Abstract
Cucurbitaceae, the gourd family of flowering plants, is a very large and diverse family, the order Cucurbitales, contains 95 genera and 950–980 species of food and ornamental plants, and wild and weedy species mostly with high genetic diversity. This review is focused on the most important cucurbit crops (Cucumis sativus, Cucumis melo, Cucurbita spp., Citrullus lanatus, Momordica charantia, Lagenaria siceraria, and Luffa acutangula) and some their wild relatives as hosts of cucurbit powdery mildews (CPM). Powdery mildews (PM) (Ascomycota, Erysiphales) are one of the most frequently encountered and easily visible groups of plant pathogenic fungi with > 900 species. They are obligate biotrophs, they colonize above-ground plant tissues, mostly leaves, though they may also colonize stems, petioles, flowers and fruits, and they are usually debilitators, not killers. These parasitic fungi have been problematic on cucurbits for a long time world-wide, causing serious economic losses in yield and quality. All economically important cucurbit crops host CPM. Seven PM species with different taxonomic positions, host ranges, geographic distributions and ecological requirements are known on cucurbits. CPM species taxonomy and denomination rapidly changed during last few decades through detailed analyses and clarifications. At least three PM species frequently parasitize cucurbits: the endoparasite Leveillula taurica (Lt) with marginal economic importance; and two ectoparasitic species, Golovinomyces orontii (Go), and Podosphaera xanthii (Px), which are economically important world-wide. The two pathogens differ in ecological requirements and distribution, though they may occur together in mixed infections. They are highly variable at the population level for virulence, race identities, and fast adaptation of pathogens. Cucurbit-CPM species interactions are diverse and complicated, and differ between hosts and their respective pathogen genera and species. Here we present a critical overview of obstacles, gaps and recent progress in these matters for six cucurbit genera with respect to resistance resources, genetics of resistance, genetic mapping and development of molecular markers, physiology and mechanisms of resistance, developments in mlo-mediated resistance, patents, and CPM resistance breeding.
I. Introduction
A. General aspects of host-pathogen interactions in powdery mildews
Powdery mildews (phylum Ascomycota, order Erysiphales) are one of the most frequently encountered plant pathogenic fungi (Glawe, Citation2008) with > 900 species. This group of fungi infects ca. 10,000 species of angiosperms (9,000 dicotyledonous and 650 monocotyledonous species) (Braun and Cook, Citation2012; Kiss et al., Citation2020). This makes them a major concern in agriculture and horticulture with one included in the top ten fungal plant pathogens (Dean et al., Citation2012). Powdery mildews are obligate biotrophs, meaning they are fully dependent on living host tissue. They epiphytically colonize above-ground plant tissues, mostly leaves, but also stems, petioles, flowers and fruits; they are debilitators not killers. When the plant remains alive during nutrient exchanges, we are speaking in such case about biotrophic interactions (Spanu and Kämper, Citation2010). Biotrophy is thus contrasted with necrotrophy, i.e., when the phytopathogens kill plant cells and tissues, to feed off the remains (Glazebrook, Citation2005; Spanu and Panstruga, Citation2017). Biotrophic interactions elicit hypersensitive responses in the host, in which a few cells undergo a strong local immune reaction, including programmed cell death (cell necrosis) at the site of infection, and which in particular stops biotrophic fungi from spreading into surrounding tissue (Saur and Hückelhoven, Citation2021).
The Erysiphales exhibit a very wide range of host specificity, ranging from broad polyphagy observed in many of the dicot mildews to the extremely narrow host ranges of Blumeria graminis, where the formae speciales (f. sp.) tritici and hordei can infect only wheat and barley, respectively (Wyand and Brown, Citation2003). Other Erysiphales cause powdery mildews, which affect many high-value fruits and vegetables, including cucurbitaceaous vegetables, grapes, strawberries and tomato (Lebeda et al., Citation2017). Their biology, etiology and pathology are, overall, similar to those of the cereal mildews (Dean et al., Citation2012).
Plant-powdery mildew interactions elicit two basic types of resistance reactions: nonhost resistance and host resistance. Host resistance is multifaceted: race-specific (vertical), race-nonspecific (partial, horizontal), field resistance, age-dependant resistance, and mlo resistance (Lebeda, Citation1984a; Bushnell, Citation2002; Panstruga and Schulze-Lefert, Citation2002; Niks and Marcel, Citation2009; Kush and Panstruga, 2017; Panstruga and Moscou, Citation2020). Cucurbits exhibit all of these resistance reactions in response to cucurbit powdery mildews (CPM) (Jahn et al., Citation2002; Lebeda et al., Citation2021a), though in many cases our knowledge is limited. The most commonly known resistance reaction across host species is the gene-for-gene relationship (race-specific interaction) between host plants and avirulence in powdery mildews, where complete defence against infection is exhibited when the host resistance allele matches a specific avirulence allele in the powdery mildew pathogen (Brown, Citation2002).
Powdery mildew fungi are variable in their virulence as expressed by races (or pathotypes), and at individual plant and population levels. This is well documented in many studies of Blumeria graminis (e.g., Dreiseitl, Citation2019), as well as in various other powdery mildews e.g., Podosphaera macularis (Block et al., Citation2021; Gent et al., Citation2020), and cucurbit powdery mildews, Golovinomyces orontii and Podosphaera xanthii (Lebeda et al., Citation2011; Citation2016a; Citation2016b; Citation2018a; Citation2021a). With the exception of B. graminis, the knowledge of virulence variation, their background, populations genetics and spatiotemporal dynamics of other powdery mildews is rather limited (Lebeda et al., Citation2021a). The species and virulence diversity of powdery mildews in many parts of the world is, in general, significantly underestimated (Glawe, Citation2008). Little is known about the requirements for pathogenicity of this class of phytopathogens at the molecular level, mainly because of their biotrophic nature (Barsoum et al., Citation2020).
The main purpose of this article is to provide a comprehensive and critical overview of the biology, variation and genetics of interactions between some cucurbits and CPM as a background for a better understanding of host-pathogen interactions and efficient resistance breeding.
B. Host genera of cucurbits
1. Taxonomy of the group
Cucurbitaceae, the gourd family of flowering plants, as a host family of various powdery mildews (Braun and Cook, Citation2012), belonging to the order Cucurbitales, contains 95 genera and 950–980 species of food and ornamental plants, and wild and weedy species (Schaefer and Renner, Citation2011). Phylogenetic relationships in the order Cucurbitales and the phylogeny and classification of its taxonomically most problematic family, Cucurbitaceae, have been the focus of previous studies (e.g., Jeffrey, Citation2005; Zhang et al., Citation2006; Schaefer et al., Citation2009). Schaefer and Renner (Citation2011) conducted a deep molecular study that recircumscribed tribes and genera within Cucurbitaceae and resulted in a more natural classification for the order Cucurbitales. They described 15 tribes of Cucurbitaceae, with summaries of their main morphological characters, ecologies, chromosome numbers, numbers of genera and species, and geographic distributions.
2. Diversity and distribution
Members of the family are annual or perennial herbs native to temperate and tropical areas and include important crops: cucumbers, gourds, melons, squashes, and pumpkins. Most species are extremely sensitive to temperatures near freezing, a factor that limits their geographic distribution and area of cultivation. The order Cucurbitales in the eurosid I clade comprises almost 2,600 species in 109 or 110 genera in eight tropical and temperate families of very different sizes, morphologies, and ecologies (Zhang et al., Citation2006; Filipowicz and Renner, Citation2010; Schaefer and Renner, Citation2011).
3. Biogeography
Cucurbitaceae are the most diverse in tropical and sub-tropical regions with hotspots in Southeast Asia, West Africa, Madagascar, and Mexico (Schaefer and Renner, Citation2011). They are of Asian origin and probably originated in the Late Cretaceous, some 60 million years ago (Schaefer et al., Citation2009). Long-distance dispersal played an important role in the biogeographical history of Cucurbitaceae (Schaefer et al., Citation2009).
4. Economic importance
Out of the ca. 1,000 species of Cucurbitaceae, 10 are of worldwide economic importance, cultivated globally (Robinson and Decker-Walters, Citation1997; Rubatzky and Yamaguchi, Citation1997), and are here considered “major crops” such as melon (Cucumis melo L.), cucumber (C. sativus L.), watermelon (Citrullus lanatus (Thunb.) Matsumura and Nakai), and squash and pumpkin (Cucurbita L.). Another 23 species of more local commercial importance, are often cultivated in their native range, and might be called “minor crops” such as bitter gourd (Momordica charantia L.), bottle gourd (Lagenaria siceraria (Molina) Standley), wax gourd (Benincasa hispida (Tunb.)), snake gourd (Trichosanthes spp.), and sponge and ridge gourd (Luffa spp.). They are also eaten as vegetables or seeds, used as sources of oils or medicines, or purposed as sponges or containers (Chomicki et al., Citation2020; Grumet et al., Citation2021).
5. Preserving of genetic diversity
Domestication of cucurbits started ca. 11,000 years ago in the New World and Asia, and apparently more recently in Africa. Some cucurbit crops were domesticated only once, others multiple times (e.g., melon from different Asian and African populations) (Chomicki et al., Citation2020). Squashes and pumpkins (Cucurbita spp.) were domesticated in the New World in pre-Columbian times. Study of cucurbit domestication in recent years has benefited from the increasing integration of archaeological and genomic data with insights from herbarium collections, the most efficient way to understand a species’ natural geographic ranges and climate adaptations.
Most domesticated cucurbit species are susceptible to fungal, bacterial and viral diseases, and insect pests (Keinath et al., Citation2017). Plant breeders therefore screen landraces and wild relatives of these crops for resistance traits that can be used in crop improvement (Lebeda et al., Citation2007a; Endl et al., Citation2018; Grumet et al., Citation2021). One of the primary strategies to counteract loss and preserve genetic diversity has been the establishment of ex situ germplasm banks, typically housing seeds collected from around the world with an emphasis on centers of diversity. Germplasm repositories with significant cucurbit holdings are documented in GeneSys (www.genesys-pgr.org); others are listed and cited in an article by Grumet et al. (Citation2021). Maintaining a collection requires appropriate storage conditions, regular viability testing, and regeneration of seed supplies when seed numbers or percent viability is low. Risks include loss of viability and genetic erosion through nonrandom viability during regeneration (Fu, Citation2017).
II. Main cucurbit genera and species as hosts of powdery mildew
Nesom (Citation2011) wisely remarked that differences in biology and patterns of evolution, as well as degrees of subjectivity in judgment, make it difficult to apply a consistent system of taxonomic ranks across all main Cucurbitaceae genera. The challenges for classification are instances where a wild progenitor/domesticated derivative relationship is hypothesized to exist as a result of artificial selection, e.g., several examples in Cucurbita where two paired taxa are morphologically distinct but molecularly identical or near-identical.
A. Cucumis
Species of Cucumis L. are characterized by a trailing, climbing or bushy growth habit, simple petiolate and mostly three- to five-lobed leaf blades, unisexual (rarely androgynous) inflorescences, triplicate sigmoid anther thecae, and fleshy often tuberculate or spiny fruits with many compressed whitish seeds. According to the most recent taxonomical study genus Cucumis belong to tribe Benincaseae (Schaefer and Renner, Citation2011).
In a revision of the genus by Kirkbride (Citation1993), 32 species were recognized, plus one additional species described by Thulin and Al-Gifri (Citation1994). Renner et al. (Citation2007) considered that Cucumis as circumscribed by Kirkbride (Citation1993) as paraphyletic and proposed merger of Cucumella Chiov., Dicoelospermum C.B.Clarke, Mukia Arn., Myrmecosicyos C. Jeffrey and Oreosyce Hook.f. with Cucumis to make the genera monophyletic. Ghebretinsae et al. (Citation2007a) confirmed that above mentioned genera were nested within Cucumis. The nesting of the five genera in Cucumis greatly changed the natural geographic range of the genus, extending it throughout the Malesian region and into Australia (Renner et al., Citation2007). These authors also supposed that the closest relative of Cucumis is Muellerargia, with one species in Australia and Indonesia, and a second in Madagascar. Molecular clocks indicate that the deepest divergences in Cucumis, including the split between C. melo and its Australian/Asian sister clade, go back to the mid-Eocene.
Cucumis was previously partitioned into two subgenera according to species origins and basic chromosome numbers (Zhuang et al., Citation2006). The first was subgen. Melo, comprised mainly of African species (2n = 2x = 24), including 30 type species grouped into six series: Humifructuosi, Melo, Hirsuti, Metuliferi, Angurioidei, and Myriocarpi. The second was subgen. Cucumis containing two species of Asian origin: C. sativus L. (2n = 2x = 14), and C. hystrix Chakr. (2n = 2x = 24). Clear division between the 2n = 2x = 14 species (C. sativus) and the 2n = 2x =24 ones (C. melo and wild species) were confirmed by analysis of nuclear ribosomal DNA ITS regions (Garcia-Mas et al., Citation2004). The number of species in the newly circumscribed Cucumis increased from 33 to 52. Ghebretinsae et al. (Citation2007b) proposed new combinations and new names within the expanded genus Cucumis. Telford et al. (Citation2011a) employed molecular phylogenetic analysis of numerous accessions to show that Cucumis comprises 25 species in Asia, the Malesian region, and Australia, rather than just two, as traditionally thought. An updated taxonomical study of species of this genus is, however, needed, as there are currently 61 accepted species in genus Cucumis, according to Plants of the World Online (Citation2023).
From the viewpoint of human use, the genus Cucumis includes cultivated species cucumber (C. sativus), melons (C. melo), the horned melon (C. metuliferus), and the West Indian gherkin (C. anguria). Some publications focused on detailed characterization and description of rarer Cucumis species (C. setosus, C. callosus, C. silentvalleyi, C. indicus (John et al., Citation2013; Citation2017), or species from Australia and Eastern Malesia (C. costatus, C. queenslandicus, C. umbellatus, and C. variabilis; Telford et al., Citation2011a). Phylogenetic relationships for the Cucumis genus constructed using chloroplast genes clarified the homologies between the wild and cultivated species, providing a theoretical basis for the genetic improvement of cultivated melon and cucumber using wild resources (Chung et al., Citation2006; Sebastian et al., Citation2010).
1. Cucumber (Cucumis sativus)
Cucumber (Cucumis sativus L.), one of the most economically important fresh and processed vegetables, is characterized by large leaves, thin tendrils, and a typically elongated, green-skinned fruit with tapered ends. C. sativus is less morphologically variable than C. melo, however in Europe and USA two different kinds of fruits are common: processing or pickling cucumbers (smaller), and fresh-market, a.k.a. slicing (longer) (Lv et al., Citation2012). Cucumber is native to the Indian subcontinent, and was carried eastward and grown in China ca. 2000 years ago. Paris et al. (Citation2012a) suggest that cucumber was introduced to Europe by two independent diffusions; the first was via Persia into eastern and northern Europe preceding the Islamic conquests, and the subsequent diffusion was probably by a mostly maritime route from Persia or the Indian subcontinent into Andalusia.
Four cross-compatible botanical varieties of C. sativus have been described: the cultivated var. sativus; wild cucumber var. hardwickii (Royle) Alef; semiwild var. xishuangbannanesis Qi et Yuan (Xishuangbanna cucumber), and var. sikkimensis Hook (Sikkim cucumber). The Xishuangbanna cultivar (C. sativus var. xishuangbannanensis) was selected for high β-carotene content in the mature pulp; its fruits are eaten boiled or raw during different stages of maturity (Renner, Citation2017). Varieties sativus, xishuangbannanesis, and sikkimensis all share several chromosomal inversions and translocations in relationship to wild var. hardwickii, suggesting chromosomal changes during domestication (Yang et al., Citation2012; Grumet et al., Citation2021).
Cucumis hardwickii was previously considered a distinct species. Sebastian et al. (Citation2010) discussed that C. hardwickii sometimes has been posited as the ancestor of C. sativus, because these two species alone in the genus share the derived chromosome number of 2n = 14. The geographic range of C. hardwickii is from northwestern Himalayas southward into the Eastern and Western Ghats and the central Plateau region of India. de Wilde and Duyfjes (Citation2010) confirmed that C. hardwickii is not sharply demarcated from feral forms of C. sativus and reduced its formal rank within C. sativus to forma. On the other hand, Meglic et al. (Citation1996) found that C. hardwickii is isozymically distinct from a large number of C. sativus samples. Qi et al. (Citation2013) state that cucumbers have been domesticated from wild Indian C. sativus var. hardwickii.
There is a strong cross incompatibility between melon (C. melo L.) and cucumber (Zhuang et al., Citation2006). The only other species (with different chromosome number) that has been successfully crossed with cucumber is C. hystrix (2n = 24), though with limited success as the chromosomal differences cause significant barriers to obtaining viable seed (Chen et al., Citation1997; Lebeda et al., Citation1999; Skálová et al., Citation2004). Chromosome doubling of the F1 sativus × hystrix plants restored partial or near-full fertility to progeny, and further fertility selection resulted in the production of a primary amphidiploid (2n = 4x = 38) that regularly produced fertile flowers and set fruit with viable seeds, and gave rise to the nothospecies Cucumis × hytivus J.-F. Chen & J. H. Kirkbr., nothsp. nov. (Chen and Kirkbride, Citation2000). Cucumis × hytivus may serve as a bridge species for increased cross-compatibility with C. sativus and C. hystrix, raising the potential for eventual introgression of valuable traits (Chen et al., Citation2003). The new synthetic species itself may be useful for further study of the genetic relationship between the two basic chromosome numbers n = 7 and n = 12 in Cucumis (Zhuang et al., Citation2006).
Due to its narrow genetic base, the intraspecific genetic diversity in cucumber is relatively low (3–12%) compared with other Cucumis species as revealed in early studies with marker types such as isozymes, RFLPs, AFLPs or RAPDs (Knerr et al. Citation1989; Dijkhuizen et al. Citation1996; Meglic and Staub, Citation1996; Meglic et al. Citation1996; Staub et al. Citation1997; Cavagnaro et al. Citation2010). Karakurt et al. (Citation2020) revealed low molecular diversity among included cucumber accessions, as indicated by AFLP and morphological characters (plant morphology, plant length, length of leaf blade, fruit length, fruit diameter and fruit stem length). Indian cucumber germplasm is most heterogeneous based on SSR analysis of genetic diversity and population structure of C. sativus (Lv et al., Citation2012).
2. Melon (Cucumis melo)
Melon (Cucumis melo L.) is an annual trailing herb with edible, fleshy fruit that may be consumed as a dessert fruit (fresh or dried), a fresh or cooked vegetable, or pickled; seeds may be used as a source of oil or protein meal. Melon varies greatly for vegetative (e.g., leaf size and shape), flower (e.g., color, size) and fruit characters (e.g., size, shape, surface features, color, texture, taste, composition). Pitrat (Citation2013) stated that cultivated melons present a wide range of phenotypic polymorphism compared with that of wild melons. Jeffrey (Citation1980) informally described two subspecies, subsp. melo, and subsp. agrestis, based on length of ovary pubescence. Kirkbride (Citation1993) updated the genus Cucumis and formalized the two C. melo subspecies. The recently designated C. melo subsp. meloides (also informally called African agrestis) is restricted to Africa (Endl et al., Citation2018). Two or three domestication events have been proposed for melon, one or two in Asia (perhaps both in India) and one in Africa (Endl et al., Citation2018; Sebastian et al., Citation2010; Zhao et al. Citation2019). The closest wild relatives of melon were found in Australia (Sebastian et al., Citation2010).
Pitrat et al. (Citation2000) provided an excellent overview of intraspecific melon nomenclature (largely repeated with some refinements by Burger et al., Citation2010) and proposed 16 botanical varieties in ssp. agrestis (5) and melo (11). Nesom (Citation2011) revisited the subject and proposed 15 varieties, seven in ssp. agrestis and four in ssp. melo. Lija and Beevy (Citation2021) provided some interesting insights of 17 varieties in their reiteration of Pitrat et al. (Citation2000) with addition of the recently proposed var. alwarensis A. Pandey & S. Rajkumar var. nov. (Pandey and Rajkumar, Citation2021).
Kirkbride (Citation1993) stated that because of the tremendous man-induced and -maintained variability of the fruit, melon cultivar groups should be treated under the code of nomenclature for cultivated plants (Brickell et al. Citation1980), not under the code of nomenclature for botanical names (Greuter et al., Citation1988). Viewed from this angle, Pitrat (Citation2017) proposed a scheme based on Horticultural Groups. Burger et al. (Citation2010) placed Horticultural Groups within the two recognized subspecies.
Sebastian et al. (Citation2010) indicated that both Cucumis trigonus Boiss. and Cucumis callosus (Rottler) Cogn. are morphologically distinct species but with DNA sequences “nearly identical to those of C. melo.” Status of C. callosus as the wild progenitor of melon is a matter of interpretation (Nesom, Citation2011; John et al., Citation2013).
The phylogenetic relationships among various groups of cultivated melon and its genetic diversity was studied by analysis of morphological characters (Kirkbride, Citation1993), cytological characters (Dane, Citation1991) and molecular polymorphism by DNA markers, such as isozymes (Akashi et al., Citation2002), restriction fragment length polymorphisms (RFLP) (Neuhansen, Citation1992), random amplified polymorphic DNA (RAPD) (e.g., Staub et al., Citation2000), simple sequence repeats or microsatellites (SSR) (e.g., Garcia-Mas et al., Citation2004), Amplified fragment length polymorphism (AFLP) (Yashiro et al., Citation2005) and SNP (Single Nucleotide polymorphism) by genotyping by sequencing (e.g., Kishor et al., Citation2020). In the study of Cui et al. (Citation2020) the population structure and genetic diversity of C. melo species were investigated using denser SNP markers of chloroplasts; the 192 SNPs effectively divided the melon populations into three groups: wild, thick-rind type cultivated melon, and thin-rind type cultivated melon using a comprehensive phylogenomic approach, that included reconstruction of the complete collection of evolutionary histories of all melon protein-coding genes across a phylogeny of 23 sequenced plants. This approach detected several gene families that expanded specifically in the Cucumis and C. melo lineages.
B. Cucurbita spp. (Cucurbita pepo, Cucurbita maxima, Cucurbita moschata, others Cucurbita spp.)
Cucurbita L. (tribe Cucurbiteae, 2n = 2x = 40) generally have herbaceous vines that grow to several meters in length and have tendrils, but some cultivars, particularly in C. pepo, have a bush plant habit with reduced tendrils (Robinson and Decker-Walters, Citation1997). They have yellow or orange flowers (corolla) of two types: female and male. Some species are pollinated by bees, sometimes also by honey bees (Abu-Hamour and Wittmann, Citation2010). Genus Cucurbita is native to the New World and includes several mesophytic annual species and perennial xerophytic species, which occupy diverse habitats from the midwestern United States to southern Argentina. The greatest species diversity occurs in Mexico (Lira et al., Citation2016), although phylogenetic data suggest that the genus likely originated in Central or South America (Schaefer et al., Citation2009). The xerophytic perennials occur in deserts and dry scrub of Mexico and the southwest United States (Nee, Citation1990), and may represent the ancestral habit of the genus (Kates et al., Citation2017). All five domesticated taxa (C. argyrosperma, C. ficifolia, C. maxima, C. moschata, C. pepo) (Paris, Citation2016) originated from mesophytic species, which form a clade and are apparently the result of a very recent radiation (∼7 mya) from a common ancestor in Central or South America (Schaefer et al., Citation2009). Gene flow is common between wild and domesticated Cucurbita and can occur between different species. Wild Cucurbita are still commonly utilized by rural people in Latin America living in proximity to wild populations (Khoury et al., Citation2020).
