CoCr while femoral head material is varied as Ti alloy and CoCr alloy in combination with acetabular cup material as UHWMPE and CoCr. The general body weight of a person undergoing total hip replacement considered for this analysis is 767N which is approximately 78.26 kg.
In addition to the force acting on the implant, this study also considers the effects of the moment induced on the implant to get accurate results. The forces and moments acting on the backing cup are acquired by analysing prior data for activities that a person undergoing THR would be engaged in on a daily basis. Activities such as walking, knee bending, sitting and standing are considered when calculating the wear on the hip implant.
2.1. Meshing and boundary conditions
For the current work, unstructured mesh is selected for the meshing (Gutmann et al., Citation2023). A mesh grid independence research is performed in order to determine the final mesh size for all of the analysis. A mesh convergence study was conducted to determine the ideal mesh size for the study (K. N. Chethan, Ogulcan, et al., Citation2020). Mesh size was varied from 5 mm with an interval of 1 mm each. There was no substantial difference in the von Mises stresses when the mesh size was less than 1 mm. So 1 mm mesh size is finalised for the complete analysis. The mesh grid independence study is shown in Figure .
The bottom of the stem is used as a fixed support for the analysis. Boundary conditions are adopted in accordance with ASTM F2996–13 (ASTM, Citation2015). An analysis of static structural behavior is performed on the models in the ANSYS R−19 to determine the von Mises stress, von Mises strain and total deformation (K. N. Chethan, Zuber, et al.,). In this analysis, sliding distance and pressure between two contact surfaces are determined with a contact tool, and a friction coefficient between them is considered.
2.2. Contact interaction behavior
Contact behavior between all the parts is defined as “frictional”. The frictional coefficient for CoCr and CoCr is taken as 0.19, for Ti and CoCr, it is 0.17 and between CoCr and UHWMPE, it is 0.16.
2.2.1. Wear estimation
The wear rates were estimated using Archard’s law of wear given by
In equation 1, V represents the volume of wear debris, Kw represents the wear coefficient, S represents the sliding distance, and Pn is the normal contact pressure. This equation can also be expressed as (K. N. Chethan, Ogulcan, et al., Citation2020; Wu et al., Citation2003):
Which can also by written as
where dh (mm/cycle) represents wear depth, σ (MPa) represents maximum normal contact pressure, and ds (m) represents sliding distance (K. N. Chethan, Ogulcan, et al., Citation2020). In estimating the life span of the hip implants, it is considered as one million cycles per year. So, the wear rate calculated for one cycle is converted into million cycles to find the wear rate per year. Wear rate is estimated between femoral head to acetabular cup and acetabular cup to backing cup. These two junctions in the hip implant experiences higher wear rate which leads to aseptic loosening resulting in hip replacement surgery.
3. Results
A preliminary research is carried out on the model to determine the optimal material combination for the stem, femoral head, acetabular cup and backing cup. It can be observed from Table that the least total deformation 0.394 mm is exhibited in the walking loading condition with the material combination of acetabular cup as UHWMPE and stem, femoral head and backing cup as CoCr. The corresponding von Mises stress and strain for this material combination is 407.99MPa and 0.002 mm/mm, respectively.
Table 2. Static study of a hip implant performed in accordance with ASTM F2996–13 standards with different materials combination
Among four activities that were studied under varied loading conditions (walking, knee bending, sit down, and stand up), the one with the least total deformation, von Mises stress and elastic strain was shown in Table .
The greatest deformation recorded under walking loading conditions is 0.688 mm when the stem is made of Ti−6Al−4 V, the acetabular cup is made of UHWMPE, and the femoral head and backing cup are made of CoCr.
As a general trend, it can be concluded that the total deformation is minimal when the stem, femoral head and backing cup are composed of CoCr and the acetabular cup is made of CoCr or UHWMPE.
Walking shows least deformation, stresses and strain compared to the other three activities. The patient’s body weight (BW) varies from 280% to 870% with different activities. Wwalking has the least load acting on the hip implants. Figure shows the deformation, stresses and strain acting on the hip implant during waking condition for an individual of 767N weight.
