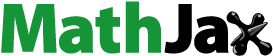
Abstract
This research discusses the machine component distortion problem after the hardening process. This research focuses on the inside diameter of the hole and gap width dimension changes in components. Austenite temperature, quench medium, and material thickness were examined to determine their effect on distortion. The components are made of AISI 4140 machinery steel group. Another idea in this study is to substitute the material with AISI 4340 to minimize the risk of distortion. This study used a Navy C-Ring specimen. This study applies the Design of Experiment, followed by statistical analysis with ANOVA and Response Surface optimization. The austenite temperature of 895.2°C, oil quench medium, and 8 mm thickness are the best hardening parameters for AISI 4140, which reduce inside diameter distortion up to 82.51% and reduce gap width distortion up to 78.16%. Austenite temperature of 900°C, oil cooling medium, and 8 mm thickness is the best hardening parameter for AISI 4340, which reduces inside diameter distortion by up to 62.23% and reduces gap width distortion by up to 86.61%. Material substitution of AISI 4140 with AISI 4340 can be done by considering the risk of warping.
1. Introduction
SC108 clamping tools are one of the cigarette machine components in the enumerator’s sub-function section. It functions as a holder for disc cutters cutting tools on the right and left sides enumerator. The disc cutter will chop or slice dry tobacco before being processed on a cigarette-making machine. One cigarette machine has 72 pairs of SC108 clamping tools and 20 replacement parts. It has a rectangular block design with a hole and slot on one side, as shown in Figure . The hole is used to hold cutting tool holders, while the slot is used to increase clamping strength. The difference in size between the hole’s inside diameter and the rectangular block’s outer side is 3 mm adrift per section (Figure ).
This component needs high toughness and hardness values for long service life. AISI 4140 steel was chosen as the raw material for making this component. AISI 4140 is used because of its high strength, wear resistance, and good toughness (Bilal et al., Citation2019; Gurumurthy et al., Citation2021; Mahmood et al., Citation2022; Zheng et al., Citation2013). The hardening process is carried out on this component to increase the material’s mechanical strength, hardness, durability, and better material toughness (Bilal et al., Citation2019; Gurumurthy et al., Citation2021). The current hardening process uses an austenite temperature parameter of 850°C, a water-oil quench medium, with a component thickness of 8 mm. Distortion problems appear after the hardening process with this critical geometry. There is a dimension change in the inside diameter and gap width contour (Figure ). Inside diameter and gap width dimension change cause the failure of the component to grip the cutting tools perfectly. A component redesign is impossible, considering the components overlapping position that can affect overall work design of the machine.
Hardening and quenching processes impact microstructural changes, material stress (Nallathambi et al., Citation2009; Safi & Givi, Citation2014), and deformation (Decroos & Seefeldt, Citation2017; Li et al., Citation2014; Sonar et al., Citation2018). Distortion is the most common heat treatment problem (Canale & Totten, Citation2005). Distortion occurs during the hardening and tempering processes (Ray et al., Citation2020). Distortion is an irreversible and unpredictable change during heat treatment (Guterres et al., Citation2017; Rajan et al., Citation2011). Two distortion problems are shape and size changes (Canale & Totten, Citation2005; Guterres et al., Citation2017; Rajan et al., Citation2011; Ray et al., Citation2020; Sonar et al., Citation2018). Components distortion is detrimental because it causes unbalanced forces (Decroos & Seefeldt, Citation2017). This affects the functional performance of parts and causes economic losses (Sonar et al., Citation2018), so it needs to be repaired. Material distortion is corrected to return parts to their original dimensions and shape (Clark et al., Citation2014). Repair processes can be carried out by welding (Hardin & Beckermann, Citation2005) and machining processes, but the costs incurred are costly and consume much time (Clark et al., Citation2014; Decroos & Seefeldt, Citation2017; Farivar et al., Citation2018; Li et al., Citation2006; Lopez-Garcia et al. Citation2022; Samuel & Prabhu, Citation2022). Nunes et al (Nunes et al., Citation2018). state that repairing distortion increases production costs by 20% to 40%. To minimize distortion and reduce economic loss, exercise optimal control over the hardening and quenching processes (Farivar et al., Citation2018; Lopez-Garcia et al., Citation2022; Samuel & Prabhu, Citation2022).
Distortion in the hardening process occurs due to the steel transformation process (B. E. A., Bhagyalaxmi et al., Citation2018; Canale & Totten, Citation2005; Farivar et al., Citation2018; Liu et al., Citation2017; Samuel & Prabhu, Citation2022; Silva et al., Citation2012) becoming: residual austenite or retained austenite (Clark et al., Citation2014; Northwood et al., Citation2007), residual material stress (Canale & Totten, Citation2005; Hardin & Beckermann, Citation2005; Lopez-Garcia et al., Citation2016; Northwood et al., Citation2007; Nunes et al., Citation2018; Samuel & Prabhu, Citation2022), as well as thermal expansion and contraction (Canale & Totten, Citation2005; Clark et al., Citation2014; Liscic et al., Citation2010; Passanha et al., Citation2022; Samuel & Prabhu, Citation2022; Silva et al., Citation2012), and heat conduction/transfer coefficient (Farivar et al., Citation2018; Li et al., Citation2014; Nallathambi et al., Citation2009, Citation2010; Rajan et al., Citation2011; Watanabe et al., Citation2020). A higher heat transfer rate in high-temperature and martensitic temperature regions increases the residual stresses that develop in a specimen (Passanha et al., Citation2022). These stresses could result in high residual stresses and distortions, increasing the probability of cracking (Canale & Totten, Citation2005). This process is not evenly distributed across the components. Material distortion is generally affected by heating rate, component shape and size, component thickness and geometry, microstructure non-uniformity, and cooling rate (Rajan et al., Citation2011). Uniform grain size and microstructure will also affect to material distortion (Zhang et al., Citation2021). At the same time, other studies (Farivar et al., Citation2018; Li et al., Citation2014; Northwood et al., Citation2007; Solic et al., Citation2018) revealed that the austenite temperature affects internal stress and distortion. Farivar et al (Farivar et al., Citation2018). also showed that austenite temperature modification could reduce distortion by 27% while maintaining its hardness properties.