Taxonomic and phylogenetic studies of this genus are on-going. Although Nee (Citation1990) classified Cucurbita species to 13 species groups (and 27 species total), and Whitaker and Bemis (Citation1975) counted up to 27 Cucurbita species; crossing experiments have revealed free crossability and unimpaired fertility in many cross-combinations (Křístková and Lebeda, Citation1997; Gong et al., Citation2013). Plants of the World Online (Citation2023) currently lists 19 accepted species.
The genus Cucurbita produces a variety of vegetables, which were part of the pre-Columbian American agricultural system (Smith, Citation2006). Sanjur et al. (Citation2002) suggest, based on mitochondrial gene analyses, at least six independent domestication events from distinct wild ancestors. C. argyrosperma likely was domesticated from a wild Mexican gourd, C. sororia (recently synonymized with C. argyrosperma subsp. sororia (L. H. Bailey) L. Merrick & D. M. Bates), probably in the region of southwest Mexico. The wild ancestor of C. moschata (domesticated in low land northern South America) is still unknown, C. andreana is supported as the wild progenitor of C. maxima (domesticated in Andes). There were confirmed two separate domestications in the C. pepo complex; one is presumed to have occurred 10,000 years ago in Mexico, and corresponds to C. pepo ssp. pepo possibly from C. pepo ssp. fraterna (Castellanos-Morales et al., Citation2018; Citation2019). The second domestication event could have taken place in eastern North America and should be extended to north-eastern Mexico for C. pepo ssp. ovifera var. ovifera, likely from C. pepo ssp. ovifera var. ozarkana (Sanjur et al., Citation2002).
Kates et al. (Citation2017) identified the wild progenitors for three of the six crop taxa: C. argyrosperma subsp. sororia is considered to be the wild ancestor of cushaw (C. argyrosperma), C. maxima Duchesne subsp. andreana (Naudin) Filov (basionym C. andreana) the wild ancestor of buttercup squash (C. maxima) and the wild varieties of C. pepo L. subsp. ovifera (L.) D. S. Decker [var. ozarkana D. S. Decker and var. texana (Scheele) Filov] are likely ancestral to acorn squash (C. pepo). A third lineage of wild C. pepo, C. pepo (L.) subsp. fraterna (L.H. Bailey) Lira (syn. C. melopepo var. fraterna (L. H. Bailey) G. L. Nesom) is also a primary relative, though it is not considered ancestral to any extant Cucurbita crop taxon (Kates et al., Citation2017). However, there is also genetic evidence indicating that C. pepo ssp. fraterna is more closely related to C. pepo ssp. ovifera than to C. pepo ssp. pepo (Kates et al., Citation2017).
The wild progenitors of the remaining crop taxa are most likely extinct though possibly rare and uncollected (Kistler et al., Citation2015), e.g., the domesticated species C. ficifolia Bouché and C. moschata Duchesne have unique haplotypes and no wild progenitor has been proposed for either (Nesom, Citation2011).
Distribution and cultivation throughout the world have resulted in secondary centers of Cucurbita diversity in Europe and Asia: Europe for C. pepo var. pepo; India and Myanmar for C. moschata, and Japan and China for C. maxima (Grumet et al., Citation2021).
Cucurbita pepo is the most economically important species and according to Martínez-González et al. (Citation2021), consists of five taxa and three crop wild relatives: C. pepo ssp. fraterna, C. pepo ssp. ovifera var. texana, and C. pepo ssp. ovifera var. ozarkana; and two cultivated: C. pepo ssp. pepo and C. pepo ssp. ovifera var. ovifera. Some genetic resources of C. pepo and C. maxima are also known with multiple disease resistance (Křístková and Lebeda, Citation1998).
Gene flow between different crops and sympatric crop wild relatives for C. pepo has been studied (Decker-Walters et al., Citation2002). Gene flow between domesticated and wild species (e.g., C. argyrosperma ssp. sororia and C. pepo ssp. fraterna (syn. C. melopepo var. fraterna) has been reported as common in Cucurbita (Sánchez-de la Vega et al., Citation2018). Martínez-González et al. (Citation2021) estimated gene flow using SNPs among 14 Mexican traditional landraces of C. pepo ssp. pepo, also including individuals from five improved cultivars of C. pepo ssp. pepo and C. pepo ssp. ovifera var. ovifera, and individuals of their wild relative C. pepo ssp. fraterna (syn. C. melopepo var. fraterna). Their results suggest that gene flow between these subspecies and cultigens, incomplete lineage sorting, and the retention of ancestral characters shaped the evolutionary trajectory of C. pepo in its area of origin and diversification. They also found evidence of the use of Mexican landraces as genetic material for the improvement of commercial cultivars.
Complete chloroplast genome sequencing of C. pepo, and phylogenetic analysis by Zhang et al. (Citation2018a) showed that C. pepo was closely related to other species of the family Cucurbitaceae, i.e., closer to Gynostemma than Citrullus, Coccinia, and Cucumisin. Four chloroplast loci were used to estimate the phylogeny of 23 taxa that represent the broad-level diversity within Cucurbita. The chloroplast evidence provides strong support for a novel grouping of the mesophytic annual C. ficifolia (known only from cultivation) with the xerophytic perennials C. foetidissima and C. pedatifolia. They also provided the first DNA evidence in support of the isozyme-based hypothesis that C. pepo subsp. ovifera var. ovifera (represented by most ornamental gourds and several squashes) was domesticated from the wild taxon C. pepo subsp. ovifera var. ozarkana.
Paris (Citation2018) comprehensively reviewed various aspects of exploitation and conservation of genetic resources of pumpkins and squash (Cucurbita spp.). There is a wide array of Cucurbita morphotypes based on variation in fruit shape, size, surface texture and color ().
Table 1. Commonly produced Cucurbita fruit morphotypes (Paris et al., Citation2012b; Khoury et al., Citation2020; Grumet et al., Citation2021).
Tropical pumpkin (C. moschata) is a commercially and nutritionally important crop cultivated widely. In India solely, the pumpkin seed market is large (Dhillon et al., Citation2020b) and in Bangladesh, pumpkins follow radish in area under cultivation (Ahamed et al., Citation2011). Matured fruits are consumed boiled, steamed, or in soups and purees. In Zambia, 40% of households use pumpkin leaves daily (Ngwerume, Citation2004) and pumpkin seeds are roasted and eaten as snack as well.
IUCN (The International Union for Conservation of Nature’s Red List of Threatened Species; Citation2023) Red List assessments addressed the majority of wild Cucurbita; seven taxa were designated Least Concern, while C. ecuadorensis and C. radicans were designated Vulnerable and Endangered, respectively. NatureServe Explorer (Citation2023) assessed conservation status for six wild Cucurbita, of which only C. okeechobeensis subsp. okeechobeensis was considered a conservation concern (see also Khoury et al., Citation2020 for more detailed references). Additionaly, Khoury et al. (Citation2020) summarized (based on Paris and Kabelka (Citation2009), Paris and Brown (Citation2005), and Behera et al. (Citation2012)) wild Cucurbita, their genepool assignments, and published breeding research. Important sources of host plant resistance against viruses (mainly), powdery and downy mildews within Cucurbita are mostly in genepool category 3: C. argyrosperma subsp. sororia, C. cordata, C. digitata, C. ecuadorensis, C. foetidissima, C. lundelliana, C. maxima subsp. andreana, C. okeechobeensis subsp. martinezii and okeechobeensis, C. palmata and C. radicans (Paris, Citation2016).
Nesom (Citation2011) differed on these categories and considered entities generally treated as Cucurbita pepo sensu lato apportioned between two species: C. pepo L. (known only from domesticated forms) and C. melopepo L. (known from wild forms and domesticates), emphasizing their geographic and molecular differences. Plants of the World Online (Citation2023) distinguishes the following varieties and subspecies within C. melopepo: var. fraterna (L. H. Bailey) G. L. Nesom, var. ozarkana (Deck.-Walt.) G. L. Nesom, and subsp. melopepo, and subsp. texana (Scheele) G. L. Nesom.
The Khoury et al. (Citation2020) review focused on distributions, conservation status, and abiotic stress tolerance potential of wild cucurbits (Cucurbita L.). In total, with regard to the current state of ex situ conservation, two taxa (C. cordata and C. × scabridifolia) were assessed as high priority for further collecting, nine medium priority (including two putative progenitors), three low priority, and two sufficiently conserved (C. pepo subsp. ovifera var. ozarcana and C. pepo subsp. fraterna). Taxa with very few germplasm accessions conserved in genebanks and botanic gardens, and thus very limited genetic diversity accessible for crop breeding and other research are C. × scabridifolia, C. cordata, C. radicans, and C. pedatifolia (Montes-Hernandez and Eguiarte, Citation2002).
C. Watermelon (Citrullus lanatus)
The genus Citrullus Schrad. ex Eckl. & Zeyh. belongs to the tribe Benincaseae and the native range of this genus is Macaronesia, Mediterranean, Tropical and South Africa, and the Arabian Peninsula to India (Plants of the World Online, Citation2023). These are trailing or scandent annual or perennial herbs with simple, usually deeply lobed leaves, simple tendrils, monoecious, solitary flowers; fruits are subglobose to ellipsoid, firm-walled, fleshy, indehiscent, green or yellowish, often mottled, many-seeded. Citrullus is 2n = 2x = 22 (Paris, Citation2015).
There is a long-standing debate about the taxonomic names of Citrullus species, subspecies and varieties. For detailed nomenclatural history and current species names see Nesom (Citation2011), Renner et al. (Citation2014), Chomicki and Renner (Citation2015). The recent complex taxonomical study of genus Citrullus by Renner et al. (Citation2017) based on morphology (as seen in herbarium specimens), chromosome counts and DNA sequences from type material resulted in the description of seven Citrullus species () that have been accepted by Plants of the World Online (Citation2023).
Table 2. Seven Citrullus species summarized from Renner et al. (Citation2017), all are 2n = 22 except C. naudinianus (2n = 24).
Watermelon (Citrullus lanatus) is consumed throughout the world for its sweet, juicy fruits. It can also be eaten as jams, glazed candy, or pickled rinds (Grumet et al., Citation2021). Cooking type watermelons are used as a multipurpose vegetable in many African countries, leaves and fruits, which may be stored for up to a year, and seed types are used to make snacks, flour, and oil in West Africa (Jensen, Citation2012). Other cultivated taxa of the genus Citrullus, the southern African citron, tsamma, or bitter watermelons (C. amarus), are used as a water source, for cooking, and as animal fodder, the egusi watermelon (C. mucosospermus) is grown for its tasty seeds that are eaten as a raw snack in West Africa; and the colocynth (C. colocynthis) is cultivated for diverse medical purposes since Ancient Egypt (Renner et al., Citation2017).
Watermelon has been domesticated for more than 4,000 years, and has been improved by domestication and modern breeding from wild watermelons with small fruits, harboring hard, pale-colored and bitter- or bland-tasting flesh, into modern sweet watermelons carrying large fruits with crisp sweet and red flesh and a thin rind (Paris, Citation2015). There are theories about place of domestication and the first cultivation of watermelon. According to Paris (Citation2015) northeastern Africa is a primary center of watermelon domestication; and in the first centuries A.D. watermelons, melons, and colocynths were distinguished by Greek, Latin and Hebrew writers. It has been confirmed that sweet watermelon (C. lanatus) originated from western, not southern Africa as previously believed, and that the South African citron melon (C. amarus) was independently domesticated (Chomicki and Renner, Citation2015). The erroneous merging of the bitter watermelon (C. amarus), with the dessert watermelon (C. citrullus), led to numerous subsequent errors, most importantly the assumption that watermelons were domesticated in southern Africa (Renner et al., Citation2017). C. lanatus subsp. cordophanus is considered a probable ancestor of sweet watermelon (Chomicki et al., Citation2020). The possible importance of C. colocynthis as a genetic contributor to the dessert watermelon was debunked (Schweinfurth, Citation1883).
Molecular markers were used in assessing genetic diversity and in classifying Citrullus accessions (Levi et al. Citation2001a). Molecular characterization among and within Citrullus spp. usually results in low genetic variability contrary to the remarkable phenotypic variation. Cultivated watermelon (C. lanatus) exhibited narrow genetic base (Levi et al., Citation2001b) as a result of many years of cultivation and selection for specific qualities. More recent data revealed broad genetic diversity among the Citrullus spp. accessions useful for enhancing disease or pest resistance in watermelon cultivars (Levi et al., Citation2013). Genome and whole genome resequencing of 414 accessions representing all extant species in the Citrullus genus revealed the evolutionary history of Citrullus (Guo et al., Citation2019), suggesting independent evolutions in C. amarus and the lineage containing C. lanatus and C. mucosospermus (close relationship and shared ancestry). Gene flow analysis further suggested admixture between C. mucosospermus and C. lanatus and gene flow also occurred between C. ecirrhosus and C. mucosospermus, and between C. ecirrhosus and C. amarus, whereas no significant gene flow was detected between C. amarus and C. mucosospermus. They also confirmed that a nonbitter allele, arising in the progenitor of sweet watermelon, is largely fixed in C. lanatus. Citrullus colocynthis, C. amarus and C. mucosospermus have been used in breeding programs as new sources of disease and pest resistance for the improvement of sweet watermelon (Guo et al., Citation2019).
D. Bitter gourd (Momordica charantia)
Genus Momordica belongs to tribe Momordiceae (Renner and Schaefer, Citation2017). Plants of the World Online (https://powo.science.kew.org/ 24.7.2023) lists 51 species accepted in genus Momordica, the majority of which are found in Africa with a minority in Asia and Australia (Schaefer and Renner, Citation2010). Chromosome counts of Momordica species indicate two basic numbers: x = 11 and x = 14, dividing the genus into two groups with distinct characteristics (Lombello, Citation2020). Some authors divide the genus into three sections: Momordica, Cochinchinensis and Raphanocarpus (Bharathi et al., Citation2012). Several Momordica species are used and cultivated, often with local importance: M. balsamina L. (balsam apple), M. charantia L. (bitter gourd), M. cochinchinensis (Lour.) Spreng. (gấc), M. cymbalaria Hook., Fenzl ex Naud., M. dioica Roxb. ex Willd. (spine gourd), M. foetida Schumach (Seshadri and More, Citation2009).
The most economically and nutritionally important species commercially cultivated, chiefly by smallholder farmers in Asia, is Momordica charantia L.; 2n= 2x = 22, which is called bitter gourd, but also bitter melon, goya, bitter apple, bitter squash, balsam-pear, cassilla, maiden apple (Dhillon et al., Citation2019). The immature fruits may be fried, stuffed, dried or pickled. Consumer preferences for fruit color, shape, skin pattern, and size vary among and within countries. It is commonly used to improve insulin sensitivity and decrease blood glucose level (Krawinkel and Keding, Citation2006), as well as anthelmintic, digestive, and laxative (Giuliani et al., Citation2016). The ripe fruits are rich in vitamin A, ascorbic acid, magnesium, phosphorus and iron. Fruits also contain two major alkaloids, momordicin and cucurbitacin (Swamy, Citation2023).
This herbaceous, tendril-bearing vine grows up to 5 m in length. Fruit color ranges from white or cream to light green to dark green, and shapes include cylindrical, elliptical, spindle and conical types. Fruits of some varieties develop irregular longitudinal ridges and warty skin. Nearly 20 bitter gourd market types based on these fruit traits are produced in Asia, with nearly half of the types cultivated in India, China, Nepal, Bangladesh, Pakistan, and Sri Lanka. It is also grown in the Caribbean and South America, where it is used as a food and as a medicine. Bitter gourd breeders have been effective in improving fruit yield and quality of the crop in the last decades for various markets (Behera et al., Citation2010); highly bitter fruit are preferred in south Asia, whereas less bitter fruit are preferred in southeast Asian countries. Modern bitter gourd varieties are monoecious (produce separate staminate and pistillate flowers on the same plant) and open-pollinated by bees (Swamy, Citation2023). Schaefer and Renner (Citation2010) stated that species tend to cross-pollinate, which tends to promote phenotypic and genotypic diversity.
Biogeographic analysis suggested that M. charantia was native to Africa (Schaefer and Renner, Citation2010) and was domesticated in eastern India and southern China (Walters and Decker-Walters, Citation1988). Indian M. charantia exhibits a large amount of genetic diversity based on several morphological characters: growth habit, maturity, and various fruit characters such as shape, size, color and surface texture (Robinson and Decker-Walters, Citation1997). According to Chomicki et al. (Citation2020) it remains unclear where this crop was domesticated; more sampling of landraces and wild forms from Madagascar, mainland Africa and Southern India will help to confirm its region of domestication. Cultivated bitter gourd is grouped in two botanical varieties based on fruit size and shape: 1. M. charantia var. charantia—long fusiform fruit (8–30 cm) that do not taper at ends; 2. M. charantia var. muricata—small round fruit (<5 cm) which taper at end (Chakravarty, Citation1990).
Bharathi et al. (Citation2012) studied interspecific hybridization within Momordica using a crossing program among seven Momordica species and two varieties of Momordica charantia. High crossability and pollen fertility were observed in var. charantia × var. muricata, whereas low crossability and moderate pollen fertility were observed in M. charantia × M. balsamina. No crossability barriers were found within the sect. Cochinchinensis. Crosses between the sect. Momordica and Cochinchinensis yielded only parthenocarpic fruits. M. cymbalaria (sect. Raphanocarpus) was neither crossable with sect. Momordica nor sect. Cochinchinensis. Joseph and Antony (2007) studied the ethnobotanical diversity of genus Momordica in southern Western Ghats of India, confirming this area as a megadiversity hotspot for Momordica comprising M. charantia var. muricata and var. charantia, M. dioica and M. sahyadrica.
Genetic diversity of M. charantia was estimated using molecular markers (Dey et al., Citation2006; Prasanth et al., Citation2020). Thirty-eight M. charantia genotypes including cultivars from different parts of India were analyzed using RAPD markers and agronomic traits. The germplasm exhibited very large morphological variation with respect to fruit shape, size and color, though the authors stated the diversity based on yield related traits and molecular analysis was not consonant with ecological distribution. Dhillon et al. (Citation2016a) dealt with genetic characterization of M. charantia based on polymorphisms of 50 simple sequence repeat (SSR) loci in 114 accessions that included landraces, breeding lines, and commercial open-pollinated and F1 hybrid cultivars widely grown in Asia. Accessions formed three subpopulations represented by five clusters. Distribution of accessions across the five clusters reflected their geographic origin to a large extent and was confirmed by Matsumura et al. (Citation2020). These authors confirmed correlations of molecular marker genotypes in terms of genetic distance and geographical origin among the landraces and the relationship of the molecular diversity with fruit phenotypic characteristics. They also revealed that the commercial bitter gourd cultivars for different market segments such as south Asian and southeast Asian types have distinct, narrow genetic bases.
E. Ridge gourd (Luffa acutangula)
Genus Luffa Mill. belongs to tribe Sicyoeae (Renner and Schaefer, Citation2017) and the native range of this genus is Mexico to Tropical America, W. Tropical Africa to Somalia, Indian Subcontinent, and Australia. Filipowicz et al. (Citation2014) in their review (revisiting genus Luffa) distinguished eight species. Plants of the World Online (https://powo.science.kew.org/ 30.7.2023) recognizes nine species in genus Luffa, including L. acutangula (L.) Roxb., L. aegyptiaca Mill., L. astorii Svenson, L. echinata Roxb., L. graveolens Roxb., L. operculata (L.) Cogn., L. quinquefida (Hook. & Arn.) Seem., L. saccata F.Muell. ex I.Telford and Luffa sepium (G.Mey.) C.Jeffrey.
Two species are economically important: ridge gourd (Luffa acutangula) and sponge gourd (Luffa aegyptiaca). Ridge gourd, also known as angled loofah or ribbed gourd is a major cultivated cucurbit in Asia. Native range and wildforms of L. acutangula are known from the Arabian Peninsula and India (Filipowicz et al., Citation2014). India is suggested as the center of origin of ridge gourd (Robinson and Decker-Walters, Citation1997). Plants are viny, monoecious, and with variability for fruit size, shape, and color. The immature fruits are eaten as cooked vegetable in the form of curries, fried, and soups; mature fruits are used as natural cleaning sponges.
The area of domestication of the sponge gourd, L. aegyptiaca, which occurs throughout Southeast Asia, is unknown. Small-fruited wild forms occur in Australia and Indonesia (Chomicki et al., Citation2020). The young fruit is eaten as a vegetable and is commonly grown for that purpose; fully ripened fruits are used to make scrubbing bath sponges. None of the neotropical Luffa species (L. astori, L. operculata, L. quinquefida, L. sepium) has been domesticated (Chomicki et al., Citation2020). One species, L. saccata, occurs in Australia (Telford et al., Citation2011b).
Genetic divesity of Luffa species has been studied by at least six groups: Marr et al. (Citation2005; L. acutangula, L. aegyptiaca; allozymic, morphological, phenological), Tyagi et al. (Citation2016; L. cylindrica; morphological and SRAP markers), An et al. (Citation2017; L. cylindrica; SSR-HRM markers), Misra et al. (Citation2017; Indian Luffa species: L. acutangula, L. aegyptiaca, L. echinata, L. graveolens; ISSR and DAMD markers), Pandey et al. (Citation2017; L. acutangula, L. aegyptiaca, semiwild L. hermaphrodita (recently syn. L. acutangula; microsatelite markers), and Perez et al. (Citation2021; L. acutangula; SNP markers).
F. Bottle gourd (Lagenaria siceraria)
Genus Lagenaria belongs to tribe Benincaseae (Renner and Schaefer, Citation2017). The native range of this genus is Tropical and South Africa, Comoros, and Madagascar. Plants of the World Online (https://powo.science.kew.org/ 18. 08. 2023) lists six Lagenaria species: L. abyssinica (Hook.f.) B. Jefferey, L. breviflora (Benth.) Roberty, L. guineensis (G.Don) C.Jeffrey, L. rufa (Gilg) C.Jeffrey, L. siceraria (Molina) Standl. and L. sphaerica (Sond.) Naudin. Bottle gourd (L. siceraria), the best-known species, has been domesticated by humans, and has spread beyond Africa. The other species are not cultivated.
Bottle gourd, also called white-flowered gourd or calabash, is an annual running or climbing vine, monoecious species with white flowers, hairy stems, and long forked tendrils. Huge variation in fruit shape is known in bottle gourd (Yetişir et al., Citation2008) which includes long and cylindrical, elongated, curved, pyriform, crooked necked, and globular. The young green tender fruits are consumed in curries and fried. Young shoots and tendrils are also cooked and oil is extracted from the seeds. Fresh bottle gourd fruit juice is popular in South Asia for its cooling, and diuretic properties (Minocha, Citation2015). Dhillon et al. (Citation2016b) presented a fascinating account of other uses of mature, dried hard shells of bottle gourd including musical instruments, cups, barrels, milk pails, ladles, fishing floats, penis sheaths, carvings, etc. Bottle gourd is widely used as a rootstock for watermelon grafting due to its natural resistance to Fusarium wilt of watermelon caused by Fusarium oxysporum f. sp. niveum (Keinath and Hassel, Citation2014).