3.1. Wear studies using Archard’s wear law
The second part of the study focuses on estimating wear between the femoral head to acetabular cup and acetabular cup to backing cup. CoCr femoral heads with polyethylene cups were found to exhibit wear behaviors in prior research, and it was reported that the wear intensity distributed more evenly along the contact of acetabular cups, and that may have the surface to preserve conforming contact geometry (HUNG & JS-S, Citation2002). Figure shows the wear pattern between femoral head to acetabular cup during different activities.
Minimum wear between the femoral head and acetabular cup found to be 0.063 mm/year when the stem is made of Ti−6Al−4 V, the acetabular cup is made of UHWMPE during walking conditions. Maximum wear was found to be 0.84 mm/year during knee bend loading condition. These results are relatively closer with the available literatures. Also, these results were more realistic as moment is considered in this study along with the loading conditions. This actually mimics the normal load distribution in THR patients. Table shows the detailed wear rate per year with different activities and different materials combinations considered for the current study.
Table 3. Sliding distance, contact pressure and wear rate per year with different activities
In the second part, it also tried to estimate the wear between the acetabular cup and backing cup. This interface has a significant effect in the loosening of hip implants which is one of the main causes for the revision surgery. The active younger patients have more wear due to their intense movement. In this work, four different activities are considered along with moment and the linear wear rate is estimated between these two contact surfaces. In general, for all four activities considered for the study, CoCr material combination shown least wear rate over the other combinations of materials made up of. A least wear of 0.007 mm/year is estimated during normal stand-up position. Whereas the maximum linear wear rate found 0.848 mm/year when acetabular cup is made of UHWMPE for the complete CoCr implant. Figure shows the different a pattern for the activities considered for the study.
In knee bend activities, a maximum linear wear rate found to be 8.48 mm/year, this is, however, matching with previously available literature. Table shows the linear wear rate per year for all the four activities along with the different widely used materials.
Table 4. Sliding distance, contact pressure and wear rate per year with different activities
4. Discussion
In current study, linear wear rate is estimated between the femoral head and acetabular cup, and between the acetabular cup and backing cup, using various material combinations which are widely available nowadays. This was attained by considering the variations in force and moment acting on the backing cup when a typical patient undergoing total hip replacement performs movements such as walking, bending the knee, standing up and sitting down. When all four components are considered of CoCr, the overall deformation and linear wear rate per year found to be least with comparison of other material combinations. When the stem is made of Ti−6Al−4 V, the acetabular cup is made of ultra-high-molecular-weight polyethylene, and the femoral head and backing cup are made of CoCr alloy, the wear rate per year is the least between the femoral head and acetabular cup, whereas it is the least between the acetabular cup and backing cup when all four components are made of CoCr.
According to Jiang et al (Jiang, Citation2007), four distinct structural models were studied, which were constructed of ultra-high molecular weight polyethylene (UHMWPE), CoCr alloy, 316 L stainless steel, and Ti−6Al−4 V alloy.
Because of their excellent mechanical qualities and biocompatibility, CoCr and Ti−6Al−4 V materials are increasingly being employed as stem materials. This research used CATIA V−6 to model stem designs with three distinct profiles. The designs included circular, elliptical, oval and trapezoidal shapes with three distinct profiles. Chethan et al (K. N. Chethan, Zuber, et al.,). found that CoCr was the best material to use in the design of the stem.
When an implant is subjected to a constant cyclic load associated with normal daily activities, von Mises stress has a significant effect on the implant’s life. Aseptic loosening is a significant issue with implants (K. N. Chethan, Zuber, et al., Citation2020; Shi et al., Citation2003).
