Abstract
Objectives: This study aimed to evaluate the subjective acceptability and physiological response of an inflatable vest as a wearable device to stimulate calming effects. Methods: A Visual Analogue Scale (VAS) was used as a subjective measurement and an electroencephalogram (EEG) as an objective/physiological measurement. Results: The results showed that the subjective scores of 30 male participants rated the prototype as an ‘excellent’ device, with an average score of 81. An increase in the theta (4–8 Hz) frequency band was the most noticeable change in EEG, which represented the calming effect behavior experienced in 64% of all participants. Conclusion: The self-regulated inflatable vest could be beneficial for therapeutic purposes by inducing a calming effect.
SUBJECTS:
1. Introduction
For many centuries, touch therapy has evolved into various models and styles. Many individuals practice this therapy, believing it to reduce muscle pain, relieve stress, and provide relaxation (Field, Citation2000). It is important to note that in the modern era, the popularity of healthcare alone is insufficient to justify its sustainable acceptance. Therefore, there is a need for scientific evidence (evidence-based) to support the efficacy of this therapy.
Touch therapy has also been used to relieve stress and anxiety, specifically for people with autism spectrum disorder (ASD) (Ayres, Citation1972). Some ASD children showed tremendous progress with this treatment, while others showed small or no improvement. In this case, encouragement is commonly emphasized when the therapy causes the smallest changes in the structure of the brains of these children. This is because drug treatments and behavioral therapies only provide slight help and control patients’ external attitudes without changing the mental conditions (Ayres, Citation1972). The therapy aforementioned is often operated using the somatosensory system, which is associated with tactile processing and proprioceptive sensations (Ross et al., Citation2019). Occupational therapists often use deep pressure therapy, a sensory integration technique that applies mechanical tactile force to the body through mechanoreceptors, initiating tactile neural transmission. This approach is widely used when working with ASD children (Bestbier & Williams, Citation2017). In addition, deep pressure also provides a calming effect by increasing serotonin and dopamine neurotransmitter levels (Taylor et al., Citation2017).
The therapeutic model for ASD, based on sensory integration, offers benefits in addressing the limitations of other systems such as behavioral therapy. This approach is cost-effective and easy to implement (Matson, Citation2017; McDougle, Citation2016). It also supports the development of medical devices in developing countries, specifically Indonesia, where the procurement cost of such systems poses a challenge in meeting market demands. However, the launch of deep pressure tools in the market has been hindered by reports stating that they are unwearable and lack intuitive design, such as Squeeze Machine (Grandin, Citation1984), Hug’m Apparatus (Krauss, Citation1987), Autism Hug Machine Portable Seat (AHMPS) (Afif et al., Citation2021), and Weighted Blanket (Champagne et al., Citation2015). Several wearable deep-pressure tools have also made progress in terms of their ease of use, such as Compression Garments (Duvall et al., Citation2019), T-Jacket (Poon et al., Citation2014), and Weighted Vests (Taylor et al., Citation2017). Studies examining the effects of deep pressure therapy yielded varied results. Some studies reported positive effects (Chen et al., Citation2016, Citation2019; Edelson et al., Citation1999; Grandin, Citation1992; Poon et al., Citation2014; Vandenberg, Citation2001; Zissermann, Citation1992), no significant effect (Carter, Citation2005; Hodgetts et al., Citation2011b; Krauss, Citation1987; Reichow et al., Citation2010; Watkins & Sparling, Citation2014), and many having unclear results (Bagatell et al., Citation2010; Fertel-Daly et al., Citation2001; Hodgetts et al., Citation2011a; Losinski et al., Citation2017). Most of the studies only focused on either physiological or psychological aspects, despite both being equally crucial for assessing comfort and calmness. It was not until studies by Edelson et al. (Edelson et al., Citation1999) using GSR and CPRS, and Hodgetts et al. (Citation2011b) using HR and behavioral observation, evaluated the effects on both aspects. Edelson reported a positive effect of Grandin’s squeeze machine, while Hodgetts evaluated the weighted vest that reported the opposite.
These inconsistencies highlight the importance of various factors. According to Duvall et al. (Duvall et al., Citation2019), the absence of standardized protocols, including consistent pressure loads and intervention durations, contributed to the inconsistency in the results. A study conducted by Edelson using Grandin’s squeeze machine demonstrated a positive therapeutic effect by allowing users to apply pressure according to their preference. In contrast, Hodgetts’ study involving a wearable vest, with the weight determined by the therapist (5–10% of body weight), did not show a therapeutic effect. Despite this discrepancy, Taj-Eldin et al. (Citation2018) argued that wearable vests offered advantages in terms of convenience and compactness. These findings lead to some questions: Can wearable vests still have a positive therapeutic effect when the pressure applied is self-determined? Therefore, the major contributions of this study are:
Examining the effects of self-regulating pressure load through an inflatable vest on subjective comfort and physiological responses.