Origin and domestication of bottle gourd is uncertain. It was considered native to Africa (Richardson, Citation1972) and archaeological findings indicated its arrival in Asia and Americas more than 10,000 years ago through human migration (Erickson et al., Citation2005). Renner and Schaefer (Citation2017) reported rind fragments from Mesoamerican archaeological deposits radiocarbon-dated to 10,000 b.p., indicating that bottle gourd was present in the Americas as a domesticated plant by that time. Clarke et al. (Citation2006) demonstrated the likley dual origin of Polynesian bottle gourd accessions: the chloroplast markers were exclusively of Asian origin, but the nuclear markers showed alleles originating in both the Americas and Asia. According to Kistler et al. (Citation2014) this species is of African origin and very likely arrived in Central America with sea currents and was domesticated there some 10,000 years ago.
Diversity of Lagenaria spp. fruit and seed morphology, and RAPD polymorphism was studied by Morimoto et al. (Citation2005; Citation2006). L. siceraria was qualitatively and quantitatively more diverse than its wild relatives (L. sphaerica, L. abyssinica, and L. breviflora), however morphological variations were not associated with RAPD variations. SSR markers developed in C. sativus have been used with limited success to characterize genetic diversity in L. siceraria (Bhawna et al., Citation2015), so this marker set may be useful for evaluating the genetic structure for association mapping, and may be a practical tool for further genetic analyses. Other marker systems have been applied to L. siceraria diveristy analysis: ISSR (Bhawna et al., Citation2014), SDS-PAGE and RAPD (Srivastava et al., Citation2014), and SNP (Contreras-Soto et al., Citation2021).
III. Recent “state of the art” of cucurbit powdery mildews (CPM)
CPM is a world-wide distributed disease of cucurbits, causing serious economic losses (Keinath et al., Citation2017). Early occurrence of CPM on cucurbits can lead to the reduction of photosynthetic capacity of plants, which negatively impacts plant growth and development, and fruit quality (Cohen et al., Citation2004; Lebeda et al., Citation2007a; Citation2009). All economically important cucurbit crops are hosts of CPM (Cohen et al., Citation2004; Křístková et al., Citation2009; Keinath et al., Citation2017, Dhillon et al., Citation2018).
Three ascomycete species from the order Erysiphales parasitize cucurbits and cause CPM. The endoparasitic Leveillula taurica (Lév.) G. Arnaud, which occurs in the Mediterranean basin, primarily, and other countries (e.g., Iran, Japan, Mexico, USA, USSR) (Khodaparast et al., Citation2016; Beltrán-Peňa et al., Citation2018), is of marginal economic importance (Sitterly, Citation1978; Keinath et al., Citation2017). The two ectoparasitic species, Golovinomyces orontii (Castagne) Heluta (Go), and Podosphaera xanthii (Castagne) U. Braun and Shishkoff (Px), are economically important world-wide (Křístková et al., Citation2009; Lebeda et al., Citation2010; Braun and Cook, Citation2012) ( and ). The taxonomy and denomination of individual CPM species rapidly changed through the last decades () and deserves, therefore, a detailed review.
Figure 1. Macroscopic symptoms of cucurbit powdery mildew on leaves, stems, flower and fruit of eight representatives of Cucurbitaceae: (A) cucumber (Cucumis sativus); (B,C) melon (Cucumis melo); (D,E) summer squash (Cucurbita pepo); (F,G) winter squash (Cucurbita moschata); (H,I) watermelon (Citrullus lanatus); (J) bitter gourd (Momordica charantia); (K) bottle gourd (Lagenaria siceraria); (L) wax gourd (Benincasa hispida). Photo credits: B. Sedláková (A–D, F–I, K–L), N. Dhillon (E,J).
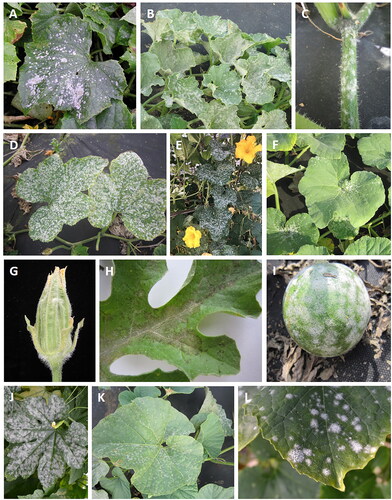
Figure 2. Microscopic characters of cucumber powdery mildews: (A) Golovinomyces orontii (conidia); (B) Podosphaera xanthii (conidia); (C) Leveillula taurica (primary conidia); (D) L. taurica (secondary conidia); (E) G. orontii (conidiophores); (F) P. xanthii (conidiophores); (G) L. taurica (conidiophores); (H) G. orontii (chasmothecium); (I) P. xanthii (chasmothecium); (J) L. taurica (chasmothecium). Photo credits: A. Lebeda (A,B), S. A. Khodaparast (C,D,G,J), L. Trecate (E,F), B. Sedláková (H,I). Bar represents: 10 µm (A–D), 20 µm (E–G), 50 µm (H,I) and 100 µm (J).
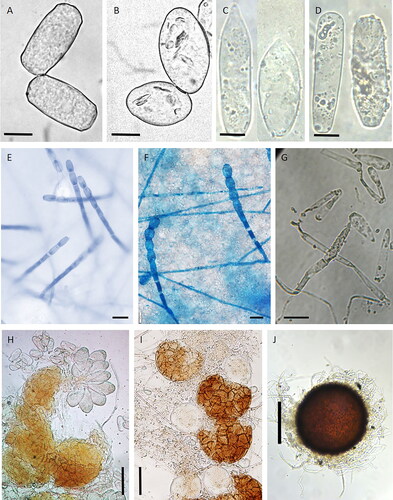
Table 3. Current and historical designations of seven Erysiphales species parasitizing members of the Cucurbitaceae family.
A. Taxonomy and biology of powdery mildews on cucurbits
Seven powdery mildew species (Helotiales, Erysiphaceae) with different hosts and geographic distributions have been recorded to infect hosts of the Cucurbitaceae family (Wu and Kirschner, Citation2017) (). Five powdery mildew species were reported on cucurbits by Braun and Cook (Citation2012). New name combinations were recently released and new species described, reflecting new insights into the taxonomy and nomenclature of these species (). If we want to obtain the most accurate information about the occurrence and harmfulness of selected PM species on cucurbits, it is necessary to take into account all previous taxonomic names under which the given species were described.
Erysiphe actinostemmatis U. Braun (Braun and Cook, Citation2012) is comparatively rare and limited to Actinostemma and Schizopepon species in China and Japan (Braun and Cook, Citation2012). It can grow on both sides of the leaf and forms solitary conidia. DNA sequence data from this species are not yet available. Neoerysiphe sechii Gregorio-Cipriano & D. González (Gregorio-Cipriano et al., Citation2020; Cadena-Iñiguez et al., Citation2022) was confirmed to be a serious pathogen on chayote (Sechium edule) and S. mexicanum in Mexico (Cadena-Iñiguez et al., Citation2022). An unnamed species of Erysiphe was found on Luffa acutangula in Thailand (Meeboon and Takamatsu, Citation2016), which indicates the presence of taxonomically unresolved species of powdery mildews in Cucurbitaceae.
1. Leveillula
Leveillula taurica (Lév.) G. Arnaud ( and ) is limited to dry and warm environments, occurring mainly in the Mediterranean basin as well as in other parts of Africa extending to South Africa, and has been observed in the Americas, Australia, Asia, and Oceania (Braun and Cook, Citation2012). Braun and Cook (Citation2012) stated that L. taurica is one of the most heterogeneous, insufficiently known and taxonomically difficult powdery mildew species. While L. taurica can infect many different plants, it is actually very host specific. Different races of L. taurica can only infect certain crops, and even specific cultivars within the same crop. It is considered a species complex, composed of plurivorous and specialized species. Leveillula taurica s. lat. formed a single complex in the phylogenetic analyses of Khodaparast et al. (Citation2001) based on ITS data. This complex is undoubtedly not uniform biologically, genetically or morphologically (Lebeda et al., Citation2019).
In a more recent analysis Khodaparast et al. (Citation2012) confirmed that L. taurica is unique in the genus, as it exhibits an intraspecific gene sequence diversity considerably higher than in other species. In several cases L. taurica sensu lato (s. lat.) on a certain host plant species has a sequence different from L. taurica on other host plants. DNA data indicated different lineages among L. taurica specimens hardly distinguishable by morphology. The authors consider host specificity for biotrophic fungi such as PM a good criterion along with rDNA sequences to discriminate cryptic taxa. Khodaparast et al. (Citation2016) collected samples of powdery mildew from Iran which showed a higher diversity in the ITS rDNA region. The Leveillula on Cucurbita was found to be morphologically different from L. taurica. rDNA ITS sequences retrieved from Cucurbita powdery mildew surprisingly showed 100% identity to those of L. thevenotiae. The whole genus Leveillula in general and L. taurica s. lat. in particular are in urgent need of phylogenetic-taxonomic multilocus analyses in order to better resolve Leveillula species.
The economically most important and world-wide distributed powdery mildew species infecting hosts of cucurbits are two ectoparasitic species: Golovinomyces orontii (Castagne) Heluta, and Podosphaera xanthii (Castagne) U. Braun & Shishkoff. The taxonomic identifications of these two pathogens have changed many times (e.g., Lebeda, Citation1983; Křístková et al., Citation2009). Braun and Cook (Citation2012) recognized a third ectoparasitic species, G. cucurbitacearum (R.Y. Zheng & G. Q. Chen) Vakal. & Kliron. These powdery mildew species can simultaneously co-infect their hosts, and, unfortunately, cannot be distinguished by their symptoms. Two of them (Px and Go) can, however, be easily distingushed by light microscopy: fibrosin bodies are present in P. xanthii only; curved conidiophore bases occur only in G. orontii. Differentiation of G. orontii and G. cucurbitacearum on morphological characters is difficult and only possible by sequence analyses. But G. orontii emend. (s. str.) conidiophore foot-cells distinctly curved at the base are evident, whereas in G. cucurbitacearum the foot-cells are mostly straight and only sometimes curved-sinuous, often throughout, but not strictly confined to the base (Braun et al., Citation2019). Based on exacting analyses, it was found that CPM caused by Go was prevalent in Central Europe, whereas Px was prevalent in other regions, e.g., North America (Lebeda and Sedláková, Citation2010) and South America (Rabelo et al., Citation2017). For several countries (e.g., Taiwan), Px appears to be the only known powdery mildew species on cucurbits (Pirondi et al., Citation2015a).
2. Podosphaera and Sphaerotheca
The genus Podosphaera consists of two morphological sections, Podosphaera and Sphaerotheca (Braun and Cook, Citation2012). Section Podosphaera (previously genus Podosphaera) parasitizes mainly woody plants (which is in close connection with dichotomously branched ascoma appendages), section Sphaerotheca (previously genus Sphaerotheca; mycelioid appendages) is further divided into the subsections Sphaerotheca and Magnicellulatae, each of which form a separate monophyletic clade derived from different ancestors (Takamatsu et al., Citation2000). More than 50% of the hosts of subsection Sphaerotheca are woody or herbaceous plants belonging to the Rosaceae, on the other hand, all hosts of subsection Magnicellulatae are herbaceous plants scattered among 40 plant families, including Cucurbitaceae. The latter subsection is morphologically supported by unique conidial germ tubes called Fuliginea-type and large outer peridium cells of the chasmothecia. The phylogeny of subsection Magnicellulatae has been discussed by Ito and Takamatsu (Citation2010).
The infection on Cucurbitaceae is caused by Podosphaera xanthii (Shishkoff, Citation2000), formerly Sphaerotheca fuliginea sensu lato, or Sphaerotheca fusca. Braun (Citation1987) widened the concept of the previous species S. fusca enormously and reduced S. erigerontis-canadensis, S. xanthii, but also S. cucurbitae, S. elsholtziae, S. melampyrin, etc., to synonymy with S. fusca. Later Braun et al. (Citation2001) re-introduced this species as P. xanthii for collections on hosts of various families characterized by having large ascomata and asci with large terminal oculi (i.e., this trait supported the separation of P. xanthii from the P. fusca group) ( and ). The host range of P. fusca in his concept include only Doronicum (Asteraceae, Senecioneae) (Braun and Cook, Citation2012). Many authors consider this division controversial and have, however, continued to consider P. xanthii synonymous with P. fusca (not only because ascomata rarely occur, but because phylogenetic approaches for recognizing fungal species should not be based on a single gene phylogeny (e.g., ITS), but on the concordance of multiple gene genealogies (e.g., Pérez-García et al., Citation2009).
Podosphaera xanthii as described by Braun and Cook (Citation2012) is a complex, heterogeneous species composed of plurivorous as well as specialized races, and has an exceptionally wide host range, infecting species from various plant families, mainly Asteraceae, Cucurbitaceae (with very high disease incidence and severity), Lamiaceae, Scrophulariaceae, Solanaceae and Verbenaceae; and its distribution is almost circumglobal (Braun and Cook, Citation2012; Hirata et al., Citation2000; Křístková et al., Citation2009; Wu and Kirschner, Citation2017). The whole complex is morphologically rather uniform and barely separable into smaller morphological units. In various examinations it could be demonstrated that plurivorous races are involved and all races on cucurbits are able to infect unrelated hosts (Braun and Cook, Citation2012). Cross inoculation tests demonstrated that Podosphaera on cucumber (Cucurbitaceae) and cosmos (Asteraceae) are co-infectious, however, it was not confirmed in all cases and moreover Hirata and Takamatsu (Citation2001) found clear genetic differences between collections on cosmos and cucumber. Based on a wide range of hosts, worldwide distribution, and obvious genetic differentiations, it is evident that Px can only be considered as a species complex. Moreover, morphology is not helpful for further differentiations within this complex, and molecular data and results of inoculation tests are insufficient for a further splitting of this complex, so it is currently preferable to maintain the name P. xanthii s. lat. (Braun and Cook, Citation2012; Ito and Takamatsu, Citation2010). More recently Yeh et al. (Citation2021) was convinced that the present Px will be subdivided into several narrowly defined species in the future, perhaps with narrow host ranges. Any revision of this complex requires, however, a phylogenetic-taxonomic multilocus approach, since ITS/28S analyses are insufficient to split this complex.
3. Golovinomyces
Genus Golovinomyces on cucurbits is taxonomically more complicated. In the first monograph of Erysiphaceae (powdery mildews) published by Salmon (Citation1900), almost all species of the genus Golovinomyces (U. Braun) Heluta in its current circumscription were assigned to a single species Erysiphe cichoracearum DC. Based on morphological differences, Blumer (Citation1933) made further attempts to split E. cichoracearum into several species. Hammarlund (Citation1945) published results of comprehensive inoculation experiments and demonstrated the existence of a plurivorous powdery mildew species within E. cichoracearum s. lat. and introduced the invalid name Erysiphe polyphaga for this fungus. Later, Braun (Citation1987) changed it to E. orontii as valid name for this fungus; but shortly thereafter the current combination Golovinomyces orontii was introduced by Heluta (Citation1988) ( and ).
That this species is plurivorous was supported firstly by the molecular sequence analysis done by Matsuda and Takamatsu (Citation2003). In their molecular examinations of the whole Golovinomyces complex, races of G. orontii clustered within the complex of Golovinomyces on Cichorioideae. G. orontii has previously often been confused with G. cichoracearum. Based on molecular sequence analyses and a morphological reassessment, G. cichoracearum s. str. is now confined to powdery mildews occurring on Scorzonera and Tragopogon spp., Asteraceae subtribe Scorzonerinae (Bradshaw et al., Citation2022). The phylogenetic analyses of the genus Golovinomyces based on a much broader sampling suggested a splitting of the G. orontii s. lat. complex into three distinct clusters (groups) based on nucleotide sequences of the ITS and 28S rDNA regions (Takamatsu et al., Citation2013).
Previously, Golovinomyces on hosts of the family Cucurbitaceae was usually assigned to G. orontii, but Braun and Cook (Citation2012) recognized a second species, viz., G. cucurbitacearum (R. Y. Zheng & G. Q. Chen) Vakal. & Kliron. Zheng and Chen (Citation1981) introduced E. cucurbitacearum as a new powdery mildew species on the basis of type material found on Cucumis sativus L. in Xinjiang Uygur AR, China (collected in 1954). Vakalounakis and Klironomou (Citation2001) reallocated E. cucurbitacearum to Golovinomyces based on the new taxonomic generic concept of the Erysiphe (s. lat.) complex published by Braun (Citation1999). They recognized this powdery mildew as a separate species morphologically distinguishable from G. orontii. Braun and Cook (Citation2012) accepted the taxonomy proposed in the latter publication and recognized two Golovinomyces species on hosts of the Cucurbitaceae, viz., G. orontii and G. cucurbitacearum. The differentiation between G. cucurbitacearum and G. orontii is difficult, but curved conidiophore bases are not uncommon in G. orontii and the conidia are larger and the length/width ratio higher, usually around 2. Braun and Cook (Citation2012) considered G. cucurbitacearum as a species with cosmopolitan distribution involving numerous host genera from the Cucurbitaceae family, whereas G. orontii was circumscribed as a plurivorous species on hosts belonging to diverse other plant families, including Asteraceae, Brassicaceae, Papaveraceae, Rubiaceae and Solanaceae.
Braun et al. (Citation2019) recently published a phylogenetic analysis of G. orontii s. lat., based on a broader sampling and rDNA ITS and 28S rDNA data, and confirmed the separation of this complex into the three previously detected clusters and an additional one constituted by sequences retrieved from G. orontii s. lat. on Vinca spp. As a result, there are four distinct species, viz., G. bolayi S. Takam., Lebeda & M. Götz, G. orontii s. str., G. tabaci (Sawada) H.D. Shin, S. Takam. & L. Kiss and G. vincae U. Braun & S. Takam. Owing to its morphological characteristics, G. cucurbitacearum was tentatively reduced to synonym with G. tabaci. Epitypification with ex-epitype sequences was realized by Qiu et al. (Citation2020), since its holotype material (HMAS 40016, collected in 1954) turned out to be too old for molecular examinations. The molecular analyses of ITS and 28S rDNA data confirmed that the powdery mildew found on C. sativus in China pertains to G. tabaci, supporting the assumed taxonomic status of E. cucurbitacearum as synonym of G. tabaci (Qiu et al., Citation2020).
Braun et al. (Citation2019) revealed that all three species of the Golovinomyces orontii s. lat, viz., G. bolayi, G. orontii s. str., and G. tabaci may occur on cucurbitaceous hosts. They also stated that true identities of Golovinomyces collections on cucurbits cited in literature (as Erysiphe cichoracearum, E. polyphaga or E. orontii) are unclear as they cannot be properly interpreted in the current sense, i.e., without molecular analyses, they cannot be assigned to any of the three species of G. orontii s. lat. Golovinomyces collections on numerous hosts of a wide range of plant genera belonging to diverse families have previously been assigned to G. orontii [≡ E. orontii] (Braun and Cook, Citation2012). However, these previous identifications are in general unreliable in the light of the revised species concept of G. orontii s. lat. based on phylogenetic data. In the future, sequence analyses are essential for reliable allocations of collections within this complex to particular species. Qiu et al. (Citation2020) stated that Golovinomyces collections on cucurbitaceous hosts should be treated and identified with utmost care, whenever possible by sequence analyses. Morphological differences between these species exist, but they are only gradual and not easily discernible.
We can only hope that the taxonomy of powdery mildew will stabilize and finally there will be agreement in the nomenclature used between taxonomists, molecular biologists and phytopathologists. We hope that these clarifications will also help to improve clear and exact descriptions of the pathogens concerned, i.e., to avoid unspecific designations, such as “powdery mildew” (without exact taxonomic species specifications), which has not been uncommon until now in many genetic and genomic papers related to different cucurbits and their resistance to PM (e.g., Choi et al., Citation2020; Xu et al., Citation2016a; Citation2017; Wang et al., Citation2018; for details see part 4 of this paper). Without this crucial information, we cannot well understand the involved host-pathogen interactions.
B. Spatiotemporal variation and mixed infections of cucurbits by CPM species
Lebeda et al. (Citation2021a) recently stressed spatiotemporal changes in the geographic distributions of Go and Px during the last three decades (Křístková et al., Citation2009; Lebeda et al., Citation2007b; Citation2009; Pirondi et al., Citation2015a). Simultaneous infections of the two pathogens in a single crop and host plant (Křístková et al., Citation2009; Lebeda et al., Citation2009) are often overlooked (Lebeda et al., Citation2018a). CPM isolates from mixed infections frequently express various reaction patterns, i.e., different races, on differential hosts under in vitro tests, and complicate practical cucurbit growing and disease management (Lebeda et al., Citation2021a).
C. Pathogenic variation of CPM species
1. General comments
The pathogenic specialization and variation (existence of pathotypes and races) of CPM has been known for nearly 100 years. There is often a very clear expression of compatibility or incompatibility in host plant–powdery mildew interactions that allows for the classification of pathotypes and races based on compatible and incompatible reaction patterns on differential hosts species or genotypes (Lebeda et al., Citation2008; Citation2011).
The first commercial loss to Px was in 1925 (Jagger, Citation1926), existence of two races was recognized in 1938, shortly after the release of “PMR 45” cantaloupe (Jagger et al., Citation1938b). During the following decades there were described many races of Px (McCreight, Citation2006; Lebeda et al., Citation2008), mostly on melon, but on other cucurbit crops as well (Lebeda et al., Citation2011; Citation2016a; Keinath et al., Citation2017; Dhillon et al., Citation2018). Enormous spatio-temporal virulence variation of Px was recognized on the population level (Lebeda et al., Citation2018a; Citation2021a). Much later this phenomenon was also recognized in Go. The first detailed evidence of Go races on cucumbers and other Cucurbita spp. were reported in the 1990s and 2000s (Pitrat et al., Citation1998; Bardin et al., Citation1999; Křístková and Lebeda, Citation1999a; Lebeda and Sedláková, Citation2004, 2006; Lebeda et al., Citation2004; Citation2007b). Very broad Go virulence variation was later shown on the population level (Lebeda et al., Citation2018a; Citation2021a). Significant variation in virulence, expressed by numerous pathotypes and/or races and their spatiotemporal fluctuations, has been described for both CPM species (Bardin et al., Citation1999; Cohen et al., Citation2004; Hong et al., Citation2018; Lebeda et al., Citation2007b; Citation2008; Citation2009; Lebeda and Sedláková, Citation2006; McCreight, Citation2006). Considerable variation in the virulence of Px populations is also reflected in a high degree of variation in their DNA profiles (Xiang et al., Citation2020). De Miccolis Angelini et al. (Citation2019) stressed that Px has great potential to evolve new, better-adapted genotypes that can overcome currently used resistance genes and modern fungicides. Virulence variation in L. taurica has not been observed on cucurbits (Lebeda et al., Citation2011; Citation2021a).
Description and denomination of CPM virulence variation, including clear specification of the differences between pathotypes and races, was not well elaborated or unified, and it was rather chaotic at the international level, prior to the 2010s (Lebeda et al., Citation2011).
