Abstract
Carbon sequestration is defined as the process of capturing and storing atmospheric carbon dioxide. Fruit crops are indispensable both for climate change mitigation and ensuring food security. However, the impact of fruit trees is not adequately investigated. This review assesses the carbon sequestration potential of fruit trees and their implications for climate change mitigation. Fruit trees use photosynthesis to absorb CO2 from the atmosphere and assimilate it into their cellulose, lowering atmospheric buildup. Horn of Africa is the most vulnerable region for climate change, and Ethiopia is also facing unpredictable weather, which brings sporadic floods and droughts that harm the agricultural sectors. Dramatic rise of CO2 from 280 ppm in 1850 to 420.2 ± 0.5 ppm in 2023 is reported to link with human activity. In most Ethiopian farms, multipurpose fruit trees are rarely cultivated, and the only experience is planting trees in the homestead areas. Even though fruit trees have an enormous potential to store carbon, the destruction of those trees is also results greenhouse gas. Tree plants, including fruit trees, are thought to absorb 0.42 to 0.65 pentagrams of carbon per year. Above- and below-ground biomasses have been described to sink more than 40% of carbon. Agroforestry practices should adopt all fruit species on the basis of carbon sequestration and climate change mitigation in their growing stratum. Therefore, in order to oblige countries to adopt versatile fruit trees to meet food and nutrition security, carbon sequestration, and climate change mitigation efforts should have both political and economic sustainability.
PUBLIC INTEREST STATEMENT
Carbon sequestration is defined as the course of apprehending and storing atmospheric carbon-dioxide and it is an effective method to reduce the atmospheric carbon-dioxide. Climate change is a pressing issue in Ethiopia exacerbated by human activities such as fossil fuel consumption and deforestation. In Ethiopia, climate variability is highly affecting the environmental settings and causing for soil erosion, drought, desertification, biodiversity loss, flooding, and water pollution, and health risks. Multipurpose fruit trees are an important carbon pool used to store large amounts of carbon in their biomass (above and below ground biomass). Globally, versatile fruit trees are extensively used to sequester carbon from the atmosphere. In general, in addition to the forest trees, cultivation of multipurpose fruits is paramount to protect our environment. Therefore, fruits selection on the basis on their nutrition composition and role of carbon sequestration is imperative for the nations such as Ethiopia.
1. Introduction
Nowadays, carbon dioxide (CO2) emissions and climate change are global concerns. Moreover, global warming, which is a result of human activity, is the most feared concern of the new millennium (Abbass et al., Citation2022). Climate change is a pressing issue exacerbated by human activities like high fossil fuel consumption and deforestation, which contribute to the loss of biodiversity (Shivanna, Citation2022). Carbon is a crucial life component found in plant biomass, soil, geological deposits, atmosphere, and seawater in dissolved forms (Patil & Kumar, Citation2017). Since the level of carbon dioxide increased from 280 parts per million (ppm) in 1850 to 420.2 ± 0.5 ppm in 2023, a number of factors are stated by different scholars for this increment (Godard, Citation2017). In Ethiopia, carbon emissions for 2020 were 18,098.00 with 2.2% increment from 2019. While in 2019, 17708.00 were reported with 3.99% rise from 2018. Similarly, carbon emissions for 2018 were about 17,028.40 with 7.22% increment from 2017. Correspondingly, carbon emissions for 2017 were nearly 16,000.00 and 4.17% increments from 2016 were also reported (Hundie, Citation2021). In general, a total of 3.3 billion tons of carbon per year is reported to be added in to the atmosphere (Ukpanyang & Terrados-Cepeda, Citation2022). The consumption of solid, liquid, and gas fuels are reported to contribute to carbon dioxide emissions, which are produced during the burning of fossil fuels and the production of cement (Friedlingstein et al., Citation2022).
The process of carbon sequestration includes CO2 absorption from the atmosphere and storing it as carbon in biomass such as tree trunks, branches, foliage, roots, and soils (Hurd et al., Citation2022).
A number of factors, such as tree age, leaf area, and photosynthetic efficiency are reported to affect the carbon capturing process during photosynthesis (Wang et al., Citation2023). The major quantities of carbon are reported to be stored in above- and below-ground biomass, dead wood, litter, and soil organic matters (Dibaba et al., Citation2019). Moreover, the amount of carbon that trees store depends on their age, species, soil type, climate, terrain, and management practices (Vacek et al., Citation2023). For instance, the rate of carbon storage is reported to rise in young trees, but it decreases after reaching the maximum size (Harris & Betts, Citation2023).
Fruit trees are stated to contribute significantly to the reduction of atmospheric carbon dioxide through carbon sequestration (DiMatteo et al., Citation2023). Because due to their structural differences from annual crops, fruit trees are told to absorb considerable quantities of atmospheric carbon (Song et al., Citation2023). Trees and grasslands sink atmospheric carbon; whereas agricultural practices (ploughing, cultivation, etc.) generate greenhouse gases (Zhan et al., Citation2023). Therefore, it’s vital to use good farming techniques that can lessen emissions through increasing the biosphere’s capacity to store carbon (Lal, Citation2023). In addition, replacement vegetation on cleared land is also reported to sequester carbon. On the other hand, plants store carbon in the soil where it can be used as soil organic carbon (SOC) through photosynthesis.
Irrespective of the potentiality of fruit crops, large numbers of smallholder farmers in Ethiopia are facing many challenges including awareness gaps, lack of improved varieties, soil depletion, variable weather conditions, pest and disease problems, weak market system, and lack of postharvest facilities, unreliable rainfall, low price, high input costs, low incentives, low output, insufficient extension services, low labour productivity, poor infrastructure and poor harvest management systems. In addition, insufficient researches and documentation on carbon sequestration potential of fruit trees are also the major hindrances affecting fruits cultivation in Ethiopia. Thus, this review aims to assess the carbon sequestration potential of fruit trees and their implications for climate change mitigation.
2. Methodology
During the literature review, the authors used various strategies. Reputable journals from the Scopus, Web of Science, and PubMed databases were used for the compilation of this review. The inclusion criteria primarily focused on articles published after 2019, except for relevant facts and books.
3. Global climate change
Agriculture is one of the key sectors in Africa and is critical to the continent’s growth. It is identified to employ over 65% of the labour force and contributes to 32% of gross domestic production (GDP) (Osabohien et al., Citation2019). However, Africa continent in general and the Horn in particular is the most at risk from climate change (Sharmake et al., Citation2023). Frequently, in the Horn of Africa, unpredictable weather affects agricultural production through bringing drought and sporadic floods (Tirado et al., Citation2015). Africa’s agriculture is therefore anticipated to be mostly vulnerable given that the continent already experiences high temperatures and diminutive precipitation (Davis, Citation2023). By the 2060s and 2090s, the region’s mean annual temperature is predicted to rise by 1.1 to 3.1°C and 1.5 to 5.1°C respectively (Mengesha Tadesse, Citation2023). These massive escalations are estimated to cause droughts and floods as well as steady degradation of the natural resources (Dongmo, Citation2022).
Apart from presenting additional complications, climate change poses a risk to realizing development and poverty reduction goals (Meyfroidt et al., Citation2022). Hence, the consequences of climate change could be quite severe because 80% of the world’s population relies on weather-dependent agriculture and the majority of which is small-scale farming or pastoralists (Bogale & Erena, Citation2022). Furthermore, extreme climate variation may disturb environmental settings and may cause soil erosion, deforestation, drought, overgrazing, desertification, biodiversity loss, flooding, and water pollution, food insecurity, energy shortage, and health threat (Oljirra, Citation2019). Thus, the people who depend on the environment, mostly the poorest and marginalized societies, will inevitably be the ones who suffer severely in effect of the climate change (Wassie, Citation2020). Correspondingly, the impacts on agricultural production, food security, and water availability are the common challenges of climate change in Ethiopia (Bogale & Erena, Citation2022).
Although fruit trees are reported to sink carbon, the attention for this approach is very low in developing countries including Ethiopia (Chakravarty et al., Citation2019). For example, apple trees intercropping with rajmash and oats were stated to store the highest ecosystem carbon, and 1.5–2 times higher than other cultivated crops (Zahoor et al., Citation2021). Moreover, fruit orchards and vineyards are told to acculumalate a substantial amounts of carbon by their structures (Sharma et al., Citation2021a). Thus, orchard management can enhance carbon pools in biomass and soil, but forest trees are chiefly considered for carbon trading and ecological amenities (Guidi Nissim et al., Citation2023). This could be partly explained by the lack of studies on the carbon storage capacity of various fruit trees (Plénet et al., Citation2022). Due to the expansion of agriculture in the tropical regions, forest resources are highly threatened. So, if fruit orchards are properly managed, they are reported to contribute a lot for sustainable development (Altieri & Koohafkan, Citation2008).