Investigating the therapeutic potential of inflatable vests as deep pressure tools by evaluating the impact of self-determined pressure.
Contributing to a better understanding of the factors influencing the therapeutic effect, including standardized protocols in pressure loads.
Providing insights into the potential benefits and advantages of inflatable vests as a convenient and compact alternative for deep pressure therapy.
These contributions will expand the existing knowledge base in the field, offering valuable insights to practitioners and researchers studying deep-pressure interventions and the therapeutic applications.
2. Materials and methods
2.1. Deep pressure tools
2.1.1. Inflatable vest
This study used an inflatable vest as a wearable therapeutic device designed to provide deep pressure to the users’ body. The vest allowed for pressure adjustment without the need for removal, making it portable and versatile. Taj-Eldin et al. (Citation2018), highlighted a vest as a tool capable of meeting these criteria. The deep pressure exerted by the vest could be generated in various patterns, including weighted, inflated, and stretched. Among these patterns, the inflatable model had been reported to offer a better therapeutic effect compared to other models (Afif, Farkhan, et al., Citation2022; Afif, Manik, et al., Citation2022; Maula et al., Citation2021). In this study, the Squease™ vest (London, UK) was used and customized by integrating an electric pump and incorporating a cotton outer fabric to achieve a higher level of pressure adjustability and enhance the tactile experience. shows the inflatable vest used.
2.1.2. Pressure data collection
The prototype vest was equipped with Force Sensing Resistor (FSR) sensors to measure the pressure load exerted on the body, according to the participant’s requests in the test (Duvall et al., Citation2019). Four Interlink406® (Camarillo, CA) FSR sensors were positioned at two points on the waist and two on the back. These points had been shown to induce a calming effect and anatomically target areas with greater pressure sensitivity (Maula et al., Citation2021; Morrison et al., Citation2015). further illustrates the placement of the FSR sensors.
2.2. Measurements tool
2.2.1. Subjective measurement
Visual analogue scale (VAS) is straight-line psychometric response scale used to measure subjective characteristics and attitudes toward inflatable vests. This is a valuable instrument for therapy efficacy documentation (Klimek et al., Citation2017). In this study, the digital version of VAS was used instead of the paper type, corresponding with a study elaborating on the advantages of increasing reliability in data collection (Whybrow et al., Citation2006). The participants were asked to rate their satisfaction with the deep pressure provided by the inflatable vest device. In this case, the rating response ranged from highly dissatisfied to highly satisfied at the left and right ends of the VAS line. The straight-line VAS represented the value interval of 0–100 and was converted to a five-grade design (Poor-Fair-Good-Very good-Excellent) (Biswas et al., Citation2018). This showed that the values 0–20, 21–40, 41–60, 61–80, and 81–100 were ‘Poor’, ‘Fair’, ‘Good’, ‘Very good’, and ‘Excellent’, respectively.
2.2.2. Physiological measurement
In this study, electroencephalogram (EEG) physiological signals were measured. EEG is an essential tool for examining the brain’s electrical activity generated directly by the central nervous system (Rayi & Murr, Citation2021; Yu & Wang, Citation2022). It has been widely reported that EEG is related to changes in human emotions, making it commonly used for measuring stress (Arsalan & Majid, Citation2021), mental workload (Hussain et al., Citation2021), sleep stages (Hussain et al., Citation2022), and other health problems (Hussain & Park, Citation2020, Citation2021; Islam et al., Citation2022).
In this study, the Muse headband (Interaxon Inc.) was used for EEG measurement because it was flexible, portable, and widely adopted for meditation and investigation (Arsalan & Majid, Citation2021). This device consisted of four electrodes: AF7, AF8, TP9, and TP10, due to a 10–20 system of electrode placement, as illustrated in .
To acquire data from the Muse headband, an android application from https://mind-monitor.com was used and the data were obtained through a Bluetooth connection. The Muse headband incorporated a noise cancellation system that differentiated the acquired EEG signals into clean or noisy signals based on their statistical properties, including variance, amplitude, and kurtosis values. The classification algorithm responsible for this, known as ‘Muse Elements’, was embedded within the headband (Arsalan & Majid, Citation2021). To mitigate powerline artifacts, the Mind Monitor application was set to a 50 Hz notch frequency filter. The recorded raw data were transformed into five frequency absolute band powers: delta (0–4 Hz), theta (4–8 Hz), alpha (8–13 Hz), beta (13–30 Hz), and gamma (30–44 Hz). This transformation was achieved using the Fast Fourier Transform (FFT), which computed the power spectral density of each frequency on each channel. The average absolute band power measured during the 1.5-min test served as a reference value for this pretest-posttest comparison study. Additionally, potential sources of noise, such as eye blinking and jaw clenching, were identified and indicated in the Mind Monitor’s absolute band power data streamer. These identified noise sources were subsequently removed as artifacts from the data.