Quenching, as one of the most important processes in heat treatments, can also cause the formation of thermal and transformational stresses, which may result in distortion and cracks (Hasan et al., Citation2018). Research related to other material distortions, as previously done, suggests that the cooling speed in the hardening process affects the occurrence of material distortion (Canale & Totten, Citation2005; Clark et al., Citation2014; Decroos & Seefeldt, Citation2017; Guterres et al., Citation2017; Hardin & Beckermann, Citation2005; Li et al., Citation2006, Citation2014; Nallathambi et al., Citation2009; Samuel & Prabhu, Citation2022; Sonar et al., Citation2018; Watanabe et al., Citation2020). There are also several problems in the quenching process, such as distortion and cracking caused by internal stress (Liu et al., Citation2017). Rapid cooling causes losses such as cracks or changes in dimensions (Bhagyalaxmi et al., Citation2023). Optimizing the heat transfer coefficient in the quenching process minimizes residual stress and distortion (Li et al., Citation2006). Meanwhile, slower cooling can also reduce the risk of distortion (Clark et al., Citation2014; Nallathambi et al., Citation2009). Mineral oils are popularly used to give a slower and more even cooling, resulting in lesser internal stresses (Passanha et al., Citation2022), although at the risk of not producing enough hardness (Bhagyalaxmi et al., Citation2023). Other studies have combined factors of austenite temperature and quench medium to determine the impact of changes on distortion (Lopez-Garcia et al., Citation2016, Citation2022; Nunes et al., Citation2018; Zheng et al., Citation2013). Austenite temperature and quenching medium are the most critical parameters that cause the risk of distortion in quench process (Lopez-Garcia et al., Citation2016). The inappropriate selection of austenite temperature and quench medium causes distortion and cracks (Solic et al., Citation2018). Quench medium and cooling speed affect the magnitude and direction of distortion after the hardening process (Totten et al., Citation2002). Research Lopez-Garcia et al (Lopez-Garcia et al., Citation2022). has also shown that distortion is closely related to heat transfer rate during quenching and components geometry. Research involving thickness and cooling speed factors is related to distortion (Nan et al., Citation2009). Previous studies (Decroos & Seefeldt, Citation2017; Li et al., Citation2014; Lopez-Garcia et al., Citation2016; Nan et al., Citation2009; Nunes et al., Citation2018; Samuel & Prabhu, Citation2022) have stated that component thickness’s difference and geometrical complexity cause phase transformations at different times to produce residual stresses and distortions. Internal stress is caused by nonuniform cooling at different locations of a component (Liu et al., Citation2017). Critical geometry drives material distortions (Lopez-Garcia et al., Citation2022; Watanabe et al., Citation2020). Thickness variations are used to evaluate the dimensions and shape distortion for different hardening parameters (Nan et al., Citation2009). Quench medium with a high cooling rate should not be used for components with complex geometries or critical sizes (Passanha et al., Citation2022). Thus, it was concluded that microstructure non-uniformity, heating temperature, cooling medium, and geometry affected material distortion. It becomes a technical challenge of quenching for every heat treatment engineer to choose the quench medium and hardening parameters that will minimize the stresses to reduce cracking and distortion (Hasan et al., Citation2018).
This study thoroughly examines the effect of austenite temperature, quench medium, and material thickness on the risk of inside diameter and gap width distortion in the hardening process. This study involves a combination of austenite temperature, quench medium, and material thickness, in experimental designs and statistical analysis to determine the impact on material dimension changes. The application of a combination of austenite temperature factors, quench medium, and material thickness simultaneously is a novelty in this research.
This research involved AISI 4340 material as a substitution option for AISI 4140 material. AISI 4340 and AISI 4140 are classified as machinery steel and similarly composed. Gurumurthy et al (Gurumurthy et al., Citation2021). research concluded that AISI 4340 has better wear resistance and distortion resistance than AISI 4140 and AISI 1040. Material quality comparison of AISI 4140 and AISI 4340 based on distortion response was carried out to know whether the material substitution process can be carried out to minimize distortion inside diameter and gap width. It is expected to know whether AISI 4340 steel can substitute AISI 4140 to reduce the risk of distortion. The novelty of this research is related to the quality comparison of AISI 4140 and AISI 4340. The research explores material distortion after the hardening process, namely the dimensions changes of inside diameter and gap width.
The experiment used a Navy C-Ring specimen, with the dependent variable being inside diameter and gap width distortion value. Navy C-Ring specimens are the standard method for analyzing steel distortion during quenching in heat treatment (Samuel & Prabhu, Citation2022; Sonar et al., Citation2018). Dimension and contour selection as responses in this study were adjusted to the critical geometric contours of the SC108 clamping component.
This research applies the Design of Experiment (DoE) to produce experiments and verify variables’ behavior and interactions (Nunes et al., Citation2018). These techniques are ideal for an effective experimental design to get minimum cost (Hazir & Ozcan, Citation2018). It will produce optimal hardening process parameters based on statistics (Anggoro et al., Citation2019). Experimental results were also statistically analyzed using ANOVA to find the contribution of each factor (Fakir et al., Citation2020). Response Surface Method (RSM) forms an equation model and process optimization (Anggoro et al., Citation2022, Citation2023; Ismartaya et al., Citation2022; Sari et al., Citation2022). Response surface models are used to build a relationship between the objective function and constraints about design variables (Li et al., Citation2006).
State-of-the-art research is the application of design of experiment methods with statistical analysis and multiple-responses optimization to identify factors contributing to distortion of AISI 4140 and AISI 4340, and find hardening process parameters that solve distortion problems. The research aims to obtain optimal hardening parameters that minimize the inside diameter and gap width distortion.
The research output can overcome the distortion problem in components manufacture, especially in steel and automotive manufacturing industries. With this research, heat treatment operators can take advantage of changes in hardening parameters to minimize the risk of steel distortion after the hardening process. Controlling component distortion is the ultimate goal of heat treatment practitioners (Hasan et al., Citation2018). Another advantage that can be obtained is that the material engineer will be assisted in selecting materials, and the design engineer can estimate dimensions or designs with the least risk of distortion.
2. Materials and methodology
2.1. Research methodology
SC108 component distortion problems are found in many observations in the manufacturing industry. Brainstorming and literature review results indicate that it is necessary to investigate all factors in the hardening process and material substitution experiments. Three factors are defined: austenite temperature, quench medium, and material thickness, contributing to material distortion. AISI 4140 steel is compared with AISI 4340 to determine which material can produce the minimum distortion. The goal is to obtain optimal hardening parameters and determine the correct type of material to minimize distortion.
