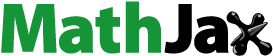
Abstract
Wheat is the most important cereal crop in the world. It contributes as a major source of protein and calories in the daily human diet. Drought has become a major abiotic stress that severely affects wheat production globally. Changing rainfall patterns, increased atmospheric CO2 levels, rises in atmospheric temperature and hot and dry winds are the major causes of drought stress. It has morphological, physiological, and biochemical consequences such as reduced yield performance, yield attributing parameters, germination, and seed vigor, early leaf senescence, early maturity, decreased chlorophyll content, decreased Rubisco activity, decreased photosynthesis, and decreased starch accumulation. Drought produces reactive oxygen species that cause oxidative damage to the plants leading to programmed cell death. Wheat plant has developed various tolerance mechanisms such as drought escape, avoidance, and tolerance to protect them from drought. Increasing trichome density and leaf waxiness, root: shoot ratio, stay green, accumulation of proline, production of various enzymes viz; superoxide dismutase (SOD), ascorbate (APX), peroxidase (POD), catalase (CAT), osmotic adjustment, ABA accumulation, and formation of dehydrins leads to drought tolerance. Screening of the various genotypes for the identification of novel trait combinations, genetic engineering, and transgenic approaches (incorporates, transfer, and introduces the desirable gene into the desired plants) and thereby the adaptable varieties can be selected and induced to develop new varieties with desired characteristics would be a major genetic management strategies to overcome drought, increase production, and ensure the food and nutritional security of the world.
REVIEWING EDITOR:
1. Introduction
Wheat (Triticum aestivum L.) is the most important, highly nutritious, widely grown, and most consumed cereal in the world (Arzani & Ashraf, Citation2017). Wheat is cultivated in 220 million hectares area in the world. Wheat has an annual production of 778 million metric tons with a 10% value addition in agriculture (Kamal et al., Citation2020). It has an average annual productivity of 3.5 tons/ha. It provides 1.8% fiber, 9.4% protein, 69% carbohydrates, and 2.5% fat (Ahmad et al., Citation2022). Increasing food and nutritional demand due to population growth makes the wheat crop’s importance even more (Hussain & Jatoi, Citation2021). It has also a major contribution to the animal feed and livestock industry with high historical and cultural significance. The average annual wheat production has increased by 2% to acquire the future food demand imposed by population growth globally (Doležel, Citation2010).
Abiotic stresses are the major yield-limiting factor in the area of low water availability for production in arid and semi-arid regions (Bhandari et al., Citation2021; Lehari et al., Citation2019). Trends on global climate change show a long-term alternation in temperature and precipitation patterns on earth experiencing the warmness, environmental disasters such as abiotic and biotic stresses, rise in sea level, and melting of glacier caps. Drought stress is one of the major abiotic stresses that farmers are facing all over the world as a result of global climate change (Mansour et al., Citation2020). Globally, only 346,895 thousand hectares of land area is under irrigation and the rest fully depends upon the natural rainfall. Annually, the temperature of the earth rises at the rate of 0.06 with a decline in precipitation of 16.09 mm (Paudel et al., Citation2020). It is predicted that about 1.8 billion people (65%) of the world’s population will face an absolute water shortage and be forced to live under water-stressed conditions by 2025 (Narwal, Citation2015). Wheat crops require 300–500 mm water which is much higher as compared to the water requirement of other crops like maize (Poudel et al., Citation2020). In developing countries, lack of irrigation was the major cause for the lower production (14% less) of wheat as compared to developed countries (Shiferaw et al., Citation2013). Drought reduces the yield of wheat by an average of 50–60% (Zhao et al., Citation2020). If the trends continue, it will further decline the production of wheat threatening the future food and nutritional security of the world (Mansour et al., Citation2020). Drought not only reduces production, it also facilitates soil erosion as well as environmental degradation (Fang & Xiong, Citation2015). Drought is the key issue but there was a lack of proper study on this matter.
By 2025, about 1.8 billion people are estimated to suffer from an absolute food deficit (El-Mouhamady et al., Citation2023). The shortage of water and aggravation in yield are parallel with the excessive increase in population (El-Mouhamady et al., Citation2023). In 1961, 3.5 billion people around the world fed on 1.36 billion hectares of land. After a half-century, the population of the world has doubled but the area under cultivation increased only by 12–13% (FAOSTAT, Citation2022). Therefore, increasing production through the increasing area is almost impossible. A sustainable increase in production is a prerequisite to meet the current and future food demands of the world. There was a prior need to increase yield by 50% to meet the food requirement of the global population to end hunger and malnutrition (Poudel et al., Citation2020). Plant species with narrow genetic diversity suffer from yield losses due to various factors such as abiotic stresses. Therefore, drought tolerance breeding using novel genetic resources is the most important strategy for sustainable wheat production and overcoming the changing climatic conditions all over the world (Hussain & Jatoi, Citation2021; Mwadzingeni et al., Citation2016). Grain yield is the main criterion that can be used for drought tolerance. Overall, drought stress has a complex effect on morphology, physiology, and biochemistry leading to growth retardation and productivity of wheat. Thus, the major objective of this study is an approach that aims to study drought stress, its causes, effects, tolerance, and management in wheat that helps plant breeders search for various ways that can help to combat or ameliorate the effect of drought stress in plants, especially food crops, and to ensure food security worldwide.
2. Results and discussion
2.1. Causes of drought stress
Climate change-induced alternations in rainfall patterns, increased atmospheric CO2 levels, rises in atmospheric temperature (Arbona et al., Citation2013; Dai, Citation2013; Nezhadahmadi et al., Citation2013), and hot and dry winds (M. A. Hossain et al., Citation2016) were the major causes of drought stress. The temperature of the earth is increasing at the rate of 0.06 with a 16.09 mm decline in precipitation annually (Paudel et al., Citation2020). The plant may experience water stress, even when there is sufficient moisture in the soil, due to various edaphic factors. These factors include salinity, low soil temperatures, and flooding, which hinder the absorption of water by the plant’s roots. This condition is referred to as pseudo drought or physiological drought (Arbona et al., Citation2013; Lisar et al., Citation2012).
2.2. Effect of drought on morphology, physiology, and biochemistry of wheat
In plants, depending upon the time, stage, and severity of water stress, drought stress causes various morphological, physiological, biochemical, and molecular abnormalities leading to a reduction in the growth and development of crops (Chachar et al., Citation2016). Tillering, jointing, booting, anthesis, and grain filling stages are the most critical stages in response to drought. Drought stress at these stages can cause yield loss of up to 69% (Khan et al., Citation2023) ().
2.2.1. Effect of drought stress on wheat morphology
Drought induces major consequences such as reduced germination percentage, seed vigor, seedling vigor, leaf area, early leaf senescence, decreased dry weight, increased root-shoot ratio, and early maturity (Anjum et al., Citation2017). Germination of seeds plays a crucial role in crop growth, establishment, and yield (Almansouri et al., Citation2001). Soil moisture deficit hinders or delays in germination of seed which ultimately leads to uneven seedling distribution, plant population, establishment, and reduced grain yield production of wheat (Hampson & Simpson, Citation1990; A. Hossain et al., Citation2012). The germination percentage of wheat was reduced by 9.4% with 1.91–2.05 days delayed in germination under drought stress (Abdoli & Saedi, Citation2012). Lower water potential under drought was the main cause for the reduction in germination percentage (Ahmad et al., Citation2022; Jungang et al., Citation2002) and seedling vigor index under drought stress as compared to normal conditions (La & Sylvestris, Citation1990). The seedling vigor index was reduced by 16.6–21.26% under drought. Lack of water reduces the water intake, delayed germination, improper root and shoot growth, nutrient uptake, and oxidative stresses lead to poor seedling vigor index. Drought reduces the leaf area index due to the aging of the leaves, shading, and competition for light (Dalirie et al., Citation2010). Drought induces lower cell enlargement of leaves (McCree & Davis, Citation1974), reduced growth (Jones et al., Citation1980), and increased abscission (Qadir et al., Citation1998) leading to a reduction in leaf area index (LAI). Lack of water decreases the nitrogen uptake by the plants which causes the remobilization of nitrogen from leaves and stems to seeds causing early leaf senescence (Hajibarat & Saidi, Citation2022). Significant decrease in photosynthesis, reduced cell growth and expansion, less nutrient uptake, increased senescence, and rapid increase in stress hormones like abscisic acid (ABA) under drought decreases the dry matter accumulation. The plants in the continued search for water proliferate their roots in the soil which helps plants to uptake more water from deep down in the soil under drought stress conditions leading to an increase in the root-to-shoot ratio (Anjum et al., Citation2017; Kou et al., Citation2022).