Just like forest trees, planting fruit trees in homesteads and other agricultural fields are indicated to improve the carbon sink (Jerikias & Zakio, Citation2022). In Ethiopia, most of the fruit trees are cultivated around the homesteads because they are important sources of food and income mainly in rural areas (Sinare et al., Citation2022). Fruit trees are described to use CO2 as an input for the photosynthesis process and it is absorbed from the atmosphere and assimilate it into their cellulose and lowering atmospheric accumulation (Gressel, Citation2008). Thus, to take use of their capacity for carbon sequestration, several fruit trees with adaptive species and cultivars might be chosen (Zsögön et al., Citation2022). Fruit trees on homesteads are reported larger than those in normal fruit orchards because they are not properly managed and hence pruning the trees could offer farmers with wood biomass energy (Lyashenko et al., Citation2022).
According to a study done on biological carbon sequestration through fruit crops (perennial crops natural “sponges” for absorbing carbon dioxide from atmosphere), CO2 is a major contributor to the greenhouse effect and can be naturally removed from the atmosphere by storing it in the biosphere (Patil & Kumar, Citation2017). In addition, mango trees had also special microclimatic conditions appeared to favor waste photodegradation, and thus eliminating the nutrients that were not incorporated into the soil (Reyes-Martín et al., Citation2021). For instance, the carbon pool of fruit trees is presented with a model example of an apple tree in Figure .
Figure 1. Typical representation of an apple tree along with a schematic depiction of carbon pools.
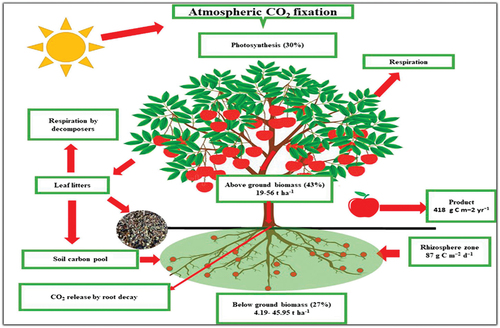
According to a study conducted at Indonesia’s Purwodadi Botanic Garden in 2022 to estimate the carbon stock of numerous local fruit species collections, the majority of the indigenous fruit trees examined are potential carbon sink and should be encouraged in restoration programmes to reduce climate change (Yulistyarini & Hadiah, Citation2022).
A study conducted on the carbon sequestration potential of mango orchards in the tropical hot and humid climate of Konkan region, India, stated that polyembryonic seedling-grown mangoes have very high carbon sequestration potential than the grafted mangoes (Ganeshamurthy et al., Citation2019).
Furthermore, a survey done on the carbon sequestration potential of orchards and vineyards in Italy indicated that strong fruit tree ecosystems have positive net ecosystem productivity and net ecosystem carbon balance (Scandellari et al., Citation2016).
Moreover, a research conducted to evaluate the contribution of Tropical Fruit Plants and Soil Properties to the potential of Carbon Sequestration in Open Land Utilization for Mixed Plantations showed that there is a connection between soil properties and soil organic carbon, with low N-total content resulting in low soil organic carbon (Oviantari et al., Citation2021).
Similarly, a study done on the carbon sequestration potential of agroforestry systems in degraded landscapes in West Java, Indonesia, indicated that carbon stock variance is correlated with species density and variety (Siarudin et al., Citation2021).
On the other hand, research conducted on the management of soil organic matter and carbon storage in tropical fruit crops in Brazil stated that converting native forest to fruit crops reduced soil organic matter content and quality, impacting carbon dynamics and agricultural soil stocks (Guimarães et al., Citation2014).
According to a research done on fruit-based systems: a feasible alternative for carbon sequestration, fruit trees may grow for years and continue to store carbon as they increase biomass (Tesfay et al., Citation2022).
Assessment studies conducted on the appraisal of carbon capture, storage, and utilization through fruit crops in India indicated that the carbon storage potential of fruit trees may vary based on growth, development, and environmental conditions (Sharma et al., Citation2021b).
A field modelling study carried out to understand the carbon sequestration by fruit trees in apple orchards in Changping District, Beijing-China reported that carbon emissions resulting from management practices would not be offset through carbon storage in apple trees before they reached the mature stage (Wu et al., Citation2012). Consequently, in addition to producing apples and providing fruits, apple production systems could be assumed as carbon sinks when fruit production is excluded (Figure ). According to a report from the United Nations Environment foresight013-brief, “Early warning, Emerging issues and futures,” It is significant that soil, through the oxidation of soil carbon contributes to climate change (Momani & Alzaghal, Citation2009).
A study done on carbon sequestration potential of tree crop plantations: Mitigation and Adaptation Strategies for Global Change in Denmark have shown that tree crop plantations can sequester carbon more effectively on low-carbon land (Kongsager et al., Citation2013).
According to a study done to assess the growth, carbon stock, and sequestration potential of oil palm plantations in Mizoram, Northeast India, there is significant carbon storage in oil palm plantations, with higher values than shifting agriculture fallows (Singh et al., Citation2018).
Similarly, research was done to evaluate the role of citrus orchards in reducing climate change through carbon sequestration in Pakistan’s Sargodha District in 2021, measuring carbon with straightforward methods can yield reliable results that increase the participation of developing countries in various payment initiatives like REDD+ (Yasin et al., Citation2021).
Correspondingly, according to the case study report from Htee Pu Climate Smart Village on the financial and environmental benefits of fruit trees in Myanmar’s central dry zone, there is an increase in interest worldwide in ecosystem restoration and rehabilitation of damaged landscapes (Manilay et al., Citation2021). Climate-Smart Agriculture can be used as a strategy by agricultural and development organizations to address degradation on small farms and related landscapes. On the other hand, carbon investors are reported to depend on farmer preferences (Roshetko et al., Citation2002). Comparatively, fruit trees and other crops often have lower carbon footprint value than animal products (Table ).
Table 1. The average carbon footprint values of a few fruits cultivated worldwide
4. The impact of climate change on food security
Climate change is reported to affect all pillars of the food security (Stavi et al., Citation2022). For example, in Ethiopia, climate change (external factors) is commonly mentioned to affect Ethiopia’s food security (Rahman et al., Citation2022). Globally, many research works are performed to evaluate the effects of climate change on agricultural production and food security of smallholder farmers (Wheeler & Von Braun, Citation2013). However, understanding about how anthropogenic climate change will affect local communities or individual households is somewhat limited. Because it is challenging to differentiate the effects of human interference and those of natural weather variations on climate change (Osberghaus & Fugger, Citation2022). Furthermore, it is difficult to anticipate both the potential good and negative effects as well as how they will all interact in different settings (Goswami et al., Citation2022). The National Adaptation Programme of Action in Ethiopia (NAPAE) highlights to accurately assess the impact of climate change across socio-economic sectors (Singh, Citation2020). Correspondingly, evaluating issues like crop consequences, productivity, carbon effects, socioeconomic changes, and technological advancements are remain challenging (Abbas, Citation2022). Climate change is reported to affect the growing seasons of agricultural crops (Thornton et al., Citation2014). Agricultural sector is the main source of income, hard currency, and food security of Ethiopia (Guyalo et al., Citation2022). Due to the dominance of small-scale farmers who mostly depend on rain-fed agriculture and traditional farming, Ethiopia is highly vulnerable to climate change and climate variability. According to Ethiopia’s regional vulnerability analysis, arid and semi-arid areas like Afar and Somali are sensitive to climate change (Bogale & Erena, Citation2022). Although Borena and Tigray regions experience good agricultural productivity in the highlands and midlands, persistent drought are affecting the lowland areas of the two regions (Deressa et al., Citation2008).
5. The role of fruit trees in mitigating climate change
5.1. Carbon pools in fruit tree ecosystems
5.1.1. Store carbon
Photosynthesis is the process by which trees absorb CO2 from the atmosphere, mixing it with water and sunshine to produce glucose and oxygen (Manilay et al., Citation2021). Researchers stated that carbon is stored in the biomass of trees (above and below the ground) as well as in the soil organic matter beneath the canopy of trees (Vashum & Jayakumar, Citation2012). Numerous studies have shown that fruit plants, such as mango plantations in the Philippines, sequester CO2 levels ranging from 47.66 to 62.33 tons/ha of CO2 (Janiola & Marin, Citation2016). While Chavan and Rasal reported that the Philippines had a higher concentration of 206.6 tons/ha CO2 (Chavan & Rasal, Citation2012). Research indicates that mango trees have various carbon stocks, including 1.5 tons/ha CO2/ha, 80.74 tons CO2/ha, and 45–85 tons CO2/ha (Babbar et al., Citation2021; Ganeshamurthy et al., Citation2019; Prasanna & Selvaraj, Citation2016). According to the study done on Jackfruit, it is reported to contain 26.7 tons of CO2 per hectare (Manilay et al., Citation2021). In addition, estimates of CO2 sequestration per tree for guava (0.012 tons CO2/tree) and mango (0.21 tons CO2/tree) were reported by the researchers (Kumar & Kunhamu, Citation2021).