Navy C-ring-shaped specimens were used to identify dimensional changes before and after the experiment. Response Surface Method with Box-Behnken design is used as the basis for DoE. RSM offers an assessment of the sensitivity of these optimum conditions to the variations of the experimental variables it quantifies the response-input variable relationship (Djimtoingar et al., Citation2022). Box-Behnken designs have been widely used in engineering applications (Hazir & Ozcan, Citation2018).
Hardening is carried out with designed process parameters; specimen measurements are carried out on the inside diameter and gap width dimensions. Measurements are carried out using a Profile Projector machine with an accuracy of 0.001 mm.
The measurement data is documented and processed with RSM. The second-order mathematical model was developed by using RSM (Hazir & Ozcan, Citation2018). Mathematical models were analyzed using ANOVA with Minitab 19 software. Anova is applied to determine the contribution of each hardening factor to distortion. Minitab 19 software is also used to perform advanced analysis of main effects and interaction plots. The last step is to optimize multiple responses with Response Surface Optimization in Design Expert 13 software. RSM is designed to optimize a response affected by multiple variables (Djimtoingar et al., Citation2022). In this study, optimization was carried out using smaller-the-better quality characteristics. The final result of the Methodology is briefly shown in Figure .
2.2. Materials
AISI 4140 and AISI 4340 are machinery steel types. Based on the carbon content, AISI 4140 and AISI 4340 are medium-carbon steel types. Material composition data for AISI 4140 is shown in Table (B. E. A., Bhagyalaxmi et al., Citation2018; Lopez-Garcia et al., Citation2022; Mehrabi et al., Citation2020), and for AISI 4340 is shown in Table (Clark et al., Citation2014; Gurumurthy et al., Citation2021; Mahmood et al., Citation2022; Safi & Givi, Citation2014).
Table 1. AISI 4140 chemical composition
Table 2. AISI 4340 chemical composition
2.3. Heat treatment process
This study applies three factors: austenite temperature, quench medium, and material thickness. Each factor has three variations in treatment levels, shown explicitly in Table .
Table 3. Factors and levels in research
The austenite temperature suggested by the distributor is between 830°C and 860°C, used in this study. The austenite temperature of 900°C was chosen because it achieves microstructural changes (Bilal et al., Citation2019). The cooling rate significantly influences steel’s microstructure (Bhagyalaxmi et al., Citation2023). Quench medium type is one of the parameters because minimizing distortion can be done by modifying the quenching process (Farivar et al., Citation2018). The purpose of the quenching process in heat treatment is to modify the steel microstructure by cooling the components from austenite temperature using different quench mediums such as brine, water, or oil (Lopez-Garcia et al., Citation2022). The quenching intensity are affected by changing the quenching type (Hasan et al., Citation2014). The quench medium in this study used the options suggested by the distributor: water, oil, and a combination of water-oil quench. Control optimization of the quenching process and appropriate selection of quenchants minimize residual stress and distortion (Li et al., Citation2006; Samuel & Prabhu, Citation2022). Quench medium must be suitable because a high cooling rate encourages higher surface and core tension (Zheng et al., Citation2013), causing axial distortion (Li et al., Citation2014). In addition, selecting a quench medium must consider the component’s geometric complexity to minimize the potential for cracks and distortion (Liscic et al., Citation2010). Thickness variations on different hardening parameters cause internal stresses, size, and shape distortions in components (Nan et al., Citation2009). The thickness factor uses three levels of variation. The thickness of the specimen is adjusted to the actual thickness dimension of the SC108 clamping component, which is 8 mm. Variation in thickness is used to identify the effect of changes in material thickness on distortion statistically.
2.4. Design of experiment and statistic analysis
Design of Experiment (DoE) methodology is a powerful technique to reduce replicates (Hazir & Ozcan, Citation2018). DoE consists of three stages: pre-experimental planning, execution, and statistical analysis of the collected data. The pre-experimental planning stage is crucial because factors, levels, and response variables must be selected according to experiment objectives (Nunes et al., Citation2018). The next pre-experimental stage is to develop an experimental design using Response Surface Method (RSM). The RSM is a set of mathematical and statistical techniques that are useful for modeling and analyzing problems in which the observed response is affected by several variables, and the aim is to obtain the optimal value of the response (Anggoro et al., Citation2021, Citation2022, Citation2023; Djimtoingar et al., Citation2022; Ismartaya et al., Citation2022; Sari et al., Citation2022). This technique is an efficient and ideal for determining experimental factors (Hazir & Ozcan, Citation2018). The RSM with Box-Behnken design is used for this research. The Box-Behnken design is more economical and efficient than FFD, especially in large experimental numbers, and slightly more labor efficient than CCD (Djimtoingar et al., Citation2022). RSM experimental design with Box-Behnken design is shown in Table .
Table 4. Response surface method experimental design (coded)
The hardening process is applied to AISI 4140 and AISI 4340 materials according to Response Surface Method design test parameters.
The advantage of RSM design presents to development of second-order quadratic mathematical models (Hazir & Ozcan, Citation2018). A second-order quadratic model was created to predict the responses. It is a method that investigates the effective parameters of a response (Ismartaya et al., Citation2022). The precision and fitness of a quadratic mathematical model are checked from its coefficient of determination (R2) and adjusted R2 (Anggoro et al., Citation2019; Djimtoingar et al., Citation2022; Zhu et al., Citation2022). The higher R2 and adjusted R2 indicate the better fitting of the model with the data (Zhu et al., Citation2022). Since the R2 is the square of the coefficient of correlation (R), the values of R2 lie between 0 and 1 (Anggoro et al., Citation2021, Citation2023; Djimtoingar et al., Citation2022). Furthermore, analysis with ANOVA was carried out. ANOVA identifies and measures the impact of different input variables on a given response (Djimtoingar et al., Citation2022). In ANOVA, the p-value is less than 0.05 showing that the factor is significant at a 95% confidence level (Hazir & Ozcan, Citation2018; Zhu et al., Citation2022).
Furthermore, multiple-responses optimization is performed using smaller-the-better quality characteristics. The best assessment of response results is carried out by utilizing the desirability function. The desirability function technique is one of the most widely used engineering applications for parameter optimization (Hazir & Ozcan, Citation2018). Hazir and Ozcan (Citation2018) state that the value of the desirability function is between 0 and 1, it increases as the desirability of the corresponding response increases.