Drought stress had a significant effect on morphological as well as phenological parameters such as plant height (Ph), tillers per plant (TPH), number of spikes per meter square (NSPMS), number of spikelets per spike (NSPS), and spike length (SL). The average plant height under drought stress was found to be reduced (Poudel et al., Citation2020). The significant decrease in plant height may be due to the dehydration of protoplasm that ultimately reduces cell division, cell expansion, and loss of cell turgidity (Salam et al., Citation2022). The average number of spikes per meter square (NSPMS) under drought stress is lower as compared to irrigated conditions is 340.025 (Poudel et al., Citation2020). Lack of water decreases water potential in plants during the vegetative phase causing tiller mortality leading to a decrease in NSPMS (Palmer & Steer, Citation1985). Drought stresses decrease the number of spikelets per spike (NSPS) by 9.5% as compared to irrigated conditions (Tatar et al., Citation2016). Lack of water decreases pollen fertility and increases the number of infertile spikelets and ultimately a decrease in NSPS (Grandgirard et al., Citation2002; Kaveh & Chayjan, Citation2014; Rawtiya & Kasal, Citation2021). Spike length was found to be reduced under drought conditions (Mohammed & Kadhem, Citation2017). A significant decrease in plant height under drought stress decreases the spike length (Mohammed & Kadhem, Citation2017). Grains per spike reduce by an average of 15.32% under drought stress (Poudel et al., Citation2020). Sterile pollen and underdeveloped ovules due to water deficit affect the fertilization of eggs which leads to a decrease in grains number per spike under drought stress (Manjarrez-Sandoval et al., Citation1989; Rawtiya & Kasal, Citation2021). Drought decreases 10.6% thousand kernel weight (TKW) as compared to irrigated conditions (Poudel et al., Citation2020). Lack of water decreases nutrient uptake, reduces photosynthesis, decreases photosynthate translocation, and accelerates early senescence, and early maturity resulting in a significant decrease in TKW (Rawtiya & Kasal, Citation2021). Drought stress also had a severe effect on phenological traits such as days to booting (DTB), days to heading (DTH), and days to anthesis (DTA) (Ahmad et al., Citation2022). An average reduction in DTB, DTH, and DTA of wheat was 4.10%, 6.78%, and 6.22%, respectively under drought stress conditions as compared to irrigated conditions (Chowdhury et al., Citation2021). The wheat plant develops a mechanism and completes its lifecycle as early as possible to escape from drought which reflects the overall decrease in DTB, DTH, and DTA, respectively (Chowdhury et al., Citation2021).
2.2.2. Effect of drought stress on wheat physiology
Drought stress has a severe effect on grain yield via disruption of various physiological activities such as reduced nutrient assimilation, mobilization, stem reserve accumulation, gametogenesis, fertilization, embryogenesis, endosperm development, and seed growth (Çakir, Citation2004). Drought stress reduces relative water content, chlorophyll content, osmotic potential, leaf water potential, leaf turgor potential as well as leaf diffusive resistance, and decreases the leaf transpiration rate (Ahmad et al., Citation2022). Drought reduces mineralization, diffusion, and mass-flow of nutrients in the soil thus reducing the nutrient assimilation (Barbara et al., Citation2007; Fierer & Schimel, Citation2002). Drought reduces the cell membrane permeability, transpiration flux, and active transport resulting in reduced nutrient mobilization in the plants (Hu & Schmidhalter, Citation2005). Low water potential in the plants results in reduced size of the plant parts such as stem and leaves for the accumulation of the reserve food materials (Wu & Ying-Ning, Citation2019). In plants, the gamete formation stage is highly sensitive to abiotic stress such as drought and heat stress. The reproductive organs exposed to such stresses showed meiotic defects due to the morphological, structural, and metabolic alterations that lead to premature gametes and reproductive sterility (Storme & Geelen, Citation2014). Drought reduces the moisture requirements in the reproductive parts such as style and stigma and reduces pollen viability leading to poor fertilization. Drought stress reduces the plant’s ability to uptake water from the soil which leads to reduced turgor pressure. Low turgor pressure leads to the reduction in the growth of plants through decreasing cell extensibility and also retards embryo and embryogenesis (B. Sharma et al., Citation2022). Drought causes incomplete seed filling therefore there would not be the proper seed growth, and seed size becomes small with lower germination capacity. Plants protect themselves against drought by closing the stomata to reduce water loss through transpiration (Anosheh et al., Citation2016). Drought affects photosystem II by slowing electron transport, increasing the ability of non-photo chemicals, and ultimately lowering the relative moisture content in the cell (Zivcak et al., Citation2013). Relative water content under drought stress decreased on average by 33.25% (Maghsoudi et al., Citation2016). A decrease in leaf water potential has a direct effect on the gaseous exchange via stomatal conductance and transpiration (Ahmad et al., Citation2018; Vesala et al., Citation2017). Closing of stomata leads to a lower supply of CO2 for photosynthesis and a decrease in photosynthetic rate (Ahmad et al., Citation2018). The drought had a direct effect on the availability of Ribulose 1,5-bisphosphate carboxylase/oxygenase (Rubisco) and also decreased the production of ATP (Farooq et al., Citation2009).
Drought damages the photosynthetic apparatus and alters the activity of various enzymes such as Rubisco involved in the Calvin cycle, which causes a decrease in atmospheric carbon fixation and conversion into organic products, ultimately reducing the grain yield (Sharifi & Mohammadkhani, Citation2016). In wheat, the flowering stage has the highest chlorophyll content (Raza, Citation2012) which significantly reduces under drought stress and leads to a decrease in leaf photosynthesis (Kura-Hotta et al., Citation1987; Prasad et al., Citation2011). Chlorophyll content decreases by 13–15% under drought stress as compared to irrigated conditions due to activation of chlorophyllase and enzyme inactivation (Nikolaeva et al., Citation2010). Reactive oxygen species produced under drought stress damage the chloroplast resulting in decreased production of chlorophyll content in plants (Mafakheri et al., Citation2010). In wheat, meiosis and micro sporogenesis stages of pollen development are highly sensitive to drought leading to pollen sterility that results in poor fertilization and a decrease in grain yield (J. Yu et al., Citation2019; Z. Zhang et al., Citation2021). Reactive oxygen species (ROS) were produced during photosynthesis and respiration of the plants. Release of surplus electrons to oxygen under drought reacts with proteins, lipids, membranes, etc. leading to oxidative damage in plant cell organelles that is; chloroplast, mitochondria, nucleic acids, changes in membrane potential, membrane lipid, and metabolic enzymes, deactivating their normal functions leads to the programmed cell death (Hasanuzzaman et al., Citation2020; Mubarik, Citation2021; R. Singh et al., Citation2016). Drought stress triggers the production of plant stress hormones, mostly abscisic acid (ABA) that play an important role in stomata activity in response to water stress with positive and negative effects such as the closure of stomata under water stress that leads to a conservation of water whereas, retards crop growth under drought as a negative consequences.
2.2.3. Effect of drought stress on wheat biochemistry
Drought stress decreases photochemical efficiency, increases the production of reactive oxygen species (ROS), increases oxidative damage, decreases starch accumulation, increases abscisic acid production, production of proline, increases in antioxidant enzymes, and decreases in cytokinins (B. Sharma et al., Citation2022). The rhizosphere of wheat has a higher rate of respiratory carbon loss under drought which reduces ATP production (Akter & Rafiqul Islam, Citation2017). Drought stress also induces the formation of superoxide and hydrogen peroxide (H2O2) (Reddy et al., Citation2004). Drought stress during the vegetative stages of the plants decreases the chlorophyll content, and efficiency of PSII photochemistry and increases the non-photochemical quenching resulting in the decreasing photochemical efficiency of plants (Y. Li et al., Citation2019). In plants, energy (ATP) is produced in mitochondria through the process of oxidative phosphorylation, involving the transport of protons through the electric transport chain. The oxygen molecule is the final destination of the electron transport through the series of proteins with oxidation-reduction reactions in the electron transport chain. Under drought stress, only 0.1–2% of electrons pass through the electron transport chain oxygen molecule reduced incompletely to form superoxide radical (•O−2) whereas, oxygen is reduced to water under normal conditions (X. Li, Citation2013). Under drought stress, plant roots first receive water-limiting signals that are transferred to leaves through xylem sap. The major stress signal produced during drought stress in plants is abscisic acid (Hartung et al., Citation2002; Jiang & Hartung, Citation2007; J. Zhang & Davies, Citation2023). Higher abscisic acid production reduces the cytokinin contents in plants (J. Zhang & Davies, Citation2023). Proline content increases under water stress and quickly dissipates due to the difference in cytosolic synthesis and mitochondrial breakdown rates (Kiyosue et al., Citation1996). Exposure to the drought for a long period leads to acclimation reactions leading to the production of antioxidant enzymes (Ajithkumar & Panneerselvam, Citation2014; He et al., Citation2017). Wheat grain composition including starch protein, gliadins, glutenin, and fibers were significantly affected by drought stress (Rakszegi et al., Citation2019). Starch is synthesized by the amyloplast of endosperm composed of glucose polymers namely, amylose and amylopectin (X. Yu, Citation2015). Drought can reduce the starch content up to 45% as compared to irrigated conditions when exposed to drought for 10 days between anthesis and harvesting (Worch et al., Citation2011). Soluble starch synthase (SSS) activity, Granule-bound starch synthase (GBSS) activity, Starch branching enzyme (SBE) activity, and Starch debranching enzyme (DBE) activity were the key enzymes for starch accumulation in wheat (Yi et al., Citation2014). Drought stress during the flowering stage of wheat significantly decreases the activity of these enzymes SSS, GBSS, SBE, and DBE which inhibits the starch synthesis activity at different levels (Bing, Citation2014; Lu et al., Citation2019). The baking quality of the wheat depends upon the protein content in the grains (Zörb et al., Citation2018). Drought stress has a great influence on the grain protein concentration which would alter the baking quality (Zörb et al., Citation2018) (V et al., Citation2019). observed the significant effect of drought stress on starch accumulation.
2.3. Drought tolerance mechanisms in wheat
In general, plants defend themselves against drought mainly through three mechanisms including drought escape, avoidance, and tolerance (). Plants develop an adaptive mechanism to escape the drought through which they rapidly complete their life cycle before starting the drought period due to which plants mature as early as compared to normal conditions (Shavrukov et al., Citation2017). The drought avoidance mechanism maintains the turgor pressure through the deep spread of roots, reduces water loss through stomatal transpiration, and preserves higher water potential in plants even when there is a lack of sufficient amount of water in the soil (Kandel, Citation2021). Drought tolerance mechanisms protect plants themselves through osmotic adjustment which maintains turgor, and results in increasing elasticity and reduction in cell size (Kandel, Citation2021).