The acetabular cup is most often replaced in THA, possibly owing to the valgus neck position of the widely cemented Poldi endoprosthesis (Št’astný et al., Citation2014). A major improvement in revision THA was achieved in 1980 when oval-shaped cups were introduced into clinical practice. Restoring the anatomical rotation center with these cups necessitated less severe bone excision (Št’astný et al., Citation2014). Sir John Charnley performed the first complete hip arthroplasty in 1958 using a low-friction UHMWPE-on-metal design. This design dealt with the issue of increased wear and shortened joint life (Das & Chakraborti, Citation2018).
The wear of the Ultra-High Molecular Weight Polyethylene (UHMWPE) acetabular cup is the most important component in determining the lifespan of metal on polyethylene (MoP) hip replacements (Zeman et al., Citation2018). It is well established that when prosthetic hip joints are exposed to high levels of stress, the UHMWPE cup subsurface wear adds to the amount of wear debris. Given that surface stress has an effect on the fatigue process, it is becoming extremely critical to decrease contact stress at the cup in order to lower the volume of UHMWPE cup wear debris (Kandemir et al., Citation2018; Korhonen et al., Citation2005; Monif, Citation2012; Rixrath et al., Citation2008; The et al., Citation2008).
According to the subject’s body weight, contact pressures during common activities such as walking, standing and sitting range between 3 and 11 MPa (Hodge et al., Citation1986; Kottan et al., Citation2022; David et al., Citation1998; Saputra et al., Citation2013). When a 1 mm or 3 mm metal backing shell is employed to support the CoCr femur head, the stresses at the UHMWPE cup fall from 27 to 26 MPa and to 25.8 MPa, respectively (Monif, Citation2012).
When a femoral head with a diameter of 32 mm was employed instead of a diameter of 22 mm, stress in the UHMWPE cup dropped by 33%, 25% and 28%, respectively. Lowered stress at the UHMWPE cup was associated with a metal backing thickness of 1 mm; however, there were no statistically significant changes in stress with increasing the metal backing thickness (K N et al.,). Griza et al (Griza et al., Citation2009). used a stem of length 165 mm and exerted a force in accordance with ASTM F 745 guidelines in their study. The lateral section of the stem was found to have the highest von Mises stresses, which was 210 MPa. Among the profiles studied by Chethan et al (K. N. Chethan, Zuber, et al., Citation2020), profile two having femoral head diameter of 36 mm, liner thickness of 2 mm with shell thickness of 1 mm displayed the least von Mises stress of 139 MPa. Additionally, the distortion of the profile two-shaped stem is the smallest at 0.042 mm. Increasing the diameter of the femur head, regardless of the material of the femur head, leads to a considerable decrease in maximum Von Mises stress on the cup made of UHWMPE. As an example, in the case of the titanium alloy femoral head, the stress on the cup drops from 21 to 14 MPa when a 32-mm-diameter femur head is employed instead of a 22 mm diameter (Monif, Citation2012). The results presented in this work matches with the previous works (B. N. S. Chethan et al., Citation2022).
4.1. Limitations of current work
The study’s first limitation is that it only considers linear elastic loading conditions when evaluating the hip implant wear. In order to prevent inaccurate wear rate figures, the dominant contribution of sliding distance or contact pressure should be examined. Furthermore, considering the rate of corrosion in the finite element approach is problematic. As a result, it is not included the corrosion in our model since, according to many academics, corrosion isn’t the primary cause of debris creation when compared to wear (K. N. Chethan, Ogulcan, et al., Citation2020). This work provides a clear understanding of the wear at the junctions of the femoral head and acetabular cup, as well as acetabular cup and backing cup, by taking into account the force and moment acting on the backing cup, and also taking into consideration more realistic loading conditions such as walking, knee bending, sitting and standing up; however, there is just a single designs addressed in this study, ignoring the varying lengths of implants, as well as the different diameters of femoral heads, cups, and backing cup thicknesses. Finally, employing a hip simulator, all of the above-mentioned studies may be carried out in real time (K. N. Chethan et al., Citation2021).