5.1.2. Above and belowground biomass
A study done in Indonesia estimated that the carbon sequestration capacity of fruit tree biomass, with average carbon stock values ranging from 382 Gg to 245,726 Gg (Gunamantha et al., Citation2021). Accordingly, Ades fruits have a carbon sequestration potential of 951.1356 tons per hectare above ground and 191.5545 tons per hectare below ground, which can help reduce climate change (Shiferaw et al., Citation2022). High CO2 exposure is reported to boost photosynthesis in tropical and subtropical fruits, and increase tree biomass, thereby reducing atmospheric carbon dioxide (Pandya et al., Citation2013). The coffee agroforestry system’s in the above-ground carbon component has a higher carbon store compared to other carbon components (Niguse et al., Citation2022). Soil organic carbon and below-ground carbon pools are reported as the next largest sources of carbon storage, each with an average capacity of 156.6 tons of carbon per hectare (Ajonina et al., Citation2014). In general, the coffee agroforestry system is stated to store 30.5 Gg of carbon, with coffee plants specifically contributing 4.4 Gg to the system .
Table 2. Carbon stock potential of fruit trees in different carbon pools
5.1.3. Litter biomass
According to a study done to assess the carbon sequestration potential of Ades fruits and its contribution to climate change mitigation, litter has a poor carbon storage capacity and low sequestration potential, with a mean carbon storage capacity of 2.1 tons C/ha−1 (Kassahun et al., Citation2015). Additionally, the litter biomass had an average carbon dioxide sequestration potential of 9.44 tons/ha−1. Litter addition is reported to increase soil organic carbon (SOC) pools and microbial biomass, indicating that aboveground litter inputs benefit SOC sequestration despite the accelerated decomposition (Feng et al., Citation2022). Moreover, rapid and consistent changes in soil C content with altered litter inputs provided important insights into plant residue management to enhance soil C sequestration (Xu et al., Citation2021). In general, aboveground plant litter inputs are important sources of soil carbon (C) (Table ).
5.1.4. Soil carbon
Soil is the world’s greatest terrestrial carbon sink, with soil organic matter constituting the majority of the carbon stored there (Berhe et al., Citation2008). How much carbon is kept in soil organic matter depends on the addition of carbon from decomposing plant residues and carbon loss through respiration, and both natural and human disturbance of the soil (Trumbore, Citation1997). Thus, by using different agricultural techniques that disturb little soil, farmers may be able to slow down or perhaps stop the loss of carbon from their fields (Reicosky, Citation2003). There was a substantial correlation between the distribution of individual soil particles and soil organic carbon (Gunamantha et al., Citation2021). For instance, the association between soil organic carbon and sand and silt particles is reported to be extremely poor and hostile (Dong et al., Citation2017). Sandy soils are stated to have low organic carbon content, but clay particles have the strongest positive relationship (Eusterhues et al., Citation2003). Hence, in order to trap more carbon in the soil, soil management and the addition of organic carbon through crop leftovers or manure are more crucial than the texture of the soil (Blanco-Canqui, Citation2013). Moreover, at a depth of 0–50 cm, 0–75 cm, and 0–25 cm, the largest positive association between clay dispersion and soil organic carbon was reported (Somasundaram et al., Citation2017).
Both exchangeable potassium and available phosphorus exhibit a stronger negative correlation with soil organic carbon (Jakšić et al., Citation2021). Soil carbon storage decreases with increased plant nutrients, but their availability is strongly linked to soil organic carbon (Elbasiouny et al., Citation2022). In addition, total nitrogen and available phosphorus were reported to have positive and negative relationships, with negligible effects at low concentrations. Similarly, soil organic carbon stock at a depth of 60 cm is reported from 73.2 tons C/ha−1 for the Syzygium-shaded coffee stratum to 103.2 ton C/ha−1 for the mixed-tree-shaded coffee stratum (Niguse et al., Citation2022). Thus, the presence of multiple plant species with varying degrees of decomposition could account for the high soil organic carbon stores in the mixed tree-shaded layer (Chatterjee et al., Citation2019). Organic matter in the soil distresses its capacity to store carbon to decline with the depth (Torn et al., Citation1997). While the distribution of individual soil particles did not demonstrate a significant association with soil organic carbon, and the average carbon stock per hectare of soil reported was 440 kg/ha−1 (Mathew et al., Citation2020).
5.2. Environmental elements affecting fruit carbon stocks
Research done on factors affecting woody carbon stock in Sirso moist evergreen Afromontane forest, southern Ethiopia: implications for climate change mitigation stated that there were differences in the carbon stock of woody species depending on the kind of community, altitude, and slope gradients (Mewded & Lemessa, Citation2020). Moreover, geographical factors and vegetation mix are vital when selecting trees for climate change mitigation, especially fruit trees (Mewded & Lemessa, Citation2020). Different altitudinal gradients are stated to have various quantities of above-ground carbons (Eshetu et al., Citation2020). For instance, in the upper altitude gradient the maximum above-ground carbon concentration was reported to be 187.3 27 tons/ha−1, while in the lower, it is estimated to be 84.7 9.2 tons/ha−1. The highest value of the upper altitude might be due to the topography, where the upper altitude has a nearly sharp slope that has isolated itself from human impact. In general, the results has shown that the altitudinal gradient has a significant effect on the above-ground biomass, and when altitude increases, the above-ground carbon also increases (Fotis et al., Citation2018).
5.2.1. Disturbance level
In addition to environmental conditions, the amount of carbon storage is influenced by a number of factors. For instance, a significant amount of carbon was reported to be stored in the tree biomass and soil in the citrus-based plantings (Yasin et al., Citation2021). Citrus trees’ age, growth patterns, and management techniques influence carbon stocks, making them potential climate change sequestered through fruit-based agroforestry systems (Dagar & Yadav, Citation2017). In every least disturbed area, the above ground biomass and carbon stocks are reported to be varied extensively (Gebeyehu et al., Citation2019).
6. Fruit tree production and research experiences in Ethiopia
Ethiopia’s agricultural sector has just lately started to produce fruit, and the majority of its native fruits are wild, and there are no adequate improved varieties (Rahiel et al., Citation2018). Most of the fruits grown in commercial farms and orchards are exotic and were only recently introduced to Ethiopia. Producers, merchants, and users are therefore unfamiliar with their management. Fruit production and consumption have a number of problems that need to be properly identified and addressed (Salgueiro et al., Citation2010). Ethiopia’s fruit is primarily grown in backyard gardens and small-scale farms, with domestic consumption being the primary market (Gebre Mariam, Citation2003). The most common fruits grown in Ethiopia are peaches, avocado, mangoes, citrus, Lemons, Mandarins, Papayas, Pineapples, and plums, and the quantity is by no means insufficient (Melke & Fetene, Citation2014). However, children are only collected and served the native fruits like “Agam,” “Kegaa,” “Shola,” and “Koshim” among others.
On the other hand, Ethiopia, where agriculture is the country’s key sector, is severely affected by the recurrent drought and poverty (Mohamed, Citation2017). Ethiopia primarily uses fruit trees for income generation (domestic market), and household consumption. However, some other African countries are integrating fruit trees for environmental protection and climate change mitigation.
A review done in Malawi reported that appropriate tree management practices can increase carbon sequestration and socioeconomic benefits (Mng’omba & Beedy, Citation2013).
According to a study conducted in Kampala, Uganda (Africa) to examine the carbon sequestration of fruit trees under various management regimes in 2022, mango fruits have a great potential to sequester carbon emissions and reducing global warming (Ganeshamurthy et al., Citation2019).
While in Ethiopia, research done on fruit trees’ role in reducing climate change is very limited, rather than most of the studies are focusing on forest trees and shrubs.
A study conducted to compare the biomass and soil carbon stock potential of various agroforestry practices and home gardens in the South Wollo Zone of Amhara regional state of Ethiopia reported that the total carbon stock in the home garden practices was 100.4 Mg C/ha−1, and the highest soil carbon stock was recorded from home garden agroforestry (94.2 Mg C/ha−1), followed by cultivated land (73 Mg C/ha−1) (Seid, Citation2022). Hence, the results of this study show that home gardens contribute the highest soil organic carbon and biomass, with a mean total ecosystem carbon of 84.3%.