2.3.1. Morphological tolerance mechanisms
Deep spread of the roots into the soil, leaf waxiness and trichome density, and sunken stomata on the lower side of leaves are the major morphological characteristics of wheat that reduce the loss of water through transpiration and help plants to maintain a higher water potential, and protect against drought for longer periods (Kvgk & G, Citation2019). The size and length of the roots increase in the continued search for water whereas the above-ground parts show restricted growth under water stress conditions that increase the root: shoot ratio (Ahmad et al., Citation2018). Drought stress causes the early senescence of leaves of wheat. The ability of the plants to remain photosynthetically active or resist against early senescence of leaves is said to be the stay-green defense mechanism in wheat (De Simone et al., Citation2014). Stay green property sustains the carbon assimilation, continues the supply of sugar in the growing reproductive organs, and helps to retain the pollen and ovule viability (Ruan, Citation2014), remobilization of the reserve to grain (Sperry et al., Citation2016), and helps to increase production under drought (Kandel, Citation2021). Therefore, the selection of drought-tolerant genotypes can also be done through the stay-green trait of wheat under drought-stress conditions (Farooq et al., Citation2014).
2.3.2. Physiological tolerance mechanisms
Physiological activities such as osmotic adjustment, ABA accumulation, closure of stomata, membrane integrity, and formation of dehydrins maintain higher water potential and lead to drought tolerance (Lambin et al., Citation2001). Osmotic adjustment produces various types of organic solutes that help to detoxify ROS, stabilize membranes, and structures of enzymes and proteins, and help in tolerance mechanisms against drought (Blum, Citation2017). Abscisic acid (ABA) produced during a stressed environment acts as a defense factor against it and it can be used as a selection index for drought tolerance (Finkelstein et al., Citation2002). Drought tolerance is the mechanism of wheat to adapt under water deficit conditions (Huang et al., Citation2017). Drought stress conditions create lower water content in the soil. Plants improve the drought tolerance mechanism through osmotic adjustment that accumulates various organic and inorganic solutes and maintains the cell turgor that allows cell expansion, growth, and development under drought-stress conditions (Aroca, Citation2013). This permits the CO2 fixation through stomata resulting in the accumulation of the osmolytes. Management of dehydration and cell membrane integrity due to osmolyte accumulation enhances the tolerance of wheat against drought stress (Ali et al., Citation2020). Various scientists consider membrane integrity to have a relationship with drought tolerance and it is an important parameter to resist the damage imposed under drought stress (Deshmukh et al., Citation1991; Premachandra et al., Citation1990). The production of cysteine has an important role in signaling pathways of plant stress, growth, and development. It is formed on the leaves of stressed plants that are involved in proteolytic activity. It has a negative role in drought tolerance (Wiśniewski & Zagdańska, Citation2001). Transpiration efficiency (TE) is an indispensable phenomenon in plants that varies according to growing conditions. Cultivars with high TE are tolerant to drought stress and vice versa (Nezhadahmadi et al., Citation2013).
2.3.3. Biochemical tolerance mechanisms
Under drought stress conditions there was an accumulation of metabolites such as glycine, betaine, proline, mannitol, and soluble sugars that help to protect the plant against drought (Ozturk et al., Citation2021). Glycine regulates the mRNA stability of ROS scavenging-related genes (Shim et al., Citation2021). Betaine protects the biological membranes from ROS under drought by improving the tissue water status (Zohaib et al., Citation2018). Proline acts as an osmoregulator that helps in ROS detoxification, membrane integrity protection, proposed suitable osmolyte, a mechanism to store carbon and nitrogen, and also helps in stress tolerance mechanism (B. Sharma et al., Citation2022). An increase in the proline content under stress conditions improves tolerance through the control storage of useful nitrogen, increases membrane stability, scavenging free radicals, and buffers cellular redox potential (Bandurska et al., Citation2017). Increased soluble sugars and proline under water stress conditions were the potential contributors to osmotic adjustment (Sánchez et al., Citation1998). Proline accumulates in larger than other amino acids (A. Kumar et al., Citation2018). In various plant species under water deficit conditions, hydroxyl radicals were generated that were scavenged by mannitol and enhanced drought tolerance. Under drought stress, soluble sugars provide optimism. Thus, an increase in the concentration of soluble sugars helps plants to protect against drought (Bala, Citation2000). The drought-induced oxidative stress was detrimental to wheat that can be ameliorated through different enzymes in plants such as superoxide dismutase (SOD), ascorbate (APX), peroxidase (POD), and catalase (CAT). These mechanisms also increased tolerance against drought-induced detrimental effects (Abdelsalam et al., Citation2022; Hasanuzzaman et al., Citation2020; Saleem et al., Citation2016).
2.3.4. Molecular mechanisms of drought stress tolerance
There are two major molecular strategies in plants for drought tolerance. Firstly, signal transduction factors, protein kinases, transcription factors, and ABA receptors, and secondly, various proteins involved in metabolisms, osmotic regulation, and ROS transportation (Bala, Citation2000). Cascades pathway, signals transduction pathways, synthesis of drought proteins, metabolic and molecular elements response, vacuolar acid invertase (Close, Citation1996), glutathione S-transferase (GST) (Trouverie et al., Citation2003), and late embryo abundant (LEA) protein (Anderson & Davis, Citation2004); expression of ABA genes and production of proteins like RAB, rubisco, helicase, proline, and carbohydrates improves the plants against drought (Pnueli et al., Citation2002). Drought stress affects some genes and produces different types of proteins and enzymes (Liu et al., Citation2014). Drought stress causes the expression of drought-tolerant genes whose main function is to protect the plants against dehydration by producing stimuli or signals that were captured and transmitted to the multiple signal transduction pathways. Ca2+, ROS, ABA, phosphoglycerol, diacylglycerol, and transcriptional regulators are the major varieties of messengers to transmit the signals through the pathways. These signals cause the expression of drought-responsive genes that reduce the oxidative damage, also maintain the cell turgor, and adaption in plants (Kaur & Asthir, Citation2017; Rabello et al., Citation2008). Plants regulate the genes that encode various antioxidant enzymes such as superoxide dismutase (SOD), catalase (CAT), and peroxidases responsible to neutralize ROS leading to a reduction in oxidative damage. Similarly, some genes code for proteins that scavenge directly to ROS. The presence of transcription factors such as AP2/ERF (ethylene-responsive element binding factor) and bZIP (basic leucine zipper) binds the promoter region of antioxidant defense genes and activates them. Some genes are responsible for the production of osmoprotectants (soluble sugars and proline) that reduce dehydration and maintain the water potential thus maintaining the cell turgor pressure. Production of ABA in different parts of the plant due to the effect of water stress transmitted around the whole plant that ultimately reaches the stomata leads to the closure of stomata and protects the plant against transpiration loss (Mittler & Blumwald, Citation2015). Drought causes an increase in the pH of the xylem cells that decreases the amount of ABA in the xylem and is deposited in the guard cell of stomata that responds accordingly to the external environmental stimuli (Shatil-Cohen et al., Citation2011). Two types of genes were present in the plants in response to drought stress that is; ABA-dependent and ABA-independent based, based on their dependence on ABA (Kim, Citation2014). Regulatory elements such as transcription factors (TFs) like MYB, NAC, bZIP, AP2/ERF, and AREB/ABF strongly governed the molecular mechanisms of drought response through an expression of drought-responsive genes through metabolic pathways (Joshi et al., Citation2016; Kim et al., Citation2010). Glucose, sucrose, and trehalose are the major factors for the regulation of drought tolerance gene expression that is involved in the growth and stress tolerance in various plants (Kaur & Asthir, Citation2017). Drought produces ROS that cause oxidative damage to the crops leading to programmed cell death to escape from drought stress (Hasanuzzaman et al., Citation2020; Mubarik, Citation2021; R. Singh et al., Citation2016).
2.4. Management of drought stress
Management of drought stress can be done through genetic management and agronomic practices (Abraha et al., Citation2015).
2.4.1. Genetic management
The traditional breeding approach involves the selection of drought tolerance cultivars based on proper agronomic traits by studying genetic variability between the plant genotypes. Although conventional breeding had some prosperity, there was slow progress in drought breeding due to the limited availability of proper genes. Due to the uncontrolled crosses between the parents, the result/outcomes were undesirable traits that were not economically suitable (Ania, Citation2003).
The modern genetic approach is the evaluation of adaptability in plants under changing climatic conditions (U. Kumar et al., Citation2010; Kumari et al., Citation2007; Nagar et al., Citation2015; Sareen et al., Citation2012) through screening of the various genotypes for the identification of novel traits combination (Reynolds & Langridge, Citation2016), genetic engineering and transgenic approaches (incorporates, transfer and introduction of the desirable gene into the desired plants) (Chapman et al., Citation2012; Zheng et al., Citation2012) and thereby the adaptable varieties can be select and induce to develop new varieties with desired characteristics (Chapman et al., Citation2012). Examples such as incorporation of dwarfism gene Rht5 in combination with photoperiod insensitive gene Ppd-D1, which shortened the duration of the reproductive phase and facilitated early flowering of the genotypes that help the plants to escape the drought at their growth stages and improved the yield component of wheat (H. Chen et al., Citation2016; L. Chen, Du, et al., Citation2018; L. Chen, Yang, et al., Citation2018; Du et al., Citation2018). Application of structural and regulatory genes including dehydration-responsive, element-binding (DREB) factors, zinc finger proteins, and NAC transcription factor gene was already done for drought tolerance (Poddar et al., Citation2022). Genetically modified organisms (GMOs) and several molecular markers such as restriction fragment length polymorphisms (RFLPs), amplified fragment length polymorphisms (AFLPs), Simple Sequence Repeat (SSR) (Galiba et al., Citation1997; Maccaferri et al., Citation2008) and Single Nucleotide Polymorphism (SNP) (Kalladan et al., Citation2013) have been used to detect drought-related quantitative trait loci (QTL). Quantitative trait loci (QTL) have a major contribution to our understating of genetic control of grain yield under drought stress (Gosal et al., Citation2010). These QTL can be successfully transferred by CRISPR-Cas9 technology to develop transgenic wheat with an ability of drought tolerance (Poddar et al., Citation2022).