Each frequency band held its meaning for stress markers and calm or relaxed markers. Delta was activated during sleep and calm state (Phutela et al., Citation2022). Theta activity, reflecting the limbic and hippocampal regions, decreased under acute stress (Gärtner et al., Citation2014; Phutela et al., Citation2022). Alpha increased with calming because it was related to relaxation (Barkana et al., Citation2022; Ehrhardt et al., Citation2022), while beta activity decreased with anxiety (Ehrhardt et al., Citation2022; Phutela et al., Citation2022). Lastly, gamma activity increased among patients with depression (Ehrhardt et al., Citation2022).
2.3. Participants
The participants involved were voluntary males between the ages of 19 and 25 from Universitas Diponegoro and Politeknik Negeri Semarang, whose health condition did not have any indications of ASD (Biswas et al., Citation2018; Krauss, Citation1987). This study adhered to the guidelines of the Declaration of Helsinki and was approved by the Health Research Ethics Committee Faculty of Medicine, Universitas Diponegoro, number 373/EC/KEPK/FK-UNDIP/IX/2021. Subsequently, each participant was required to give their informed consent before participating in the experiment.
2.4. Procedures
Each participant completed an online questionnaire, providing information such as names, ages, heights, weights, and clothing sizes. They were also given explanations about the operational patterns of the prototype. The prototype was attached to their bodies through the adjustments of the Velcro on the right and left sides of the tool.
Before using the inflatable vest, participants were instructed to sit comfortably and undergo a 1.5-min baseline EEG measurement to establish a reference condition. Furthermore, participants were given enough time to familiarize themselves with the vest and adjust the pressure using the control button during the pre-experimental phase. They inflated the vest to the desired pressure for 1.5 min, while EEG measurements were recorded (Foo et al., Citation2018). In the final phase, the prototype was removed, and participants were asked to complete the VAS. The sequential procedures of this study were depicted in , and the real experimental scenario was illustrated in .
2.5. Statistical analysis
For statistical analysis, descriptive statistics were used to analyze the subjective ratings of the inflatable deep pressure vest (Chen et al., Citation2019). Due to the small sample size, a normality test using the Shapiro-Wilk test was also conducted (n < 50) (Mishra et al., Citation2019). Additionally, data with a P-value > 0.05 were considered to follow a normal distribution, and the distribution was visually described using scatter diagrams. These tools provided information about the similarity or differences in preferred values reported by participants. The average absolute band power data were analyzed for the different values before and during the intervention. Paired sample t-tests were used for normally distributed data, while the Wilcoxon test was used for non-normally distributed data. The statistical analysis was performed using IBM SPSS 25 software.
3. Results
3.1. Visual Analogue Scale (VAS)
In this study, a total of 30 male adolescents participated, with the mean age, height, and weight being 21.2 ± 1.47 years (p = 0.11), 167.5 ± 5.73 cm (p = 0.20), and 58.4 ± 8.45 kg (p = 0.20), respectively. The time taken to understand the prototype’s operational patterns was not normally distributed (p = 0.00), with a median value of 42 s (interquartile range 13–173 s). This short period confirmed that typical adolescents found the prototype easy to operate. The average VAS value was 81.0, indicating the ‘Excellent’ category rated by 16 participants (53.3%). Additionally, 13 participants (43.3%) rated it as ‘Very good’, and 1 participant (3.3%) rated it as ‘Good’. None of participants selected the ‘Poor’ or ‘Fair’ categories. The distribution of VAS categories is illustrated in .
On average, the pressure selected by participants on the waist was smaller than on the back, with an average of 0.77 ± 0.472 kPa and 1.49 ± 0.560 kPa, respectively. This showed that the smallest and largest pressured applied were 0.09 kPa and 3.51 kPa, respectively. Subsequently, all pressure data were normally distributed with a p-value > 0.05. All the mean load data (front waist and back) were shown in , where the parameters were widely distributed despite being categorized as ‘Very Good’ and ‘Excellent’.
3.2. Electroencephalogram (EEG)
Out of the 30 participants, 5 refused to wear the EEG headband and were excluded from the EEG analysis. The absolute band power changes of 25 participants at each frequency are presented in full in . Subsequently, the changes in absolute band powers were identified before and during the intervention that occur whether they increase or decrease by looking at the positive or negative values.