5. Conclusions
Along with the force and moment considered in this work, there are several factors which affects the wear rate in the contact surfaces. The surface finish in the contacting region has a significant effect on the wear debris generation. In previous literature, it tries to find the wear rate in the contacting surfaces considering different loads acting on it. But in all those works, moment is neglected. Moment acting on hip implants along with the loads has a significant effect on the implants. In this work, different loading conditions are considered along with moment acting on it. Hence, this works carries novelty, also the linear wear is close with the real conditions in which these implants are used. Oval-shaped cross-section stem is considered for this study. Linear wear is estimated at the contact surfaces of the femoral head to the acetabular cup and the acetabular cup to the backing cup. A constant femoral head diameter of 28 mm, an acetabular cup thickness of 4 mm, and a backing cup thickness of 2 mm. The Archard’s law used to estimate the linear wear rate. Acetabular cup is made of UHWMPE and the stem, femoral head, and backing cup are made of CoCr, the least overall deformation is 0.394 mm during walking loads. When the stem is considered Ti−6Al−4 V and the acetabular cup of UHWMPE, the minimum wear between the femoral head and acetabular cup is 0.063 mm/year. During a typical standing posture, an acetabular cup-to-backing cup wear rate of 0.007 mm/year is estimated. It is required to consider other activities and also different body weights loading conditions to know how this design responds to these conditions. Before concluding this work, it is necessary to evaluate the obtained results with experimental studies using hip simulator.
Submission declaration
This paper is not being considered for publication elsewhere.
Author contributions
Conceptualisation, Chethan K N; methodology, Sawan Shetty, Satish Shenoy B; software, Numa Shaikh, Chethan K N; validation, Chethan K N, Satish Shenoy; formal analysis, Numa Shaikh; investigation, Numa Shaikh, Sawan Shetty, Shaymasunder Bhat N, Chethan K N; resources, Numa Shaikh, Satish Shenoy B, Shaymasunder Bhat N; data curation, Numa Shaikh; writing—original draft preparation, Numa Shaikh.; writing—review and editing, Chethan K N, Satish Shenoy; visualisation, Numa Shaikh.; supervision, Satish Shenoy B, Shaymasunder Bhat N, Chethan K N; project administration, Chethan K N.; All authors have read and agreed to the published version of the manuscript.
Acknowledgments
The authors thank the Department of Aeronautical and Automobile Engineering, Manipal Institute of Technology, Manipal Academy, Manipal for providing the high computational facility to carry out this research.
Disclosure statement
No potential conflict of interest was reported by the author(s).
Data availability statement
The original contributions presented in the study are included in the article and supplementary materials.
Additional information
Funding
Notes on contributors

Chethan K N
Chethan K N is working as an Associate Professor in the Department of Aeronautical and Automobile Engineering, Manipal Institute of Technology, Manipal Academy of Higher Education, Manipal, Karnataka, INDIA. He holds B. E. (Mechanical Engineering), M.Tech (Manufacturing Engineering & Technology), and Ph.D. (Computational Biomechanics) degrees. Author has worked as post-doctoral research associate in New Jersey institute of Technology, USA. He has a more than 10 years of teaching and research experience. His area of interest includes Biomechanics of the hip joint, Finite element analysis of biomedical implants, Composite materials, and Manufacturing of medical implants. The author has published more than 35 articles in reputed journals related to finite element analysis of hip implants, mechanical characterisation of materials, and composite materials.
References
- Agarwal, A. K., Pramanik, S., Kumar Agarwal, A., Rai, K. N. Chronology of total hip replacement and materials development Chronology of Total Hip Joint Replacement and Materials Development. 2005.
- ASTM. (2015). Standard Practice for Finite Element Analysis (FEA) of non-modular metallic orthopaedic hip femoral stems 1.
- Babaniamansour, P., Ebrahimian-Hosseinabadi, M., & Zargar-Kharazi, A. (2017). Designing an optimized novel femoral stem. Journal of Medical Signals and Sensors, 7(3), 170.