2. Pathotypes
Pathotype is nontaxonomic category describing pathogenicity on the level of host range of specific PM species and isolate (Holliday, Citation1998). CPM pathotypes are based on intergeneric and interspecific differences in host–CPM interactions (Lebeda et al., Citation2008; Citation2011). Two differential sets for the determination of pathotypes have been proposed (Bertrand Citation1991; del Pino et al. Citation2002; Lebeda et al. Citation2008; Citation2011). Bertrand (Citation1991) assembled the most frequently used differential set for pathotype determination; it included one genotype from each of four species (Cucumis sativus, C. melo, Cucurbita pepo, Citrullus lanatus) representing three agriculturally important cucurbit genera plus two genotypes from a fifth species, melon, Cucumis melo L. (Lebeda et al., Citation2011) that was later expanded on Cucurbita maxima (Křístková and Lebeda, Citation1999a). Pathogenicity is described by a specific code (Lebeda et al., Citation2011). Pathotype could be considered as a basic pathogenicity category providing information on host range on agriculturally important cucurbits (Lebeda et al., Citation2011), however their usage in genetic studies and breeding has been limited.
3. Races
Race (physiological race) is the pathogenicity (virulence) category of plant pathogenic fungi, including CPM, characterized by virulence specialization and variation to different genotypes, cultivars and lines of one host species (Holliday, Citation1998), but in some cases has involved taxonomically related wild species, e.g., Lactuca spp.–Bremia lactucae interactions (Spring et al., Citation2018). CPM races are characterized by the respective interactions of different isolates of Go and Px with different cultivars of a given host species (Bardin et al., Citation1999; Bertrand, Citation1991; Bertrand et al., Citation1992; Lebeda and Sedlakova, Citation2010; Pitrat et al., Citation1998). Races of Go and Px have, to date, been differentiated only on melon (Lebeda et al., Citation2008; Citation2011; Citation2016a). McCreight (Citation2006) and Lebeda et al. (Citation2008; Citation2011) summarized the different genotypes and systems used for CPM race identification and denomination. An overview of the various sets of CPM race differentials was shown in chronological order, starting from the appearance of the second race of Px (in 1938) (Lebeda et al., Citation2011). The most frequently used sets of melon differentials included 11 genotypes of C. melo, that can differentiate CPM races originating from melon (McCreight, Citation2006), however also from other cucurbits, such as cucumber, Cucurbita spp., and watermelon (Lebeda and Sedláková, Citation2006; Citation2010; Lebeda et al. Citation2004; Citation2007a; Citation2008). This situation was critically reviewed from the viewpoint of the current state, gaps, and perspectives in our understanding of pathogenicity variation in two CPM pathogens with the main focus on race variation and identification (Lebeda et al., Citation2008; Citation2011) with the aim to develop a unified set of melon differential as well as a unified system of CPM race identification and denomination (Lebeda et al., Citation2011; Citation2016a; Citation2016b; Citation2021a; Citation2021b).
The process of developing a set of differentials was initiated at the end of the 1970s by carrying out some basic studies related to CPM (Lebeda, Citation1983; Citation1984b; Citation1986; Lebeda et al., Citation2008; Lebeda and Sedláková, Citation2010). Discussions at the IXth Eucarpia Meeting on Cucurbitaceae in Avignon, France in 2008, and the collaboration between the Czech Republic (A. Lebeda et al.) and the U.S. (J. D. McCreight) led to the International Cucurbit Powdery Mildew Initiative (ICPMI) (Lebeda et al., Citation2011; Citation2016a; Citation2016b; Citation2018a; Citation2018b). Discussion at Cucurbitaceae 2016, XIth Eucarpia Meeting on Genetics and Breeding of Cucurbitaceae (Warsaw, Poland) by the authors and representatives of nine international melon breeding companies led to the unanimous agreement to implement the proposed system for uniform virulence variation and race determination, and denomination of MCPM, as well as CPM on other cucurbits, was summarized in recent publications (Lebeda et al., Citation2016a; Citation2016b; Citation2018a; Citation2018b; Citation2021a; Citation2021b) and .
Table 4. GRINTable Footnotea accession numbers, septet numbers, sources and origins of the ICPMIb melon (Cucumis melo) cucurbit powdery mildew race differentials (Lebeda et al., Citation2016a; Citation2016b; Citation2018a; Citation2018b; Citation2021a; Citation2021b).
The proposed triple septet of 21 CPM differentials (Lebeda et al., Citation2016a; Citation2016b; Citation2018a; Citation2018b; Citation2021b) was the next logical step in facilitating communication within and among researchers, commercial breeders, plant pathologists, extension specialists and crop consultant communities (). During this process, a fourth septet was established with the addition of melon accession SVI105, designed as 4.1 (). Additional melon accessions deemed crucial for characterization of CPM virulence variation in the future may be added to the fourth septet (Lebeda et al., Citation2021b).
Establishment of a uniform system for CPM virulence characterization, and race determination and denomination is based upon four components: (1) a standard set of race differentials, (2) a uniform screening methodology, (3) a uniform code for the host-CPM interactions/scores (Lebeda et al., Citation2016a; Citation2016b; Citation2018a; Citation2018b), and (4) a system that is open to the addition of new differential accessions. The proposed set of 22 melon differentials was acceptable to commercial melon breeders and pathologists attending Eucarpia Cucurbitaceae 2016 (Warsaw, Poland) (Lebeda et al., Citation2016a). Rijk Zwaan Breeding B.V. (De Lier, The Netherlands) took on the task of increasing the entire differential set () for distribution to the international melon CPM community. The seeds have recently been deposited in the United States Department of Agriculture (USDA), National Plant Germplasm System (NPGS), North Central Regional Plant Introduction Station (NCRPIS), Ames, Iowa, U.S.A., which will be responsible for distribution of the seed material to requestors (Lebeda et al., Citation2021b).
Broad international application of the above described system of CPM virulence variation description and denomination can substantially contribute to various studies focused on interactions between cucurbits and CPM, and their understanding and clear interpretation. Until recently this aspect was seriously missing and sometimes the description and interpretation of obtained data were not fully clear, understandable and/or sometimes confusing (see many remarks in different parts of this review).
4. Pathogenic variation on population level
Virulence variation on the level of individual CPM isolates has been known for a rather long time (see details above). However, when investigating pathogens, resistance-breeding programs should take a population based, evolutionary approach (McDonald and Linde, Citation2002a; Citation2002b). Such an approach would incorporate detailed knowledge of the spatiotemporal dynamics of CPM populations, more specifically: (1) pathogen species structure, (2) the frequencies and dynamics of CPM virulence factors, and (3) the spectrum of well-defined v-phenotypes (races) as determined by in vitro testing (Lebeda et al., Citation2016a; Citation2016b; Citation2021a). The most important v-factors and v-phenotypes (those persisting in the pathogen population with strong virulence patterns) should be treated with the highest priority. This approach was applied to analysis of CPM populations for virulence variation in 115 isolates of Go and Px, collected in the Czech Republic in 2010–2012 (Lebeda et al., Citation2018a). Our current detailed study was based on data from long-term (2001–2012) observations of CPM populations in the Czech Republic (which revealed CPM species fluctuations and the spatiotemporal dynamics of virulence within and among pathogen populations) and their evaluation on a fixed set of differential C. melo accessions (Lebeda et al., Citation2021a). Diversity analyses of the resulting population data showed enormous changes in virulence in time and space. This is the first study based on such a high number of analyzed CPM isolates (in total 619: 417 Go, 202 Px), and such an approach provides a framework to predict the most efficacious resistance sources for breeding programs and is theoretically more effective than reliance on single CPM isolates or races (Lebeda et al., Citation2021a).
However, studies of genetic structure of populations of Go and Px reported by different authors did not completely explain the relationship between DNA profile of fungal isolates and their other parameters, e.g., geographic origin and virulence phenotype on host plants (Lebeda et al., Citation2018a). RAPD analysis of P. xanthii from southern Italy revealed high genetic variation among isolates, but no marker was associated with geographic origin, host plant, or physiological race (Miazzi et al., Citation2011). Phylogenetic analysis of Italian and Czech populations of Go inferred by multilocus sequence typing revealed two main distinct and genetically different phylogenetic groups related to geographically distant populations (Italian, Czech), but no genetic differentiation between Italian and Czech populations of Go was detected (Pirondi et al., Citation2016). We are still missing more detailed population studies, based on classical virulence variation detection (Lebeda et al., Citation2016a) combined with molecular approaches (Pirondi et al., Citation2015b) that may contribute to a better understanding of the evolutionary forces responsible for spatiotemporal shifts in virulence and genetic variation within and among populations of both CPM species on local, regional, country and continental level (Lebeda et al., Citation2018a; Citation2021a).
IV. Host—powdery mildew interactions, resistance mechanisms and breeding
A. Brief history of CPM resistance breeding in cucurbits
Cucurbits display enormous phenotypic and genetic variation. Some genera (e.g., Cucumis, Cucurbita, Citrullus) are widely cultivated and have extraordinary human importance (Rubatzky and Yamaguchi, Citation1997; Lebeda et al., Citation2007a). Cucurbits belong to one of the oldest domesticated plants, known as one of the first vegetable crops 8,000—12,000 years ago, both in the Old and New Worlds (Hancock, Citation2004). Intense scientific breeding of cucurbits started ca. one hundred years ago, in the first decades of 20th century (the 1920s and 1930s) and was focused on improving the production and quality of fruits, modifying plant architecture and sex expression, and resistance to a wide range of pathogens and diseases (Robinson and Decker-Walters, Citation1997), including CPM (Sitterly, Citation1978; Jahn et al., Citation2002).
B. Cucumber (Cucumis sativus)
1. Introduction
Cucumber breeding has been primarily based on the utilization of suitable genotypes within the species, i.e., intraspecific variability, due to its genetic isolation and lack of cross compatability with wild relatives (Esquinas-Alcazar and Gulick, Citation1983), the exception being Cucumis hystrix, which gave rise to the synthetic species Cucumis hytivus (Chen et al., Citation2003; Zhuang et al., Citation2006). Interspecific (intergeneric) hybridization with melon (Cucumis melo L.) remains unsuccessful (Naegele and Wehner, Citation2017; Grumet et al., Citation2021).
Research on cucumber CPM was undertaken beginning in the 1920s, and cultivars or genotypes with resistance to CPM were reported mainly on the basis of field observations of natural infection (Sitterly, Citation1978; Jahn et al., Citation2002). Crossing experiments (resistant × susceptible parents) and detailed examination of the genetic background of resistance led to the discrimination of resistance genes beginning in the 1970s (). Another foliar pathogen, cucurbit downy mildew (CDM), Pseudoperonospora cubensis Berk. Curt. Rostov., has dominated host plant resistance research and breeding world-wide since 1984. Consequently CPM was rarely observed on this crop and lost its economic importance in many regions of the world (Lebeda and Cohen, Citation2011; Spring et al., Citation2018). Advanced techniques, e.g., protein markers (enzymes, isoenzymes) and molecular mapping were applied to the study of economically more important pathogens. The interest of molecular biologists to study the interactions between cucurbits and CPM is notable since the turn of the century (Grumet et al., Citation2017).
Table 5. Cucumber (Cucumis sativus) genes (synonyms) controlling responses to Podosphaera xanthii and Golovinomyces orontii, and their respective sources per Wehner (Citation2005-2006) and Weng and Wehner (Citation2016).
Host-pathogen interaction research is complex and typically falls into one of two approaches. One line is focused on the pathogen (taxonomy, identification, ecological requirements, physiological specialization to host, virulence, geographic distribution, reaction to fungicides, interaction with host plants), while the second line focused on host species (reaction to the pathogen on various levels, from assessment of visual symptoms to mapping resistance genes and studying principles of resistance on the level of metabolomics).
The most important results related to cucumber breeding for resistance to CPM, achieved in the 20th century, are discovery of resistance sources, determination of the inheritance of resistance, and linkage and pleiotropy of CPM resistance as critically reviewed by Jahn et al. (Citation2002). However, they concluded that very recent observations suggest that the interaction between CPM and its cucumber hosts may be a dynamic process subject to shifts similar to those discussed for melon. Jahn et al. (Citation2002) describe the situation in the last months of 2000, when most CPM-resistant cucumbers in the Cornell greenhouse showed more mildew than previously observed. They meant that overlapping generations with some mildew susceptible plants had permitted the pathogen to evolve. However, the resistant lines did not become fully susceptible. It appeared that the pathogen was able to overcome one of the three genes for resistance. Comparable results have not been observed in the field (Jahn et al., Citation2002).
2. Inoculation experiments, screening of responses to (specific races) Gc and Px, detection of resistant genotypes, sources of resistance
Much research has been dedicated to evaluation of responses to CPM under conditions of natural infection, selection of suitable genotypes, hybridization of contrasting (resistant and susceptible to CPM) cucumber genotypes, development of breeding lines, genetic markers and gene mapping. Unfortunately, in many of these papers the basic information on taxonomic status of the pathogen is missing. This is evident in many papers (e.g., Wang et al., Citation2023a,Citationb) dealing with application of modern molecular techniques, genetic mapping and sequencing published in the 21st century. Authors of such studies usually omitted the taxonomic identity of the causal agent of CPM. In the light of knowledge of the high variability in virulence between Px and Go, and within each species, and of spatio-temporal shifts of virulence (Lebeda et al., Citation2021a) the value of such reports falls short of expectations. Exact CPM species determination and specification in the study of He et al. (Citation2013) is almost unique, including specification of pathotype or race (Lebeda et al., Citation2011; Citation2016a).
Experiments aimed at evaluation of responses of cucumber genotypes are most frequently based on the visual assessment of infection development on host plants (leaves), under conditions of natural infection (field or greenhouse), or after inoculation of plants, grown in greenhouse or growth chamber, by dusting CPM conidia or by spraying their water suspension (Lebeda and Sedláková, Citation2010), as well as under different temperatures (Trecate et al., Citation2019).
Various methods of inoculation and resistance screening of cucumbers and other cucurbits against Go and Px on the level of plants, leaves and leaf disks were reported in recent decades (for summary see Morishita et al., Citation2002; Citation2003; Lebeda and Sedláková, Citation2010), but when CPM species information was included they were mostly focused on resistance to Px.
Among 177 cucumber accessions screened for resistance to Px in a greenhouse after spraying with CPM conidia, 108 accessions were partially resistant, and novel genes for resistance were defined (Zijlstra and Groot, Citation1992).
Corbaz and Taillens (Citation1994) reported that a set of cucumber cultivars previously resistant to Px in the United States were susceptible to Px and Go in Switzerland. They explained the contrasting results (resistant vs. susceptible) by the presence of more virulent strains of Px and clearly pointed out that the responses of cucumber cultivars differ with each PM species. Unfortunately, this conclusion was not seriously taken in consideration by many other researchers.
The development of CPM (Px) was assessed on a set of cucumber cultivars in a greenhouse after artificial inoculation (Aalbersberg and Stolk, Citation1995). Higher levels of resistance were observed in commercial cultivars, which suffered from chlorosis under low light intensity. The cv. Aramon was highly resistant. Stem resistance and only partial leaf resistance was observed on cv. Flamingo. This phenomenon was later studied on the hybrid cv. Profito, resistant to CPM and sensitive to leaf chlorosis (Zijlstra et al., Citation1995).
Thirteen cucumber accessions were assessed for their responses to a mixed, natural infection of Go and Px in a greenhouse (Lebeda and Křístková, Citation1997; Křístková and Lebeda, 1999b), where resistance was expressed as percentage of maximum infection degree (%maxID and/or sum%maxID, respectively) and as "Area below curve (ABC, for leaves/L/, stems/S/and petioles/P/)" of the disease infection progress (ABC-L + S + P). The cvs. Corona F1 (ABC (L + S + P) = 2811) and Flamingo F1 (ABC (L + S + P) = 2020) were the most susceptible. Accessions PI 435946, PI 426170 and PI 390258 were not infected on the stems or petioles during the test; their ABC values were much lower than the other accessions tested (PI 435946 = 544, PI 426170 = 277, PI 390258 = 250). There were significant differences among the 13 accessions for field resistance to CPM (Lebeda and Křístková, Citation1997; Křístková and Lebeda, 1999b). The three resistant accessions exhibited similar responses in the later experiments in the United States (Block and Reitsma, Citation2005).
Block and Reitsma (Citation2005) evaluated 977 cucumber accessions from the U.S. National Plant Germplasm collection in a greenhouse for their responses to inoculation with field isolates of Px. A substantial portion of the accessions were susceptible. Ninety-four accessions contained at least one resistant plant. Seventeen of the 20 most-resistant accessions came from Asian sources, including China (PIs 418962, 418964, 432860, 432870), India (PIs 197085, 197088, 605930), Japan (PIs 279465, 288238, 390258, 390266), Pakistan (PI 330628), the Philippines (PIs 426169, 426170), and Taiwan (PIs 321006, 321009, 321011). PI 390258, PI 426170 and PI 435946) previously evaluated for resistance to a natural mixture of Go and Px in a greenhouse (Lebeda and Křístková, Citation1997), were not infected on the stems and petioles during the whole growing period. PI 390258 and PI 426170 were among the 20 most resistant accessions (Block and Reitsma, Citation2005), as in the previous study.
A set of 295 germplasm accessions of cucumber (stored at the National Institute of Vegetables and Tea Science, Japan) was screened by assessing CPM (Px) infection development on inoculated cotyledons and first true leaves (excised from inoculated plants 7 and 14 days after germination) and placed in petri dishes (Morishita et al., Citation2003). Many of the resistant accessions were Chinese cultivars and lines. Accession PI 197088-5, a progeny of PI 197088 from India, exhibited the highest level of resistance to Px. The authors concluded that resistance of PI 197088-5 is temperature independent and very likely due to two gene pairs, a recessive gene and an incompletely dominant gene with the genotype designated as aaBB (Morishita et al., Citation2003).
A set of 140 local cucumber genotypes collected in northern India and exotic germplasm was screened for CPM (Px) resistance to identify tolerant genotypes under natural (field) and artificial conditions and then validated with eight known SSR markers linked to CPM resistance (Sharma et al., Citation2021). Genotype GS140 was resistant. Accession PI 197088 (GS180), which has been exploited extensively for cultivar development and reported to be resistant to PM (Morishita et al., Citation2003; Block and Reitsma, Citation2005) was highly susceptible in this study, which suggested a wide diversity of pathogen virulence in India that is also known in other countries and notable among countries (McCreight, Citation2006; Lebeda et al. Citation2018a; Citation2021a; Citation2021b).
During recent decades plant pathologists and plant breeders have been interested in exploiting wild relatives of cultivated crops (Maxted et al., Citation2006; Lebeda et al., Citation2014; Dempewolf et al., Citation2017; Lebeda and Burdon, Citation2023) in resistance research and breeding. This concept and strategy is being used for cucurbits (Lebeda et al., Citation2007a; Grumet et al., Citation2021). One of the first attempts to search for resistance in wild Cucumis was the screening of a set of 57 accessions of 20 wild Cucumis species and varieties, mostly originating from the African gene center for resistance to Go and Px in response to artificial inoculation (Lebeda, Citation1984b). Seedlings (cotyledon stage) were substantially more susceptible than adult plants. Inter- and intra-specific variation was detected in the interactions of wild Cucumis spp. and both CPM pathogens. Eighty percent of the accessions were susceptible as adult plants to Go, and 40% to Px. Adult plants of Cucumis ficifolius (IVT 1801, PI 280231), C. anguria (PI 147065), C. anguria var. anguria (CUC 9/1974), C. dinteri (PI 374209), and C. sagittatus (PI 282441) were resistant to both pathogens (Lebeda, Citation1984b).
Numerous cucumber cultivars and accessions and their related wild Cucumis spp. are resistant to Go and Px. These sources have mostly race-specific resistance and their efficacy depends on specific isolates (races) and local pathogen population virulence structure, the knowledge of which is crucial for efficient resistance breeding and disease management (Lebeda et al., Citation2021a).
3. Detection of resistance genes, inheritance studies, applications in breeding
Pierce and Wehner (Citation1990) provided the first comprehensive review of cucumber genes and linkage groups. Gene lists for all economically important cucurbit crops are updated and published by The Cucurbit Genetics Cooperative (Wehner and Ng, Citation2005) with the most recent gene list for cucumber () authored by Weng and Wehner (Citation2016).
Genes pm-1, pm-2, pm-3 and pm-h controlling resistance to Px were included in the 2005 cucumber gene list (Wehner, Citation2005). In the most recent cucumber gene list (Weng and Wehner, Citation2016), only gene pm-h was retained, and genes Pm1.1 and pm5.1 were added. The authors of this list consider that CPM is caused mainly by Px (formerly P. fusca or S. fuliginea), and that resistance to CPM in cucumber is in general quantitative. Previous results (some summarized above) show, however, that CPM resistance in cucumber must address both CPM species (Px and Go) as well as quantitative and qualitative types of responses, which means race-specific interactions, common to the broad array of CPM pathogen species on their respective crop hosts (Brown, Citation2002).
Crossing experiments of Japanese CPM-resistant cvs. Natuhusinari and Natsufushinary, with susceptible cv. Kurume-otial No. 1, and on the basis of field observations in response to natural CPM infection (without clear information on CPM species) led to the conclusion that CPM resistance is recessive and based on monogenic resistance-factors (Fujieda and Akiya, 1962).
Crossing experiments of cv. Natuhusinari with PI 200815 and PI 200818 whereby the degree of infection was assessed led to the conclusion of three recessive resistance factors (r-factors) in response to Px though they were not demonstrated with certainity (Kooistra, Citation1968). Though Go has been the prevailing or most common PM species in northern Europe (Křístková et al., Citation2009), Kooistra (Citation1968) noted Px cleistothecia characteristics were observed in identification of resistance gene pm-3 in PI 200815 and PI 200818. Kooistra (Citation1971) postulated that dull skin color was conditioned by one dominant gene, closely linked with one of the genes for CPM resistance. The results of Kooistra (Citation1971) in relation to the genes pm-1, pm-2, and pm-3 in the cucumber gene lists (last time by Wehner and Ng, Citation2005) are not adequately supported by clearly defined experimental parameters. The recent cucumber gene list (Weng and Wehner, Citation2016) removed, therefore, these genes from the lists but referred to them in the accompanying text for historical context.
Cucumber lines consistently classified as resistant, intermediate or susceptible to CPM incited by Px in Wisconsin (U.S.A.) were used by Shanmugasundaram et al. (Citation1971a) for study of the inheritance of resistance to Px isolated from greenhouse-grown cucumber in Wisconsin and maintained in a greenhouse by aerial inoculation of susceptible plants. It was concluded that recessive gene s determines hypocotyl (intermediate) resistance and is also essential for true leaf resistance. Leaf (complete) resistance is controlled by dominant R gene, which only expresses itself in the presence of gene s. Gene I is an inhibitor gene that prevents the expression of complete resistance conferred by R, but does not affect gene s. Therefore Shanmugasundaram et al. (Citation1971a) concluded that the resistant line 9362, PI 234517 and Natsufushinari have the genotype RR ii ss, whereas the susceptible Chicago Pickling is RR II SS, and that of intermediate resistant PI 212233 and Pixie is rr II ss.