Table 1. The changes of absolute band powers before and during the intervention.
illustrates the percentage of participants who experienced an increase in EEG. Delta, theta, and alpha are colored blue, representing support for the calm state, while beta and gamma are colored red, representing the opposite. Among these bands, theta (dark blue) exhibited the most significant increase, with 64% of participants experiencing heightened activity. Increases in delta and alpha (light blue) were observed in 60% of participants, indicating improved sleep quality and relaxation/meditation, respectively. On the other hand, beta and gamma increases (light red) were found in 58% of participants that represent anxiety and depression.
Figure 8. The percentage of increased absolute band powers in each frequency band from baseline to wearing vest.
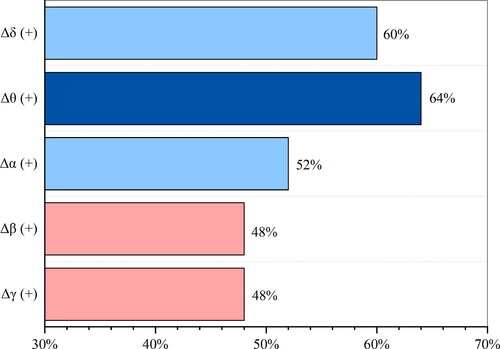
To further analyze the data for each frequency band, significant changes were calculated. shows the results of a comparison test, which concluded that both increasing and decreasing values across all frequency bands did not exhibit significant changes.
Table 2. Comparison test in the changes of absolute band powers.
4. Discussion
This study focused on modifying an adjustable deep-pressure inflatable vest and examining its comfortability and therapeutic effects. The evaluation primarily revolved around subjectively and objectively assessing the self-regulated inflatable pressure. This study incorporates the VAS assessment to capture the subjective perception of comfort, complemented by EEG measurements to quantify the users’ physiological response. This particular aspect, combining subjective comfort assessment with objective physiological measurements in the use of an inflatable vest, has not been previously investigated, as outlined in .
Table 3. The comparison of the previous with this present study.
In terms of subjective evaluations, nearly all participants rated the inflatable vest as ‘Excellent’, aligning with previous studies that evaluated therapeutic tools such as assistive sleeping bags and shape memory alloy (SMA) compression garments (Biswas et al., Citation2018; Foo et al., Citation2018). However, inflatable vests offered simpler and more flexibility than sleeping bags, which possess larger dimensions and were only used when resting. Additionally, when compared to SMA compression garments, inflatable vests also provided portable and ease-of-use advantages without repeated removal and wearability. This is due to the absence of irritative heat generation, as seen with the metal alloy springs present in SMA compression garments (Holschuh & Newman, Citation2014).
From a physiological standpoint, the deep pressure vest was found to impact brain waves, as depicted in . The dark blue color representing the theta band indicates its close association with calming states, influenced by limbic and hippocampal activity. The light blue color of the delta band suggests its role as an indicator of sleep quality, although it is also active during calm states. Notably, 60% of participants experienced an increase in the delta band, indicating a state of calmness while wearing the inflatable vest. The relationship between alpha and beta bands as stress markers is characterized by decreased alpha bands and increased beta bands (Barkana et al., Citation2022; Ehrhardt et al., Citation2022). The results are in line with this statement, the increase in alpha was experienced by more participants, and vice versa in the beta band. The increase in the gamma band, which is colored red is less of the total participants showed that indications of depression decreased more dominantly in participants when wearing inflatable vests.
In this study, most participants experienced an increase in theta power when wearing the inflatable vest, which aligns with the therapeutic goal of anxiety reduction. Theta (4–8 Hz) is associated with the limbic system and hippocampus (Phutela et al., Citation2022), which may increase the production of neurotransmitters similar to the effect of medication (Vandenberg, Citation2001). Several studies showed that there is an observed increase in theta power during meditation, which serves as a potential indicator of mindfulness (Cahn & Polich, Citation2006; Carpenter et al., Citation2022; Lomas et al., Citation2015). In the human brain, thetas are responsible for the release of gamma-aminobutyric acid/GABA that can induce relaxation, reduce anxiety, and alleviate stress. A different study reported that an imbalance between excitatory neurons (mediated by glutamate) and inhibitory neurons (mediated by GABA) may cause autism. Subsequently, GABA levels, the principal inhibitory neuron that plays an important role in sensory integration (SI), have repeatedly been found to be lower in children with autism (Afif, Farkhan, et al., Citation2022; Horder et al., Citation2018; Rojas et al., Citation2014). Therefore, an increase in theta band will be very useful in providing sensory therapy effects to ASD children.