2.5. Material preparation
Research of Hardin and Beckermann (Citation2005) (Hardin & Beckermann, Citation2005); Nan et al. (Citation2009) (Nan et al., Citation2009); Silva et al. (Citation2012) (Silva et al., Citation2012); Clark et al. (Citation2014) (Clark et al., Citation2014); Farivar et al. (Citation2018) (Farivar et al., Citation2018); Sonar et al. (Citation2018) (Sonar et al., Citation2018); Nunes et al. (Citation2018) (Nunes et al., Citation2018); and Zhang et al. (Citation2021) (Zhang et al., Citation2021) using a Navy C-ring shaped specimen. Navy C ring specimens are appropriate for studying material distortion relationships (Totten et al., Citation2002). Navy C-ring specimens are specifically designed to observe and evaluate dimensional changes or distortions (Nunes et al., Citation2018) due to various hardening processes (Nan et al., Citation2009; Samuel & Prabhu, Citation2022). Generally, the distortion that is assessed on Navy C-ring specimens is inside diameter, outside diameter, and gap width (Northwood et al., Citation2007). Details of the Navy C-ring used in this study are shown in Figure . This study evaluates the distortion in inside diameter and gap width, adjusted for the actual problems in the SC108 clamping tools component. Figure shows the distortion of the Navy C-ring that occurred in this study.
2.6. Dimension measurement
The Navy C-Ring specimens’ inside diameter and gap width were measured before and after the hardening process. Dimensional measurement aims to record changes in size that occur due to the hardening process. Measurements were made using a Profile Projector measurement machine.
3. Result and discussion
3.1. AISI 4140 experiment result and analysis
AISI 4140 material experiment with the RSM experimental design produce data value inside diameter and gap width distortion, as shown in Table .
Table 5. AISI 4140 dimensional measurement results test
The RSM was used as an optimization technique to obtain the optimal value by forming a regression model (Anggoro et al., Citation2019, Citation2022). The RSM utilizes system modeling and problem analysis to create a response of interest (Anggoro et al., Citation2021, Citation2022). Furthermore, the experimental results are processed to obtain a quadratic equation, as shown in the following formula model. EquationEquation 11
1 shows the inside diameter distortion (ID) model, while EquationEquation 2
2
2 shows the gap width (GW) distortion model.
The regression model is a mathematical model that shows the relationship between the independent variables (x) and response (y). This model can predict response, optimize the process, and control the process (Anggoro et al., Citation2022). The quadratic model in EquationEquation 11
1 for inside diameter distortion has an R2 value of 0.9622 and an Adjusted R2 of 0.8941. These results indicate that the independent variables in a formed quadratic model can explain 96.22% of dependent variable diversity.
ANOVA testing was carried out on experimental results with Response Surface Method analysis. ANOVA was used to evaluate the significance of the regression model and individual model coefficients of the parameters contributing to the response variables (Anggoro et al., Citation2019, Citation2021). The results of ANOVA analysis for experimental inside diameter distortion value produce data are shown in Table .
Table 6. Analysis of variance for inside diameter distortion AISI 4140
ANOVA results in Table show that the contribution for each hardening factor to the inside diameter distortion value achieved is: austenite temperature (A) 40.35% and 0.75%, quenching medium (B) 15,04% and 2.26%, thickness (C) 17.04% and 3.01%. P-value and its percentage (%) contribution provided confidence to the significance level of all variables. The regression model provided a p-value lower than 0.05, indicating that both models are statistically significant at a 95% confidence level (Anggoro et al., Citation2021). 5% significance level of the p-value confirms that the difference between the means is statistically significant for the ratio of variance F comparable to the standard table value (Anggoro et al., Citation2021; Fakir et al., Citation2020). The significant contribution value and strong p-value indicate that the austenite temperature is a factor that significantly influences the inside diameter distortion value. However, all factors also significantly affect the inside diameter distortion value. This result is reinforced by the main effects and interaction plots for distortion of the inside diameter in Figure below.
Figure shows that all factors significantly affect the response of the inside diameter. The minimal austenite temperature provides the most negligible in-diameter distortion response. The slower cooling rate of the medium quench will provide a minimal distortion response. Similarly, the material thickness factor, the thicker the hardened material, the less distortion response will be. Figure shows the interaction of each factor level and the impact of distortions. The subject matter component has a thickness of 8 mm. Thus, the evaluation is carried out in Figure , row 3, with a red line. In line with the main plot effects graph, the minimal austenite temperature and the slowest medium quench provide minimal distortion.
EquationEquation 22
2 shows the gap width (GW) distortion model material AISI 4140.
The quadratic model in EquationEquation 22
2 gap width distortion has an R2 value of 0.9408 and an Adjusted R2 of 0.8342. These results indicate that the independent variables in the formed quadratic model can explain 94.08% of dependent variable diversity. The results of ANOVA analysis for experimental gap width distortion value produce data are shown in Table .
Table 7. Analysis of Variance for gap width distortion AISI 4140
ANOVA results in Table show that the contribution for each hardening factor to gap width distortion value achieved are: austenite temperature (A) 28.37%, quench medium (B) 19.32% and 19.03%, thickness (C) 19.32% and 10.12%. All setting factors have the same percentage contribution and p-value, indicating that the austenite temperature, quench medium, and thickness significantly affect the gap width distortion value. The main effects and interaction plots for gap width distortion in Figure reinforce the ANOVA results.
Figure shows that all factors significantly affect the response gap width in AISI 4140 material. The highest austenite temperature gives the smallest distortion response at the response gap width. The slowest medium quench cooling rate provides minimal distortion response. Just like gap width distortion, the thicker the hardened material, the less distortion response will be. Figure shows the interaction of each factor level and the impact of distortions. The subject matter component has a thickness of 8 mm, so the evaluation is carried out on Figure , row 3, with a red line. In line with the main plot effects graph, the maximum austenite temperature and the slowest medium quench provide minimal distortion.
3.2. AISI 4140 multiple-responses optimization of distortion
The multiple-responses optimization process are carried out using the features in Design Expert 13 software. The optimization process is set to a minimum position for inside diameter and gap width distortion. It means that smaller-the-better-quality characteristics selected the distortion responses value. The optimization process is carried out for each medium quench factor. The medium quench factor is categorical data, so the coded value must be an integer. Material thickness is set at 8 mm according to the dimensions of the SC108 clamping tools component. Thus, the optimization process produces hardening parameters that follow actual conditions.