A study done on a comparative assessment of soil organic carbon stock potential under agroforestry practices and other land uses in the lowlands of Bale, Ethiopia, stated that shade-grown coffee agroforestry in Ethiopia’s Dallo Mena district stores the highest carbon (Mengistu & Asfaw, Citation2017). Consequently, agroforestry practices offer a dual advantage including income generation, providing ecosystem services, storing and conserving significant amounts of carbon inside the system (Montes-Londoño, Citation2017). In addition, coffee fruits are the most profitable land-use practices since they provide a variety of benefits like income generation, carbon sequestration, and biodiversity preservation (Niguse et al., Citation2022). In July 2019, Ethiopia’s government gained international attention when it announced that it had set a record by planting more than 350 million trees in a single day (Fikreyesus et al., Citation2022). The Green Legacy Initiative programme, which aims to plant 20 billion trees in four years, was launched by the country’s leaders in 2019 (Fikreyesus et al., Citation2022). However, the government of Ethiopia lacks interest to integrate multipurpose fruits with forest trees. In 2023, Ethiopia’s government and society are prioritizing the promotion and advocacy of versatile fruit trees, which are projected to become the primary focus in the coming years.
7. The role of fruit trees for sustainable environment
Fruit tree planting is important for a healthy and prosperous future because all fruit trees play a crucial role in maintaining the balance of our planet (De Groot et al., Citation2002). Here are a few of the contributions that fruit plants perform. For example, storm water management in urban areas are reported to require trees including fruit trees for hydration and reducing erosion problems (Zhang et al., Citation2016). Thus, the inclusion of trees may assist in some of the drainage concerns if the area is extremely wet (Figure ).The best places to put trees and other vegetation as a mitigation means are around buildings or nearby the pavement in parking lots and on streets (Akbari, Citation2009). But, the most efficient way to cool a structure is reported to plant deciduous trees or vines to the west, particularly if they shade the building’s windows and a portion of its roof (Maleki, Citation2011). Moreover, vegetation is described to reduce urban heat by providing shade and evapotranspiration, because tree plants cool the environment (Aboelata & Sodoudi, Citation2019). For instance, shaded surfaces are stated to be cool between 11–25°C, absorb less heat, and reduce radiation from direct exposed areas (Bayu, Citation2022). In addition, comparative monoculture integration of fruit trees with other plant species slightly increases crop diversity, which benefits some taxa’s species richness without reducing yields (Jayathilake et al., Citation2021).
Moreover, integrating native fruit plants in coffee farms for bird conservation is indicated to be crucial (Carlo et al., Citation2004).
Fruit trees produce oxygen and absorb carbon dioxide, making substantial contributions to the oxygen and carbon dioxide cycle (Olah et al., Citation2009).
Fruit trees engage in a process known as photosynthesis, which converts water and carbon dioxide gas into oxygen and glucose using sunlight (Mohan et al., Citation2009). The trees aid in air purification and better air quality during the gas-exchanging process (Bolund & Hunhammar, Citation1999). Fruit trees are therefore critical to the oxygen and carbon dioxide cycles and to the world ecology (Wittwer, Citation1995). Foot-tall trees, like apple trees, can sustain four people at once by releasing up to 15 tons of oxygen per acre while absorbing 10–20 tons of carbon dioxide annually (Uning et al., Citation2020).
Fruit-bearing trees mitigate the negative effects of CO2 pollution, yet they still require CO2 for survival (Ramirez & Kallarackal, Citation2015). The air is cleaned or filtered by trees, which remove carbon dioxide and release new oxygen into the atmosphere (Cooper & Alley, Citation2010). One acre of mature fruit trees is reported to be able to absorb as much CO2 as 26,000 miles of driving (Aiyeloja, Citation2013).
8. Review gaps and future lines of work
In the coming years, fruits carbon sequestration research is poised to revolutionize fruit production and sustainable environment. In Ethiopia, the fruit production segment is hindered by poor growers’ skill and lack of improved varieties. Although there are numerous types of fruits in the country, they are not characterized based on their role of carbon sequestration. Even in agroforestry systems, the idea of carbon sequestration is almost abandoned. Researches conducted on multipurpose and resilient fruits are not adequate. In addition, international cooperation with institutes, companies, and universities are weak pertinent to climate change mitigation. The societal insights about the cultivation of multipurpose fruits are not widely addressed. Therefore, fruits production models should be calibrated and integrated with predictive and systematic agronomic approaches.
9. Conclusion
In conclusion, climate change is a pressing issue in Ethiopia exacerbated by human activities such as fossil fuel consumption and deforestation. Although, 85% of the country’s population depends on weather-dependent agriculture, climate change is posing risks to realizing development and poverty reduction goals in Ethiopia. The country has already experienced high temperatures and diminutive precipitation. In Ethiopia, climate variation is affecting environmental settings and causing soil erosion, drought, desertification, biodiversity loss, flooding, and water pollution, and health threats. On the other side, different countries in the world are using fruits for various purposes such as for food and nutrition security, climate change mitigation, and sustainable development. Because fruit trees are an important carbon pool used to store large quantities of carbon in their biomass. Both above and below ground biomass are used to store different amounts of carbon. Globally, multipurpose fruit trees are widely used or cultivated to sequester carbon from the atmosphere. However, it is not widely practiced in Ethiopia, rather unintended fruit cultivation activities are adept largely in the homestead areas. The type of fruits proposed for cultivation is not considered on the basis of their versatile advantage, rather they are selected based on their economic response alone. Thus, fruit tree cultivation experiences are not assuming climate change mitigation strategies through carbon sequestration in Ethiopia. In general, in addition to forest trees, the cultivation of multipurpose fruits is paramount to protect our environment. Hence, selection of fruits on the basis of their nutrition composition and the role of carbon sequestration are vital for countries such as Ethiopia.
Authors’ contribution
The concept of the study was developed by Yohannes Gelaye while the design, data analysis and synthesis, interpretation and drafting and final write-up of the paper were performed by both Yohannes Gelaye and Sewnet Getahun.
Availability of data and materials
Data sharing is not applicable to this article as no new data were created or analyzed in this study.
Public interest statementCleanVersion.docx
Download MS Word (13.2 KB)About the Author.docx
Download MS Word (14 KB)Acknowledgments
The authors acknowledge https://hindawi.writefull.ai/software.
Disclosure statement
No potential conflict of interest was reported by the author(s).
Supplementary material
Supplemental data for this article can be accessed online at https://doi.org/10.1080/23311932.2023.2294544.
Additional information
Funding
Notes on contributors
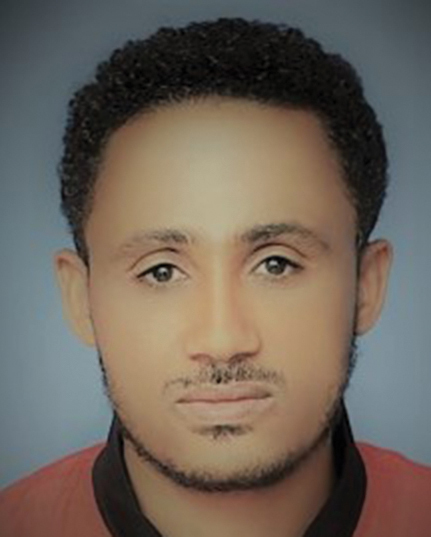
Yohannes Gelaye
Yohannes Gelaye is a lecturer and researcher in the Department of Horticulture, College of Agriculture and Natural resources, Debre Markos University, Ethiopia. He did his Master’s degree in Horticulture at Bahir Dar University, Ethiopia. Since December 2014, Yohannes is working at Debre Markos University and teaching courses like Plant biotechnology, Plant propagation, Design and agricultural experimentation, Vegetable and fruit crops production and management, Ornamental horticulture, Plant physiology, Coffee production, processing and quality control, Crop protection, and Nutrition sensitive agriculture. His research interest is Biotechnology, Biochar in agriculture, Adapting to drought stress, Byproduct utilization, Climate change induced disease and pests, Drought induced physio-morphological, molecular and biochemical changes, Agro-nanotechnology, Artificial Intelligence, Crops and soil improvement, Postharvest science and technology, Food safety, and Nutrition, and food security.