Participants were free to control the pressure load of the inflatable vest, and their preference was compared to the subjective score. According to Duvall et al. (Citation2019), the pressure load and distribution affected the comfort. However, in this study, it was found that the amount of pressure varied greatly as recorded in with VAS scores ranging from ‘Very Good’ to ‘Excellent’. These results were in line with Reynolds et al. (Citation2015), which reported that an inflatable vest (Vayu vest) with a self-regulated pressure load (a firm hug) can reduce arousal and provide a calming effect.
This study had several limitations. Firstly, pressure sensors were only placed at a limited number of points, serving as representatives. Therefore, subsequent studies were needed to explore a wider range of pressure points, potentially using a mat sensor to determine the force distribution. Secondly, the participants involved were restricted to healthy adolescents, rather than directly applying the findings to ASD children, because they were expected to provide appropriate feedback.
5. Conclusion
The self-regulated inflatable vest is subjectively evaluated as an excellent device that physiologically helps to stimulate calming effect. It is necessary to optimize the device into a more compact and simpler device, specifically in the pressure control section. Additionally, further study is needed involving individuals with anxiety disorders, such as those with ASD, and it should also consider the duration of pressure, and evaluate its effects using additional physiological measurements, such as skin conductance, and heart rate variability.
Acknowledgments
The authors are also grateful to all participants, specifically Dr. M. Tauviqirrahman, ST., MT., and Dr.-Ing. Ir. Paryanto, ST., MT., Ilham Alkian, S.Si, M.Ling, and Hagata Aersada Ginting for the valuable suggestions in writing this article.
Disclosure statement
No potential conflict of interest was reported by the author(s).
Additional information
Funding
Notes on contributors
Mohamad Izzur Maula
M. I. Maula is a master’s student at the Department of Mechanical Engineering, Diponegoro University, Semarang. His research focuses on the biomechanics and engineering design especially deep pressure therapeutic tools.
Ilham Yustar Afif
I. Y. Afif is a lecturer at the Department of Mechanical Engineering, Universitas Muhammadiyah Semarang. His research focuses on the engineering design and biomechanics.
Muhammad Imam Ammarullah
M. D. P. Lamura is a doctoral student at the Department of Mechanical Engineering, Diponegoro University, Semarang. His research focuses on finite element analysis, tribology, and contact mechanics.
M. Danny Pratama Lamura
M. I. Ammarullah is a lecturer at the Department of Mechanical Engineering, Pasundan University, Bandung. His research interest is on the hip joint in the field of biotribology, biomechanics, and biomaterials.
Jamari Jamari
J. Jamari is a Professor in Mechanical Engineering at Diponegoro University. Research interest covers many aspects of material design, surface treatment, including wear characterization and lubrication technology.
Tri Indah Winarni
T. I. Winarni is a Professor in Faculty of Medicine Universitas Diponegoro. Her primary focus lies in autism spectrum disorders, with a special interest in fragile X syndrome and has explored various research areas, including the development of deep pressure therapy to alleviate anxiety.
References
- Afif, I. Y., Farkhan, M., Kurdi, O., Maula, M. I., Ammarullah, M. I., Setiyana, B., Jamari, J., & Winarni, T. I. (2022). Effect of short-term deep-pressure portable seat on behavioral and biological stress in children with autism spectrum disorders: A pilot study. Bioengineering, 9(2), 48. https://doi.org/10.3390/bioengineering9020048
- Afif, I. Y., Manik, A. R., Munthe, K., Maula, M. I., Ammarullah, M. I., Jamari, J., & Winarni, T. I. (2022). Physiological effect of deep pressure in reducing anxiety of children with ASD during traveling: A public transportation setting. Bioengineering, 9(4), 157. https://doi.org/10.3390/bioengineering9040157
- Afif, I. Y., Maula, M. I., Aliyafi, M. B., Aji, A. L., Winarni, T. I., & Jamari, J. (2021). Design of hug machine portable seat for autistic children in public transport application. IOP Conference Series: Materials Science and Engineering, 1096(1), 012034. https://doi.org/10.1088/1757-899X/1096/1/012034
- Arsalan, A., & Majid, M. (2021). Human stress classification during public speaking using physiological signals. Computers in Biology and Medicine, 133(April), 104377. https://doi.org/10.1016/j.compbiomed.2021.104377
- Ayres, A. J. (1972). Sensory integration and learning disorders. Western Psychological Services.