The three best hardening process parameters are the results of an optimization process based on the type of quench medium used. The desirability value determines the best hardening parameter (Anggoro et al., Citation2019, Citation2022; Ismartaya et al., Citation2022). The highest desirability value indicates that the hardening parameter produces the minimum inside diameter and gap width distortion values. A comparison of the best hardening parameters based on the type of quench medium is shown in Table below.
Table 8. Optimum comparison of hardening parameter for AISI 4140
Response surface graph of hardening parameter A2.88-B3-C2 for AISI 4140 inside diameter and gap width distortion are shown in Figures below.
The best hardening parameter for AISI 4140 steel is A2.88-B3-C2, with 895.2°C austenite temperature, oil quenches medium and 8 mm of thickness. This hardening parameter is predicted to produce the minimum distortion value, namely 0.025 mm inside diameter distortion and 0.093 mm gap width distortion. This hardening parameter has the highest desirability with a value of 0.977. The desirability function (dF) can be used to optimize the multiple-response in RSM (Anggoro et al., Citation2021; Ismartaya et al., Citation2022). The desirability value ranges from 0 to 1 (Hazir & Ozcan, Citation2018). If the value of desirability is 0, the predicted response value is unacceptable, and if the value of desirability is 1, the prediction of the response value is as desired (Anggoro et al., Citation2022).
The response surface graphs for austenite temperature in Figures show behavioral similarities with the main effects plot graphs in Figure and Figure . Austenite temperatures that are too low generally present minimal risk of distortion. Similarly, the material’s behavior changes the cooling rate of the quench medium. The risk of distortion decreases with a decrease in the cooling rate of the material. However, it is different from the behavior shown after the change in thickness. In multiple-responses optimization, it is known that thickness changes do not significantly affect distortion response. This shows that the selected hardening process parameters are not influenced by the thickness of the material, considering that in its selection, the thickness of the material is set at a value of 8 mm.
At this stage, experiments were also carried out on Navy C-ring specimens using actual parameters currently used in the industry. Austenite temperature of 850°C, water-oil quench medium, and 8 mm of thickness produce an inside diameter distortion value of 0.143 mm and a gap width distortion of 0.426 mm. The results of this experiment will be used as the main comparison parameter.
3.3. AISI 4340 experiment result and analysis
AISI 4340 material experiment with the Response Surface Method experimental design produce data value inside diameter and gap width distortion, as shown in Table below.
Table 9. AISI 4340 dimensional measurement results test
Furthermore, the experimental results are processed to obtain a quadratic equation, as shown in the following formula. EquationEquation 33
3 shows the model for inside diameter distortion (ID), while EquationEquation 4
4
4 shows the gap width (GW) distortion model.
The quadratic model in EquationEquation 33
3 for inside diameter distortion has an R2 value of 0.9244 and an Adjusted R2 of 0.7883. These results indicate that the independent variables in a formed quadratic model can explain 92.44% of dependent variable diversity.
ANOVA testing was carried out on experimental results with Response Surface Method analysis. The results of ANOVA analysis for experimental inside diameter distortion value produce data as shown in Table .
Table 10. Analysis of Variance for inside diameter distortion AISI 4340
ANOVA results in Table show that the contribution for each hardening factor to the inside diameter distortion value achieved is: austenite temperature (A) 3.16% and 7.37%, quenching medium (B) 22.11% and 2.11%, thickness (C) 34.74% and 1.05%. The significant contribution value and strong p-value indicate that quench medium and material thickness are the factors that significantly influence the inside diameter distortion value. This result aligns with the main effects and interaction plots graph for inside-diameter distortion in Figure .
Figure shows that only the medium quench and thickness factors significantly affect the distortion response inside the diameter in AISI 4340. Minimal austenite temperature provides the least distortion response. The slower cooling rate of the medium quench will provide a minimal distortion response. Similarly, the material thickness factor, the thicker the hardened material, the less distortion response will be. Figure shows the interaction of each factor level and the impact of distortion inside the diameter. Like the previous one, the evaluation was carried out in Figure , row 3 with a red line. In line with the main plot effects graph, the minimal austenite temperature and the slowest medium quench provide minimal distortion.
EquationEquation 44
4 shows the gap width (GW) distortion model of pada material AISI 4340.
The quadratic model in EquationEquation 44
4 gap width distortion has an R2 value of 0.9275 and an Adjusted R2 of 0.7971. These results indicate that the independent variables in the formed quadratic model can explain 92.75% of dependent variable diversity. The results of ANOVA analysis for experimental inside diameter distortion value produce data as shown in Table below.
Table 11. Analysis of Variance for gap width distortion AISI 4340
ANOVA results on Table show that the contribution for each hardening factor to gap width distortion value achieved are: austenite temperature (A) 9.07% and 9.07%, quench medium (B) 11.20% and 2.32%, thickness (C) 43.44% and 2.51%. The significant contribution value and strong p-value indicate that material thickness is the most substantial factor significantly influencing gap width distortion value. However, the austenite temperature and quench medium also have an effect of up to 31.68% on the gap width distortion value. The ANOVA results are reinforced by main effects and interaction plots for gap width distortion in Figure below.
Figure shows that only the thickness factor significantly affects the gap width response in AISI 4340 material. At response gap width, the lowest austenite temperature gives the smallest distortion response. The slowest cooling rate of the medium quench and thicker material provides the least distortion response. Figure displays the interaction of each factor level and the impact of distortions. Just like before, the evaluation was done on Figure row 3 with a red line referring to a thickness of 8 mm. The minimal austenite temperature and the slowest medium quench provide minimal gap width distortion in line with the main plot effects graph.
3.4. AISI 4340 multiple-responses optimization of distortion
The AISI 4340 optimization method used in this section is the same as the distortion optimization process for AISI 4140. It means that the distortion responses value was selected by smaller-the-better quality characteristics. The multiple-responses optimization results are the three best hardening process parameters based on the type of quench medium used. The best hardening parameter is selected based on the highest desirability value. Table below compares the best hardening parameters based on the type of quench medium.
Table 12. Optimum comparison of hardening parameter for AISI 4340
Response surface graph of hardening parameter A2.88-B3-C2 for AISI 4140 inside diameter and gap width distortion are shown in Figures below.
The response surface graphs for austenite temperature in Figures show behavioral similarities with the main effects plot graphs in Figure and Figure . In AISI 4340, Austenite temperature does not significantly affect distortion. However, changes in the quench medium provide similar behavior. The risk of distortion decreases with a decrease in the cooling rate of the material. Unlike before, multiple-response optimization in AISI 4340 shows that the thickness factor has a significant effect. The thicker the material will reduce the risk of material distortion.