According to Pierce and Wehner (Citation1990) several reports indicated that more than one gene controls resistance to CPM incited by Px, with interactions occurring among loci (Hujieda and Akiya, Citation1962; Kooistra, Citation1968; Shanmugasundaram et al., Citation1971b). The resistance genes pm-1 and pm-2 were firstly reported by Hujieda and Akiya (Citation1962) in a cultivar they developed, Natsufushinari. Kooistra (Citation1968), using this same cultivar, later confirmed their findings and identified one additional gene (pm-3) from USDA accessions PI 200815 and PI 200818. Shimizu et al. (Citation1963) reported three recessive genes that are also responsible for resistance of cvs. Aojihai and Sagamihan. Several genes with specific effects were identified more recently (Shanmugasundaram et al., Citation1971b), but unfortunately, direct comparisons were not made to determine their relationship with pm-1, pm-2, and pm-3. Fanourakis (Citation1984) considered a CPM resistance gene in an extensive linkage study and proposed that it was the same gene used by Shanmugasundaram et al. (Citation1971b), which also determines resistance of the hypocotyl at the seedling stage of growth. Because expression of this resistance response is easily observed and since it is frequently labeled in the literature as “pm,” we believe that this gene should be added to the list as pm-h, with the understanding that this may be the same gene as pm-1, pm-2, or pm-3 (Pierce and Wehner, Citation1990).
De Ruiter et al. (Citation2008) tested plants in the greenhouse under conditions of natural CPM infection (without specification of pathogen species), and they classified CPM resistance, from PI 200815, into true leaf resistance (pm-l) and hypocotyl resistance (pm-h), which were linked loci in cucumber chromosome 5.
QTL studies of the highly resistant PI 197088-1 identified two and three loci for CPM (Px) resistance at 26 °C and 20 °C, respectively (Sakata et al., Citation2006). One major QTL acts at both temperatures, while other QTLs are temperature specific and their combinations play an important role in expressing high level of resistance to CPM (Sakata et al., Citation2006).
In a study of leaf disks on agar medium in Petri dishes inoculated using a sedimentation tower with crosses of resistant cucumber line S06 with susceptible inbred line S94 Liu et al. (Citation2008) detected five QTls (PM1.1, PM1.2, PM2.1, PM2.2, PM5.1) for resistance to Px (without clear proof of species determination) located on linkage groups (LG) 1, 2 and 5, explaining 34–45% of phenotypic variation. QTLs PM1.1, PM2.1, PM5.1 were consistently detected under three test conditions and are proposed as useful for marker assisted selection (MAS) (Liu et al., Citation2008).
4. Genetic (molecular) mapping
Progress in study of the cucumber genome, including genetic mapping, draft genome assembly, sequencing and whole genome features was comprehensively summarized in chapter “The Cucumber Genome” (Weng, Citation2017). In general cucumber has advantages for genome research because of its relatively small genome size, low repetitive DNA and short life cycle. Cucumber was one of the first among major horticultural crops to have a publicly released draft genome (Weng, Citation2017).
Data on current progress in genetic and genomic-aided breeding for stress resistance in cucumber were provided by Das et al. (Citation2022). High-density maps and easily accessible high throughput sequencing technologies enable quick identification of molecular markers or cloning of candidate genes/QTL for several biotic and abiotic stresses (Das et al., Citation2022).
Information and data on genome mapping confirm the narrow genetic background of cucumber compared with melon (Danin-Poleg et al., Citation2001), and revealed nine SSRs common to melon and cucumber maps (Danin-Poleg et al., Citation2000). Linkages among molecular, isozyme, disease-resistance and morphological markers in cucumber are continually being elucidated (e.g., Kennard et al., Citation1994; Walters et al., Citation2001; Simko et al., Citation2021), however we are still missing comparisons of genes for resistance to each CPM species.
Quantitative trait loci associated with Px resistance have been mapped to six cucumber chromosomes (Sakata et al., Citation2006; Pérez-García et al., Citation2009; Fukino et al., Citation2013; Nie et al., Citation2015a; Citation2015b; Xu et al., Citation2016a; Citation2016b).
A cucumber genetic linkage map based on a population of 111 recombinant inbred lines derived from a cross of susceptible with resistant CS-PMR1, which possesses strong and temperature-independent resistance to CPM, validated the four QTLs pm3.1, pm5.1, pm5.2, pm5.3 (Fukino et al., Citation2013).
QTL mapping of resistance to Px (identified by microscopic observation) in cucumber line WI 2757 identified six genomic regions on four chromosomes conditioning responses to this pathogen: pm1.1 and pm1.2 (chromosome 1) conferred leaf resistance; a minor QTL pm3.1 (chromosome 3) and pm4.1 (chromosome 4), and two major QTL, pm5.1 and pm5.2 (chromosome 5) (He et al., Citation2013). The latter two QTL (pm5.1 and pm5.2) are recessively inherited and linked and are the major resistance factors for Px resistance in WI 2757. Dominance gene action seems to be important for host resistance. QTL pm1.1 and pm1.2 had contributed moderately to host resistance, while pm3.1 and pm4.1 promoted susceptibility. The mechanisms of interactions among cotyledon, true leaf and hypocotyl resistance QTLs (pleiotropic, epistatic, or linkage) remain to be determined (He et al., Citation2013).
Mapping of a dominantly inherited, major-effect QTL for CPM resistance in cucumber, identified two tandemly arrayed, cysteine-rich, receptor-like protein kinase genes as possible candidates of this resistance (Xu et al., Citation2016b). This is the first report of dominantly inherited CPM resistance (the Pm1.1) in cucumber, namely in the North China type, indeterminate cucumber inbred line Jin5-508. Dominantly inherited PM resistance major-effect QTL, Pm1.1 that was located in a 41.1 kb region of cucumber chromosome 1 harboring two tandemly arrayed, cysteine-rich recepto-like protein kinase genes (Xu et al., Citation2016b).
Whole-genome resequencing of cucumber resistant lines SSL508-28, Jin5-508, and susceptible D8 was accomplished by a three year evaluation of reactions to CPM after inoculation in greenhouses (Xu et al., Citation2016a). Powdery mildew conidia were collected from naturally-infected D8 plants in a greenhouse. To better describe the resistant phenotype difference of SSL508-28 and D8, fungal growth was cytologically assessed. Two candidate genes, Csa2M435460.1 and Csa5M579560.1, for CPM resistance in cucumber were identified (Xu et al., Citation2016a). The fact that PM was not taxonomically determined on the level of species diminishes the value of these results.
Further genomic studies provided valuable information for the fine mapping of Pm5.1 and breeding of cucumber with enhanced resistance to CPM (Xu et al., Citation2017). However, also in this case the CPM species is not specified in the text and photos documenting microscopic observations of CPM structures on infected leaves do not allow an exact determination of species.
QTL mapping of PI 197088 proved that its resistances to CDM and CPM pathogens are conferred by 11 (three with major effect) and four (one major effect) QTLs, respectively, and three of them are co-localized. QTL pm5.1 for CPM resistance explained 32.4% the phenotypic variance and the minor-effect QTL pm6.1 contributed to disease susceptibility. Three CPM QTL, pm2.1, pm5.1, and pm6.1, were co-localized with CDM QTL dm2.1, dm5.2, and dm6.1, respectively, which was consistent with the observed linkage of CPM and CDM resistances in PI 197088 (Wang et al., Citation2018). Unfortunately, the authors automatically assumed that CPM infection observed in the three years of field trials was caused by P. xanthii, without any proof of species identification.
Badri Anarjan et al. (Citation2021) applied CPM-resistant molecular markers derived from two CPM-resistant candidate genes (CsaMLO8 and CsLRR-RPK2) to 100 Korean inbred lines derived from crosses of 12 CPM-resistant lines with eight susceptible lines in order to evaluate the accuracy of marker-assisted selection. Furthermore, they attempted to understand the origin of CPM resistance in Korean cucumber inbred lines. Plant responses to natural CPM infection, presumably incited by Px, in a greenhouse were evaluated as susceptible/resistant on the basis of visual assessment of leaf area covered by mycelium. Results provided reliable evidence that confirms two CPM-resistant candidate genes and efficiently utilized these genes for the detection of CPM-resistant resources and inbred lines. Furthermore, the CsLRR-RPK2- CAPS marker showed a stronger correlation with CPM-resistant phenotype in comparison with the CsaMLO8-InDel marker. In addition, results revealed that unidentified CPM-resistant sources used in breeding programs in Korea are not related to either CPM-resistant candidate gene (CsaMLO8 and CsLRR-RPK2).
Badri Anarjan et al. (Citation2021) stressed, however, that, in comparison with melon, where numerous races of CPM were identified, race identification of CPM in cucumbers has not been well developed. The authors stated without evidence that Px race 1 has been dominant in Korea though six races have been detected on melon in that country (Kim et al., Citation2016b), and therefore the virulence population structure on cucumber is not known. They hypothesized that each allele of CsLRR-RPK2 and CsaMLO8 may be responsible for different races of CPM in cucumbers, and recommended breeders continuously select CPM-resistant plants followed by self-pollination in order to accumulate CPM-resistant allele pairs (CsaMLO8 and CsLRR- RPK2) in CPM-resistant lines.
In order to understand the genetic diversity and the genetic relationships among the germplasm collections, Lee et al. (Citation2020) applied a genotyping-by-sequencing (GBS) approach to obtain dense genome-wide markers coverage. They used 264 accessions of C. sativus, C. anguria, C. myriocarpus and C. zeyheri. One hundred of the accessions consisted of advanced breeding lines developed for commercial breeding, the remainder were wild accessions that were systematically sampled from the USDA National Plant Germplasm System (NPGS) based on their genetic diversity. This set of accessions was evaluated for resistance to CPM, without specifying the species, in 2018 and 2019. Sixteen accessions showed high susceptibility in 2018 and five accessions (PI 438678, Ames 23537, PI 196477, Ames 23543, and PI 264217) showed strong resistance to CPM infection. Most of the test lines were susceptible to CPM. None of the accessions exhibited strong CPM resistance in 2019. Csa5G471070 and Csa5G453160 were nominated as the candidate genes for the CPM resistance, as significant SNPs were located in chromosome 5. Interestingly, among the WW (Korean semi-white white spine) type breeding lines, BN-019 exhibited relatively strong CPM resistance, sufficient for elite line development (Lee et al., Citation2020).
Liu et al. (Citation2021) evaluated CPM resistance of a cucumber core germplasm (CG) set that consisted of 94 resequenced lines in four field trials in 3 years (2013, 2014, and 2016). Adult plants were assessed for responses to natural CPM infection (species not specified). Using genome-wide association study (GWAS) analysis, 13 loci (pmG1.1, pmG1.2, pmG2.1, pmG2.2, pmG3.1, pmG4.1, pmG4.2, pmG5.1, pmG5.2, pmG5.3, pmG5.4, pmG6.1, and pmG6.2) associated with CPM resistance were detected on all chromosomes except for Chr.7. Candidate genes (Csa5G622830 and CsGy5G015660), reported in previous CPM resistance studies and cucumber orthologues of several CPM susceptibility (S) genes (PMR5, PMR-6, and MLO) that are colocalized with certain QTLs were analyzed for their potential contributions to resistance QTL effects on both CPM and CDM in the CG population.
Liu et al. (Citation2021) presented an overview of mapped and cloned genes and QTL for CPM resistance in cucumber () with the following basic comments and conclusions. Most of the QTL showed recessive inheritance, indicating that the resistance is governed by loss-of-function mutant alleles of certain susceptible genes (Schouten et al., Citation2014). Indeed, a mutant allele of the cucumber susceptibility gene CsaMLO8 (named as CsMLO1) (Berg et al., Citation2015; Nie et al. Citation2015b) explained the contribution of hypocotyl resistance to CPM by the QTL pm5.1, derived from the accession PI 197088 (Berg et al., Citation2015). In additional to hypocotyl resistance, this QTL was suggested to be necessary for complete or true leaf resistance (Kooistra, Citation1968; Zhang et al., Citation2011a). Susceptibility genes (S-genes) is an alternative strategy for achieving durable resistance (Pavan et al., Citation2009), more than 180 S-genes were identified in various plant species (Van Schie and Takken, Citation2014). Several S-genes have proved to be functional S-genes for CPM, such as MLO-like genes (Mildew Locus O) and PMR (powdery mildew resistant) genes for which cucumber orthologs were identified and mapped to specific chromosomes (Schouten et al., Citation2014). Some of the S-genes colocalize with QTLs for CPM resistance, which may indicate that mutations in certain S-genes explain the QTL effect. This was proven to be true for the loss-of-function mutation in CsaMLO8 that contributes to one QTL for hypocotyl resistance (Berg et al., Citation2015).
Table 6. Sources of resistance, mapped and cloned genes/quantitative trait loci (QTL) for powdery mildew resistance in cucumber (Cucumis sativus) summarized from Liu et al. (Citation2021).
Shimomura et al. (Citation2021) performed QTL analysis using double-digest restriction-site-associated DNA sequencing (ddRAD-Seq) in a population from a cross between highly CPM resistant “Kyuri Chukanbohon Nou 5 Go” (K5) developed from the weedy cucumber line CS-PMR1 and the Japanese cv. Kaga-aonaga-fushinari, which is susceptible to CPM. Line K5 is a progeny from crosses between CS-PMR1 and cvs. Sharp 1 (Saitama Gensyu Ikuseikai Co., Saitama, Japan) and Rira (Enza Zaden BV, Enkhuizen, the Netherlands), and is a Japanese native type cucumber susceptible to Px. The two parental cultivars and the F3 individuals from each F2 progeny plant (F2: 3) were used in leaf disk assays. The Px isolate used in this study originated from a single ascospore of pxB strain, as described by Fukino et al. (Citation2008).
Resistance of the two cucumber populations and their parents was evaluated using leaf disk assays and image analysis (Shimomura et al., Citation2021). They detected one major QTL on chromosome 5 effective at 20 °C and 25 °C, and one minor QTL on chromosome 1 effective at 20 °C. Two additional QTLs were detected in a position different from the major QTL in a subpopulation: one on chromosome 3 effective at 20 °C and one on Chr. 5 effective at both 20 °C and 25 °C. The resistance alleles at all four QTLs were contributed by cv. Kyuri Chukanbohon Nou 5 Go. These results can be used to develop practical DNA markers tightly linked to genes for CPM resistance (Shimomura et al., Citation2021).
Although pm5.3 was necessary for resistance, the three other QTLs were also suggested to be important, especially pm5.1 (Shimomura et al., Citation2021). Furthermore, the difference in resistance between K5 and CS-PMR1 can also be explained by the presence of resistance alleles at pm3.2 and pm5.1, neither of which is present in CS-PMR1. K5 has been used for producing cucumber germplasm resistant to CPM (Px, without specification of race). To develop new cultivars with desired traits introgressed from genetic resources, breeders perform repeated backcrossing and selection for many years. However, it is likely that unfavorable linkages between disease resistance and other important agronomic traits will adversely affect the efficiency of breeding during gene introgression for disease resistance. The most desirable cucumber breeding program for CPM resistance using K5 would seem to require accumulation of the K5 alleles: pm1.1, pm3.2, pm5.1, and most importantly pm5.3 (Shimomura et al., Citation2021).
Recent and most advanced studies are focused on research and development of combined resistances in cucumbers. Lai et al. (Citation2022), for example, reported that RNA-seq data of cucumber under biotic stress conditions, including CDM and CPM were retrieved from NCBI Short Read Archive (SRA) database under the accession numbers SRP009350 and SRP212890, respectively (Adhikari et al., Citation2012; Zhang et al., Citation2020).
Kaur and Sharma (Citation2022) summarized recent advances in cucumber improvement, including development of cultivars resistant to CPM. Unfortunately, authors mention only Px as a unique species causing CPM on cucumbers. They present lists of genes, molecular markers and/or linkage groups reported for responses to this CPM.
5. Physiological background and mechanisms of resistance to CPM
Mechanisms of cucumber resistance to Px and Go are not very well understood. Two different host defense mechanisms against CPM have been identified: hypersensitive response (HR) and cell wall thickening (CWT) (Tek and Calis, Citation2022). HR restricted PM in genotype VT18; CWT was highly expressed in CPM-resistant cv. Melten. The authors stressed that although HR is generally known as a dominant pathogen resistance mechanism, other defense mechanisms could provide resistance against CPM pathogens. CPM-susceptible cucumber cv. Stela F1 did not express HR response against either CPM species, but occurred to a limited extent in CPM-resistant C. melo PI 313970 (Sedlářová et al., 2009). These authors proposed the two basic mechanisms, the first mechanism targets conidial germination and appressoria formation, the second one reduces pathogen growth and reproduction with minima or no HR involvement and may be influenced by the first mechanism (Sedlářová et al., 2009). From a physiological point of view, peroxidase could be involved in resistance mechanisms of cucumbers against CPM (Luhová et al., Citation1999), as well as nitric oxide playing the crucial role in resistance against CDM and CPM (Sedlářová et al., Citation2016).
It was stressed that CPM resistance is complex and polygenic in cucumbers (Tek and Calis, Citation2022; Tek et al., Citation2022; Sun et al., Citation2022). They underline the fact that the strategy of resistance breeding is based on the continuous involvement of R- genes in response to new strains (races) of the CPM pathogens. The MLO-based resistance reported in various plants is complex in cucumbers and involves additional mechanisms such as HR and papillae formation.
Recent CPM resistance research is focused on MLO genes encoding a novel type of plant-specific integral membrane protein with a yet unknown basic biochemical mode of action that varies among plant species. The MLO allele acts early and confers preinvasion resistance by terminating fungal pathogenesis before plant entry during the process of cell wall penetration (Nie et al., Citation2015b). In cucumber, several MLO-like genes have been identified as susceptibility genes to CPM (Schouten et al., Citation2014; Berg et al., Citation2015). In addition, CmMLO2 has been identified in muskmelon as a gene related to CPM pathogenesis (Cheng et al., Citation2013).
A MLO-like gene was identified as the candidate for the major locus pm5.1 through map-based cloning (Nie et al., Citation2015a), designated as CsMLO1 and confirmed to mediate CPM resistance in cucumber in several experiments (Nie et al., Citation2015b). Nie et al. (Citation2015b) revealed that loss of function of CsMLO1 conferred durable CPM resistance, and that this loss of function is necessary; however CsMLO1 alone may not be sufficient for CPM resistance in cucumber. Berg et al. (Citation2015) provided evidence in cucumber of the role of CSaMLO8 gene for CPM-susceptibility.
Molecular mechanisms of CPM resistance in cucurbits are still poorly understood (Xu et al., Citation2016b). Comparative gene expression analysis revealed two concatenated genes, Csa1M064780 and Csa1M064790 encoding the same function of a cysteine-rich receptor-like protein kinase, as the most likely candidate genes. GFP fusion protein-aided subcellular localization indicated that both candidate genes were expressed in the plasma membrane, and that Csa1M064780 was also expressed in the nucleus. This first report of dominantly inherited CPM resistance in cucumber provided new insights into understanding phenotypic and genetic mechanisms of CPM resistance in cucumber (Xu et al., Citation2016b). However, the crucial weaknesses of this study are lack of clear determination of the CPM pathogen on species level, evidence of isolate purity and information on its virulence, i.e., specification of race.
Nie et al. (Citation2021) emphasized that for elucidation of the mechanism of Csmlo1 resistance, genetic factors for the mlo-resistance still need to be explored. Their study was aimed at the function of long noncoding RNAs (lncRNAs), a class of RNAs that have poor protein coding potential and their involvement in mlo-resistance to CPM in cucumber. Differential expression (DE) analysis showed 119 lncRNAs and 136 mRNAs correlated with CPM resistance. Functional analysis of these DE lncRNAs and DE mRNAs revealed they were significantly associated with phenylpropanoid biosynthesis, phenylalanine metabolism, ubiquinone and other terpenoid-quinone biosynthesis, and endocytosis. Two lncRNAs, LNC_006805 and LNC_012667 might play important roles in CPM resistance. The LNC_011741 interacts putatively with four resistance-related genes, among them with Csa7G390190, which encodes an unknown protein that might provide CPM resistance in cucumber (Nie et al., Citation2021).
Recently it was reported that transcripts of the gene CsGy5G015660, encoding a putative leucine-rich repeat receptor-like serine/threonine-protein kinase (RPK2), showed specific accumulation in CPM-resistant line PM-R prior to the appearance of disease symptoms, and was accordingly considered a strong candidate gene for CPM resistance. Natural variation of CsGy5G015660 allele was observed among 115 core germplasm accessions tested by authors (Zhang et al., Citation2021a).
Zhang et al. (Citation2020) used cucumber CPM-resistant cv. BK2 and a susceptible cv. H136 to screen and identify differentially expressed genes (DEGs) in response to Px. There were 97 DEGs between BK2 and H136 under the control condition, suggesting a similarity in the basal gene expression between cvs. BK2 and H136. A large number of hormone signaling-related DEGs (9.2% of all DEGs) between BK2 and H136 were identified, suggesting an involvement of hormone signaling pathways in the resistance to CPM. Defense-related DEGs belonging to Class I were only induced in susceptible H136 and defense-related DEGs belonging to Class II were only induced in resistant BK2. The peroxidase, NBS, glucanase and chitinase genes that were grouped into Class I and II might contribute to production of the resistance to CPM in resistant cultivars. Furthermore, several members of Pathogen Response-2 family, such as glucanases and chitinases, were identified as DEGs, suggesting that cucumber might enhance the resistance to CPM by accelerating the degradation of the pathogen cell walls (Zhang et al., Citation2020).
Differentially accumulated metabolites (DAMs) and differentially expressed genes were screened in CPM resistant BK2 and a susceptible H136 in response to S. fuliginea (Px) infection (Zhang et al., Citation2021b). Most DEGs and DAMs were enriched in several primary and secondary metabolic pathways, including flavonoid, hormone, fatty acid and diterpenoid metabolisms. Many flavonoid-related metabolites were significantly accumulated in BK2 compared with H136, which suggested an essential role of flavonoids in resistance responses. Changes in expression of CYP73A, CYP81E1, CHS, F3H, HCT and F3’M genes provided a probable explanation for the differential accumulation of flavonoid-related metabolites. Interestingly, more hormone-related DEGs were detected in BK2 compared to H136, suggesting a violent response of hormone signaling pathways in the CPM-resistant variety. The number of fatty acid metabolism-related DAMs in H136 was larger than that in BK2, indicating an active fatty acid metabolism in the CPM-susceptible “H136.” It was concluded that many differentially expressed transcription factor genes were identified in response to Px infection, providing some potential regulators for the improvement of CPM resistance. CPM resistance of cucumber was controlled by a complex network consisting of various hormonal and metabolic pathways (Zhang et al., Citation2021b).
The response of two cucumber lines (resistant and susceptible) to CPM (species not specified) was studied in early stages of pathogenesis (24 and 48 hours after inoculation). They identified 5,478 DEGs between the lines. The functions of DEGs were analyzed and the complex regulatory network for CPM resistance was assessed, which involves plant hormone signal transduction, phenylpropanoid biosynthesis, plant–pathogen interaction and the MAPK (Mitogen-activated protein kinase) signaling pathway (Zheng et al., Citation2021).