2.4.2. Agronomic management
Agronomic practices play a vital role in drought management and successful wheat cultivation (Paul et al., Citation2019). Crop rotation, irrigation schedule, sowing time, seed priming, mulching, nutrient management, method of sowing, use of artificial protectants, etc. were the major management approaches that can help to decrease or complete eradication of drought stress effect (A. Singh et al., Citation2011). Crop rotation enhances the soil-water relationships and increases the biomass as well as grain yield of the crops (Pierce & Rice, Citation2015). Seed priming increases seedling vigor and increases germination percentage which maintains optimum plant population and increases the yield under drought stress (B. Sharma et al., Citation2022). Mulching the soil with organic mulch helps in soil water conservation through reduced soil evaporation that conserves water, suppresses weed growth, enhances nitrogen use efficiency, and overall improves the growth and development of plants (S. Y. Chen et al., Citation2007). Proper timing and sourcing with an adequate dose of fertilizer application are essential for the better growth and development of the crops exposed to stressed environments through the remobilization of nutrients and increased grain filling (S. Y. Chen et al., Citation2007; Waraich et al., Citation2012). The exogenous application of various drought stress protectants such as osmoprotectants, phytohormones, signaling molecules, and trace elements neutralizes the harmful effect and protects plants from drought (P. Sharma et al., Citation2012; Yang & Zhang, Citation2006).
3. Conclusion
Drought stress is one of the major abiotic constraints leading to an enormous yield loss of wheat globally. Although drought had become a serious threat to wheat production, an in-depth study on the effect and tolerance mechanism of drought had not been done yet. So, further understanding of all the mechanisms associated with the drought to plants should be clearly understood. The drought stress tolerance mechanisms would be the major strategies for wheat management in the present scenario of global climate change-induced drought stress. Drought-tolerant wheat varieties are a prerequisite to balance the production and food demand of the growing population. For the development of drought-tolerant high-yielding varieties, there is a need for a proper understanding of the physiological, biochemical, and molecular basis of drought tolerance. Traditional and modern molecular genetics approaches along with agronomic management practices would help plants to overcome drought stress easily. Thus the information gathered through this review can be employed in drought breeding programs for the development of drought-tolerant wheat varieties.
Major highlight of the paper
Drought stress has a complex effect on morphology, physiology, and biochemistry leads to retardation of growth and productivity of wheat. Thus, this review approach to combat drought stress. Drought has become worldwide issue, major reason for drought stress is water scarcity. So, there is urgent need of searching for ways that can be helpful in ameliorating or completely eradication drought stress in plants, especially food crops, to ensure food security worldwide. Although there were various research and reviews regarding the individual study on drought stress effects, causes, tolerance, and management but a combined study had been presented in this review.
Disclosure statement
No potential conflict of interest was reported by the author(s).
Additional information
Notes on contributors
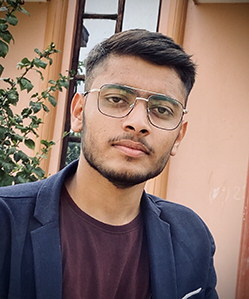
Shivalal Nyaupane
The author Shivalal Nyaupane was born on 9 July, 2001 as the Son of Mr. Reshamraj Nyaupane and Laxmi Nyaupane at Arbeni-5 in Gulmi district, Nepal. He had completed his School Leaving Certificate (S.L.C) in 2072 from Shaligram Higher Secondary School. He received intermediate Degree in Science (+2) from Manimukunda College in 2075 and joined his Bachelor degree at Institute of Agriculture and Animal Science (IAAS), Paklihawa Campus, Bhairahawa, Rupandehi under B.Sc. Ag programme in 2076. The author is a dedicated, hardworking and self-driven individual, with an honest interest in plant breeding research works. He published some articles in international journals and has been an active part of agriculture-related training and seminars. Interested researchers can reach out to him via phone (9867372642) and email ([email protected]).
References
- Abdelsalam, N. R., Abdel-Megeed, A., Ghareeb, R. Y., Ali, H. M., Salem, M. Z., Akrami, M., Al-Hayalif, M. F., & Desoky, E.-S M. (2022). Genotoxicity assessment of amino zinc nanoparticles in wheat (Triticum aestivum L.) as cytogenetical perspective. Saudi Journal of Biological Sciences, 29(4), 1–15. https://doi.org/10.1016/j.sjbs.2021.11.059
- Abdoli, M., & Saedi, M. (2012). Effects of water deficiency stress during seed growth on yield and its components, germination and seedling growth parameters of some wheat cultivars. International Journal of Agriculture and Crop Sciences, 4, 1110–1118.
- Abraha, M. T., Hussein, S., Laing, M., & Assefa, K. (2015). Genetic management of drought in tef: Current status and future research directions. Global Journal of Crop, Soil Science and Plant Breeding, 3, 156–161.
- Ahmad, A., Aslam, Z., Javed, T., Hussain, S., Raza, A., Shabbir, R., Mora-Poblete, F., Saeed, T., Zulfiqar, F., Ali, M. M., Nawaz, M., Rafiq, M., Osman, H. S., Albaqami, M., Ahmed, M. A. A., & Tauseef, M. (2022). Screening of wheat (Triticum aestivum L.) genotypes for drought tolerance through agronomic and physiological response. Agronomy, 12(2), 287. https://doi.org/10.3390/agronomy12020287
- Ahmad, Z., Waraich, E. A., Akhtar, S., Anjum, S., Ahmad, T., Mahboob, W., Hafeez, O. B. A., Tapera, T., Labuschagne, M., & Rizwan, M. (2018). Physiological responses of wheat to drought stress and its mitigation approaches. Acta Physiologiae Plantarum, 40(4), 80. https://doi.org/10.1007/s11738-018-2651-6
- Ajithkumar, I. P., & Panneerselvam, R. (2014). ROS scavenging system, osmotic maintenance, pigment and growth status of Panicum sumatrense Roth. under drought stress. Cell Biochemistry and Biophysics, 68, 587–595. https://doi.org/10.1007/s12013-013-9746-x
- Akter, N., & Rafiqul Islam, M. (2017). Heat stress effects and management in wheat. A review. Agronomy for Sustainable Development, 37(5), 6. https://doi.org/10.1007/s13593-017-0443-9
- Ali, M., Gul, A., Hasan, H., Gul, S., Fareed, A., Nadeem, M., Siddique, R, Jan, S. U., & Jamil, M. (2020). Cellular mechanisms of drought tolerance in wheat. In M. Ozturk & A. Gul (Eds.), Climate change and food security with emphasis on wheat (pp. 155–167). Elsevier. https://doi.org/10.1016/B978-0-12-819527-7.00009-1
- Almansouri, M., Kinet, J. M., & Lutts, S. (2001). Effect of salt and osmotic stresses on germination in durum wheat (Triticum durum Desf.). Plant and Soil, 231(2), 243–254. https://doi.org/10.1023/A:1010378409663
- Anderson, J. V., & Davis, D. G. (2004). Abiotic stress alters transcript profiles and activity of glutathione S-transferase, glutathione peroxidase, and glutathione reductase in Euphorbia esula. Physiologia Plantarum, 120(3), 421–433. https://doi.org/10.1111/j.0031-9317.2004.00249.x
- Ania, W. (2003). Use of biotechnology in agriculture—Benefits and risks. Biotechnology, 3, 1–6.
- Anjum, S. A., Ashraf, U., Zohaib, A., Tanveer, M., Naeem, M., Ali, I., Tabassum, T., & Nazir, U. (2017). Growth and developmental responses of crop plants under drought stress: A review. Zemdirbyste-Agriculture, 104(3), 267–276. https://doi.org/10.13080/z-a.2017.104.034
- Anosheh, H. P., Moucheshi, A. S., Pakniyat, H., & Pessarakli, M. (2016). Stomatal responses to drought stress. Water Stress and Crop Plants, 1, 24–40.
- Arbona, V., Manzi, M., de Ollas, C., & Gómez-Cadenas, A. (2013). Metabolomics as a tool to investigate abiotic stress tolerance in plants. International Journal of Molecular Sciences, 14(3), 4885–4911. https://doi.org/10.3390/ijms14034885
- Aroca, R. (2013). Plant responses to drought stress: From morphological to molecular features. In Plant responses to drought stress from morphological to molecular features. Springer. https://doi.org/10.1007/978-3-642-32653-0
- Arzani, A., & Ashraf, M. (2017). Cultivated ancient wheats (Triticum spp.): A potential source of health-beneficial food products. Comprehensive Reviews in Food Science and Food Safety, 16(3), 477–488. https://doi.org/10.1111/1541-4337.12262
- Bala, R. (2000). Metabolic engineering for stress tolerance: Installing osmoprotectant synthesis pathways. Annals of Botany, 86, 709–716. https://doi.org/10.1006/anbo.2000.1254
- Bandurska, H., Niedziela, J., Pietrowska-Borek, M., Nuc, K., Chadzinikolau, T., & Radzikowska, D. (2017). Regulation of proline biosynthesis and resistance to drought stress in two barley (Hordeum vulgare L.) genotypes of different origin. Plant Physiology and Biochemistry: PPB, 118, 427–437. https://doi.org/10.1016/j.plaphy.2017.07.006
- Barbara, S., Resources, N., & Collins, F. (2007). Microbial stress-response physiology and its implications. Ecology, 88, 1386–1394.