- Bagatell, N., Mirigliani, G., Patterson, C., Reyes, Y., & Test, L. (2010). Effectiveness of therapy ball chairs on classroom participation in children with autism spectrum disorders. The American Journal of Occupational Therapy: official Publication of the American Occupational Therapy Association, 64(6), 895–903. https://doi.org/10.5014/ajot.2010.09149
- Barkana, B. D., Ozkan, Y., & Badara, J. A. (2022). Analysis of working memory from EEG signals under different emotional states. Biomedical Signal Processing and Control, 71(PB), 103249. https://doi.org/10.1016/j.bspc.2021.103249
- Bestbier, L., & Williams, T. I. (2017). The immediate effects of deep pressure on young people with autism and severe intellectual difficulties: Demonstrating individual differences. Occupational Therapy International, 2017, 7534972–7534977. https://doi.org/10.1155/2017/7534972
- Biswas, T. T., Infirri, R. S., Hagman, S., & Berglin, L. (2018). An assistive sleeping bag for children with autism spectrum disorder. Fashion and Textiles, 5(18), 1–12. https://doi.org/10.1186/s40691-018-0133-5
- Cahn, B. R., & Polich, J. (2006). Meditation states and traits: EEG, ERP, and neuroimaging studies. Psychological Bulletin, 132(2), 180–211. https://doi.org/10.1037/0033-2909.132.2.180
- Carpenter, L. L., Kronenberg, E. F., Tirrell, E., Kokdere, F., Beck, Q. M., Temereanca, S., Fukuda, A. M., Garikapati, S., & Hagberg, S. (2022). Mechanical affective touch therapy for anxiety disorders: Feasibility, clinical outcomes, and electroencephalography biomarkers from an open-label trial. Frontiers in Psychiatry, 13(April), 877574. https://doi.org/10.3389/fpsyt.2022.877574
- Carter, S. L. (2005). An empirical analysis of the effects of a possible sinus infection and weighted vest on functional analysis outcomes of self-injury exhibited by a child with autism. Journal of Early and Intensive Behavior Intervention, 2(4), 252–258. https://doi.org/10.1037/h0100318
- Champagne, T., Mullen, B., Dickson, D., & Krishnamurty, S. (2015). Evaluating the safety and effectiveness of the weighted blanket with adults during an inpatient mental health hospitalization. Occupational Therapy in Mental Health, 31(3), 211–233. https://doi.org/10.1080/0164212X.2015.1066220
- Chen, H. Y., Yang, H., Chi, H. J., & Chen, H. M. (2019). Parasympathetic effect of deep pressure input on third molar extraction in adolescents. Journal of the Formosan Medical Association = Taiwan yi Zhi, 118(9), 1317–1324. https://doi.org/10.1016/j.jfma.2019.05.026
- Chen, H. Y., Yang, H., Meng, L. F., Chan, P. Y. S., Yang, C. Y., & Chen, H. M. (2016). Effect of deep pressure input on parasympathetic system in patients with wisdom tooth surgery. Journal of the Formosan Medical Association = Taiwan yi Zhi, 115(10), 853–859. https://doi.org/10.1016/j.jfma.2016.07.008
- Duvall, J. C., Schleif, N., Dunne, L. E., & Holschuh, B. (2019). Dynamic compression garments for sensory processing disorder treatment using integrated active materials. Journal of Medical Devices, 13(2), 021001. https://doi.org/10.1115/1.4042599
- Edelson, S. M., Edelson, M. G., Kerr, D. C. R., & Grandin, T. (1999). Behavioral and physiological effects of deep pressure on children with autism: A pilot study evaluating the efficacy of Grandin’s Hug Machine. The American Journal of Occupational Therapy: Official Publication of the American Occupational Therapy Association, 53(2), 145–152. https://doi.org/10.5014/ajot.53.2.145
- Ehrhardt, N. M., Fietz, J., Kopf-Beck, J., Kappelmann, N., & Brem, A. K. (2022). Separating EEG correlates of stress: Cognitive effort, time pressure, and social-evaluative threat. The European Journal of Neuroscience, 55(9–10), 2464–2473. https://doi.org/10.1111/ejn.15211
- Fertel-Daly, D., Bedell, G., & Hinojosa, J. (2001). Effects of a weighted vest on attention to task and self-stimulatory behaviors in preschoolers with pervasive developmental disorders. The American Journal of Occupational Therapy: Official Publication of the American Occupational Therapy Association, 55(6), 629–640. https://doi.org/10.5014/ajot.55.6.629
- Field, T. (2000). Touch therapy. Churchill Livingstone.
- Foo, E., Lee, J. W., Ozbek, S., & Holschuh, B. (2018). Preliminary study of the subjective comfort and emotional effects of on-body compression. In Proceedings of the 2018 ACM International Symposium on Wearable Computers (pp. 128–131). ACM. https://doi.org/10.1145/3267242.3267279
- Gärtner, M., Rohde-Liebenau, L., Grimm, S., & Bajbouj, M. (2014). Working memory-related frontal theta activity is decreased under acute stress. Psychoneuroendocrinology, 43, 105–113. https://doi.org/10.1016/j.psyneuen.2014.02.009
- Grandin, T. (1984). My experiences as an autistic child and review of selected literature. Journal of Orthomolecular Psychiatry, 13(3), 144–174.