- Bhawe, A. K., Shah, K. M., Somani, S., Shenoy B, S., Bhat N, S., Zuber, M., & K N, C. (2022). Static structural analysis of the effect of change in femoral head sizes used in total hip arthroplasty using finite element method. Cogent Engineering, 9(1). https://doi.org/10.1080/23311916.2022.2027080
- Chen, D. W., Lee, M. S., Lin, C.-L. (2018). Finite Element Analysis of Stresses from Hip Implants with Different Head Sizes.
- Chethan, K. N., Ogulcan, G., Zuber, M., Shenoy, S., & Shenoy b, S. (2020). Wear estimation of trapezoidal and circular shaped hip implants along with varying taper trunnion radiuses using finite element method. Computer Methods and Programs in Biomedicine, 196, 105597. https://doi.org/10.1016/j.cmpb.2020.105597
- Chethan, K. N., Shyamasunder Bhat, N, & Satish Shenoy, B (2014). Biomechanics of hip joint: A review. International Journal of Biomedical Engineering and Technology, 15(4), 341. https://doi.org/10.1504/IJBET.2014.064824
- Chethan, K. N., Shyamasunder Bhat, N., Zuber, M., & Satish Shenoy, B. (2021). Finite element analysis of hip implant with varying in taper neck lengths under static loading conditions. Computer Methods and Programs in Biomedicine, 208, 106273. https://doi.org/10.1016/j.cmpb.2021.106273
- Chethan, K. N., Zuber, M., Bhat, S. N., & Shenoy, S. B. (2019). Comparative study of femur bone having different boundary conditions and bone structure using finite element method. The Open Biomedical Engineering Journal, 12(1), 115–14. https://doi.org/10.2174/1874120701812010115
- Chethan, K. N., Zuber, M., Shyamasunder, B. N., Satish, S. B., & Shepherd, D. (2020). Optimized trapezoidal-shaped hip implant for total hip arthroplasty using finite element analysis. Cogent Engineering, 7(1), 1–18. https://doi.org/10.1080/23311916.2020.1719575
- Chethan, B. N. S., Zuber, M., S, S. B., & Shenoy B, S. (2022). Evolution of different designs and wear studies in total hip prosthesis using finite element analysis: A review. Cogent Engineering, 9(1). https://doi.org/10.1080/23311916.2022.2027081
- Clarke, B. (2008). Normal bone anatomy and physiology. Clinical Journal of the American Society of Nephrology: CJASN, 3 Suppl 3(Supplement_3), S131–S139. https://doi.org/10.2215/CJN.04151206
- Colic, K., & Sedmak, A. (2016). The current approach to research and design of the artificial hip prosthesis: A review. Rheumatology and Orthopedic Medicine, 1(1). https://doi.org/10.15761/ROM.1000106
- Das, S. S., & Chakraborti, P. (2018). Development of biomaterial for total hip joint replacement. Proceedings of the IOP Conference Series: Materials Science and Engineering Sikkim, India, (Vol. 377, No. (1), pp. 012177). IOP Publishing.
- David, E. K., Robbins, C. E., & Lavine, L. (1998). Hip Biomechanics During Gait.