Yang et al. (Citation2022) recently studied the expression profile of the OSCA (Hyperosmolality-gated calcium-permeable channels) gene family related to abiotic and biotic stress response in cucumber. Some cucumber OSCA genes were found to be differentially expressed in responses to various biotic stresses, which has not been reported to date in other plant species. This study lays a foundation for research on the biological function and evolutionary process of OSCA family genes in cucumber. Gene expression analysis indicated that the CsOSCA genes have different expression patterns in various tissues, and some of them were regulated by three osmotic-related abiotic stresses (salt, drought and ABA) and three biotic stresses (CPM, CDM, and root-knot nematode), which is of great significance for exploiting stress-resistant cucumber varieties (Yang et al., Citation2022).
Valine-glutamine (VQ) motif-containing proteins are plant-specific transcription factors with critical functions in plant growth, development and defense reactions (Lai et al., Citation2022). RNA-seq analysis revealed changes in the expression of some CsVQ genes (such as CsVQ2, CsVQ9, CsVQ12, CsVQ13, CsVQ20 and CsVQ22) in response to CDM and CPM inoculation. Their findings provide a theoretical basis for studying the potential roles of CsVQ genes in the development and stress response of cucumber in the future (Lai et al., Citation2022).
Bioinformatics approaches are being applied in cucumber resistance research. The NBS-LRR (NLR) gene family plays a pivotal role in regulating disease defense response in plants. Zhang et al. (2022) perfomed bioinformatical analysis to systematically characterize and understand the role of the CC-NBS-LRR(CNL) family of genes in disease defense responses in cucumber plants.
C. Melon (Cucumis melo)
1. Introduction
A review of the achievements in research and breeding of melon for different traits (Thakur et al., Citation2019; Xu et al., Citation2022) and resistance to CPM through the 20th century concluded (Jahn et al., Citation2002) that all genes for CPM resistance in European, Japanese and U.S. varieties had been derived from germplasm collected in India (Pitrat et al., Citation1998), which is one of the centers of origin of this species. Melon CPM resistance breeding started in 1925 in the U.S.A. after powdery mildew caused significant production losses to growers in Imperial Valley, California (Jagger, Citation1926; Jagger and Scott, Citation1937). The first source of resistance identified was California accessison #525, which originated from India. A cross with Hale’s Best led to the first CPM-resistant, orange flesh melon breeding line Powdery Mildew Resistant Cantaloupe No. 50 (Jagger et al., Citation1938a). Inheritance studies of CPM (regarded at that time as incited by E. cichoracearum see McCreight, Citation2004) resistance led to the description of the gene Pm-1 (Jagger et al., Citation1938b).
Next studies have led to the identification of resistance genes to Go and Px, and their specification with respect to race (). As stated by Jahn et al. (Citation2002), in spite of the progress in molecular mapping, no useful linkages have been reported until the end of the 20th century for any of the melon PM resistance genes (Pitrat et al., Citation1998).
Table 7. Melon (Cucumis melo) resistance genes and quantitative trait loci (QTL) to Golovinomyces orontii (Go) and Podosphaera xanthii (Px) and their sources as summarized by Dogimont and Sari (Citation2022).
2. Inoculation experiments, screening of responses to (specific races) Gc and Px, detection of resistant genotypes, sources of resistance germplasm evaluation
The melon gene list is regularly updated within the activities of the Cucurbit Genetics Cooperative with the last version published by Dogimont and Sari (Citation2022). They provide all available data and reports on genes conferring resistance to CPM. Resistance is frequently race-specific and effective against respective races of Px, however, a succession of new races rapidly takes place (Hosoya et al., Citation2000).
McCreight (Citation2003) reported resistance to Px in melon accession PI 313970, which originated in India. Three genes for resistance to Px race 1 and race 2 U.S. were identified in growth chamber and greenhouse tests in the cross PI 313970 × Top Mark. A recessive gene conditioned resistance of true leaves to race 1. A recessive gene appeared to condition resistance of cotyledons to race 2 U.S., although a second recessive gene may be involved. A semi-dominant gene conditioned resistance of true leaves to race 2 U.S. Limited data suggested linkage of the recessive gene for resistance to race 1 and the semi-dominant gene for resistance to race 2 U.S. The resistance reaction of PI 313970 to infection of true leaves by race 2 U.S. included water-soaked spots and resistant blisters (Sedlářová et al., 2009), but segregation data for the resistant blister reaction were inconclusive, so McCreight (Citation2003) concluded that allelic relationships of these genes with previously reported melon CPM resistance genes remain to be determined.
C. melo genotypes MR-1 and PI 124112 were considered for a long time as highly resistant to CPM (e.g., Sitterly, Citation1978; Jahn et al., Citation2002). This conclusion was primarilly made on field studies and reaction to Px. However, detailed experimental study based on inoculation of leaf disks, derived from adult C. melo plants, by Go and Px isolates showed that both genotypes are susceptible to some isolates of both CPM species and that there could be located unknown race-specific resistance factors (genes). In some cases there was also recorded expression of incomplete resistance to both CPM (Křístková et al., 2002, 2004).
McCreight (Citation2006) evaluated twenty melon cultigens or their reaction to Px race 1 in two open field tests in Imperial Valley, California (USA), and one growth chamber test in Salinas, California. The 11 PM race differentials reacted as expected; Iran H, which had not been tested previously against a U.S. population of Px race 1, was susceptible in all three tests. Consistent with previous reports, Perlita, Seminole, PI 179901, PI 234607 and PI 313970 were resistant in all three tests. Amarillo, Moscatel Grande and Negro, previously reported resistant to Px race 1 in Spain, were resistant in the field tests, but were highly susceptible in the growth chamber. PI 236355, which was previously reported resistant to Px race 1, was susceptible in all three tests.
McCreight and Coffey (Citation2012) turned their attention to accessions C. melo ssp. agrestis as potential useful sources of genes for host plant resistance to Px races 1, S, SW, SD and SDW, where race S does not infect PI 313970 and SD infects PI 313970, and SDW also infects watermelon.
Alvarez et al. (Citation2005) evaluated 128 accessions C. melo for resistance to Px races 1 and 2. The screening revealed that sources of natural resistance CPM are limited, and intra-specific variability for resistance to CPM in C. melo was poor. Nevertheless, six Spanish cultivars and the accessions TGR-1551, CUM-313, and CUM-129 were resistant to races 1 and 2.
Sales Júnior et al. (2011) evaluated the reactions of 144 families, 19 lines and three cultivars (Yellow Queen, Chilton, Hy Mark) of melon to Px under field conditions at two locations in Brazil. Families 4, 5, 11, 14, 18, 23, 33, 42, 101, 112 and 140 were identified as resistant at both sites. Lines LOF-027, LOF-105, LPS-124, MEL-008 and cv. Chilton showed resistance to race 1.
Lee et al. (Citation2014) studied responses of 26 melon cultivars to Px race 1. They observed that the degree of resistance of cotyledons was not correlated with whole plant responses, but CPM reactions in the first true leaf was highly correlated with resistance reactions of the whole plant. Resistant cultivars demonstrated strong resistance in three seasons from November 2010 through May 2011. Interestingly, five of 10 cultivars claimed resistant to race 1 were highly susceptible in all three seasons.
Nunes et al. (Citation2017c) reported that five accessions of melon exhibited resistance to Px in inoculated greenhouse tests in Spain and Mexico. Accessions (AC-02, AC-09, AC-15 and AC-34) exhibited characteristics of botanical variety acidulus, whereas accession AM-55 exhibited characteristics of variety momordica. Further studies of inheritance of resistance to Px (race not specified) in accessions AM-55 and AC-15, exotic types from India and Brazil, respectively, indicated a monogenic, dominant inheritance in AM-55 and two independent genes with epistatic interaction in AC-15 (Nunes et al., Citation2017b).
Brazilian germplasm, a set of Indian landraces, and COMAV (Institute for the Conservation and Breeding of Agricultural Biodiversity, Valencia, Spain) melon core collection, representing the whole variability of the species, were assessed for resistance against some common races in Spain and Brazil (Nunes et al., Citation2017a). The 123-SNP (single nucleotide polymorphisms) genotyping platform was used to assess the molecular relationships of the resistant accessions. Accessions AL-1, BA-3, CE-3, and RN-2, within the Brazilian collection, presented resistance against all the races of Px assayed in all conditions tested, which were identified as races 1, 3, and 5 in Brazil, and race 3.5 in Spain. AL-1, CE-3 and RN-2 were molecularly more similar to wild Agrestis and Acidulus melons from Asia and Africa, whereas BA-3 grouped with Momordica types. Molecular analysis confirmed that these new Brazilian sources of resistance differ from those previously reported, constituting interesting materials to encourage genetic breeding programmes, especially in Brazil and Spain.
El-Sayed et al. (Citation2019) confirmed the existence of the genetic system controlling resistance to Ec (identity not verified by authors), and the suitability of inbred melon line 4 (Edisto 47) as a good genetic source for development of CPM-resistant breeding lines in Egypt. Edisto 47 was previously reported resistant to Px race 1 (Thomas, Citation1978).
Exploitation of melon CPM-resistant genotypes is often, as stated by Chikh-Rouhou et al. (Citation2022), complicated by low quality fruit and unfavorable agronomic characteristics. They looked for novel sources of resistance within Tunisian landraces as a convenient step to obtain new CPM-resistant melon varieties/hybrids suitable for Mediterranean markets. Several Tunisian melon landraces have been tested against three common races of Px in Mediterranean regions (2, 3.5, and 5), using phenotypic approaches in two independent experiments (artificial inoculations in growth chambers and natural of infection in a greenhouse). All the tested landraces were susceptible to races 3.5 and 5; several landraces were resistant to race 2. Under natural conditions of infection, Px race 2 was prevalent in the field and the resistance of TUN-16, TUN-19, and TUN-25 was confirmed. The resistances were race-specific and underlie a high genetic influence reflected in the high value of the estimated heritability, h2 = 0.86.
3. Reports on resistant genotypes, lines, cultivars
Henning et al. (Citation2005a) provided pedigree of the hybrid melon Hannah´s Choice F1, developed at Cornell University, Ithaca (U.S.A.). One parent, NPCM Rd was derived from a CPM-resistant Charentais type that was crossed with a PMR version of Iroquois and a monecious Charentais type. The second parent, ZBC5TUF14 was derived from PI 414723 × TAMU Valde. PI 414723 is resistant to a wide array of Px races (McCreight, Citation2006) in addition to multiuple virus resistances (Anagnostou et al., Citation2000) and melon aphid (Aphis gossypii) (McCreight et al., Citation1984). TAM Uvalde is an orange flesh, U.S. western shipper type melon with resistance to Px races 1 and 2 (Crosby et al., Citation2008). The hybrid combines resistance to the P. xanthii races 1 and 2, and some resistance to Fusarium wilt (Fusarium oxysporum f. sp. melonis) race 2. Hannah´s Choice F1 is the first melon to have combined resistance to three potyviruses, namely watermelon mosaic virus (WMV), papaya ringspot virus (PRSV), and zucchini yellow mosaic virus (ZYMV). Also, it has shown some field tolerance to spider mites (Tetranychus urticae). Lastly, it has shown some field tolerance to cucurbit downy mildew (Pseudoperonospora cubensis), angular leaf spot (Pseudomonas syringae pv. lachrymans), and gummy stem blight (Didymella bryoniae) (Henning et al., Citation2005a).
PMR Delicious 51 is a new and improved version of Delicious 51 an orange flesh, U.S. Eastern type melon. PMR Delicious 51 has excellent resistance to Px races 1 and 2 and resistance to Fusarium wilt (Fusarium oxysporum f. sp. melonis) race 2 (Henning et al., Citation2005b).
Melon breeding line Carmen with resistance to Px, Aphis gossypii and cucurbit yellow stunting disorder virus (CYSDV) was derived through backcrossing from the cross between the line TGR-1551 (Group Agrestis) and Bola de Oro (Group Ibericus sub-group amarillo) by Palomares-Rius et al. (Citation2018). Though no replicated bioassays were carried out for CPM resistance, its responses to natural CPM infection were recorded along with those of PMR 45, WMR 29, Edisto 47, and PI 414723, which led to the identification of the predominant Px race 5 in the greenhouse were its PMR was noted. Authors reported that no plants of Carmen showed symptoms of CPM under natural conditions of infection and they carried the resistant allele of the PM3 and MRGH63 markers associated with the dominant gene in LG5 of TGR-1551. Regarding the recessive gene on LG12, none of the plants carried the TGR-1551 allele at the SNP61 locus, therefore, indicating that the CPM resistance found in Carmen comes from the dominant gene located on LG5 (Palomares-Rius et al., Citation2018).
Vachev et al. (Citation2022) report the development of the cantaloupe hybrid Triton that combines CPM and Fusarium wilt resistance with improved fruit quality.
4. Detection of resistance genes, inheritance studies, applications in breeding, genetic analyses
The first reference genome (Version 3.5) of melon with 375 Mb total length and 27,427 protein-coding genes released by Garcia-Mas et al. (Citation2012) in the framework of the MELONOMICS project was updated to Version 3.5.1 by Argyris et al. (Citation2015). An improved assembly (Version 3.6.1) of the melon genome and new genome annotation (Version 4.0) were provided by Ruggieri et al. (Citation2018), which corrected the order and the orientation of 21 previous scaffolds and identified 8,000 new genes. Castanera et al. (Citation2019) improved the melon genome assembly (Version 4.0) with the PacBio singlemolecule real-time (SMRT) sequencing technology, reduced the unassigned sequences substantially, made a great effort to distinguish new gene or transposon variants related to important phenotypes. Recently, several melon genome assemblies by de novo sequencing based on genetically diverse individuals provide insights into genome structures, genome evolution, diversification, and identified candidate genes for several agronomic traits of melon (Shin et al., Citation2019; Zhang et al., Citation2019; Yang et al., Citation2020).
Kuzuya et al. (Citation2003; Citation2006) proved the suitability of the protocol to detect resistance to Px at the haploid level, as the haploid breeding is powerful tool for the production of pure lines in melon.
Anagnostou et al. (Citation2000) show that the breeding line 414723-4S3 derived from PI 414723 can serve as a source of dominant monogenic resistance to PRSV as well as to Px race 1. They mentioned that heterogeneity of PI 414723 was reported not only with respect to viruses, but also with respect to PMR genes, and a recessive gene for CPM race 1 resistance, as opposed to the dominant one identified by Anagnostou et al. (Citation2000), which was reported in line 92417 that has common ancestry with PI 414723 (McCreight et al., Citation1987).
Resistance of melon aphid-resistant PMAR No. 5 (likely synonymous with breeding line AR 5) to Px race 1 appeared to be conferred by two dominant and independent genes (Fukino et al., Citation2004). PMR of PMAR No. 5 may be attributed to PMR 5 or to PI 414723 the source of melon aphid resistance (McCreight et al., Citation1984).
An AFLP marker linked to the melon Pm-1 gene that confers resistance to Px was developed by Teixeira et al. (Citation2006; Citation2008).
Pitrat and Besombes (Citation2008) studied the inheritance to Px in melon progeny 90625 derived from a bulk increase of two plants of PI 313970 (J. D. McCreight, personal communication), which is a potential source of resistance to CPM strains (races) recently characterized in France. They concluded that five CPM resistance genes could be present in 90625. Two independent semi-dominant genes (A and B) are involved in resistance to strains 06Sm10 and S87-7, respectively. Resistance to the other strains seems to be under a digenic control, including A and B or not, with three other recessive genes. Some of these RILs could be used as additional differential lines for the characterization of the races.
Yuste-Lisbona et al. (Citation2010a; Citation2011b) studied the inheritance of resistance to Px races 1, 2 and 5 in the African melon TGR-1551 from a cross with the susceptible “Bola de Oro” under artificial inoculation. The segregation ratios for resistance/susceptibility observed in the different populations and the results of the progeny test indicated that resistance to Px races 1, 2 and 5 was governed by two independent genes, one dominant and one recessive, which means that the genetic control is a dominant-recessive epistasis. They conluded that this was the first report of a recessive melon gene that confers resistance to more than one Px race. TGR-1551 is a potentially valuable source of CPM-resistance genes. These authors constructed a genetic linkage map and identified a major QTL conferring resistance to Px in TGR-1551 (Yuste-Lisboa et al., Citation2010b; Citation2011b). They detected a dominant QTL for resistance to either races 1, 2 or 5, all located at the same genomic region of G4. Therefore, these QTL were considered as a unique QTL designated as Pm-R. In addition, four codominant markers, previously described as linked to the dominant CPM resistance gene, were linked to the Pm-R QTL, which suggests the correspondence between this QTL and the dominant resistance gene Pm-R. One of the codominant markers, PM3-CAPS, co-segregated with the Pm-R QTL, and the markers PM2-CAPS and PM4-dCAPS flanked this QTL (Yuste-Lisbona et al., Citation2011a, Citation2011b).
Yuste-Lisbona et al. (Citation2011a) cloned and sequenced the selected AFLP fragments of the genotype TGR-1551 bearing a dominant gene that provide resistance to the Px races 1, 2, and 5. They identified the NBS-LRR genes (the nucleotide binding site (NBS)-leucine-rich repeat (LRR) gene family) in LG V, harboring candidate genes for resistance to Px races 1, 2, and 5. Evaluation of other resistant germplasm showed that the codominant markers were also linked to the Pm-w resistance gene carried by the breeding line WMR-29, proving their usefulness as genotyping tools in melon breeding programmes (Yuste-Lisbona et al., Citation2011a).
López-Martín et al. (Citation2022) used several densely genotyped BC3 families derived from the cross between TGR-1551, resistant to Px races 1, 2, and 5, and the susceptible “Bola de Oro” to finely map these resistance regions. Further phenotyping and genotyping of selected BC5, BC5S1, BC5S2, BC4S1, BC4 × PMS, and (BC4 × PMS) S1 offspring allowed for the narrowing of the candidate intervals to a 250 and 381 kb region of chromosomes 5 and 12, respectively. They confirmed the temperature effect on resistance provided by the dominant gene. High resolution melting markers (HRM) were tightly linked to both resistance regions and will be useful in marker-assisted selection programs. Candidate R genes with variants between parents that caused a potential modifier impact on the protein function were identified within both intervals. These candidate genes provide targets for future functional analyses to better understand the resistance to CPM in melons.
Genetic analysis of resistance to Px race 2 F in wild melon material Yuntian-930 showed that this resistance was controlled not just by two major genes, but was also affected by polygene and the environment (Xian et al., Citation2011).
Wang et al. (Citation2011) used two F2 populations derived from Ano2 × Hami413 and Ano2 × Queen to map resistance to Px race 1 using Bulked Segregation Analysis (BSA), comparative genomics and Resistance Gene Analogues (RGA) mapping. They found that the resistance to CPM in Ano2 was conferred by a dominant gene, Pm-AN. Genetic analysis revealed that Pm-AN is located between codominant markers RPW and MRGH63B in linkage group V. Synteny analysis showed that markers in this region were collinear between melon and cucumber.
Fazza et al. (Citation2013) studied inheritance of resistance to Px races 1, 3 and 5 through analyses of 87 F2 plants from the cross of PI 414723 (resistant to these races) × Védrantais (susceptible). Resistance to all three races could be explained by segregation of a single dominant gene, although a digenic model could also be accepted. A genetic map was assembled with 206 markers, and co-segregation analysis of resistance phenotypes indicated the existence of two linked loci in linkage group II, one conferring resistance to races 1 and 5 (denominated Pm-x1,5), and the second to race 3 (denominated Pm-x3), located 5.1 cM apart.
Cui et al. (Citation2020) used BSA and QTL analyses to identify two CPM resistance loci, Pm2.1 and pm12.1, and mapped them on chromosomes 2 and 12 of PMR 6, resistant to the Px race 2 F. They developed a new marker-assisted selection system to identify CPM-resistant and susceptible genotypes, and assayed 93 melon accessions. Nucleotide diversity (pi) and fixation index (Fst) for the two PMR loci were estimated using resequencing data of 336 melons from three groups: C. melo ssp. agrestis (156 accessions), C. melo ssp. melo (106 accessions), and intermediate types (interspecific between the two sub-species). The lowest pi was observed in ssp. agrestis, and the highest Fst value was between ssp. agrestis and ssp. melo.
5. Genetic mapping and development of molecular markers
QTL mapping has led to the construction of genetic map and identification of DNA markers related to CPM (Px races 1, 2, 3, and 5, and Gc race 1), and CDM (Perchepied et al., Citation2005) resistances (). Two independent major genes, PmV.1 and PmXII.1, were identified and shown to be involved in major gene resistance to CPM. Three digenic epistatic interactions involving four loci were detected for two Px races and one Go race. Co-localization between PmV.1, resistance genes, and resistance gene homologues was observed. Linkage between the major resistance QTL to P. cubensis, pcXII.1, and one of the two CPM resistance genes, PmXII.1, was demonstrated (). Fukino et al. (Citation2008) identified QTLs for resistance to Px strains pxA and pxB.
Table 8. Melon (Cucumis melo) quantitative trait loci (QTL) for resistance to Podosphaera xanthii, including description and number of reported QTL, and their respective parental lines (Dogimont and Sari, Citation2022).
Liu et al. (Citation2010) described a unique race of Px, denoted pxCh1, in the Shanghai area based on the base of a set of 10 melon CPM race differentials. Resistance to Px race pxCh1 in Ames 31282 (from China; originally reported as PI 134198, which originated from Pakistan), was controlled by a single, dominant gene, designated Pm-8, in a cross with susceptible inbred S717. A search for a molecular marker linked to Pm-8 was initiated using the same cross. BSA of their F2 progeny revealed one sequence-related amplified polymorphism (SRAP) marker of 148 bp linked to Pm-8, at 3.9 cM. The SRAP segment was recovered, cloned, and sequenced and converted into a sequence-amplified characterized region marker for marker-assisted selection of Pm-8 in breeding programs to develop melon cultivars resistant to Px race pxCh1. Development of molecular markers to select melon resistant lines and to differentiate the CPM races was used in South Korea (Kim et al., Citation2015a; Citation2016a; Citation2016b).
Zhang et al. (Citation2013) developed CAPD (CAPD-Dde 1) marker that can be used for marker-assisted selection in CPM resistance breeding. The putative resistance gene cluster provides a potential target site for further fine mapping and cloning of Pm-2F.
Ning et al. (Citation2014) studied the inheritance and location of a CPM resistance gene in Edisto 47 to Px race1 strains Px1A and Px1B in Xinjiang, which reportedly have different pathogenicities. Resistance to Px1A was controlled by two dominant genes identified two QTL on LGII and LGV. Edisto 47 has one dominant gene for resistance to Px1B. Genetic analysis showed that Pm-Edisto47-1 was located between simple sequence repeat (SSR) markers CMGA36 and SSR252089, at a genetic distance of 2.1 cM to both markers. Synteny analysis showed that two genes named MELO3C015353 and MELO3C015354 were predicted as candidates for Pm-Edisto47-1 in this region.
Wang et al. (Citation2016) used the F2 populations from the cross of TARI-08874 (C. melo ssp. melo) and Bai-li-gua (C. melo ssp. agrestis) to map quantitative trait loci (QTLs) for fruit-related traits and PMR in two trials. They identified one major QTL for PMR in LG2 that was closely linked to the SSR marker CMBR120. They supposed that pyramiding desirable traits to develop new high-quality melon cultivars that are resistant to CPM should be possible.
Li et al. (Citation2017) used an F2 melon population, derived from a cross between PMR-resistant MR-1 and susceptible cv. Top Mark, for identification of major Px resistance genes by BSA (bulked segregant analysis); 17 candidate genes were identified, seven of which were predicted as candidate genes related to CPM resistance.