- Bhandari, R., Gnawali, S., Nyaupane, S., Kharel, S., Poudel, M., & Panth, P. (2021). Effect of drought & irrigated environmental condition on yield & yield attributing characteristic of bread wheat-A review. Reviews in Food and Agriculture, 2(2), 59–62. https://doi.org/10.26480/rfna.02.2021.59.62
- Bing, Y. I. (2014). Effect of drought stress during flowering stage on starch accumulation and starch synthesis enzymes in sorghum grains. Journal of Integrative Agriculture, 13, 2399–2406.
- Blum, A. (2017). Osmotic adjustment is a prime drought stress adaptive engine in support of plant production. Plant, Cell & Environment, 40(1), 4–10. https://doi.org/10.1111/pce.12800
- Çakir, R. (2004). Effect of water stress at different development stages on vegetative and reproductive growth of corn. Field Crops Research, 89(1), 1–16. https://doi.org/10.1016/j.fcr.2004.01.005
- Chachar, M. H., Chachar, N. A., & Chachar, Q. (2016). Physiological characterization of six wheat. International Journal of Educational Research, 4, 13.
- Chapman, S. C., Chakraborty, S., Dreccer, M. F., & Howden, S. M. (2012). Plant adaptation to climate change opportunities and priorities in breeding. Crop and Pasture Science, 63(3), 251–268. https://doi.org/10.1071/CP11303
- Chen, H., Moakhar, N. P., Iqbal, M., Pozniak, C., Hucl, P., & Spaner, D. (2016). Genetic variation for flowering time and height reducing genes and important traits in western Canadian spring wheat. Euphytica, 208(2), 377–390. https://doi.org/10.1007/s10681-015-1615-9
- Chen, L., Du, Y., Lu, Q., Chen, H., Meng, R., Cui, C., Lu, S., Yang, Y., Chai, Y., Li, J., Liu, L., Qi, X., Li, H., Mishina, K., Yu, F., & Hu, Y.-G. (2018). The photoperiod-insensitive allele Ppd-D1a promotes earlier flowering in Rht12 dwarf plants of bread wheat. Frontiers in Plant Science, 9, 1312. https://doi.org/10.3389/fpls.2018.01312
- Chen, L., Yang, Y., Cui, C., Lu, S., Lu, Q., Du, Y., Su, R., Chai, Y., Li, H., Chen, F., Yu, F., & Hu, Y.-G. (2018). Effects of Vrn-B1 and Ppd-D1 on developmental and agronomic traits in Rht5 dwarf plants of bread wheat. Field Crops Research, 219, 24–32. https://doi.org/10.1016/j.fcr.2018.01.022
- Chen, S. Y., Zhang, X. Y., Pei, D., Sun, H. Y., & Chen, S. L. (2007). Effects of straw mulching on soil temperature, evaporation and yield of winter wheat: Field experiments on the North China Plain. Annals of Applied Biology. 150(3), 261–268. https://doi.org/10.1111/j.1744-7348.2007.00144.x
- Chowdhury, M. K., Hasan, M. A., Bahadur, M. M., Islam, M. R., Hakim, M. A., Iqbal, M. A., Javed, T., Raza, A., Shabbir, R., Sorour, S., Elsanafawy, N. E. M., Anwar, S., Alamri, S., Sabagh, A. E., & Islam, M. S. (2021). Evaluation of drought tolerance of some wheat (Triticum). Agronomy, 11(9), 1792. https://doi.org/10.3390/agronomy11091792
- Close, T. J. (1996). Dehydrins: Emergence of a biochemical role of a family of plant dehydration proteins. Physiologia Plantarum, 97(4), 795–803. https://doi.org/10.1034/j.1399-3054.1996.970422.x
- Dai, A. (2013). Increasing drought under global warming in observations and models. Nature Climate Change, 3(1), 52–58. https://doi.org/10.1038/nclimate1633
- Dalirie, M. S., Sharifi, R. S., & Farzaneh, S. (2010). Evaluation of yield, dry matter accumulation and leaf area index in wheat genotypes as affected by terminal drought stress. Notulae Botanicae Horti Agrobotanici Cluj-Napoca, 38, 182–186.
- De Simone, V., Soccio, M., Borrelli, G. M., Pastore, D., & Trono, D. (2014). Stay-green trait-antioxidant status interrelationship in durum wheat (Triticum durum) flag leaf during post-flowering. Journal of Plant Research, 127(1), 159–171. https://doi.org/10.1007/s10265-013-0584-0
- Deshmukh, P., Sairam, R., & Shukla, D. (1991). Measurement of ion leakage as a screening technique for drought resistance in wheat genotypes. Indian Journal of Plant Physiology, 34, 89–91.
- Doležel, J. (2010). Development of chromosome-specific BAC resources for genomics of bread. Cytogenetic and Genome Research, 1–13. https://doi.org/10.1159/000313072
- Du, Y., Chen, L., Wang, Y., Yang, Z., Saeed, I., Daoura, B. G., & Hu, Y.-G. (2018). The combination of dwarfing genes Rht4 and Rht8 reduced plant height, improved yield traits of rainfed bread wheat (Triticum aestivum L.). Field Crops Research, 215, 149–155. https://doi.org/10.1016/j.fcr.2017.10.015
- El-Mouhamady, A. B. A., El-Hawary, M. M., Ali, M., & Habouh, F. (2023). Transgenic wheat for drought stress tolerance: A review. Middle East Journal of Agriculture Research, 12(1), 77–94. https://doi.org/10.36632/mejar/202
- Fang, Y., & Xiong, L. (2015). General mechanisms of drought response and their application in drought resistance improvement in plants. Cellular and Molecular Life Sciences: CMLS, 72(4), 673–689. https://doi.org/10.1007/s00018-014-1767-0
- FAOSTAT. (2022). FAOSTAT. http://www.fao.org/faostat/en/#data/QC
- Farooq, M., Hussain, M., & Siddique, K. H. M. (2014). Drought stress in wheat during flowering and grain-filling periods drought stress in wheat during flowering and grain-filling. Critical Reviews in Plant Sciences, 33(4), 331–349. https://doi.org/10.1080/07352689.2014.875291
- Farooq, M., Wahid, A., Kobayashi, N., Fujita, D., & Basra, S. M. A. (2009). Plant drought stress: Effects, mechanisms and management. Agronomy for Sustainable Development, 29(1), 185–212. https://doi.org/10.1051/agro:2008021
- Fierer, N., & Schimel, J. P. (2002). Effects of drying ± rewetting frequency on soil carbon and nitrogen transformations. Soil Biology and Biochemistry, 34(6), 777–787. https://doi.org/10.1016/S0038-0717(02)00007-X
- Finkelstein, R. R., Gampala, S. S. L., & Rock, C. D. (2002). Abscisic acid signaling in seeds and seedlings. The Plant Cell, 14(suppl 1), S15–S45. https://doi.org/10.1105/tpc.010441
- Galiba, G., Kerepesi, I., Snape, J. W., & Sutka, J. (1997). Location of a gene regulating cold-induced carbohydrate production on chromosome 5A of wheat. Theoretical and Applied Genetics, 95(1–2), 265–270. https://doi.org/10.1007/s001220050558
- Gosal, S. S., Wani, S. H., & Kang, M. S. (2010). Biotechnology and drought tolerance. Water and Agricultural Sustainability Strategies, 23(1), 19–54. https://doi.org/10.1080/15427520802418251
- Grandgirard, J., Poinsot, D., Krespi, L., Nénon, J. P., & Cortesero, A. M. (2002). Costs of secondary parasitism in the facultative hyperparasitoid Pachycrepoideus dubius: Does host size matter? Entomologia Experimentalis et Applicata, 103(3), 239–248. https://doi.org/10.1046/j.1570-7458.2002.00982.x
- Hajibarat, Z., & Saidi, A. (2022). Senescence-associated proteins and nitrogen remobilization in grain filling under drought stress condition. Journal of Genetic Engineering and Biotechnology, 20(1), 1–14. https://doi.org/10.1186/s43141-022-|00378-5
- Hampson, C. R., & Simpson, G. M. (1990). Effects of temperature, salt, and osmotic potential on early growth of wheat (Triticum aestivum). II. Early seedling growth. Canadian Journal of Botany, 68(3), 529–532. https://doi.org/10.1139/b90-073
- Hartung, W., Sauter, A., & Hose, E. (2002). Abscisic acid in the xylem: Where does it come from, where does it go to ? Journal of Experimental Botany, 53(366), 27–32. https://doi.org/10.1093/jexbot/53.366.27
- Hasanuzzaman, M., Bhuyan, M. H. M. B., Zulfiqar, F., Raza, A., & Fotopoulos, V. (2020). Reactive oxygen species and antioxidant defense in plants under abiotic stress: Revisiting the crucial role of a universal defense regulator.
- He, F., Sheng, M., & Tang, M. (2017). Effects of rhizophagus irregularis on photosynthesis and antioxidative enzymatic system in Robinia pseudoacacia L. under drought stress. Frontiers in Plant Science, 8, 1–14.