- Grandin, T. (1992). Calming effects of deep touch pressure in patients with autistic disorder, college students, and animals. Journal of Child and Adolescent Psychopharmacology, 2(1), 63–72. https://doi.org/10.1089/cap.1992.2.63
- Hodgetts, S., Magill-Evans, J., & Misiaszek, J. (2011a). Effects of weighted vests on classroom behavior for children with autism and cognitive impairments. Research in Autism Spectrum Disorders, 5(1), 495–505. https://doi.org/10.1016/j.rasd.2010.06.015
- Hodgetts, S., Magill-Evans, J., & Misiaszek, J. E. (2011b). Weighted vests, stereotyped behaviors and arousal in children with autism. Journal of Autism and Developmental Disorders, 41(6), 805–814. https://doi.org/10.1007/s10803-010-1104-x
- Holschuh, B., & Newman, D. (2014). Low spring index, large displacement Shape Memory Alloy (SMA) coil actuators for use in macro- and micro-systems. In Reliability, Packaging, Testing, and Characterization of MOEMS/MEMS, Nanodevices, and Nanomaterials XIII (Vol. 8975, p. 897505). SPIE. https://doi.org/10.1117/12.2044406
- Horder, J., Petrinovic, M. M., Mendez, M. A., Bruns, A., Takumi, T., Spooren, W., Barker, G. J., Künnecke, B., & Murphy, D. G. (2018). Glutamate and GABA in autism spectrum disorder-a translational magnetic resonance spectroscopy study in man and rodent models. Translational Psychiatry, 8(1), 106. https://doi.org/10.1038/s41398-018-0155-1
- Hussain, I., Hossain, M. A., Jany, R., Bari, M. A., Uddin, M., Raihan, A., Kamal, M., Ku, Y., & Kim, J.-S. (2022). Quantitative Evaluation of EEG-Biomarkers for Prediction of Sleep Stages. Sensors, 22(8), 3079. https://doi.org/10.3390/s22083079
- Hussain, I., & Park, S. J. (2020). HealthSOS: Real-time health monitoring system for stroke prognostics. IEEE Access. 8, 213574–213586. https://doi.org/10.1109/ACCESS.2020.3040437
- Hussain, I., & Park, S. J. (2021). Quantitative evaluation of task-induced neurological outcome after stroke. Brain Sciences, 11(7), 900. https://doi.org/10.3390/brainsci11070900
- Hussain, I., Young, S., & Park, S. J. (2021). Driving-induced neurological biomarkers in an advanced driver-assistance system. Sensors (Basel, Switzerland), 21(21), 6985. https://doi.org/10.3390/s21216985
- Islam, M. S., Hussain, I., Rahman, M. M., Park, S. J., & Hossain, M. A. (2022). Explainable artificial intelligence model for stroke prediction using EEG signal. Sensors (Basel, Switzerland), 22(24), 9859. https://doi.org/10.3390/s22249859
- Klimek, L., Bergmann, K. C., Biedermann, T., Bousquet, J., Hellings, P., Jung, K., Merk, H., Olze, H., Schlenter, W., Stock, P., Ring, J., Wagenmann, M., Wehrmann, W., Mösges, R., & Pfaar, O. (2017). Visual analogue scales (VAS) - measuring instruments for the documentation of symptoms and therapy monitoring in case of allergic rhinitis in everyday health care. Allergo Journal International, 26(1), 16–24. https://doi.org/10.1007/s40629-016-0006-7
- Krauss, K. E. (1987). The effects of deep pressure touch on anxiety. The American Journal of Occupational Therapy: Official Publication of the American Occupational Therapy Association, 41(6), 366–373. https://doi.org/10.5014/ajot.41.6.366
- Lomas, T., Ivtzan, I., & Fu, C. H. Y. (2015). A systematic review of the neurophysiology of mindfulness on EEG oscillations. Neuroscience and Biobehavioral Reviews, 57, 401–410. https://doi.org/10.1016/j.neubiorev.2015.09.018
- Losinski, M., Cook, K., Hirsch, S., & Sanders, S. (2017). The effects of deep pressure therapies and antecedent exercise on stereotypical behaviors of students with autism spectrum disorders. Behavioral Disorders, 42(4), 196–208. https://doi.org/10.1177/0198742917715873
- Matson, J. L. (2017). Handbook of treatments for autism spectrum disorder. Springer.