The PCR-based InDel marker developed by Choi et al. (Citation2020) distinguished PM (without species specification) resistant MR-1 from susceptible Top Mark and their progeny. They suggest that this InDEl marker could be used to develop CPM resistant varieties based on MR-1.
The novel QTL and candidate gene identified by Cao et al. (Citation2020; Citation2022) provide insights into the genetic mechanism of Px (assumed species and race not specified) resistance in melon. They considered the tightly linked competitive allele-specific PCR (KASP) markers developed in this research potentially useful for MAS to improve CPM resistance in melon breeding programs.
Whole genome re-sequencing of four melon lines (SCNU1154, Edisto 47, MR-1, and PMR 5) identified SNPs and InDels associated with Px defense genes that will serve as candidate polymorphisms in the search for sources of resistance against Px and could accelerate marker-assisted breeding of melon (Natarajan et al., Citation2016).
Howlader et al. (Citation2020) developed CPM race 5-specific resistance SNP markers using whole-genome resequencing potentially useful for MAS breeding programmes in melon.
Cui et al. (Citation2022a) identified two genes for resistance to Px race 2 in breeding line MR-1. CPM resistance in MR-1 leaves was linked to a dominant gene (CmPMRl), whereas stem resistance was under the control of a recessive gene (CmPMrs), with the dominant gene epistatic to the recessive gene. The CmPMRl gene was mapped to a 50 Kb interval on chromosome 12, while CmPMrs was mapped to an 89 Kb interval on chromosome 10. The CmPMRl candidate gene MELO3C002441 and the CmPMrs candidate gene MELO3C012438 were identified through sequence alignment, functional annotation, and expression pattern analyses of all genes within these respective intervals. MELO3C002441 and MELO3C012438 were localized to the cellular membrane. MELO3C002441 protein clustered with soybean GmNPR4 and GmNRP4-like proteins, and was genetically distant from NPR proteins in other crops, suggesting that this gene is an anchor protein repeat sequence-containing gene that is functionally similar to the soybean NPR4 gene (Ankyrin repeat protein). MELO3C012438 was 90% homologous to the cucumber CsMLO8 gene (and Arabidopsis AtMLO2, AtMLO6, and AtMLO12) that has previously been linked to partial PMR in cucumber stem, petiole, and hypocotyl tissues. CsMLO8 is a recessive resistance gene and a member of the MLO gene family identified within the region of this chromosome identified via finally-mapping. These results offer new genetic resources and markers to guide future MAS for PM resistance in melon.
Branham et al. (Citation2021) combined traditional QTL mapping with whole-genome resequencing of the parents to identify two major QTLs associated with resistance to Px race 1, narrow their intervals, pinpoint candidate genes, and develop linked codominant markers for MAS of PM resistance.
Sun et al. (Citation2021) performed whole transcriptome sequencing using the leaves of PM-resistant (M1) and a CPM-susceptible (B29) to identify circRNAs and determine their molecular functions. A total of 303 circRNAs were identified and >50% circRNAs were derived from exonic regions. Expression levels were significantly altered in 17 and 23 circRNAs after CPM infections in B29 and M1, respectively. Melon circRNAs may participate in the response to biotic stimuli, oxidation reduction, metabolic processes, and the regulation of gene expression based on the functional annotation of circRNA parental genes. Furthermore, 27 circRNAs were predicted to be potential targets or “sponges” for 18 microRNAs (miRNAs).
Ünlü et al. (Citation2022) mapped a QTL on linkage group 1 for resistance to Px race 5 in a cross of PMR 6 (resistant to Px races 1, 2 and 5) with TKÜ3 (susceptible) and identified molecular markers linked with the Pm-R gene, which provides potential for MAS for resistance to Px race 5. The SSR12202 marker is linked to the Pm-R gene QTL at 2.12 cM genetic distance, and the CMCT170b and CMMS30-3 markers are determined in the support interval of this QTL.
Zhang et al. (Citation2023a) characterized the recessive gene Cmpmr2F associated with the resistance against the Px race 2 F. Fine genetic mapping revealed the major-effect region of a 26.25-kb interval on chromosome 12, which harbored the Cmpmr2F gene corresponding to the MELO3C002403, encoding allantoate amidohydrolase.
6. MLO
As stated by Kusch and Panstruga (Citation2017), loss-of-function mutations of one or more of the appropriate Mildew resistance locus o (mlo) genes are an apparently reliable “weapon” to protect plants from infection by PM fungi, as they confer durable broad-spectrum resistance. Originally detected as a natural mutation in an Ethiopian barley landrace, this so-called mlo-based resistance has been successfully employed in European barley breeding and agriculture for nearly four decades. More recently, mlo-mediated resistance was discovered to be inducible in virtually every plant species of economic or scientific relevance. By now, mlo resistance has been found (as natural mutants) or generated (by induced mutagenesis, gene silencing, and targeted or nontargeted gene knock-out) in a broad range of monocotyledonous and dicotyledonous plant species.
Cheng et al. (Citation2012) reported that CmMlo1 is mainly expressed in melon cotyledons and flowers, with a tissue-specific distribution manner. Because CmMlo1 expression was not obvious under PM stress, but under cadmium stress, and its expression was significantly upregulated, they conclude that CmMlo1 is possibly involved in abiotic stress response.
Cheng et al. (Citation2013) indicated that melon CmMLO2 is mainly expressed in the leaves and fruits, and as its expression in the leaves changed significantly after CPM inoculation, they concluded that CmMLO2 is related with the pathogenesis of CPM, and may play an important role in biotic stress response.
Cheng et al. (Citation2015) identified CmMLO2_1 a likely natural mutation of CmMLO2 in the inbred line C18, described as a disease-resistant wild melon C. chate (possibly synonymous with C. melo Group Chate) that may be related to natural resistance to Px race 1. Agrobacterium-mediated transformation of susceptible inbred line G24 with CmMLO2 resulted in resistance to Px race 1 Cheng et al. (Citation2013).
Andolfo et al. (Citation2019) stress that the role of MLO susceptibility genes in plant-pathogen interactions is still elusive, though it is known they are strongly upregulated following CPM infection. They provided insight on the PPR (Putative Promoter Regions) of the MLO gene family, reported the characterization of a candidate MLO susceptibility gene in melon, and highlighted PPR diagnostic features that could be used to identify MLO susceptibility genes across cultivated species. This information is extremely valuable for breeding purposes, as the inactivation of MLO susceptibility genes, for example by targeted silencing and genome editing, might lead to rapid preliminary identification of PMR genotypes.
Iovieno et al. (Citation2015) exploited recently available genomic information to provide a comprehensive overview of the MLO gene family in Cucurbitaceae. They reported the identification of 16 MLO homologs in C. melo, 14 in C. lanatus and 18 in C. pepo genomes. Bioinformatic treatment of data allowed phylogenetic inference and the prediction of several ortholog pairs and groups. They identified a series of conserved amino acid residues and motifs that are likely to play a major role in the function of MLO proteins.
7. WRKY proteins
Jiao et al. (Citation2018) and Wani et al. (Citation2021) summarize recent knowledge on the WRKY proteins which constitute a large family of transcription factors playing a wide range of regulatory roles in multiple biological processes; however, little information is known about WRKY genes in melon. A multiple sequence alignment and phylogenetic analysis using melon, cucumber and watermelon predicted WRKY domains indicated that melon WRKY proteins could be classified into three main groups (I-III). Expression profiles of CmWRKY derived from RNA-seq data and quantitative RT-PCR (qRT-PCR) analyses showed distinct expression patterns in various tissues, and the expression of 16 CmWRKY were altered following CPM infection of melon. Jiao et al. (Citation2018) found 24 WRKY genes co-expressed with 11 VQ family genes in melon. Comparative genomic analyses would promote PMR studies in melon.
Chen et al. (Citation2021b) identified 57 candidate WRKY genes in the melon genome. Expression of 16 selected melon WRKY genes was detected by qRT-PCR after treatment with salicylic acid (SA) or CPM infection (species not specified). qRT-PCR analysis showed that 16 CmWRKY genes exhibited distinct expression patterns upon CPM infection, whereby expression levels of nine genes were inhibited, and seven genes were induced.
8. Physiological background and resistance to CPM
Liu et al. (Citation2014) investigated differential gene expression in incompatible Px-melon interactions and identified one EST containing homologous sequences to S-adenosylmethionine decarboxylase (SAMDC) cDNA. Given this, the SAMDC gene was cloned and designated CmSAMDC. It was 1,095 bp long and encoded a 364-amino acid peptide with a molecular mass of 40 kD. The deduced CmSAMDC protein was shown to have two conserved regions of a putative proenzyme cleavage site and a PEST domain. Expression of CmSAMDC in the PMR melons increased more sharply than in susceptible melons, and the higher polyamines (PAs) and hydrogen peroxide (H2O2) contents in resistant melons were found as well, accompanied by up-regulation of stress-responsive defense enzyme activities. They hypothesized that CmSAMDC and, perhaps its orthologous genes, might be involved in responses of plants to biotrophic pathogens.
Zhu et al. (Citation2018) conducted a comparative transcriptome analysis of mRNA transcripts from Px-resistant MR-1 and susceptible Top Mark. Leaves inoculated with Px spores were collected from 0 to 168 hpi in order to identify candidate regulators for PMR at different infection stages. They identified 1,888 and 2,842 differentially expressed genes in MR-1 and Top Mark, respectively, during the infection period. Gene function analysis of these differentially expressed genes (DEGs) classified them into seven functional groups: pathogen recognition, signal transduction, transcription factors (TFs), phytoalexin biosynthesis, other primary metabolite functions, MLOs, and pathogenesis-related (PR) proteins. Expression of ten of these genes in MR-1 and Top Mark was validated via qRT-PCR. They concluded that PRR and R proteins in MR-1 quickly perceive and detect the corresponding PAMPs and pathogenic effectors released by Px and induce defense signal transduction, including JA, SA, ABA, ET, Ca2+ and ROS pathways, while PTI and ETI are delayed or repressed in Top Mark after Px infection. Analysis of TFs among the identified DEGs implicated well-studied TF families with known important roles in defense resistance, such as the MYB, WRKY, NAC, and ERF families, which are activated and subsequently regulate the production of downstream disease defense associated with metabolites, including PR proteins, phenolic compounds, phenylpropanoids, flavonoids and stilbenoids. Some primary metabolism and MLO genes may also play an important role in the defense response via atypical mechanisms. Hypersensitive necrosis ultimately occurs at infection sites on MR-1 leaves that limit further PM development.
Polonio et al. (Citation2019) used RNA-sequencing to identify differentially expressed genes in melon leaves upon inoculation with Px, using RNA samples obtained at different time points during the early stages of infection and their corresponding uninoculated controls. In parallel, melon plants were phenotypically characterized using imaging techniques. Authors found a high number of differentially expressed genes (DEGs) in infected plants, which allowed the identification of many plant processes that were dysregulated by the infection. Among those, genes involved in photosynthesis and related processes were found to be upregulated, whereas genes involved in secondary metabolism pathways, such as phenylpropanoid biosynthesis, were downregulated. These changes in gene expression could be functionally validated by chlorophyll fluorescence imaging and blue-green fluorescence imaging analyses, which corroborated the alterations in photosynthetic activity and the suppression of phenolic compound biosynthesis. They concluded that CPM disease in melon is a consequence of a complex and multifaceted process that involves the dysregulation of many plant pathways such as primary and secondary metabolism.
The study of Zhou et al. (Citation2020) was based on the fact that long noncoding RNAs (lncRNAs) have emerged as new regulators in plant response to biotic stresses. They identified the intricate regulatory roles of lncRNAs in melon response to CPM fungi. A total of 539 lncRNAs were identified from PMR (MR-1) and susceptible (“Top Mark”), in which 254 were significantly altered after CPM infection. Multiple target genes of lncRNAs were found to be involved in hydrolysis of chitin, callose deposition and cell wall thickening, plant-pathogen interaction and plant hormone signal transduction pathways. Additionally, 42 lncRNAs that have various functions with microRNAs (miRNAs), including lncRNAs are targeted by miRNAs and function as miRNA precursors or miRNA sponges.
Beraldo-Hoischen et al. (Citation2021) studied the role of calose deposition in several melon accessions carrying different PMR genes during infection by Px races 1, 2, and 5. They observed the influence of temperature in some cases affecting the timing of fungal development arrest. Four resistance behaviors were observed: type I and II, as described previously, as well as an earlier and a later type II: IIa and IIb, respectively. Melon genotypes, showing posthaustorial resistance mechanism types IIa and IIb, mainly those genotypes carrying QTL of linkage group V for PMR such as TGR-1551, seemed to exhibit different responses according to temperature, affecting fungal development.
Wang et al. (Citation2021a) integrated transcriptomic and methylomic analyses to explore whether DNA methylation was involved in modulating transcriptional acclimation of melon to Px infection. Net photosynthetic rate (Pn), stomatal conductance (Gs), actual photochemical efficiency (ФPSII) and maximum PSII quantum yield (Fv/Fm) were significantly decreased in Px-infected plants relative to uninfected ones, revealing apparent physiological disorders. In total, 4,808 differentially expressed genes (DEGs) were identified by global analysis of gene expression in uninfected and Px-infected plants. Comparative methylome uncovered that 932 DEGs were associated with hypermethylation, while 603 DEGs were associated with hypomethylation in melon upon Px infection. Among these differential methylation-involved DEGs, a set of resistance-related genes including R genes and candidate genes in metabolic and defense pathways were further identified, demonstrating that DNA methylation might function as a new regulatory layer for melon resistance to Px infection.
In oriental melon (Cucumis melo Group Makuwa) leaves it was found that red light promoted salicylic acid (SA) accumulation and powdery mildew resistance by activating the transcription of CmlCS, the key gene for SA biosynthesis. The red light also induced the expression of CmWRKY42 under powdery mildew stress, and this protein directly bound to the CmlCS promoter (Wang et al., Citation2023a).
D. Cucurbita spp
1. Inoculation experiments, screening of response to (specific races) Gc and Px, detection of resistant genotypes, sources of resistance
Jahn et al. (Citation2002) stressed, that although CPM-resistant cultivars of summer and winter squash are appearing in seed catalogues, the history and effort is not so long as for melon. Sources of high-level resistance to CPM have not been identified in the cultivated species, requiring a broadened search for resistance in wild species that are typically only partly compatible with the domesticated species (Jahn et al., Citation2002).
Recent advances in genetics and breeding of C. moschata were reviewed by Kesh and Yadav (Citation2023). They concluded that CPM incited by Px, CDM, crown rot, root rot and fruit rot (Phytophthora capsici) are the major fungal diseases affecting the cultivation and production of C. moschata. Developing resistant cultivars against these diseases is the major objective in their breeding program. CPM is one of the most destructive fungal diseases of C. moschata, most damaging after fruit set (Guo et al., Citation2018).
CPM resistance studies in Cucurbita pepo and C. moschata were limited until the end of 20th century, despite the fact that CPM-tolerant or resistant hybrids began to appear in seed catalogues and included summer squashes (crookneck, vegetable marrow, zucchini), the resistance to CPM was often connected to reduced yield (Jahn et al., Citation2002).
Recent results suggest that gene flow between the subspecies of C. pepo and their cultigens, incomplete lineage sorting and the retention of ancestral characters shaped the evolutionary trajectory of C. pepo in its area of origin and diversification. This material is an important source of genetic diversity (Martínez-González et al., Citation2021), including resistance against various diseases (Paris, Citation2016, 2017).
Early reports (Whitaker, Citation1956; Rhodes, Citation1964; Sitterly, Citation1972) mention a source of resistance to CPM in wild Cucurbita lundelliana. Whitaker (Citation1956) described PM tolerance in C. lundelliana, whereas Rhodes (Citation1964) described the reaction as resistance to Erysiphe cichoracearum controlled by single dominant gene. This gene was transferred to C. moschata (Rhodes, Citation1964), and C. pepo (Rhodes, Citation1959). Sitterly (Citation1972) transferred hybrid material developed by Rhodes to bush forms of C. pepo, but the effort did not culminate in the release of CPM-resistant cultivars, as the level of resistance was not sufficient when transferred into cultivated types, and there was very likely some undesirable linkage drag (Jahn et al., Citation2002).
Whitaker and Munger in the early 1970s sought to exploit CPM resistance in Cucurbita okeechobeensis ssp. martinezii (Com) (Jahn et al., Citation2002), which resulted in production of several viable seeds from the cross of C. moschata cv. Butternut × Com. Two F1 Butternut × Com plants are the progenitors of all commercial resistance used in C. pepo and C. moschata through the end of 20th century. This resistance is incompletely dominant and may be controlled by more than one gene (Jahn et al., Citation2002).
Holdsworth et al. (Citation2016) mapped Com-derived single nucleotide polymorphism (SNP) alleles in a set of taxonomically and morphologically diverse and resistant C. pepo and C. moschata cultivars bred at Cornell University that, by common possession of Pm-0, form a shared-trait introgression panel. A single 516.4 kb Com-introgression was present in all of the resistant cultivars and absent in a diverse set of heirlooms that predated the Pm-0 introgression. The interval was refined to a final candidate interval of 76.4 kb and CAPS markers were developed inside this interval to facilitate marker-assisted selection (MAS).
Cohen et al. (Citation2003) utilized a PM-resistant C. pepo straightneck-type squash, presumably derived from Com in a backcross breeding program with C. pepo open-pollinated cv. True French. CPM species Px race 5 was specified according to Pitrat et al. (Citation1998) based on interactions with the set of melon CPM differential genotypes. They concluded that resistance was conferred by the single incompletely dominant gene, designated Pm-0. They stressed that race-specific resistance to CPM had not, to their knowledge, been previously reported in squash, perhaps due to the recent commercial deployment of resistance and the less extensive culture of squashes relative to melons.
Within C. moschata, two CPM resistance genes were designated by Adeniji and Coyne (Citation1983). The gene pm-1L was derived from La Primera and was found to be recessive to the pm-1P allele for susceptibility in Ponca. The pm-1L allele was dominant to the pm-1W allele for susceptibility in Waltham Butternut. The other gene, designated pm-2S, was derived from the tropical pumpkin C. moschata Seminole, conferred low-to-moderate resistance, and was recessive to susceptibility.
2. Reports on resistant cultivars and resistance sources used in the breeding
Jahn et al. (Citation2002) summarized reports on additional potential sources of resistance through the end of the 20th century. Keinath and DuBose (Citation2000) reported various responses to CPM among C. pepo and C. maxima cultivars and breeding material in two years of field observations. Cucurbita maxima cultivars Mammoth Gold, Big Max, Rouge Vif d´ Etamps, and Lumina, and C. pepo cultigens HMX 66886, HMX6688, and Magic Lantern had lower CPM severity ratings than the other 19 cultigens tested. The authors stated the CPM species present was Px without specifying means for such determination.
The responses of large sets of Cucurbita spp. were assessed visually in the field experiments under natural infection of both CPM species. The degree of susceptibility to Go varied within Cucurbita species (Lebeda and Křístková, Citation1994), and C. pepo cultivars (Lebeda and Křístková, Citation1996a; Citation1996b). Křístková and Lebeda (Citation1999c) proved on the set of C. pepo the phenomenon of adult susceptibility to Go, and the role of disease assessment of various parts of plants, i.e., petioles, leaf blades (Křístková and Lebeda, 2000a). Complex resistance study (viruses/CMV, WMV-2 and ZYMV/and CPM resistance) showed that C. maxima can be considered more resistant to CMV, WMW-2 and CPM compared with C. pepo responses to these pathogens (Křístková and Lebeda, Citation1999d).
Cohen et al. (Citation1993) proved by in vitro test with leaf disks of seven edible cultivar groups of C. pepo inoculated with Px, that cultivars of cocozelle, and vegetable marrow were the most susceptible, while relatively resistant cultivars were found in the scallop and straightneck groups. Large differences in responses to CPM were observed among C. pepo morphotypes, and groups of cultivars (Halliwell et al., Citation1986; Cohen et al., Citation1993; Lebeda and Křístková, Citation1996a; Citation1996b; Citation2000). Many authors pointed out at clear relation between plant response to CPM and plant age (developmental stage), as well as influences of environmental factors, e.g., shading (Palti, Citation1961; Schnathorst, Citation1965; Aust and Hoyningen-Huene, Citation1986; Leibovich et al., Citation1996).
Leibovich et al. (Citation1996) hastened the appearance of CPM and increased the severity of infection by shading partially resistant and susceptible squash plants, thus facilitating identification of resistant individuals.
Caligiore-Gei et al. (Citation2022) studied the response of five C. moschata accessions, including commercial cultivars and breeding lines, to natural infection by Px in a one-year field trial in which the advanced breeding line BL717/1 showed promise as a source of CPM resistance. Infante-Casella et al. (Citation2007; Citation2008) evaluated zucchini and yellow summer squash breeding lines and cultivars, and pumpkins for CPM tolerance.
Generally, isolates of Go were less aggressive on C. maxima than Px isolates on ad- and abaxial leaf surfaces, though infection by both CPM species was more severe on adaxial surfaces vs. abaxial surfaces (Křístková and Lebeda, Citation2001).
Cho et al. (Citation2005) evaluated 104 Cucurbita spp. accessions in order to identify and develop CPM-resistant genotypes under field conditions, of which C. okeechobeensis ssp. martinezii was identified as a CPM resistance source. Interspecific hybridization was then used to transfer the resistance from C. okeechobeensis subsp. martinezii to C. moschata cv. Jaechenjarae.
3. Detection of resistance genes, inheritance studies, applications in breeding
Pitrat and de Vaulx (Citation1979) reported resistance to CPM and two viruses (cucumber mosaic virus, watermelon mosaic virus) in C. lundelliana, C. martinezii, and C. okeechobeensis. Combined CPM and virus resistances was one of the breeding aims in C. pepo (Jahn et al., Citation2002).
Paris and Cohen (Citation2002) addressed the performance of the incompletely dominant CPM resistance derived from C. okeechobeensis in F1 hybrids whereby one parent was CPM-resistant, i.e., heterozygous CPM-resistant. They reported that yields (no. fruit per 10 m2, no. marketable fruit per 10 m2 and marketable yield–kg/10 m2) of three such CPM-resistant zucchini, cocozelle, vegetable marrow hybrids were significantly higher than their respective unrelated, susceptible counterparts. Interestingly, the hybrid pairs did not significantly differ for percent marketable fruit or weight per fruit. Pairwise comparisons of CPM-resistant and susceptible hybrids were insignificant in an in vitro test of excised leaf disks, though mean CPM incidence on the three CPM-resistant hybrids was significanly (P = 0.01) lower than on the three susceptible hybrids. Resistance was derived from CPM-resistant straighneck squash (C. pepo) that originated, presumably, from the crosses with C. okeechobeensis. They further noted that another source of resistance to the CPM, C. moschata PI 369364, occurred earlier in the parentage of the resistant hybrids Hybrid 629 and Hybrid 653.
CPM resistance is one of many horticulturally important traits introgressed to the cultivated C. pepo in Israel (Paris et al., Citation2006). Zucchini and cocozelle squash cultivars were developed with combined resistances to CPM and zucchini yellow mosaic virus (ZYMV). Vegetable marrow squash with combined CPM and ZYMV resistances were under development by these authors along with similarly resistant acorn squash that also combined black fruit exterior color and fruit flesh soluble solids ranging up to 17%.