- Hossain, A., Teixeira da Silva, J. A., Lozovskaya, M. V., & Zvolinsky, V. P. (2012). High temperature combined with drought affect rainfed spring wheat and barley in South-Eastern Russia: I. Phenology and growth. Saudi Journal of Biological Sciences, 19(4), 473–487. https://doi.org/10.1016/j.sjbs.2012.07.005
- Hossain, M. A., Wani, S. H., Bhattacharjee, S., Burritl, D. J., & Tran, L. S. P. (2016). Drought stress tolerance in plants, vol 1: Physiology and biochemistry. Journal of Physiology and Biochemistry, 1(1), 1–526.
- Hu, Y., & Schmidhalter, U. (2005). Drought and salinity: A comparison of their effects on mineral nutrition of. Journal of Plant Nutrition and Soil Science, 168(4), 541–549. https://doi.org/10.1002/jpln.200420516
- Huang, L., Zhang, H., Song, Y., Yang, Y., Chen, H., & Tang, M. (2017). Subcellular compartmentalization and chemical forms of lead participate in lead tolerance of Robinia pseudoacacia L. with Funneliformis mosseae. Frontiers in Plant Science, 08, 1–12. https://doi.org/10.3389/fpls.2017.00517
- Hussain, A., & Jatoi, W. A. (2021). Drought tolerance indices of wheat (Triticum aestivum L.) genotypes under water deficit conditions. Plant Cell Biotechnology and Molecular Biology, 20, 1–19.
- Jiang, F., & Hartung, W. (2007). Long-distance signalling of abscisic acid (ABA): The factors regulating the intensity of the ABA signal. Journal of Experimental Botany, 59(1), 37–43. https://doi.org/10.1093/jxb/erm127
- Jones, M. B., Leafe, E. L., & Stiles, W. (1980). Water stress in field-grown perennial ryegrass. I. Its effect on growth, canopy photosynthesis and transpiration. Annals of Applied Biology, 96(1), 87–101. https://doi.org/10.1111/j.1744-7348.1980.tb04772.x
- Joshi, R., Wani, S. H., Singh, B., Bohra, A., Dar, Z. A., Lone, A. A., Pareek, A., & Singla-Pareek, S. L. (2016). Transcription factors and plants response to drought stress: Current understanding and future directions. Frontiers in Plant Science, 7, 1–15. https://doi.org/10.3389/fpls.2016.01029
- Jungang, W., Guocang, C., & Chenglie, Z. (2002). The effects of water stress on soluble protein content, the activity of SOD, POD and CAT of two ecotypes of reeds (Phragmites communis). Acta Botanica Boreali-Occidentalia Sinica, 22, 561–565.
- Kalladan, R., Worch, S., Rolletschek, H., Harshavardhan, V. T., Kuntze, L., Seiler, C., Sreenivasulu, N., & Röder, M. S. (2013). Identification of quantitative trait loci contributing to yield and seed quality parameters under terminal drought in barley advanced backcross lines. Molecular Breeding, 32(1), 71–90. https://doi.org/10.1007/s11032-013-9853-9
- Kamal, T., Atiq, M., Khan, U., Khan, F. U., & Ahmed, S. (2020). Comparison among different stability models for yield in bread wheat. Sarhad Journal of Agriculture, 36, 282–290.
- Kandel, S. (2021). Wheat responses, defence mechanisms and tolerance to drought stress: A review article. International Journal for Research in Applied Sciences and Biotechnology, 8(5), 99–109. https://doi.org/10.31033/ijrasb.8.5.14
- Kaur, G., & Asthir, B. (2017). Molecular responses to drought stress in plants. Biologia Plantarum, 61(2), 201–209. https://doi.org/10.1007/s10535-016-0700-9
- Kaveh, M., & Chayjan, R. A. (2014). Modeling drying characteristics of terebinth fruit under infrared fluidized bed condition evaluation and modeling some engineering properties of three safflower varieties the effect of hydro-priming on germination characteristics. Seedling Growth and Ant, XLVII, 1–7.
- Khan, M. A., Waseem Akram, M., Iqbal, M., Ghulam Muhu-Din Ahmed, H., Rehman, A., Arslan Iqbal, H. S. M., & Alam, B. (2023). Communications in soil science and plant analysis multivariate and association analyses of quantitative attributes reveal drought tolerance potential of wheat (Triticum aestivum L.) genotypes. Communications in Soil Science and Plant Analysis, 54(2), 178–195. https://doi.org/10.1080/00103624.2022.2110893
- Kim, T. H. (2014). Mechanism of ABA signal transduction: Agricultural highlights for improving drought tolerance. Journal of Plant Biology, 57(1), 1–8. https://doi.org/10.1007/s12374-014-0901-8
- Kim, T. H., Böhmer, M., Hu, H., Nishimura, N., & Schroeder, J. I. (2010). Guard cell signal transduction network: Advances in understanding abscisic acid, CO2, and Ca2+ signaling. Annual Review of Plant Biology, 61(1), 561–591. https://doi.org/10.1146/annurev-arplant-042809-112226
- Kiyosue, T., Yoshiba, Y., Yamaguchi-Shinozaki, K., & Shinozaki, K. (1996). A nuclear gene encoding mitochondrial proline dehydrogenase, an enzyme involved in proline metabolism, is upregulated by proline but downregulated by dehydration in Arabidopsis. Plant Cell, 8(8), 1323–1335. https://doi.org/10.2307/3870304
- Kou, X., Han, W., & Kang, J. (2022). Responses of root system architecture to water stress at multiple levels: A meta-analysis of trials under controlled conditions. Frontiers in Plant Science, 13, 1–16. https://doi.org/10.3389/fpls.2022.1085409
- Kumar, A., Sharma, S. K., Sharma, C. L., & Iiwbr, I. (2018). Impact of water deficit (salt and drought) stress on physiological, biochemical and yield attributes on wheat (Triticum aestivum) varieties. Indian Journal of Agricultural Sciences, 86, 144–152. https://doi.org/10.56093/ijas.v88i10.84255
- Kumar, U., Joshi, A. K., Kumari, M., Paliwal, R., Kumar, S., & Röder, M. S. (2010). Identification of QTLs for stay green trait in wheat (Triticum aestivum L.) in the ‘Chirya 3’ × ‘Sonalika’ population. Euphytica, 174(3), 437–445. https://doi.org/10.1007/s10681-010-0155-6
- Kumari, M., Singh, V. P., Tripathi, R., & Joshi, A. K. (2007). Variation for staygreen trait and its association with canopy temperature depression and yield traits under terminal heat stress in wheat. International Journal of Plant Breeding, 186, 357–363. https://doi.org/10.1007/1-4020-5497-1_44
- Kura-Hotta, M., Satoh, K., & Katoh, S. (1987). Relationship between photosynthesis and chlorophyll content during leaf senescence of rice seedlings. Plant Cell Physiology, 28, 1321–1329.
- Kvgk, V. P., & G, R. R. (2019). Various plant’s responses and strategies to cope with the water deficit: A review. Journal of Pharmacognosy and Phytochemistry, 8, 159–168.
- La, F., & Sylvestris, T. (1990). Influence of drought on the concentration and distribution of 2,4-diaminobutyric acid and other free amino acids in tissues of flatpea (la Thyrus sylvestris L.) liming. Environmental and Experimental Botany, 30, 497–504.
- Lambin, E. F., Turner, B. L., Geist, H. J., Agbola, S. B., Angelsen, A., Bruce, J. W., Coomes, O. T., Dirzo, R., Fischer, G., Folke, C., George, P. S., Homewood, K., Imbernon, J., Leemans, R., Li, X., Moran, E. F., Mortimore, M., Ramakrishnan, P. S., Richards, J. F., … Xu, J. (2001). The causes of land-use and land-cover change: Moving beyond the myths. Global Environmental Change, 11(4), 261–269. https://doi.org/10.1016/S0959-3780(01)00007-3
- Lehari, K., Kumar, M., Burman, V., Vaishali, A., Kumar, V., Chand, P., & Singh, R. (2019). Morphological, physiological and biochemical analysis of wheat genotypes under drought stress. Journal of Pharmacognosy and Phytochemistry, SP2, 1026–1030.
- Li, X. (2013). Targeting mitochondrial reactive oxygen species as novel therapy for inflammatory diseases and cancers. 1–19.
- Li, Y., Song, H., Zhou, L., Xu, Z., & Zhou, G. (2019). Tracking chlorophyll fluorescence as an indicator of drought and rewatering across the entire leaf lifespan in a maize field. Agricultural Water Management, 211, 190–201. https://doi.org/10.1016/j.agwat.2018.09.050
- Lisar, S. Y. S., Motafakkerazad, R., & Hossain, M. M. (2012). Water stress in plants: Causes, effects and responses. In I. M. Mofizur Rahman (Ed.), Water stress (vol. 10, pp. 39363). Water stress.
- Liu, J., Chen, N., Chen, F., Cai, B., Dal Santo, S., Tornielli, G. B., Pezzotti, M., & Cheng, Z.-M. (2014). Genome-wide analysis and expression profile of the bZIP transcription factor gene family in grapevine (Vitis vinifera). BMC Genomics, 15(1), 1–18. https://doi.org/10.1186/1471-2164-15-281
- Lu, H., Hu, Y., Wang, C., Liu, W., Ma, G., Han, Q., & Ma, D. (2019). Effects of high temperature and drought stress on the expression of gene encoding enzymes and the activity of key enzymes involved in starch biosynthesis in wheat grains. Frontiers in Plant Science, 10, 1–17. https://doi.org/10.3389/fpls.2019.01414
- Maccaferri, M., Sanguineti, M. C., Corneti, S., Ortega, J. L. A., Salem, M. B., Bort, J., DeAmbrogio, E., del Moral, L. F. G., Demontis, A., El-Ahmed, A., Maalouf, F., Machlab, H., Martos, V., Moragues, M., Motawaj, J., Nachit, M., Nserallah, N., Ouabbou, H., Royo, C., Slama, A., & Tuberosa, R. (2008). Quantitative trait loci for grain yield and adaptation of durum wheat (Triticum durum Desf.) across a wide range of water availability. Genetics, 178(1), 489–511. https://doi.org/10.1534/genetics.107.077297
- Mafakheri, A., Siosemardeh, A. F., Bahramnejad, B., Struik, P. C., & Sohrabi, Y. (2010). Effect of drought stress on yield, proline and chlorophyll contents in three chickpea cultivars. Australian Journal of Crop Science, 4, 580–585.