References
- Abbas, S. (2022). Climate change and major crop production: Evidence from Pakistan. Environmental Science and Pollution Research, 29(4), 5406–16. https://doi.org/10.1007/s11356-021-16041-4
- Abbass, K., Qasim, M. Z., Song, H., Murshed, M., Mahmood, H., & Younis, I. (2022). A review of the global climate change impacts, adaptation, and sustainable mitigation measures. Environmental Science and Pollution Research, 29(28), 42539–42559. https://doi.org/10.1007/s11356-022-19718-6
- Aboelata, A., & Sodoudi, S. (2019). Evaluating urban vegetation scenarios to mitigate urban heat island and reduce buildings’ energy in dense built-up areas in Cairo. Building and Environment, 166, 106407. https://doi.org/10.1016/j.buildenv.2019.106407
- Aiyeloja, A. (2013). Forest: Nature at your service. Journal of Agriculture, Socioeconomics and Sustainable Environment, 35–45. https://d1wqtxts1xzle7.cloudfront.net/36415367/Forest_Nature_at_your_Service._Aiyeloja__A.A._Ph.D-libre.pdf?1422368294=&response-content-disposition=inline%3B+filename%3DFORESTS_NATURE_AT_YOUR_SERVICE.pdf&Expires=1702388131&Signature=JhR3tgF2XWPYvzcj4cSDHBNjrACt9WaRQvDxtnAB5IrFGPWYRXqPlSSQsPmS1nPSbYJu2yTqXkgLrAklCERfPK4YUPd0byF7Cz5XbR-4C96OVPMs6neLdYvTE4IcYRDbZi60aAEp0pWOl1ZOnuPllsjTgXH7R39OaaUKVNUjL3lfehlTXsyfIDg4jGd7bwzs~3iwxkDVjN4U2KRbXVTWI5XKjw0S6xBwLkIwRDsezN0DALP4RPeRNWTJwq~B0hi48n13l6hZu9FdDw--SWs7sfxSMflt9aGDvi078e84eHlEXVGnh~eXzgUdjx3LaG-HY4ipp6~nV-C-maEfvgkdFw__&Key-Pair-Id=APKAJLOHF5GGSLRBV4ZA.
- Ajonina, G., Kairo, J., Grimsditch, G., Sembres, T., Chuyong, G., Mibog, D., Nyambane, A., FitzGerald, C., & Marine, K. (2014). Carbon pools and multiple benefits of mangroves in central Africa. Assessment for REDD+.
- Akbari, H. (2009) Cooling our communities. A guidebook on tree planting and light-colored surfacing.
- Altieri, M. A., & Koohafkan, P. (2008) Enduring farms: Climate change, smallholders and traditional farming communities Third World Network (TWN) Penang.
- Babbar, D., Areendran, G., Sahana, M., Sarma, K., Raj, K., & Sivadas, A. (2021). Assessment and prediction of carbon sequestration using Markov chain and InVEST model in Sariska Tiger reserve, India. Journal of Cleaner Production, 278, 123333. https://doi.org/10.1016/j.jclepro.2020.123333
- Bayu, T. (2022) Building-green space integration modeling for net-zero micro climate change in residential unit: In the case of Debre Birhan.
- Berhe, A. A., Harden, J. W., Torn, M. S., & Harte, J. (2008). Linking soil organic matter dynamics and erosion‐induced terrestrial carbon sequestration at different landform positions. Journal of Geophysical Research: Biogeosciences, 113(G4), 113. https://doi.org/10.1029/2008JG000751
- Bhattacharyya, S. S., Ros, G. H., Furtak, K., Iqbal, H. M., & Parra-Saldívar, R. (2022). Soil carbon sequestration–an interplay between soil microbial community and soil organic matter dynamics. Science of the Total Environment, 815, 152928. https://doi.org/10.1016/j.scitotenv.2022.152928
- Blanco-Canqui, H. (2013). Crop residue removal for bioenergy reduces soil carbon pools: How can we offset carbon losses? Bio Energy Research, 6(1), 358–371. https://doi.org/10.1007/s12155-012-9221-3
- Bogale, G. A., & Erena, Z. B. (2022). Drought vulnerability and impacts of climate change on livestock production and productivity in different agro-Ecological zones of Ethiopia. Journal of Applied Animal Research, 50(1), 471–489. https://doi.org/10.1080/09712119.2022.2103563
- Bolund, P., & Hunhammar, S. (1999). Ecosystem services in urban areas. Ecological Economics, 29(2), 293–301. https://doi.org/10.1016/S0921-8009(99)00013-0
- Carlo, T. A., Collazo, J. A., & Groom, M. J. (2004). Influences of fruit diversity and abundance on bird use of two shaded coffee plantations. Biotropica, 36(4), 602–614. https://doi.org/10.1111/j.1744-7429.2004.tb00354.x
- Chakravarty, S., Pala, N. A., Tamang, B., Sarkar, B. C., Manohar, K. A., Rai, P., Puri, A., & Shukla, G. (2019). Ecosystem services of trees outside forest. Sustainable Agriculture, Forest and Environmental Management, 327–352. https://link.springer.com/chapter/10.1007/978-981-13-6830-1_10
- Chatterjee, N., Nair, P. R., Nair, V. D., Bhattacharjee, A., Filho, E. D. M. V., Muschler, R. G., & Noponen, M. R. (2019). Do coffee agroforestry systems always improve soil carbon stocks deeper in the soil?—A case study from Turrialba, Costa Rica. Forests, 11(1), 49. https://doi.org/10.3390/f11010049
- Chavan, B., & Rasal, G. (2012). Total sequestered carbon stock of Mangifera indica. Journal of Environment & Earth Science, 2(1). .
- Choinova, V. (2023) Identifying data for horticulture categories to propose a standard carbon footprint model.
- Cooper, C. D., & Alley, F. C. (2010) Air pollution control: A design approach Waveland press.
- Dagar, J., & Yadav, R. (2017). Climate resilient approaches for enhancing productivity of saline agriculture. Journal of Soil Salinity and Water Quality, 9(1), 9–29.
- Davis, V. (2023). Impact of climate changes on agriculture and livestock. International Journal of Climatic Studies, 2(1), 28–39. https://doi.org/10.47604/ijcs.1829
- De Groot, R. S., Wilson, M. A., & Boumans, R. M. (2002). A typology for the classification, description and valuation of ecosystem functions, goods and services. Ecological Economics, 41(3), 393–408. https://doi.org/10.1016/S0921-8009(02)00089-7
- Deressa, T., Hassan, R. M., & Ringler, C. (2008) Measuring Ethiopian farmers’ vulnerability to climate change across regional states Intl food policy res inst.
- Dibaba, A., Soromessa, T., & Workineh, B. (2019). Carbon stock of the various carbon pools in Gerba-Dima moist afromontane forest, South-western Ethiopia. Carbon Balance and Management, 14(1), 1–10. https://doi.org/10.1186/s13021-019-0116-x
- Di Matteo, G., Luzzi, G., Basile, A., Sposato, A., Bertini, G., Neri, U., Pennelli, B., Napoli, R., & Nardi, P. (2023). Carbon concentrations and carbon storage capacity of three old-growth forests in the Sila National Park, Southern Italy. Journal of Forestry Research, 34(1), 233–242. https://doi.org/10.1007/s11676-022-01549-3
- Dong, X., Hao, Q., Li, G., Lin, Q., & Zhao, X. (2017). Contrast effect of long-term fertilization on SOC and SIC stocks and distribution in different soil particle-size fractions. Journal of Soils and Sediments, 17(4), 1054–1063. https://doi.org/10.1007/s11368-016-1615-y
- Dongmo, C. (2022). Resilience to environmental challenges and the national disaster insurance program in Kenya. Energy Policy Advancement: Climate Change Mitigation and International Environmental Justice, 145–161. https://link.springer.com/chapter/10.1007/978-3-030-84993-1_7
- Elbasiouny, H., El-Ramady, H., Elbehiry, F., Rajput, V. D., Minkina, T., & Mandzhieva, S. (2022). Plant nutrition under climate change and soil carbon sequestration. Sustainability, 14(2), 914. https://doi.org/10.3390/su14020914
- Eshetu, E. Y., Hailu, T. A., & Tejada Moral, M. (2020). Carbon sequestration and elevational gradient: The case of Yegof mountain natural vegetation in North East, Ethiopia, implications for sustainable management. Cogent Food & Agriculture, 6(1), 1733331. https://doi.org/10.1080/23311932.2020.1733331
- Eusterhues, K., Rumpel, C., Kleber, M., & Kögel-Knabner, I. (2003). Stabilisation of soil organic matter by interactions with minerals as revealed by mineral dissolution and oxidative degradation. Organic Geochemistry, 34(12), 1591–1600. https://doi.org/10.1016/j.orggeochem.2003.08.007
- Feng, J., He, K., Zhang, Q., Han, M., & Zhu, B. (2022). Changes in plant inputs alter soil carbon and microbial communities in forest ecosystems. Global Change Biology, 28(10), 3426–3440. https://doi.org/10.1111/gcb.16107
- Fikreyesus, D., Gizaw, S., Mayers, J., & Barrett, S. (2022) Mass tree planting: Prospects for a green legacy in Ethiopia.