The best hardening parameter which gives lower for AISI 4340 steel, is A3-B3-C2, with a 900°C austenite temperature, oil quenches medium, and 8 mm of thickness. This hardening parameter is predicted to produce the minimum distortion value, namely 0.054 mm inside diameter distortion and 0.057 mm gap width distortion. This hardening parameter has the highest desirability with a value of 0.801.
3.5. Distortion comparison
Experiments Analysis results and dimensional change predictions for AISI 4140 and AISI 4340 are compared in Table below. Comparison tables are made to reach the parameters process that can provide the minimum Navy C-ring distortion. This section also compares the distortion values between the actual hardening parameters that are currently used and the new hardening parameters.
Table 13. Comparison table of hardening parameter and distortion result
The results of multiple-responses optimization show that medium austenite temperature will produce a large distortion. The oil cooling medium has the slowest cooling rate. Slower cooling is proven to reduce the level of distortion that occurs (Hasan et al., Citation2018). This is in line with the research of Passanha et al. (Citation2022), which states that mineral oil provides slower cooling and produces less internal stress. AISI 4140 and AISI 4340 materials show identical results that high austenite temperatures with slower cooling produce lower actual distortion values.
In AISI 4140 material, the hardening process with new parameters produces an actual distortion value lower than the estimate. AISI 4140 material is superior, with more minor inside diameter distortion, but the gap width distortion is more extensive than AISI 4340. The hardening process with new parameters on AISI 4340 produces a higher actual inside diameter distortion value than predicted. However, AISI 4340 material has the advantage of a minimal actual gap width distortion.
4. Conclusion
Research experiments using the Response Surface Method design and Navy C-ring specimens have revealed the effect of hardening process parameters on the distortion of AISI 4140 and AISI 4340 steel materials. This research produces outputs that solve the problem of post-hardening distortion in the steel manufacturing industry.
In AISI 4140, all austenite temperature factors, quench medium, and material thickness contribute equally to inside diameter and gap width distortion. In AISI 4340, only the material thickness factor significantly contributes to inside diameter and gap width distortion. However, the main effects plot graph shows nearly the same hardening process behavior for the two materials. Using very low or very high austenite temperatures will result in minimal inside diameter and gap width distortion. The slower the cooling rate by the quench medium, the less distortion will occur for both materials. The same goes for the thickness factor. AISI 4140 and AISI 4340 materials give a similar response; the thicker the material is in the hardening process, the less risk of distortion.
Multiple-responses optimization for inside diameter and gap width significantly affects material distortion, resulting in the best hardening process parameters. The optimal hardening parameters for AISI 4140 are austenite temperature 895.2°C with medium oil quench. The parameter can guide inside diameter distortion production of 0.025 mm (a decrease of up to 82.51% compared to current distortion) and a gap width distortion of 0.093 mm (a reduction of up to 78.16% compared to current distortion). On the other hand, AISI 4340 optimal hardening parameters are the temperature of 900°C with medium oil quench. The parameter can guide inside diameter distortion production of 0.054 mm (a decrease of up to 62.23% compared to the current distortion) and a distortion of a gap width of 0.057 mm (a reduction of up to 86.61% compared to the current distortion).
In multiple-responses optimization, austenite temperature significantly affects material distortion, according to the research of Northwood et al (Northwood et al., Citation2007);. Farivar et al (Farivar et al., Citation2018);. Solic et al (Solic et al., Citation2018). Quench oil medium is a cooling medium that provides minimal distortion values. This research follows Liscic et al (Liscic et al., Citation2010). and Li et al (Li et al., Citation2014);. Canale et al (Canale & Totten, Citation2005);. Clark et al (Clark et al., Citation2014);. Decroos et al (Decroos & Seefeldt, Citation2017);. Lopez-Garcia et al (Lopez-Garcia et al., Citation2022);. Nunes et al (Nunes et al., Citation2018);. Hasan et al. research; which states that oil cooling medium has a low cooling rate and effectively reduces the risk of distortion.
Research shows that AISI 4340 can substitute AISI 4140 if the user wants a smaller gap width distortion value, but this does not apply to inside diameter distortion. Substitution of AISI 4140 material with AISI 4340 can be done, considering that the gap width distortion will decrease to 38.71% but the inside diameter distortion will increase to 53.70%.
The research results are expected to be used by design engineers to determine a design, size, or dimension that minimizes distortion risk. It can help heat treatment operators to determine hardening process parameters that minimize the distortion of SC108 components. Design or material engineers may also consider substituting AISI 4140 materials to minimize gap width distortion.
Study results showed that temperature changes in the components affected material distortion. In actual industry, the hardening process always ends with the tempering process. Heating tempering leads to changes in the temperature of the material. Ray et al. (Citation2020) stated that distortion occurs during the hardening and tempering processes. In future studies, it is possible to include temperature factors to determine the impact of changes in temperature on the risk of component distortion.
Acknowledgments
The authors are very grateful to PT. ATMI Solo - Heat Treatment Division and Politeknik ATMI Surakarta for the use of its facilities and laboratories.
Disclosure statement
No potential conflict of interest was reported by the author(s).
References
- Anggoro, P. W., Bawono, B., Jamari, J., Tauviqirrahman, M., & Bayuseno, A. P. (2021, March). Advanced design and manufacturing of custom orthotics insoles based on hybrid Taguchi-response surface method. Heliyon, 7(3), e06481. https://doi.org/10.1016/j.heliyon.2021.e06481
- Anggoro, P. W., Bawono, B., Setyohadi, D. B., Ratnasari, L., Fergiawan, P. K., Tauviqirrahman, M., Jamari, A. P., & Bayuseno, J. (2023, June). Optimisation of the machining time required by insole orthotic shoes for patients with clubfoot using the Taguchi and respon surface methodology approach. Heliyon, 9(6), e16860. https://doi.org/10.1016/j.heliyon.2023.e16860
- Anggoro, P. W., Bawono, B., Tauviqirrahman, M., Bayuseno, A. P., & Jamari, J. (2019, August). Design and manufacturing orthotics shoe insole with optimum surface roughness using the CNC milling. Journal of Engineering Science & Technology, 14(4), 1799–22. Corpus ID: 208876928.