- Maghsoudi, K., Emam, Y., & Pessarakli, M. (2016). Effect of silicon on photosynthetic gas exchange, photosynthetic pigments, cell membrane stability and relative water content of different wheat cultivars under drought stress conditions. Journal of Plant Nutrition, 39(7), 1001–1015. https://doi.org/10.1080/01904167.2015.1109108
- Manjarrez-Sandoval, P., González-Hernández, V. A., Mendoza-Onofre, L. E., & Engleman, E. M. (1989). Drought stress effects on the grain yield and panicle development of sorghum. Canadian Journal of Plant Science, 69(3), 631–641. https://doi.org/10.4141/cjps89-079
- Mansour, H. A., El, S., Mohamed, S., & Lightfoot, D. A. (2020). Molecular studies for drought tolerance in some Egyptian wheat genotypes under different irrigation systems. Open Agriculture, 5(1), 280–290. https://doi.org/10.1515/opag-2020-0030
- McCree, K. J., & Davis, S. D. (1974). Effect of water stress and temperature on leaf size and on size and number of epidermal cells in grain sorghum 1. Crop Science, 14(5), 751–755. https://doi.org/10.2135/cropsci1974.0011183X001400050041x
- Mittler, R., & Blumwald, E. (2015). The roles of ROS and ABA in systemic acquired acclimation. The Plant Cell, 27(1), 64–70. https://doi.org/10.1105/tpc.114.133090
- Mohammed, A. K., & Kadhem, F. A. (2017). Effect of water stress on yield and yield components of bread wheat genotypes. Iraqi Journal of Agricultural Sciences, 48, 729–739.
- Mubarik, M. S. (2021). A manipulative interplay between positive and negative regulators of phytohormones: A way forward for improving drought tolerance in plants. Physiologia Plantarum, 172, 1269–1290. https://doi.org/10.1111/ppl.13325
- Mwadzingeni, L., Shimelis, H., Dube, E., Laing, M. D., & Tsilo, T. J. (2016). Breeding wheat for drought tolerance: Progress and technologies. Journal of Integrative Agriculture, 15(5), 935–943. https://doi.org/10.1016/S2095-3119(15)61102-9
- Nagar, S., Singh, V. P., Arora, A., Dhakar, R., & Ramakrishnan, S. (2015). Assessment of terminal heat tolerance ability of wheat genotypes based on physiological traits using multivariate analysis. Acta Physiologiae Plantarum, 37, 1–9.
- Narwal, S. (2015). Genetic and molecular dissection of drought tolerance in wheat and barley. Journal of Wheat Researc, 7, 1–13.
- Nezhadahmadi, A., Prodhan, Z. H., & Faruq, G. (2013). Drought tolerance in wheat. The Scientific World Journal, 2013, 1–12. https://doi.org/10.1155/2013/610721
- Nikolaeva, M. K., Maevskaya, S. N., Shugaev, A. G., & Bukhov, N. G. (2010). Effect of drought on chlorophyll content and antioxidant enzyme activities in leaves of three wheat cultivars varying in productivity. Russian Journal of Plant Physiology, 57, 94–102.
- Ozturk, M., Turkyilmaz Unal, B., García-Caparrós, P., Khursheed, A., Gul, A., & Hasanuzzaman, M. (2021). Osmoregulation and its actions during the drought stress in plants. Physiologia Plantarum. 172(2), 1321–1335. https://doi.org/10.1111/ppl.13297
- Palmer, J. H., & Steer, B. T. (1985). The generative area as the site of floret initiation in the sunflower capitulum and its integration to predict floret number. Field Crops Research, 11, 1–12. https://doi.org/10.1016/0378-4290(85)90088-7
- Paudel, B., Zhang, Y., Yan, J., Rai, R., Li, L., Wu, X., Chapagain, P. S., & Khanal, N. R. (2020). Farmers’ understanding of climate change in Nepal Himalayas: important determinants and implications for developing adaptation strategies. Climate Change, 158(3–4), 485–502. https://doi.org/10.1007/s10584-019-02607-2
- Paul, C., Debnath, B., & Paul, C. (2019). Plant science today species. Plant Science Today, 6(2), 147–150. https://doi.org/10.14719/pst.2019.6.2.490
- Pierce, F. J., & Rice, C. W. (2015). Crop rotation and its impact on efficiency of water and nitrogen use. In W. L. Hargrove (Ed.), Cropping strategies for efficient use of water and nitrogen (pp. 21–42). Wiley. https://doi.org/10.2134/asaspecpub51.c3
- Pnueli, L., Hallak-Herr, E., Rozenberg, M., Cohen, M., Goloubinoff, P., Kaplan, A., & Mittler, R. (2002). Molecular and biochemical mechanisms associated with dormancy and drought tolerance in the desert legume Retama raetam. The Plant Journal, 31(3), 319–330. https://doi.org/10.1046/j.1365-313X.2002.01364.x
- Poddar, S., Chandra, B., Viswavidyalaya, K., & Roy, S. (2022). Response and breeding of wheat under drought stress. Agriculture and Environment, 3, 1–6.
- Poudel, M. R., Ghimire, S., Pandey, M. P., Dhakal, K. H., & Thapa, D. B. (2020). Evaluation of wheat genotypes under irrigated, heat stress and drought conditions. Journal of Biology and Today’s World, 9, 212.
- Prasad, P. V. V., Pisipati, S. R., Momčilović, I., & Ristic, Z. (2011). Independent and combined effects of high temperature and drought stress during grain filling on plant yield and chloroplast EF-Tu expression in spring wheat. Journal of Agronomy and Crop Science, 197(6), 430–441. https://doi.org/10.1111/j.1439-037X.2011.00477.x
- Premachandra, G. S., Saneoka, H., & Ogata, S. (1990). Cell membrane stability, an indicator of drought tolerance, as affected by applied nitrogen in soyabean. The Journal of Agricultural Science, 115(1), 63–66. https://doi.org/10.1017/S0021859600073925
- Qadir, G., S, M., & C, M. A. (1998). Effect of water stress on growth and yield performance of four wheat cultivars. Pakistan Journal of Biological Sciences, 2(1), 236–239. https://doi.org/10.3923/pjbs.1999.236.239
- Rabello, A. R., Guimarães, C. M., Rangel, P. H., da Silva, F. R., Seixas, D., de Souza, E., Brasileiro, A. C., Spehar, C. R., Ferreira, M. E., & Mehta, Â. (2008). Identification of drought-responsive genes in roots of upland rice (Oryza sativa L). BMC Genomics, 9(1), 1–13. https://doi.org/10.1186/1471-2164-9-485
- Rakszegi, M., Darkó, É., Lovegrove, A., Molnár, I., Láng, L., Bedő, Z., Molnár-Láng, M., & Shewry, P. (2019). Drought stress affects the protein and dietary fiber content of wholemeal wheat flour in wheat/Aegilops addition lines. PLoS One, 14(2), e0211892. https://doi.org/10.1371/journal.pone.0211892
- Rawtiya, A. K., & Kasal, Y. G. (2021). Drought stress and wheat (Triticum aestivum L.) yield: A review. The Pharma Innovation Journal, 10, 1007–1012.
- Raza, M. A. S., Jamil, M., Ijaz, M., Khan, M. A., Saleem, M. F., & Khan, I. H. (2012). Evaluating the drought stress tolerance efficiency of wheat (triticum aestivum l.) Cultivars. Russian Journal of Agricultural and Socio-Economic Sciences, 12, 6.
- Reddy, A. R., Chaitanya, K. V., & Vivekanandan, M. (2004). Drought-induced responses of photosynthesis and antioxidant metabolism in higher plants. Journal of Plant Physiology, 161(11), 1189–1202. https://doi.org/10.1016/j.jplph.2004.01.013
- Reynolds, M., & Langridge, P. (2016). Physiological breeding. Current Opinion in Plant Biology, 31, 162–171. https://doi.org/10.1016/j.pbi.2016.04.005
- Ruan, Y. (2014). Sucrose metabolism: Gateway to diverse carbon use and sugar signaling. https://doi.org/10.1146/annurev-arplant-050213-040251
- Salam, A., Ali, A., Afridi, M. S., & Ali, S. (2022). Agrobiodiversity: Effect of drought stress on the eco-physiology and morphology of wheat chapter 33 agrobiodiversity. Effect of Drought Stress on the Eco-Physiology and Morphology of Wheat, 23, 23. https://doi.org/10.1007/978-3-030-73943-0
- Saleem, M. F., Sammar Raza, M. A., Ahmad, S., Khan, I. H., & Shahid, A. M. (2016). Understanding and mitigating the impacts of drought stress in cotton-A review understanding and mitigating the impacts of drought stress in cotton-A review. Pakistan Journal of Agricultural Sciences, 53, 1–16. https://doi.org/10.21162/PAKJAS/16.3341
- Sánchez, F. J., Manzanares, M., De Andres, E. F., Tenorio, J. L., & Ayerbe, L. (1998). Turgor maintenance, osmotic adjustment and soluble sugar and proline accumulation in 49 pea cultivars in response to water stress. Field Crops Research, 59(3), 225–235. https://doi.org/10.1016/S0378-4290(98)00125-7
- Sareen, S., Tyagi, B. S., & Sharma, I. (2012). Response estimation of wheat synthetic lines to terminal heat stress using stress indices. Journal of Agricultural Science, 4, 97–104.