- Maula, M. I., Aji, A. L., Aliyafi, M. B., Afif, I. Y., Ammarullah, M. I., Winarni, T. I., & Jamari, J. (2021). The subjective comfort test of autism hug machine portable seat. Journal of Intellectual Disability - Diagnosis and Treatment, 9(3), 182–188. https://doi.org/10.6000/2292-2598.2021.09.02.4
- McDougle, C. J. (2016). Autism spectrum disorder. Oxford University Press.
- Mishra, P., Pandey, C. M., Singh, U., Gupta, A., Sahu, C., & Amit, K. (2019). Descriptive statistics and normality tests for statistical data. Annals of Cardiac Anaesthesia, 22(1), 67–72. https://doi.org/10.4103/aca.ACA_157_18
- Morrison, A., Knoche, H., & Manresa-Yee, C. (2015). Designing a vibrotactile language for a wearable vest. Lecture Notes in Computer Science (Including Subseries Lecture Notes in Artificial Intelligence and Lecture Notes in Bioinformatics), 9187, 655–666. https://doi.org/10.1007/978-3-319-20898-5_62
- Phutela, N., Relan, D., Gabrani, G., Kumaraguru, P., & Samuel, M. (2022). Stress classification using brain signals based on LSTM network. Computational Intelligence and Neuroscience, 2022, 7607592–7607513. https://doi.org/10.1155/2022/7607592
- Poon, K. K., Chew, I., Tan, A., & Teh, J. (2014). The effectiveness of the t. jacket for children with autism spectrum disorders. Journal of Applied Research in Intellectual Disabilties, 27(4), 384.
- Rayi, A., & Murr, N. (2021). Electroencephalogram. In StatPearls [Internet]. StatPearls Publishing.
- Reichow, B., Barton, E. E., Sewell, J. N., Good, L., & Wolery, M. (2010). Effects of weighted vests on the engagement of children with developmental delays and autism. Focus on Autism and Other Developmental Disabilities, 25(1), 3–11. https://doi.org/10.1177/1088357609353751
- Reynolds, S., Lane, S. J., & Mullen, B. (2015). Effects of deep pressure stimulation on physiological arousal. The American Journal of Occupational Therapy: official Publication of the American Occupational Therapy Association, 69(3), 6903350010p1–6903350010p5. https://doi.org/10.5014/ajot.2015.015560
- Rojas, D. C., Singel, D., Steinmetz, S., Hepburn, S., & Brown, M. S. (2014). Decreased left perisylvian GABA concentration in children with autism and unaffected siblings. NeuroImage, 86, 28–34. https://doi.org/10.1016/j.neuroimage.2013.01.045
- Ross, T. A., Kennedy, T., Barton, M., & Cadogan, A. (2019). Pressure Seeker©: Integration of self-regulated deep pressure solutions into wearables. Materials Today: Proceedings, 16, 1431–1435.
- Taj-Eldin, M., Ryan, C., O'Flynn, B., & Galvin, P. (2018). A review of wearable solutions for physiological and emotional monitoring for use by people with autism spectrum disorder and their caregivers. Sensors (Basel, Switzerland), 18(12), 4271. https://doi.org/10.3390/s18124271
- Taylor, C. J., Spriggs, A. D., Ault, M. J., Flanagan, S., & Sartini, E. C. (2017). A systematic review of weighted vests with individuals with autism spectrum disorder. Research in Autism Spectrum Disorders, 37, 49–60. https://doi.org/10.1016/j.rasd.2017.03.003
- Vandenberg, N. L. (2001). The use of a weighted vest to increase on-task behavior in children with attention difficulties. The American Journal of Occupational Therapy: official Publication of the American Occupational Therapy Association, 55(6), 621–628. https://doi.org/10.5014/ajot.55.6.621
- Watkins, N., & Sparling, E. (2014). The effectiveness of the Snug Vest on stereotypic behaviors in children diagnosed with an autism spectrum disorder. Behavior Modification, 38(3), 412–427. https://doi.org/10.1177/0145445514532128
- Whybrow, S., Stephen, J. R., & Stubbs, R. J. (2006). The evaluation of an electronic visual analogue scale system for appetite and mood. European Journal of Clinical Nutrition, 60(4), 558–560. https://doi.org/10.1038/sj.ejcn.1602342
- Yu, C., & Wang, M. (2022). Survey of emotion recognition methods using EEG information. Cognitive Robotics, 2(May), 132–146. https://doi.org/10.1016/j.cogr.2022.06.001
- Zissermann, L. (1992). The effects of deep pressure on self-stimulating behaviors in a child with autism and other disabilities. The American Journal of Occupational Therapy: Official Publication of the American Occupational Therapy Association, 46(6), 547–551. https://doi.org/10.5014/ajot.46.6.547