- Fotis, A. T., Murphy, S. J., Ricart, R. D., Krishnadas, M., Whitacre, J., Wenzel, J. W., Queenborough, S. A., Comita, L. S., & Hector, A. (2018). Above‐ground biomass is driven by mass‐ratio effects and stand structural attributes in a temperate deciduous forest. Journal of Ecology, 106(2), 561–570. https://doi.org/10.1111/1365-2745.12847
- Friedlingstein, P., Jones, M. W., O’Sullivan, M., Andrew, R. M., Bakker, D. C., Hauck, J., Le Quéré, C., Peters, G. P., Peters, W., Pongratz, J., Sitch, S., Canadell, J. G., Ciais, P., Jackson, R. B., Alin, S. R., Anthoni, P., Bates, N. R., Becker, M. … Zaehle, S. (2022). Global carbon budget 2021. Earth System Science Data, 14(4), 1917–2005. https://doi.org/10.5194/essd-14-1917-2022
- Ganeshamurthy, A., Ravindra, V., & Rupa, T. (2019). Carbon sequestration potential of mango orchards in India. Current Science, 117(12), 2006–2013. https://doi.org/10.18520/cs/v117/i12/2006-2013
- Ganeshamurthy, A., Ravindra, V., Rupa, T., & Bhatt, R. (2019). Carbon sequestration potential of mango orchards in the tropical hot and humid climate of Konkan region, India. Current Science, 116(8), 1417–1423. https://doi.org/10.18520/cs/v116/i8/1417-1423
- Gebeyehu, G., Soromessa, T., Bekele, T., & Teketay, D. (2019). Carbon stocks and factors affecting their storage in dry afromontane forests of Awi zone, northwestern Ethiopia. Journal of Ecology and Environment, 43(1), 1–18. https://doi.org/10.1186/s41610-019-0105-8
- Gebre Mariam, S. (2003) Status of commercial fruit production in Ethiopia Ethiopian Agricultural Research Organization.
- Godard, O. (2017). Justice and climate change: Data and proposals: Proposals, arguments and justification, global climate Justice. Edward Elgar Publishing.
- Goswami, A., Krishna, M. M., Vankara, J., Gangadharan, S. M. P., Yadav, C. S., Kumar, M., Khan, M. M., & Doulamis, A. D. (2022). Sentiment analysis of statements on social media and electronic media using machine and deep learning classifiers. Computational Intelligence and Neuroscience 2022, 2022, 1–18. https://doi.org/10.1155/2022/9194031
- Gressel, J. (2008). Transgenics are imperative for biofuel crops. Plant Science, 174(3), 246–263. https://doi.org/10.1016/j.plantsci.2007.11.009
- Guidi Nissim, W., Castiglione, S., Guarino, F., Pastore, M. C., & Labra, M. (2023). Beyond cleansing: Ecosystem services related to phytoremediation. Plants, 12(5), 1031. https://doi.org/10.3390/plants12051031
- Guimarães, D. V., Gonzaga, M. I., & Melo Neto, J. D. O. (2014). Manejo da matéria orgânica do solo e estoques de carbono em cultivos de frutas tropicais. Revista Brasileira de Engenharia Agrícola e Ambiental, 18(3), 301–306. https://doi.org/10.1590/S1415-43662014000300009
- Gunamantha, I., Sudiana, I., Sastrawidana, D., Suryaputra, I., & Oviantari, M. (2021). The evaluation of soil fertility status of open space in campus area and their suitability for tropical fruits production. Journal of Soil Science and Environmental Management, 12(2), 78–85. https://doi.org/10.5897/JSSEM2021.0872
- Guyalo, A. K., Alemu, E. A., & Degaga, D. T. (2022). Impact of large-scale agricultural investments on the food security status of local community in Gambella region, Ethiopia. Agriculture & Food Security, 11(1), 1–28. https://doi.org/10.1186/s40066-022-00381-6
- Harris, S. H., & Betts, M. G. (2023). Selecting among land sparing, sharing, and triad in a temperate rainforest depends on biodiversity and timber production targets. Journal of Applied Ecology, 60(4), 737–750. https://doi.org/10.1111/1365-2664.14385
- Hundie, S. K. (2021). Income inequality, economic growth and carbon dioxide emissions nexus: Empirical evidence from Ethiopia. Environmental Science and Pollution Research, 28(32), 43579–43598. https://doi.org/10.1007/s11356-021-13341-7
- Hurd, C. L., Law, C. S., Bach, L. T., Britton, D., Hovenden, M., Paine, E. R., Raven, J. A., Tamsitt, V., & Boyd, P. W. (2022). Forensic carbon accounting: Assessing the role of seaweeds for carbon sequestration. Journal of Phycology, 58(3), 347–363. https://doi.org/10.1111/jpy.13249
- Jakšić, S., Ninkov, J., Milić, S., Vasin, J., Živanov, M., Jakšić, D., & Komlen, V. (2021). Influence of slope gradient and aspect on soil organic carbon content in the region of Niš, Serbia. Sustainability, 13(15), 8332. https://doi.org/10.3390/su13158332
- Janiola, M. D. C., & Marin, R. A. (2016). Carbon sequestration potential of fruit tree plantations in southern Philippines. Journal of Biodiversity and Environmental Science, 8(5), 164–174. 2220-6663.
- Jayathilake, H. M., Warren-Thomas, E., Nelson, L., Dolman, P., Bumrungsri, S., Juthong, W., Carrasco, L. R., & Edwards, D. P. (2021). Fruit trees and herbaceous plants increase functional and phylogenetic diversity of birds in smallholder rubber plantations. Biological Conservation, 257, 109140. https://doi.org/10.1016/j.biocon.2021.109140
- Jerikias, M., & Zakio, M. (2022). Climate change mitigation: In Situ management methods of indigenous fruit trees in chivi communal area, Masvingo Province, Zimbabwe, climate change adaptations in dryland Agriculture in semi-arid areas. Springer.
- Kassahun, K., Soromessa, T., & Belliethathan, S. (2015) Forest carbon stock in woody plants of Ades forest, Western Hararghe zone of Ethiopia and its variation along environmental factors: Implication for climate change mitigation. Forest 5.
- Kongsager, R., Napier, J., & Mertz, O. (2013). The carbon sequestration potential of tree crop plantations. Mitigation and Adaptation Strategies for Global Change, 18(8), 1197–1213. https://doi.org/10.1007/s11027-012-9417-z
- Kumar, B. M., & Kunhamu, T. (2021). Carbon sequestration potential of agroforestry systems in India: A synthesis. Agroforestry and Ecosystem Services, 389–430. https://link.springer.com/chapter/10.1007/978-3-030-80060-4_15
- Lakso, A., Wunsche, J., Palmer, J., & Corelli Grappadelli, L. (1999). Measurement and modeling of carbon balance of the apple tree. HortScience, 34(6), 1040. https://doi.org/10.21273/HORTSCI.34.6.1040
- Lal, R. (2023). Farming systems to return land for nature: It’s all about soil health and re-carbonization of the terrestrial biosphere. Farming System, 1, 100002. https://doi.org/10.1016/j.farsys.2023.100002
- Lyashenko, S., Gorbenko, O., Kelemesh, A., Kalinichenko, A., Stebila, J., & Patyka, V. (2022). Non-waste technology for utilization of tree branches. Applied Sciences, 12(17), 8871. https://doi.org/10.3390/app12178871
- Maleki, B. A. (2011). Shading: Passive cooling and energy conservation in buildings. International Journal on Technical and Physical Problems of Engineering (IJTPE), 3, 72–79.
- Manilay, A., Barbon, W. J., Cabriole, M. A., Myae, C., Thant, P. S., Gummadi, S., Monville-Oro, E., & Gonsalves, J. F. (2021) Financial and environmental benefits from fruit trees in Myanmar’s central dry zone: Case study from htee pu climate Smart village.