- Sharifi, P., & Mohammadkhani, N. (2016). Effects of drought stress on photosynthesis factors in wheat genotypes during anthesis. Cereal Research Communications, 44(2), 229–239. https://doi.org/10.1556/0806.43.2015.054
- Sharma, B., Yadav, L., Shrestha, A., Shrestha, S., Subedi, M., Subedi, S., & Shrestha, J. (2022). Drought stress and its management in wheat (Triticum aestivum L.): A review. Agricultural Science and Technology, 14(1), 3–14. https://doi.org/10.15547/10.15547/ast.2022.01.001
- Sharma, P., Jha, A. B., Dubey, R. S., & Pessarakli, M. (2012). Reactive oxygen species, oxidative damage, and antioxidative defense mechanism in plants under stressful conditions. Journal of Botany, 2012, 1–26. https://doi.org/10.1155/2012/217037
- Shatil-Cohen, A., Attia, Z., & Moshelion, M. (2011). Bundle-sheath cell regulation of xylem-mesophyll water transport via aquaporins under drought stress: A target of xylem-borne ABA? The Plant Journal, 67(1), 72–80. https://doi.org/10.1111/j.1365-313X.2011.04576.x
- Shavrukov, Y., Kurishbayev, A., Jatayev, S., Shvidchenko, V., Zotova, L., Koekemoer, F., de Groot, S., Soole, K., & Langridge, P. (2017). Early flowering as a drought escape mechanism in plants: How can it aid wheat production? Frontiers in Plant Science, 8, 1–8. https://doi.org/10.3389/fpls.2017.01950
- Shiferaw, B., Smale, M., Braun, H.-J., Duveiller, E., Reynolds, M., & Muricho, G. (2013). Crops that feed the world 10. Past successes and future challenges to the role played by wheat in global food security. Food Security, 5(3), 291–317. https://doi.org/10.1007/s12571-013-0263-y
- Shim, J. S., Park, S.-H., Lee, D.-K., Kim, Y. S., Park, S.-C., Redillas, M. C. F. R., Seo, J. S., & Kim, J.-K. (2021). The rice glycine-rich protein 3 confers drought tolerance by regulating mRNA stability of ROS scavenging-related genes. Rice, 14(1), 31. https://doi.org/10.1186/s12284-021-00473-0
- Singh, A., Singh, D., Kang, J. S., & Aggarwal, N. (2011). Management practices to mitigate the impact of high temperature on wheat: A review. IIOAB Journal, 2, 11–22.
- Singh, R., Singh, S., Parihar, P., Mishra, R. K., Tripathi, D. K., Singh, V. P., Chauhan, D. K., & Prasad, S. M. (2016). Reactive oxygen species (ROS): Beneficial companions of plants’ developmental processes. Frontiers in Plant Science, 7, 1–19. https://doi.org/10.3389/fpls.2016.01299
- Sperry, J. S., Wang, Y., Wolfe, B. T., Mackay, D. S., Anderegg, W. R. L., McDowell, N. G., & Pockman, W. T. (2016). Pragmatic hydraulic theory predicts stomatal responses to climatic water deficits. New Phytologist, 212(3), 577–589. https://doi.org/10.1111/nph.14059
- Storme, N. D., & Geelen, D. (2014). The impact of environmental stress on male reproductive development in plants: biological processes and molecular mechanisms. Plant, Cell & Environment, 37(1), 1–18. https://doi.org/10.1111/pce.12142
- Tatar, Ö., Brück, H., & Asch, F. (2016). Photosynthesis and remobilization of dry matter in wheat as affected by progressive drought stress at stem elongation stage. Journal of Agronomy and Crop Science, 202(4), 292–299. https://doi.org/10.1111/jac.12160
- Trouverie, J., Thévenot, C., Rocher, J. P., Sotta, B., & Prioul, J. L. (2003). The role of abscisic acid in the response of a specific vacuolar invertase to water stress in the adult maize leaf. Journal of Experimental Botany, 54(390), 2177–2186. https://doi.org/10.1093/jxb/erg234
- V, P., Ali, K., Singh, A., Vishwakarma, C., Krishnan, V., Chinnusamy, V., & Tyagi, A. (2019). Starch accumulation in rice grains subjected to drought during grain filling stage. Plant Physiology and Biochemistry, 142, 440–451. https://doi.org/10.1016/j.plaphy.2019.07.027
- Vesala, T., Sevanto, S., Grönholm, T., Salmon, Y., Nikinmaa, E., Hari, P., & Hölttä, T. (2017). Effect of leaf water potential on internal humidity and CO2 dissolution: Reverse transpiration and improved water use efficiency under negative pressure. Frontiers in Plant Science, 8, 1–10. https://doi.org/10.3389/fpls.2017.00054
- Waraich, E. A., Ahmad, R., Halim, A., & Aziz, T. (2012). Alleviation of temperature stress by nutrient management in crop plants: A review. Journal of Soil Science and Plant Nutrition, 12(2), 221–244. https://doi.org/10.4067/S0718-95162012000200003
- Wiśniewski, K., & Zagdańska, B. (2001). Genotype-dependent proteolytic response of spring wheat to water deficiency. Journal of Experimental Botany, 52(360), 1455–1463. https://doi.org/10.1093/jexbot/52.360.1455
- Worch, S., Rajesh, K., Harshavardhan, V. T., Pietsch, C., Korzun, V., Kuntze, L., Börner, A., Wobus, U., Röder, M. S., & Sreenivasulu, N. (2011). Haplotyping, linkage mapping and expression analysis of barley genes regulated by terminal drought stress influencing seed quality. BMC Plant Biology, 11, 1–14. https://doi.org/10.1186/1471-2229-11-1
- Wu, Q., & Ying-Ning, Z. (2019). Arbuscular mycorrhizal fungi and tolerance of drought stress in plants chapter 2 arbuscular mycorrhizal fungi and tolerance of drought stress in plants. https://doi.org/10.1007/978-981-10-4115-0
- Yang, J., & Zhang, J. (2006). Grain filling of cereals under soil drying. New Phytologist. 169(2), 223–236. https://doi.org/10.1111/j.1469-8137.2005.01597.x
- Yi, B., Zhou, Y-f., Gao, M-y., Zhang, Z., Han, Y., Yang, G-d., Xu, W., & Huang, R-d (2014). Effect of drought stress during flowering stage on starch accumulation and starch synthesis enzymes in sorghum grains. Journal of Integrative Agriculture, 13(11), 2399–2406. https://doi.org/10.1016/S2095-3119(13)60694-2
- Yu, J., Jiang, M., & Guo, C. (2019). Crop pollen development under drought: From the phenotype to the mechanism. International Journal of Molecular Sciences, 20(7), 1550. https://doi.org/10.3390/ijms20071550
- Yu, X. (2015). Effect of drought stress on the development of endosperm starch granules and the composition and physicochemical properties of starches from soft and hard wheat. https://doi.org/10.1002/jsfa.7439
- Zhang, J., & Davies, W. (2023). Increased synthesis of ABA in partially dehydrated root tips and ABA transport from roots to leaves. Journal of Experimental Botany, 38, 2015–2023.
- Zhang, Z., Hu, M., Xu, W., Wang, Y., Huang, K., Zhang, C., & Wen, J. (2021). Understanding the molecular mechanism of anther development under abiotic stresses. Plant Molecular Biology, 105(1–2), 1–10. https://doi.org/10.1007/s11103-020-01074-z
- Zhao, W., Liu, L., Shen, Q., Yang, J., & Han, X. (2020). Water effects of water stress on photosynthesis, yield, 1–19.[AQ]
- Zheng, B., Chenu, K., Fernanda Dreccer, M., & Chapman, S. C. (2012). Breeding for the future: What are the potential impacts of future frost and heat events on sowing and flowering time requirements for Australian bread wheat (Triticum aestivium) varieties? Global Change Biology, 18(9), 2899–2914. https://doi.org/10.1111/j.1365-2486.2012.02724.x
- Zivcak, M., Brestic, M., Balatova, Z., Drevenakova, P., Olsovska, K., Kalaji, H. M., Yang, X., & Allakhverdiev, S. I. (2013). Photosynthetic electron transport and specific photoprotective responses in wheat leaves under drought stress. Photosynthesis Research, 117(1–3), 529–546. https://doi.org/10.1007/s11120-013-9885-3
- Zohaib, A., Tabassum, T., Jabbar, A., Anjum, S. A., Abbas, T., Mehmood, A., Irshad, S., Kashif, M., Nawaz, M., Farooq, N., Nasir, I. R., Rasool, T., Nadeem, M., & Ahmad, R. (2018). Effect of plant density, boron nutrition and growth regulation on seed mass, emergence and offspring growth plasticity in cotton. Scientific Reports, 8(1), 10489. https://doi.org/10.1038/s41598-018-26308-5
- Zörb, C., Ludewig, U., & Hawkesford, M. J. (2018). Perspective on wheat yield and quality with reduced nitrogen supply. Trends in Plant Science. 23(11), 1029–1037. https://doi.org/10.1016/j.tplants.2018.08.012