- Delikanli, Y. E., & Kayacan, M. C. (2019). Design, manufacture, and fatigue analysis of lightweight hip implants. Journal of Applied Biomaterials and Functional Materials, 17(2), 228080001983683. https://doi.org/10.1177/2280800019836830
- Foucher, K. C. (2016). Identifying clinically meaningful benchmarks for gait improvement after total hip arthroplasty. Journal of Orthopaedic Research, 34(1), 88–96. https://doi.org/10.1002/jor.22996
- Griza, S., Zanon, G., Silva, E. P., Bertoni, F., Reguly, A., & Strohaecker, T. R. (2009). Design aspects involved in a cemented THA stem failure case. Engineering Failure Analysis, 16(1), 512–520. https://doi.org/10.1016/j.engfailanal.2008.06.016
- Gutmann, C., Shaikh, N., Shenoy, B. S., Shaymasunder Bhat, N., Keni, L. G., K N, & Chethan, C. (2023). Wear estimation of hip implants with varying chamfer geometry at the trunnion junction: A finite element analysis. Biomedical Physics & Engineering Express, 9(3), 035004. https://doi.org/10.1088/2057-1976/acb710
- Hampton, S. J., Andriacchi, T. P., & Galante, J. O. (1980). Three dimensional stress analysis of the femoral stem of a total hip prosthesis. Journal of Biomechanics, 13(5), 443–448. https://doi.org/10.1016/0021-9290(80)90038-X
- Herrmann, S., Kaehler, M., Souffrant, R., Rachholz, R., Zierath, J., Kluess, D., Mittelmeier, W., Woernle, C., & Bader, R. (2012). HiL simulation in biomechanics: A new approach for testing total joint replacements. Computer Methods and Programs in Biomedicine, 105(2), 109–119. https://doi.org/10.1016/j.cmpb.2011.07.012
- Hodge, W. A., Fujant, R. S., Carlsont, K. L., Burgesst, R. G., Harris, W. H., Manntt, R. W. (1986). Contact pressures in the human hip joint measured in vivo (synovial joints/osteoarthritis/postsurgical rehabilitation/Joint replacement prostheses/gait analysis).
- Hua, X., Li, J., De Pieri, E., & Ferguson, S. J. (2022). Multiscale biomechanics of the biphasic articular cartilage in the natural hip joint during routine activities. Computer Methods and Programs in Biomedicine, 215, 106606. https://doi.org/10.1016/j.cmpb.2021.106606
- HUNG, J.-P., & JS-S, W. U. (2002). A comparative study on wear behavior of hip prosthesis by finite element simulation. Biomedical Engineering: Applications, Basis and Communications, 14(4), 139–148. https://doi.org/10.4015/S1016237202000218
- Hu, C. Y., & Yoon, T. R. (2018). Recent updates for biomaterials used in total hip arthroplasty. Biomaterials Research, 22(1), 1–12. https://doi.org/10.1186/s40824-018-0144-8
- Jiang, H. (2007). Static and Dynamic Mechanics Analysis on Artificial Hip Joints with Different Interface Designs by the Finite Element Method. Journal of Bionic Engineering, 4(2), 123–131. https://doi.org/10.1016/S1672-6529(07)60024-9
- Jun, Y., & Choi, K. (2010). Design of patient-specific hip implants based on the 3D geometry of the human femur. Advances in Engineering Software, 41(4), 537–547. https://doi.org/10.1016/j.advengsoft.2009.10.016
- Kandemir, G., Smith, S., & Joyce, T. J. (2018). The influence of contact stress on the wear of cross-linked polyethylene. Proceedings of the Institution of Mechanical Engineers, Part H: Journal of Engineering in Medicine, 232(10), 1008–1016. https://doi.org/10.1177/0954411918796047
- Kim, J. T., & Yoo, J. J. (2016). Implant design in cementless hip arthroplasty. Hip & Pelvis, 28(2), 65. https://doi.org/10.5371/hp.2016.28.2.65
- Koch, F. W., Koch, A. K., Amling, M., & Hahn, M. (2018). Histopathologische Befunde am Interface einer künstlichen Hüftpfanne mit einer 3D-Titannetz-Beschichtung nach einer Standzeit von 27 Jahren. Osteologie, 27(3), 165–171. https://doi.org/10.1055/s-0038-1673539