- Mathew, I., Shimelis, H., Mutema, M., Minasny, B., & Chaplot, V. (2020). Crops for increasing soil organic carbon stocks–A global meta analysis. Geoderma, 367, 114230. https://doi.org/10.1016/j.geoderma.2020.114230
- Mayer, M., Krause, H.-M., Fliessbach, A., Mäder, P., & Steffens, M. (2022). Fertilizer quality and labile soil organic matter fractions are vital for organic carbon sequestration in temperate arable soils within a long-term trial in Switzerland. Geoderma, 426, 116080. https://doi.org/10.1016/j.geoderma.2022.116080
- Melke, A., & Fetene, M. (2014). Apples (Malus domestica, Borkh.) phenology in Ethiopian highlands: Plant growth, blooming, fruit development and fruit quality perspectives. American Journal of Experimental Agriculture, 4(12), 1958. https://doi.org/10.9734/AJEA/2014/9783
- Mengesha Tadesse, M. (2023). Impact of climate change on crop and irrigation water requirement: The case of lake ziway catchment. Haramaya University.
- Mengistu, B., & Asfaw, Z. (2017). Comparative assessment of soil organic carbon stock potential under agroforestry practices and other land uses in lowlands of bale. International Journal of Environment, 6(3), 1–14. https://doi.org/10.3126/ije.v6i3.18094
- Mewded, B., & Lemessa, D. (2020). Factors affecting woody carbon stock in Sirso moist evergreen afromontane forest, southern Ethiopia: Implications for climate change mitigation. Environment Development and Sustainability, 22(7), 6363–6378. https://doi.org/10.1007/s10668-019-00483-5
- Meyfroidt, P., De Bremond, A., Ryan, C. M., Archer, E., Aspinall, R., Chhabra, A., Camara, G., Corbera, E., DeFries, R., & Díaz, S. (2022). Ten Facts about land systems for sustainability. Proceedings of the National Academy of Sciences, 119(7), e2109217118. https://doi.org/10.1073/pnas.2109217118
- Mng’omba, S. A., & Beedy, T. (2013). Positioning fruit trees into climate change/variability scenarios: Opportunities and constraints in the placement of fruit tree species in payment for environmental services. Scientific Research and Essays, 8(28), 1343–1348. https://doi.org/10.5897/SREX10.010
- Mohamed, A. A. (2017). Food security situation in Ethiopia: A review study. International Journal of Health Economics and Policy, 2, 86–96.
- Mohan, L., Chen, J., & Anderson, C. W. (2009). Developing a multi‐year learning progression for carbon cycling in socio‐ecological systems. Journal of Research in Science Teaching: The Official Journal of the National Association for Research in Science Teaching, 46(6), 675–698. https://doi.org/10.1002/tea.20314
- Momani, N., & Alzaghal, M. H. (2009). Early warning systems for disasters in Jordan: Current and future trends. Journal of Homeland Security and Emergency Management, 6(1). https://doi.org/10.2202/1547-7355.1663
- Montes-Londoño, I. (2017). Tropical dry forests in multi-functional landscapes: Agroforestry systems for conservation and livelihoods. Integrating Landscapes: Agroforestry for Biodiversity Conservation and Food Sovereignty, 47–78.
- Niguse, G., Iticha, B., Kebede, G., & Chimdi, A. (2022). Contribution of coffee plants to carbon sequestration in agroforestry systems of Southwestern Ethiopia. The Journal of Agricultural Science, 160(6), 1–8. https://doi.org/10.1017/S0021859622000624
- Olah, G. A., Goeppert, A., & Prakash, G. S. (2009). Chemical recycling of carbon dioxide to methanol and dimethyl ether: From greenhouse gas to renewable, environmentally carbon neutral fuels and synthetic hydrocarbons. The Journal of Organic Chemistry, 74(2), 487–498. https://doi.org/10.1021/jo801260f
- Oljirra, A. (2019). The causes, consequences and remedies of deforestation in Ethiopia. Journal of Degraded and Mining Lands Management, 6(3), 1747. https://doi.org/10.15243/jdmlm.2019.063.1747
- Osabohien, R., Matthew, O., Gershon, O., Ogunbiyi, T., & Nwosu, E. (2019). Agriculture development, employment generation and poverty reduction in West Africa. The Open Agriculture Journal 13, 13(1), 82–89. https://doi.org/10.2174/1874331501913010082
- Osberghaus, D., & Fugger, C. (2022). Natural disasters and climate change beliefs: The role of distance and prior beliefs. Global Environmental Change, 74, 102515. https://doi.org/10.1016/j.gloenvcha.2022.102515
- Oviantari, M., Sudiana, I., Sastrawidana, I., Suryaputra, I., & Gunamantha, I. (2021). Contribution of tropical fruit plants and soil properties to the potential of carbon sequestration in Open land utilization for mixed plantations, 4th International Conference on Innovative Research Across Disciplines (ICIRAD 2021) (pp. 47–56). Atlantis Press.
- Pandya, I. Y., Salvi, H., Chahar, O., & Vaghela, N. (2013). Quantitative analysis on carbon storage of 25 valuable tree species of Gujarat, incredible India. Indian Journal of Scientific Research, 4, 137–141.
- Patil, P., & Kumar, A. K. (2017). Biological carbon sequestration through fruit crops (perennial crops-natural “sponges” for absorbing carbon dioxide from atmosphere). Plant Archives, 17, 1041–1046.
- Plénet, D., Borg, J., Barra, Q., Bussi, C., Gomez, L., Memah, M.-M., Lescourret, F., & Vercambre, G. (2022). Net primary production and carbon budget in peach orchards under conventional and low input management systems. The European Journal of Agronomy, 140, 126578. https://doi.org/10.1016/j.eja.2022.126578
- Prasanna, D., & Selvaraj, V. (2016). Pt and pt-sn nanoparticles decorated conductive polymer-biowaste ash composite for direct methanol fuel cell. Korean Journal of Chemical Engineering, 33(4), 1489–1499. https://doi.org/10.1007/s11814-015-0245-1
- Rahiel, H. A., Zenebe, A. K., Leake, G. W., & Gebremedhin, B. W. (2018). Assessment of production potential and post-harvest losses of fruits and vegetables in northern region of Ethiopia. Agriculture & Food Security, 7(1), 1–13. https://doi.org/10.1186/s40066-018-0181-5
- Rahman, M. S., Huang, W.-C., Toiba, H., & Efani, A. (2022). Does adaptation to climate change promote household food security? Insights from Indonesian fishermen. International Journal of Sustainable Development & World Ecology, 29(7), 611–624. https://doi.org/10.1080/13504509.2022.2063433
- Ramirez, F., & Kallarackal, J. (2015). Responses of fruit trees to global climate change. Springer.
- Reicosky, D. (2003). Conservation agriculture: Global environmental benefits of soil carbon management. Conservation Agriculture: Environment, Farmers Experiences, Innovations, Socio-Economy, Policy, 3–12. https://link.springer.com/chapter/10.1007/978-94-017-1143-2_1
- Reyes-Martín, M. P., Ortiz-Bernad, I., Lallena, A. M., San-Emeterio, L. M., Martínez-Cartas, M. L., & Ondoño, E. F. (2021). Reuse of pruning waste from subtropical fruit trees and urban gardens as a source of nutrients: Changes in the physical, chemical, and biological properties of the soil. Applied Sciences, 12(1), 193. https://doi.org/10.3390/app12010193
- Roshetko, J. M., Delaney, M., Hairiah, K., & Purnomosidhi, P. (2002). Carbon stocks in Indonesian homegarden systems: Can smallholder systems be targeted for increased carbon storage? American Journal of Alternative Agriculture, 17(3), 138–148. https://doi.org/10.1079/AJAA200116
- Salgueiro, L., Martins, A., & Correia, H. (2010). Raw materials: The importance of quality and safety. A review. Flavour and Fragrance Journal, 25(5), 253–271. https://doi.org/10.1002/ffj.1973
- Scandellari, F., Caruso, G., Liguori, G., Meggio, F., Palese, M., Zanotelli, D., Celano, G., Gucci, R., Inglese, P., Pitacco, A., & Tagliavini, M. (2016). A survey of carbon sequestration potential of orchards and vineyards in Italy. European Journal of Horticultural Science, 81(2), 106–114. https://doi.org/10.17660/eJHS.2016/81.2.4
- Seid, Y. M. (2022). Biomass and soil carbon stocks of selected agroforestry practice and cultivated land, in Tehuledere District. https://doi.org/10.21203/rs.3.rs-1935909/v1
- Sharmake, M. A., Sultan, K., Hussain, R., Rehman, A., & Hussain, A. (2023). Decadal impacts of climate change on Rainfed Agriculture community in Western Somaliland, Africa. Sustainability, 15(1), 421. https://doi.org/10.3390/su15010421
- Sharma, S., Rana, V. S., Prasad, H., Lakra, J., & Sharma, U. (2021a). Appraisal of carbon capture, storage, and utilization through fruit crops. Frontiers in Environmental Science, 9, 700768. https://doi.org/10.3389/fenvs.2021.700768
- Sharma, S., Rana, V. S., Prasad, H., Lakra, J., & Sharma, U. (2021b). Appraisal of carbon capture, storage, and utilization through fruit crops. Frontiers in Environmental Science, 9, 258. https://doi.org/10.3389/fenvs.2021.700768
- Shiferaw, H., Kassawmar, T., & Zeleke, G. (2022). Above and belowground woody-biomass and carbon stock estimations at Kunzila watershed, Northwest Ethiopia. Trees, Forests and People, 7, 100204. https://doi.org/10.1016/j.tfp.2022.100204
- Shivanna, K. (2022). Climate change and its impact on biodiversity and human welfare. Proceedings of the Indian National Science Academy 88, 160–171.