- Anggoro, P. W., Purharyono, Y., Anthony, A. A., Tauviqirrahman, M., Bayuseno, A. P., & Jamari, J. (2022, May). Optimisation of cutting parameters of new material orthotic insole using a Taguchi and response surface methodology approach. Alexandria Engineering Journal, 61(5), 3613–3632. https://doi.org/10.1016/j.aej.2021.08.083
- Bhagyalaxmi, Hegde, A., Sharma, S., & Jayashree, P. K. (2023, May). Analysis of tempering temperature and vegetable oil quenchant viscosity effect on mechanical properties of 42CrMo4 steel. Cogent Engineering, 10(1). https://doi.org/10.1080/23311916.2023.2216052
- Bhagyalaxmi, B. E. A., Sharma, S., & Kini, V. (2018, December). Effect of heat treatment and mechanical characterization of AISI 4140 steel. International Journal of Mechanical and Production Engineering Research and Development, 8(6), 603–610. https://doi.org/10.24247/IJMPERDDEC201863
- Bilal, M. M., Yaqoob, K., Zahid, M. H., Haq, E. U., Tanveer, W. H., Wadood, A., & Ahmed, B. (2019, November-December). Effect of austempering conditions on the microstructure and mechanical properties of AISI 4340 and AISI 4140 steels. Journal of Materials Research and Technology, 8(6), 5194. https://doi.org/10.1016/j.jmrt.2019.08.042
- Canale, L. D. C. F., & Totten, G. E. (2005, January). Overview of distortion and residual stress due to quench processing part I: Factors affecting quench distortion. International Journal of Materials and Product Technology, 24(1/2/3/4), 4. https://doi.org/10.1504/IJMPT.2005.007941
- Clark, A., Bowers, R. J., & Northwood, D. O. (2014, May). Heat treatment effects on distortion, residual stress, and retained austenite in carburized 4320 steel. Materials Science Forum, 783-786, 692–697. https://doi.org/10.4028/www.scientific.net/MSF.783-786.692
- Decroos, K., & Seefeldt, M. (2017, December). The effect of size on the distortion behavior after carburisation and quenching processes of gears. International Journal of Metallurgical & Materials Engineering, 3(2), 139. https://doi.org/10.15344/2455-2372/2017/139
- Djimtoingar, S. S., Derkyi, N. S. A., Kuranchie, F. A., & Yankyera, J. K. (2022, November). A review of response surface methodology for biogas process optimization. Cogent Engineering, 9(1). https://doi.org/10.1080/23311916.2022.2115283
- Fakir, R., Barka, N., Brousseau, J., & Caron-Guillemette, G. (2020, July). Analysis of the mechanical behavior of AISI 4340 steel cylindrical specimens heat treated with fiber laser. Journal of Manufacturing Processes, 55, 41–56. https://doi.org/10.1016/j.jmapro.2020.03.039
- Farivar, H., Prahl, U., Hans, M., & Bleck, W. (2018, August). Microstructural adjustment of carburized steel components towards reducing the quenching-induced distortion. Journal of Materials Processing Technology, 264, 313–327. https://doi.org/10.1016/j.jmatprotec.2018.08.040
- Gurumurthy, B. M., Kini, A. U., Sharma, S., Hiremath, P., Gowrishankar, M. C., & Jones, I. P. (2021, September). Effect of intercritical processing temperature on mechanical properties, microstructure and microhardness of ferrite-bainite medium carbon dual phase Steels. Cogent Engineering, 8(1), 1999062. https://doi.org/10.1080/23311916.2021.1999062
- Guterres, N. F. D. S., Rusnaldy, R., & Widodo, A. (2017, January). Gear distortion analysis due to heat-treatment process. AIP Conference Proceedings, 1788(1), 030038. https://doi.org/10.1063/1.4968291
- Hardin, R. A., & Beckermann, C. (2005). Simulation of heat treatment distortion. Proceeding of the 59th SFSA Technical and Operating Conference, Chicago (p. 3.3).
- Hasan, H. S., Khaleefah, R. H., Al Haboubi, N. A., & Salman, R. D. (2018, December). Effect of agitation, temperature, and quenching medium on cooling curve and cooling rate for steels. Al-Nahrain Journal for Engineering Sciences, 21(4), 473–478. https://doi.org/10.29194/NJES.21040473
- Hazir, E., & Ozcan, T. (2018, September). Response surface methodology integrated with desirability function and genetic algorithm approach for the optimization of CNC machining parameters. Arabian Journal for Science and Engineering, 44(3), 2795–2809. https://doi.org/10.1007/s13369-018-3559-6
- Ismartaya, K., Karyadi, G. B., Bawono, B., & Anggoro, P. W. (2022). Optimalisasi Ketangguhan dan Kekerasan Baja AISI 4340 pada Shank Holder Dovetail Cutter paska Proses Hardening dengan Minimasi Biaya Proses. Jurnal Rekayasa Mesin. December, 17(3), 473–484. https://doi.org/10.32497/jrm.v17i3.3982
- Li, Z., Ferguson, B. L., Nemkov, V., Goldstein, R., Jackowski, J., & Fett, G. (2014, December). Effect of quenching rate on distortion and residual stresses during induction hardening of a full-float truck axle shaft. Journal of Materials Engineering and Performance, 23(12), 4170–4180. https://doi.org/10.1007/s11665-014-1196-0
- Li, Z., Grandhi, R. V., & Srinivasan, R. (2006, February). Distortion minimization during gas quenching process. Journal of Materials Processing Technology, 172(2), 249–257. https://doi.org/10.1016/j.jmatprotec.2005.10.018
- Liscic, B., Tensi, H. M., Canale, L. C. F., Totten, G. E., Liscic, B., Tensi, H. M., Canale, L. C. F., & Totten, G. E. (2010). Quenching theory, and technology (2nd ed.). CRC Press, Taylor & Francis Group. https://doi.org/10.1201/9781420009163
- Liu, Y., Qin, S., Hao, Q., Chen, N., Zuo, X., & Rong, Y. (2017, January). Finite element simulation and experimental verification of internal stress of quenched AISI 4140 cylinders. Metallurgical and Materials Transactions A, 48(3). https://doi.org/10.1007/s11661-016-3916-6
- Lopez-Garcia, R. D., Garcia-Pastor, F. A., Castro-Roman, M. J., Alfaro-Lopez, E., & Acosta-Gonzalez, F. A. (2016, February). Effect of immersion routes on the quenching distortion of a long steel component using a finite element model. Transactions of the Indian Institute of Metals, 69(9), 1645–1656. https://doi.org/10.1007/s12666-015-0738-y
- Lopez-Garcia, R. D., Medina-Juarez, I., & Maldonado-Reyes, A. (2022, April). Effect of quenching parameters on distortion phenomena in AISI 4340 steel. Metals - Open Access Metallurgy Journal, 12(5), 759. https://doi.org/10.3390/met12050759
- Mahmood, N. J., Hussein, A. A., Hasan, A. S., & Ali, O. M., Effect of AISI 4140 carbon steel heat treatments on specified mechanical properties. 4th International Conference on Materials Engineering & Science, AIP Conference Proceedings. November. 2022; 2660: pp. 020065-1-0120065–8. https://doi.org/10.1063/5.0107707.