- Korhonen, R. K., Koistinen, A., Konttinen, Y. T., Santavirta, S. S., & Lappalainen, R. (2005). The effect of geometry and abduction angle on the stresses in cemented UHMWPE acetabular cups – finite element simulations and experimental tests. BioMedical Engineering Online, 4(1). https://doi.org/10.1186/1475-925X-4-32
- Kottan, N., Gowtham, N. H., & Basu, B. (2022). Development and validation of a finite element model of wear in UHMWPE liner using experimental data from hip simulator studies. Journal of Biomechanical Engineering, 144(3). https://doi.org/10.1115/1.4052373
- Lunn, D. E., Chapman, G. J., & Redmond, A. C. (2019). Hip kinematics and kinetics in total hip replacement patients stratified by age and functional capacity. Journal of Biomechanics, 87, 19–27. https://doi.org/10.1016/j.jbiomech.2019.02.002
- Merola, M., & Affatato, S. (2019). Materials for hip prostheses: A review of wear and loading considerations. Materials, 12(3), 495. https://doi.org/10.3390/ma12030495
- Monif, M. M. (2012). Finite element study on the predicted equivalent stresses in the artificial hip joint. Journal of Biomedical Science and Engineering, 05(2), 43–51. https://doi.org/10.4236/jbise.2012.52007
- Morlock, M. M., Bishop, N., & Huber, G. (2011). Biomechanics of hip arthroplasty. In Tribology in Total Hip Arthroplasty (pp. 11–24). Springer Berlin Heidelberg. https://doi.org/10.1007/978-3-642-19429-0_2
- Pickering, S., Donnelly, B., & Crawford, R. (2009). Computerized Hip Navigation. Surgical Treatment of Hip Arthritis: Reconstruction, Replacement, and Revision, 445–455. https://doi.org/10.1016/B978-1-4160-5898-4.00060-4
- Rixrath, E., Wendling-Mansuy, S., Flecher, X., Chabrand, P., & Argenson, J. N. (2008). Design parameters dependences on contact stress distribution in gait and jogging phases after total hip arthroplasty. Journal of Biomechanics, 41(5), 1137–1142. https://doi.org/10.1016/j.jbiomech.2007.12.009
- Saputra, E., Anwar, I. B., Jamari, J., & Van Der Heide, E. (2013). Finite element analysis of artificial hip joint movement during human activities. Procedia Engineering, 68, 102–108. https://doi.org/10.1016/j.proeng.2013.12.154
- Shi, B., Ajayi, O. O., Fenske, G., Erdemir, A., & Liang, H. (2003). Tribological performance of some alternative bearing materials for artificial joints. Wear, 255(7–12), 1015–1021. https://doi.org/10.1016/S0043-1648(03)00276-X
- Shrestha, S. (2017). Wear behavior of Ti-6Al-4V for Joint Implants manufactured by Electron Beam Melting.
- Šťastný, E., Trč, T., Handl, M., Kos, P., Kautzner, J., Philippou, T., & Lisý, J. (2014). Mid-term results of revision total hip arthroplasty using the oval-shaped uncemented Trč-Cingr cup. International Orthopaedics, 38(5), 935–940. Epub 2014 Jan 16. PMID: 24430429; PMCID: PMC3997770. https://doi.org/10.1007/s00264-013-2269-7
- The, B., Hosman, A., Kootstra, J., Kralj-Iglic, V., Flivik, G., Verdonschot, N., & Diercks, R. (2008). Association between contact hip stress and RSA-measured wear rates in total hip arthroplasties of 31 patients. Journal of Biomechanics, 41(1), 100–105. https://doi.org/10.1016/j.jbiomech.2007.07.010
- Wang, Q. J., & Chung, Y. (Eds.) (2013). Encyclopedia of Tribology. Springer US. https://doi.org/10.1007/978-0-387-92897-5
- Wu, J. S., Hung, J. P., Shu, C. S., & Chen, J. H. (2003). The computer simulation of wear behavior appearing in total hip prosthesis. Computer Methods and Programs in Biomedicine, 70(1), 81–91. PMID: 12468129. https://doi.org/10.1016/s0169-2607(01)00199-7
- Zeman, J., Ranuša, M., Vrbka, M., Gallo, J., Křupka, I., & Hartl, M. (2018). UHMWPE acetabular cup creep deformation during the run-in phase of THA’s life cycle. Journal of the Mechanical Behavior of Biomedical Materials, 87, 30–39. https://doi.org/10.1016/j.jmbbm.2018.07.015