- Siarudin, M., Rahman, S. A., Artati, Y., Indrajaya, Y., Narulita, S., Ardha, M. J., & Larjavaara, M. (2021). Carbon sequestration potential of agroforestry systems in degraded landscapes in west java, Indonesia. Forests, 12(6), 714. https://doi.org/10.3390/f12060714
- Sinare, H., Peterson, G., Börjeson, L., & Gordon, L. (2022). Ecosystem services in Sahelian village landscapes 1952–2016: estimating change in a data scarce region. Ecology and Society, 27(3). https://doi.org/10.5751/ES-13292-270301
- Singh, A. K. (2020) Climate change vulnerability and Adaptation in the livestock sector in Ethiopia orangebooks publication.
- Singh, S. L., Sahoo, U. K., Kenye, A., & Gogoi, A. (2018). Assessment of growth, carbon stock and sequestration potential of oil palm plantations in Mizoram, Northeast India. Journal of Environmental Protection, 09(9), 912. https://doi.org/10.4236/jep.2018.99057
- Somasundaram, J., Reeves, S., Wang, W., Heenan, M., & Dalal, R. (2017). Impact of 47 years of no tillage and stubble retention on soil aggregation and carbon distribution in a vertisol. Land Degradation & Development, 28(5), 1589–1602. https://doi.org/10.1002/ldr.2689
- Song, R., Zhu, Z., Zhang, L., Li, H., & Wang, H. (2023). A simple method using an allometric model to quantify the carbon sequestration capacity in vineyards. Plants, 12(5), 997. https://doi.org/10.3390/plants12050997
- Stavi, I., Roque de Pinho, J., Paschalidou, A. K., Adamo, S. B., Galvin, K., de Sherbinin, A., Even, T., Heaviside, C., & van der Geest, K. (2022). Food security among dryland pastoralists and agropastoralists: The climate, land-use change, and population dynamics nexus. The Anthropocene Review, 9(3), 299–323. https://doi.org/10.1177/20530196211007512
- Tesfay, H. M., Negash, M., Godbold, D. L., & Hager, H. (2022). Assessing carbon pools of three indigenous agroforestry systems in the Southeastern Rift-Valley landscapes, Ethiopia. Sustainability, 14(8), 4716. https://doi.org/10.3390/su14084716
- Thornton, P. K., Ericksen, P. J., Herrero, M., & Challinor, A. J. (2014). Climate variability and vulnerability to climate change: a review. Global Change Biology, 20(11), 3313–3328. https://doi.org/10.1111/gcb.12581
- Tirado, M., Hunnes, D., Cohen, M., & Lartey, A. (2015). Climate change and nutrition in Africa. Journal of Hunger & Environmental Nutrition, 10(1), 22–46. https://doi.org/10.1080/19320248.2014.908447
- Torn, M. S., Trumbore, S. E., Chadwick, O. A., Vitousek, P. M., & Hendricks, D. M. (1997). Mineral control of soil organic carbon storage and turnover. Nature, 389(6647), 170–173. https://doi.org/10.1038/38260
- Trumbore, S. E. (1997). Potential responses of soil organic carbon to global environmental change. Proceedings of the National Academy of Sciences (Vol. 94, pp. 8284–8291).
- Ukpanyang, D., & Terrados-Cepeda, J. (2022). Decarbonizing vehicle transportation with hydrogen from biomass gasification: An Assessment in the Nigerian urban Environment. Energies, 15(9), 3200. https://doi.org/10.3390/en15093200
- Uning, R., Latif, M. T., Othman, M., Juneng, L., Mohd Hanif, N., Nadzir, M. S. M., Abdul Maulud, K. N., Jaafar, W. S. W. M., Said, N. F. S., Ahamad, F., & Takriff, M. S. (2020). A review of Southeast Asian oil palm and its CO2 fluxes. Sustainability, 12(12), 5077. https://doi.org/10.3390/su12125077
- Vacek, Z., Vacek, S., & Cukor, J. (2023). European forests under global climate change: Review of tree growth processes, crises and management strategies. Journal of Environmental Management, 332, 117353. https://doi.org/10.1016/j.jenvman.2023.117353
- Vashum, K. T., & Jayakumar, S. (2012). Methods to estimate above-ground biomass and carbon stock in natural forests-a review. Journal of Ecosystem & Ecography, 2(4), 1–7. https://doi.org/10.4172/2157-7625.1000116
- Wang, L., Zheng, J., Wang, G., Dang, Q.-L., & Tissue, D. (2023). Combined effects of elevated CO2 and warmer temperature on limitations to photosynthesis and carbon sequestration in yellow birch. Tree Physiology, 43(3), 379–389. https://doi.org/10.1093/treephys/tpac128
- Wassie, S. B. (2020). Natural resource degradation tendencies in Ethiopia: A review. Environmental Systems Research, 9(1), 1–29. https://doi.org/10.1186/s40068-020-00194-1
- Wheeler, T., & Von Braun, J. (2013). Climate change impacts on global food security. Science, 341(6145), 508–513. https://doi.org/10.1126/science.1239402
- Wittwer, S. H. (1995) Food, climate, and carbon dioxide: The global environment and world food production CRC press.
- Wu, T., Wang, Y., Yu, C., Chiarawipa, R., Zhang, X., Han, Z., Wu, L., & Bernacchi, C. J. (2012). Carbon sequestration by fruit trees-Chinese apple orchards as an example. PloS One, 7(6), e38883. https://doi.org/10.1371/journal.pone.0038883
- Xu, S., Sayer, E. J., Eisenhauer, N., Lu, X., Wang, J., & Liu, C. (2021). Aboveground litter inputs determine carbon storage across soil profiles: A meta-analysis. Plant and Soil, 462(1–2), 429–444. https://doi.org/10.1007/s11104-021-04881-5
- Yasin, G., Farrakh Nawaz, M., Zubair, M., Qadir, I., Saleem, A. R., Ijaz, M., Gul, S., Amjad Bashir, M., Rehim, A., Rahman, S. U., & Du, Z. (2021). Assessing the contribution of citrus orchards in climate change mitigation through carbon sequestration in Sargodha District, Pakistan. Sustainability, 13(22), 12412. https://doi.org/10.3390/su132212412
- Yulistyarini, T., & Hadiah, J. (2022). Carbon stock potential of Indonesian local fruit trees, some collections of Purwodadi Botanic garden, IOP Conference Series: Earth and Environmental Science (pp. 012057). IOP Publishing. https://doi.org/10.1088/1755-1315/976/1/012057
- Zahoor, S., Dutt, V., Mughal, A., Pala, N. A., Qaisar, K., & Khan, P. (2021). Apple-based agroforestry systems for biomass production and carbon sequestration: Implication for food security and climate change contemplates in temperate region of Northern Himalaya, India. Agroforestry Systems, 95(2), 367–382. https://doi.org/10.1007/s10457-021-00593-y
- Zhang, Q., Gao, B., Tian, M., Shi, H., Hua, X., & Wang, M. (2016). Enantioseparation and determination of triticonazole enantiomers in fruits, vegetables, and soil using efficient extraction and clean-up methods. Journal of Chromatography Biomedical Sciences and Applications, 1009, 130–137. https://doi.org/10.1016/j.jchromb.2015.12.018
- Zhan, Y., Yao, Z., Groffman, P. M., Xie, J., Wang, Y., Li, G., Zheng, X., & Butterbach‐Bahl, K. (2023). Urbanization can accelerate climate change by increasing soil N2O emission while reducing CH4 uptake. Global Change Biology.
- Zsögön, A., Peres, L. E., Xiao, Y., Yan, J., & Fernie, A. R. (2022). Enhancing crop diversity for food security in the face of climate uncertainty. The Plant Journal: For Cell and Molecular Biology, 109(2), 402–414. https://doi.org/10.1111/tpj.15626