- Mehrabi, A., Sharifi, H., Asadabad, M. A., Najafabadi, R. A., & Rajaee, A. (2020, August). Improvement of AISI 4340 steel properties by intermediate quenching – microstructure, mechanical properties, and fractography. International Journal of Materials Research (Formerly Zeitschrift Fuer Metallkunde), 111(9), 711–779. https://doi.org/10.3139/146.111939
- Nallathambi, A. K., Kaymak, Y., Specht, E., & Bertram, A. (2010, January). Sensitivity of material properties on distortion and residual stresses during metal quenching processes. Journal of Materials Processing Technology, 210(2), 204–211. https://doi.org/10.1016/j.jmatprotec.2009.09.001
- Nallathambi, A. K., Specht, Y., Kaymak, E., Bertram, A., & Bertram, A. (2009, April). Optimum strategies to reduce residual stresses and distortion during the metal quenching process. Journal of ASTM International, 6(4), 101806. https://doi.org/10.1520/JAI101806
- Nan, C., Northwood, D. O., Bowers, R. J., Sun, X., & Bauerle, P. (2009, May). The use of Navy C-ring specimens to study distortion in ferritic nitrocarburized 1010 steel. WIT Transactions on Engineering Sciences, 62, 1743. https://doi.org/10.2495/SECM090021
- Northwood, D. O., He, L., Boyle, E., & Bowers, R. (2007, March). Retained austenite – residual stress – distortion relationships in carburized SAE 8620 steel. Materials Science Forum, 539-543, 539–543. https://doi.org/10.4028/www.scientific.net/MSF.539-543.4464
- Nunes, M. M., Silva, E. M. D., Renzetti, R. A., & Brito, T. G. (2018, October). Analysis of quenching parameters in AISI 4340 steel by using design of experiments. Materials Research, 22(1), e20180315. https://doi.org/10.1590/1980-5373-MR-2018-0315
- Passanha, J., Sharma, S., Hegde, A., Lakshmi, B., Shankar, G., & Murthy, G. (2022, November). Experimental and statistical analysis of vegetable oil-brine egg yolk emulsion quench on the properties of AISI 4140 steel. Cogent Engineering, 9(1). https://doi.org/10.1080/23311916.2022.2146626
- Rajan, T. V., Sharma, C. P., & Sharma, A. (2011). Heat treatment: principles and techniques (2nd ed.). PHI Learning Private Limited.
- Ray, B. C., Prusty, R. K., & Nayak, D. (2020). Phase transformations and heat treatments of steels (1st ed.). CRC Press, Taylor & Francis Group. https://doi.org/10.1201/9780429019210-1
- Safi, S. M., & Givi, M. K. B. (2014, February). A new step heat treatment for steel AISI 4340. Metal Science and Heat Treatment, 56(1), 19–22. https://doi.org/10.1007/s11041-014-9707-z
- Samuel, A., & Prabhu, K. N. (2022, March). Residual stress, and distortion during quench hardening of steels: A review. Journal of Materials Engineering and Performance, 31(7), 5161–5188. https://doi.org/10.1007/s11665-022-06667-x
- Sari, N. K., Purbasari, I. Y., Anggoro, P. W., Jamari, J., Bayuseno, A. P., & Arellano-Garcia, H. (2022). Reuse of wheat flour liquid waste for enzymatic hydrolysis to yield glucose-derived biotanol. Cogent Engineering, 9(1). https://doi.org/10.1080/23311916.2022.2101229
- Silva, A. D. D., Pedrosa, T. A., Gonzalez-Mendez, J. L., Jiang, X., Cetlin, P. R., & Altan, T. (2012). Distortion in quenching an AISI 4140 C-ring – Predictions and experiments. Materials and Design, 42, 55–61. https://doi.org/10.1016/j.matdes.2012.05.031
- Solic, S., Podgornik, B., & Leskovsek, V. (2018, October). The occurrence of quenching cracks in high-carbon tool steel depending on the austenitizing temperature. Engineering Failure Analysis, 92, 140–148. https://doi.org/10.1016/j.engfailanal.2018.05.008
- Sonar, T., Lomte, S., Gogte, C., & Balasubramanian, V. (2018). Minimization of distortion in heat treated AISI D2 tool steel: Mechanism and distortion analysis. Procedia Manufacturing, 20, 113–118. https://doi.org/10.1016/j.promfg.2018.02.016
- Totten, G., Howes, M., & Inoue, T. (2002). Handbook of residual stress and deformation of steel. ASM International.
- Watanabe, K., Yamada, M., Nakasaki, M., Matsumoto, R., & Utsunomiya, H. (2020). Determination of transformation plasticity coefficient of steel by horizontal quenching of shaft. Procedia Manufacturing, 50, 498–502. https://doi.org/10.1016/j.promfg.2020.08.090
- Zhang, G., He, X., Zhang, Q., Wang, W., & Wang, M. (2021, January). Comparison of microstructure and heat treatment distortion of gear steels with and without Nb addition. Journal of Iron and Steel Research International, 28(12), 488–495. https://doi.org/10.1007/s42243-020-00521-x
- Zheng, L., Zheng, D., Zhao, L., Wang, L., & Zhang, K. (2013, May). Novel water-air circulation quenching process for AISI 4140 steel. Metals and Materials International, 19(6), 1373–1376. https://doi.org/10.1007/s12540-013-6034-7
- Zhu, Z., Jin, Z., Wu, D., Wang, X. A., Yu, Y., Guo, X., & Wang, X. (. (2022, July). Assessment of surface roughness in milling of beech using a response surface methodology and an adaptive network-based fuzzy inference system. Machines, 10(7), 567. https://doi.org/10.3390/machines10070567