Zhang et al. (Citation2016) developed four new cultivars of C. pepo ball type summer squashes, namely One BallPlus, Six Ball, Eight BallPlus, and Cue BallPlus that combined all of the desirable characteristics of the existing ball squashes with CPM (species not specified) resistance, and one or two other important traits such as ZYMV resistance, watermelon mosaic virus (WMV) resistance. Virus resistances were tested by inoculating the seedlings at the cotyledon stage in greenhouses and CPM resistance was evaluated after midseason when CPM occurred in greenhouse and field experiments in two years.
CPM-resistant squash (C. moschata Duch.) Kwangmyeong was bred from a cross between CPM-resistant parents Sigol and inbred line PRS 1031 at National Horticultural Research Institute (NHRI), South Korea. Kwangmyeong was vigorous and highly tolerant to CPM (Cho et al., Citation2009).
The NHRI PM (likely Px—see Cho et al., Citation2003; Citation2005) resistance breeding program was based on the interspecific cross of resistant C. martinezii with local C. moschata cv. Jecheonjaerae followed by backrosses and selfing; lines selected in 1997 were named Mansu and Chensu (Cho et al., Citation2004a; Citation2004b). Their PM resistance is slightly lower than the C. martinezii but their fruit quality is similar to Jecheonjaerae. A single dominant gene determined CPM resistance at the seedling stage in material from a cross of CPM-resistant C. martinezii with susceptible C. moschata cv. Vonye No. 402 (Cho et al., Citation2005).
LaPlant et al. (Citation2016) identified under field conditions of natural infection of CPM (species not specified) in the U.S.A. (Freeville, NY) several CPM-resistant inbred lines of pumpkin (C. pepo). Development of these PMR pumpkin lines began in the mid-1980s. The immediate source of their resistance was PMR Bush Ebony, an acorn squash with CPM resistance derived from an introgression with C. okeechobeensis subsp. martinezii (LaPlant et al., Citation2016).
LaPlant et al. (Citation2016) provided pedigrees of pumpkin (C. pepo) lines developed for resistance to CPM. A delicata squash (C. pepo) was first crossed with PMR Bush Ebony in 1986. The resulting hybrid was crossed and then backcrossed to Spirit, a small jack-o-lantern pumpkin. Selection for CPM resistance occurred in every subsequent generation, beginning in 1990 after the first cross to Spirit. The resulting progeny from the backcross were crossed with Howden, a large jack-o’-lantern pumpkin, and the progeny of this cross became the parents of all the PMR pumpkin lines. The PMR pumpkin lines express substantial resistance to CPM in all of the evaluated portions of the plant. The lines with the highest levels of resistance overall were: NY14-555, NY14-556, NY14- 557, NY14-558, NY15-560, NY14-564, NY14-567, and NY14-568.
Infante-Casella et al. (Citation2008) organized a low fungicide input field experiment (in 2007) in order to indentify C. pepo cultivars tolerant to Px. Cultivars Gladiator, Super Hero and Charisma were described as “tolerant” to CPM, but authors did not provide supporting data to demonstrate 100% yield (compared with disease-free situation) while exhibiting infection levels comparable to susceptible genotypes.
A set of 228 accessions representing pumpkin species C. pepo, C. maxima, C. moschata and C. argyrosperma was screened for resistance to Px at cotyledon, and first and second true leaf stages in a plastic house (Luitel et al., Citation2016). Based on responses at the 2-leaf stage lines IT 110859 (C. pepo) and IT 278459 (C. argyrosperma) were resistant. Twenty-one acccessions representing all four pumpkin species exhibited intermediate CPM resistance at the 2-true-leaf stage and may be used by growers for rational CPM management: C. pepo (2), C. maxima (5), C. moschata (13), and C. argyrosperma (1).
A set of 31 C. pepo cultivars represented by different morphotypes was evaluated for field resistance to natural CPM (E. cichoracearum and S. fuliginea) infection (Lebeda et al., Citation1999). Significant differences in field resistance were found between morphotypes. Scallop and acorn cultivars were the most resistant. Straightneck, crookneck and ornamental gourd groups showed medium levels of resistance. The most susceptible were zucchini, cocozelle, pumpkin and vegetable marrow groups. Isozyme spectra of peroxidases for 11 selected C. pepo cultivars representing different morphotypes with various levels of field resistance were analyzed. They fell into three groups which corresponded with the their respective level of field resistance and thus peroxidase assays have potential for marker assisted selection.
Cucurbita moschata Inbred 112-2 was highly resistant to Px through eight years of observations Zhou et al. (Citation2010).
Four CPM resistance genes () were listed in the 2005 gene list for Cucurbita species (Paris and Brown, Citation2005): Pm, pm-1 and pm-2 were first reported effective against Gc, while Pm-0 provided resistance to Px. Recent Cucurbita gene list updates (Paris and Kabelka, Citation2009; Paris and Padley, 2014–2015) attribute actions of Pm and Pm-0 to Px, while pm-1 and pm-2 remain specified as active against Gc though it is possible they are effective against Px rather than Gc (see McCreight, Citation2004).
Table 9. Cucurbita spp. genes for cucurbit powdery mildew (CPM) resistance (Paris and Brown, Citation2005; Paris and Kabelka, Citation2009; Paris and Padley, Citation2014-2015).
4. Genetic (molecular) mapping
Montero-Pau et al. (Citation2017) reported, that C. pepo inbred line MU-CU-16 (zucchini morphotype) was chosen to represent the whole genome sequence. Several transcriptomes of C. pepo were produced, and some genes annotated in these transcriptomes have been functionally characterized (Martínez et al., Citation2013; Citation2014). Montero-Pau et al. (Citation2017) reported, that tools for functional characterization were at that time being developed, such as high throughput reverse genetic tools. The first TILLING (Targeted Induced Local Lesion IN Genomes) resource for this species has been produced (Vicente-Dólera et al., Citation2014). Montero-Pau et al. (Citation2017) report, that major QTL were found for growth habit, leaf morphology, early flowering, fruit size and shape and flesh color. They did not mention disease resistance.
The first report whole-genome sequencing of C. maxima and C. moschata provided molecular evidence of allotetraploidization event in Cucurbita (Sun et al., Citation2017).
Formisano et al. (Citation2010) proved by AFLP analyses that commercial C. pepo squash hybrids carrying disease resistances (CPM and viruses) introgressed from C. moschata have high genetic similarity. The ultimate source of CPM resistance was C. okeechobeensis (Small) Bailey, using C. moschata as a genetic bridge (Jahn et al., Citation2002). Formisano et al. (Citation2010) concluded that the resistances are inherited monogenically and oligogenically.
QTL mapping and molecular markers for CPM resistance in pumpkin (C. moschata) was done using F2 individuals from a cross between TG201 (susceptible female parent) and TG10 (resistant, male parent) heirloom from Hongikbio Inc., Korea (Park et al., Citation2020). The single major QTL was located in 6.9–7.3 Mb region of TG10 chromosome 3, and furthermore seven sets of indel (insertion and deletion variation between TG201 and TG10 genome) PCR markers were designated. From genomic sequence comparison on the basis of breeding history, Park et al. (Citation2020) confirmed that the CPM resistance region was introduced from C. okeechobeensis subsp. martinezii.
Resistance is controlled by single dominant gene of C. lundelliana when crossed with C. moschata (Rhodes, Citation1964). Genotypes developed with interspecific introgression have not been commercialized due to linkage drag and moderate resistance in the genetic background of cultivars (Jahn et al., Citation2002). Resistance within C. moschata is reported to be oligogenic, i.e., pm-1 L and pm-2S. The pm locus had three alleles of which pm-1 L from La-Primera was dominant to pm-1 W from susceptible Waltham Butternut but recessive to pm-1P from highly susceptible Ponca. Another allele, pm-2S from resistant Seminole is recessive to Pm-2 from susceptible Ponca (Paris and Brown, Citation2005). Cho et al. (Citation2003) selected two improved lines namely Sigol and Sangol by backcrossing and selfing of C. moschata cv. Jecheonjaerae with C. okeechobeensis subsp. martinezii for CPM resistance and with C. moschata cv. Seoulmadi for fruit quality traits.
Holdsworth et al. (Citation2016) mapped C. okeechobeensis ssp. martinezii-derived single nucleotide polymorphic alleles in resistant and diverse cultivars of C. moschata and C. pepo using GBS. CAPS markers NBS_S9_1495924 and S9_1539675 co-segregated with the resistant phenotype and were developed for MAS of CPM resistance in C. moschata and C. pepo. Guo et al. (Citation2018) conducted the transcriptome analysis of CPM-treated and untreated C. moschata inbred line 112-2 using RNA sequencing. A complex regulatory network involving hormone signal transduction pathways, transcription factors and defense responses metabolism controlled genes expressing resistance to CPM. Additionally, six differentially expressed genes, bHLH87, HSF, ERF014, MLO3, WRKY21 and SGT1 involved in defense responses were identified. The expression of SGT 1 was upregulated in resistant plants six hours following inoculation with Px. Two of the genes identified and cloned from the transcriptome were named cmSGT1 and cmbHLH87, showing 89% similarity to melon lines SGT 1 and bHLH87. Overexpression of cmSGT1 and cmbHLH87 in transgenic tobacco improved CPM resistance by enhancing hypersensitive response, H2O2 accumulation and upregulated expression of salicylic acid-dependent defense genes (Guo et al., Citation2019; Citation2020).
5. MLO gene family
The Mildew resistance locus o (Mlo) is a plant-specific gene family that encodes a protein with seven-transmembrane (TM) domains that play an important role in CPM resistance. Iovieno et al. (Citation2015) exploited available genomic information to provide a comprehensive overview of the MLO gene family in Cucurbitaceae, and identified 16 MLO homologs in C. melo, 14 in C. lanatus and 18 in C. pepo genomes. They identified a series of conserved amino acid residues and motifs that are likely to play a major role for the function of MLO proteins. Their data here could be conveniently used for breeding and research of CPM-resistant cucurbit cultivars.
Porterfield and Meru (Citation2017) identified the MLO approach for development of durable and broad- spectrum resistance to CPM in watermelon and squash (C. pepo, C. maxima and C. moschata) and identified candidate susceptibility genes for CPM and CDM. Four MLO homologs in C. maxima and five in C. moschata clustered within Clade V, a clade containing all MLO susceptibility genes in dicots, and had highly conserved transmembrane domains and C-terminal CPM interaction motif. Sixty-three candidate PMR genes were identified among the four species, 16 of which had close similarity to functionally proven PMR homologs in model species. In C. maxima and C. moschata, candidate susceptibility genes were found in all chromosomes except chromosomes 9, 10, 11 and 12. For the two species, most candidate homologs were located at similar positions on respective chromosomes, which suggested high levels of synteny between these two species.
The identification and description of MLO family genes in pumpkin (C. maxima) was reported by Win et al. (Citation2018). Twenty genes designated CmaMLO1 to CmaMLO20 with important roles in resistance to Px have been identified using an in silico cloning method with the MLO gene sequences of cucumber, melon watermelon, and C. pepo as probes. These CmaMLOs were evenly distributed on 15 of the 20 C. maxima chromosomes without any obvious clustering.
Win et al. (Citation2018) comprehensively reported on MLO genes on C. maxima. Expression analysis showed that four of the five CmaMLO genes were upregulated in Px-infected pumpkins. Phylogenetic and expression analysis provided preliminary evidence that these five genes could be susceptibility genes important for CPM resistance.
Zhu et al. (Citation2021) analyzed 20, 21, and 18 candidate MLO genes in C. moschata, C. maxima and C. pepo, respectively, designated as CmoMLO, CmaMLO and CpeMLO, respectively. Expression analysis in the susceptibility (S) and resistance (R) lines showed that CpeMLO1, CpeMLO2 and CpeMLO5 might be involved in the susceptibility response. CpeMLO4 and CpeMLO6 exhibited opposite expression patterns in the R/S lines and might be involved in the resistance response. All these data would be beneficial for future functional analysis of MLOs in Cucurbita species.
6. Long noncoding RNAs, GWAS, VQ gene family
Mechanisms of plant defense against CPM have been previously reported (Ellinger et al., Citation2013; Hu et al., Citation2019). Additionally, several PM resistance-related genes have been identified in wheat (Qie et al., Citation2019), cucumber (Liu et al., Citation2017; Xu et al., Citation2017; Citation2019), and melon (Romero et al., Citation2008). In pumpkin (C. moschata), WRKY21, MLO3, and SGT1 were identified as candidate genes conferring PM resistance (Guo et al., Citation2018). Moreover, the overexpression of CmSGT1 (Guo et al., Citation2019) and CmbHLH87 (Guo et al., Citation2020) can improve CPM resistance in tobacco. Although these studies increase our understanding of the mechanisms of plant resistance to CPM, the molecular mechanisms underlying CPM resistance in C. pepo remain largely unknown.
Long noncoding RNAs (lncRNAs) play an important role in regulating plant responses to biotic stresses, including PM of wheat (Xin et al. Citation2011; Zhang et al. Citation2016). Tian et al. (Citation2022) systematically identified 2,363 reliably expressed lncRNAs from the leaves of CPM-susceptible (PS) and CPM-resistant (PR) C. pepo. Among the 2,363 lncRNAs, 113 and 146 CPM-responsive were identified in PS and PR, respectively. Six PM-responsive lncRNAs were predicted as potential precursors of microRNAs (miRNAs). In addition, 58 CPM-responsive lncRNAs were predicted as targets of miRNAs and one CPM-responsive lncRNA was predicted as an endogenous target mimic (eTM). Furthermore, 5,200 potential cis target genes and 5,625 potential trans target genes were predicted for CPM-responsive lncRNAs. Functional enrichment analysis showed that these potential target genes are involved in different biological processes, such as the plant-pathogen interaction pathway, MAPK signaling pathway, and plant hormone signal transduction pathway. Their research laid a foundation for further research on the regulatory mechansims of lncRNAs in response to CPM infection.
Alavilli et al. (Citation2022) identified a novel gene that involves Px resistance in pumpkins (C. moschata) through a genome-wide association study (GWAS). The allelic variation identified in the CmoCh3G009850 gene encoding for AP2-like ethylene-responsive transcription factor (CmoAP2/ERF) was proven to be involved in CPM resistance. The qRT-PCR assay validated the elevated expression of CmoAP2/ERF during CPM infection in PMR compared with PMS. Wang et al. (Citation2021b) reported, that, based on the strategy of QTL-seq combined with linkage analysis and molecular markers, the major locus CpPM10.1 was defined as a region of approximately 20.9 kb that contained five coding genes.
Xu and Wang (Citation2022) identified 44 VQ genes in C. pepo, which were differentially regulated under a variety of abiotic stresses and CPM, indicating that they play an important role in plant stress response and other aspects.
7. Physiological and structural background of resistance to PM
Evaluation of 248 C. maxima and C. moschata lines for the resistance to CPM performed by inoculation with infected (CPM species not specified) pumpkin leaves in a greenhouse led to the detection of three resistant C. maxima accessions and one C. moschata accession. The resistance levels against CPM were positively correlated with the thickness of epidermal cell wall and cuticle layer, which may play a role to prevent CPM infection (Kim et al., Citation2014).
Chen et al. (Citation2020) studied the enzymatic activities of CPM-resistant and susceptible C. moschata genotypes. They found out that CPM resistance in pumpkin seedlings is associated with the maintenance of photosynthetic performance and the regulation of defense-related enzyme activities. Increased peroxidase (POD) and phenylalanine ammonia lyase (PAL) activities are probably vital for the pumpkin seedlings to restrict CPM infection and systemic disease establishment.
Margaritopoulou et al. (Citation2022) analyzed the response of three courgette (C. pepo L. var. cylindrica) cultivars with different levels of genetic resistance to Px using cytological, molecular, and biochemical approaches. Results showed that the plants were under a primed state and induced systemic acquired resistance (SAR) responses, exhibiting enhanced callose production, upregulation of salicylic acid (SA) defense signaling pathway genes, and accumulation of SA and defense metabolites. Additionally, the intermediate resistant varieties showed an altered epigenetic landscape in histone marks that affect transcriptional activation.
Zhang et al. (Citation2021c) investigated the responses of two commercial pumpkin (C. pepo) cultivars to Px infection. Their results suggest that one possible mechanism of C. pepo to prevent Px infection is activation, enhanced activity and gene expression of the phenylpropanoids pathway to synthesize phenolic substances and lignin, ROS scavenging defense enzymes to eliminate the harmful effects of ROS, and signaling pathway marker gene expression to improve plant disease resistance.
Reyad et al. (Citation2022) examined the effects of Trichoderma asperellum (MW965676), Streptomyces rochei (MN700192), and a mixture of the two applied as foliar sprays with or without shikimic acid seed priming treatment on CPM severity on C. pepo with respect to the morphological changes induced by CPM and the treatments. Plants treated with a mixture of T. asperellum, S. rochei and shikimic acid exhibited increased thickness of the midvein and dimensions of the main midvein bundle, a larger number of xylem rows in the main midvein bundle, and greater mean diameters of vessels and of parenchyma cells in the ground tissues, as well as increased thickness of the upper and lower epidermis, lamina, palisade tissue and spongy tissue. This study is the first step toward a more profound understanding of the use of T. asperellum and S. rochei with shikimic acid-primed seeds as a potential means for attenuating CPM infection in squash.
Jorkesh et al. (Citation2018) evaluated C. moschata and C. pepo lines for CPM resistance for their use in grafting experiments. Out of ten lines, they found one line, G7 as highly resistant based on disease severity and combining ability with other lines. Keinath et al. (Citation2010) reported the potential of hybrid Cucurbita seedlings used as rootstocks for growing of cucurbits free of or less infected by CPM. The physiological and biological background of this phenomenon is remains to be determined.
8. Patents
Some Cucurbita spp. genotypes were patented as a valuable sources of CPM resistance. For example, inbred C. pepo pumpkin HSPMR7B1 and ATTC C. pepo accession PTA-11250 with the mutant allele Pm2 were patented by Hollar Seeds in the U.S.A. (Zhang, Citation2013a; Citation2013b). PTA-11250 is useful for F1 hybrid pumpkin development.
The C. pepo variety CTC12873BP with declared resistances to CPM, ZYMV and WMV was patented by Suh and Cho (Citation2017). C. pepo hybrid cv. HSMPR7B1 with the mutant allele Pm2 (ATCC PTA-11250), was patented by Zhang (Citation2011) for producing plants with improved desired traits, e.g., disease resistance, herbicide resistance, and insect or pest resistance.
Dyche and Kuijpers (Citation2013) patented a plant of C. maxima and C. moschata useful for producing plants with resistance to CPM.
New CPM resistance in C. maxima plant reported by Atarashi et al. (Citation2018) was patented by the company Takii Shubyo KK and Takii & Co ltd., has CPM (species not specified) resistance locus on chromosome 3 comprising specific a polynucleotide. This CPM resistance of C. maxima plant having CPM resistance locus on chromosome 3, is new (Atarashi et al., Citation2018). Atarashi et al. (Citation2019) patented a new marker for CPM resistance on C. maxima chromosome 3.
E. Watermelon (Citrullus lanatus)
1. Introduction
Taxonomic studies distinguished two botanical varieties within C. lanatus: subsp. vulgaris (Schrad. ex Eckl. et Zeyh.) var. lanatus (watermelon) and subsp. lanatus var. citroides (L.H. Bailey) Mansf. ex Greb.) (citron watermelon) (Laghetti and Hammer, Citation2007; Chomicki and Renner, Citation2015). Modern watermelon breeding has led to the development of predominantly red sweet cultivars, that have a narrow genetic diversity and generally low levels of resistance to biotic and abiotic stresses (Levi et al., Citation2017a; Citation2017b; Grumet et al., Citation2021).
Citrullus lanatus subsp. lanatus var. citroides (citron watermelon) exhibits wider genetic variation than watermelon and may be a source of valuable genes (Ngwepe et al., Citation2019). Watermelon and citron watermelon are genetically compatible (Levi et al., Citation2000; Citation2001a,Citationb; Citation2017a) and the two botanic varieties can be readily crossed (Wechter et al., Citation2012). Citron is, thus, an important genetic resource for watermelon breeding (Norton et al., Citation1995), though its genetic utility for watermelon breeding has received attention only recently (Ngwepe et al., Citation2019). Laghetti and Hammer (Citation2007) considered the Corsican citron as a potential valuable souŕce of resistance to various fungal pathogens. Mashilo et al. (Citation2022) explained and stressed the importance of citron genetic resources for watermelon breeding. Citrullus genetic resources from a general viewpoint contain various genes conferring resistance against many diseases and pests, including CPM (Levi et al., Citation2017a).
Watermelon was widely considered resistant to CPM during the 20th century (Sitterly, Citation1978; Jahn et al., Citation2002), and susceptibility was a rarity, when accession PI 269677 was identified as susceptible to Px among 590 accessions (Robinson et al., Citation1975). PM resistance was attributed to a single dominant gene, Pm (Guner and Wehner, Citation2003).
Accessions of C. lanatus, C. mucosospermus and C. amarus have been valuable sources of resistance to CPM (Px), now considered a major disease of watermelon (Keinath et al., Citation2017; Levi et al., Citation2017a). Sources of resistance, mostly (race-specific, against melon CPM races 1 and 2 W) (see McCreight, Citation2006) have been identified in Citrullus accessions PI 189225, PI 482326, PI 482328, PI 494531, PI 532738 and the mode of inheritance has been determined in some watermelon germplasm. C. lanatus and C. mucosospermus lines with multiple disease resistance (CPM and Phytophthora fruit rot) were also identified and developed (Levi et al., Citation2017a). Most watermelon cultivars are not resistant to melon race 1 W, which has been shown to cause severe damage in nine states across the U.S.A. (Davis et al., Citation2007; Tetteh et al., Citation2010).
2. Inoculation experiments, screening of response to (specific races) Gc and Px, detection of resistant genotypes, sources of resistance
Inoculation experiments and experimental studies focused on interactions between Citrullus spp. and CPM yielded data that mostly support race-specific host-pathogen interaction (see below), although there is some information on field resistance in watermelon (Jahn et al., Citation2002)
Cohen and Eyal (Citation1988) used Px from C. melo and Go from tobacco in controlled inoculation tests, and concluded that all eight tested watermelon cultivars were resistant to Px race 1 and susceptible to Px race 2. C. colocynthis was resistant to both races of Px.
The tobacco isolate of Go was moderately infective to C. lanatus and C. colocynthis (Cohen and Eyal, Citation1988). In contrast to the Israeli results, C. lanatus cv. Sugar Baby proved susceptible to isolates of Go collected from C. pepo and C. maxima in 1998 in the Czech Republic (Křístková and Lebeda, Citation2000b).
Since the beginning of the 21st century, USDA and university researchers have assayed the responses of USDA NPGS acccessions of Citrullus spp. and closely related species, and watermelon cultigens to Px races 1 (Thomas et al., Citation2005; Davis et al., Citation2006; Citation2007) and 2 (Tetteh et al., Citation2010). None of the 266 watermelon accessions tested by Thomas et al. (Citation2005) for resistance to Px race 2 (artificial inoculation in growth room) was highly resistant.
Watermelon breeding line PI 525088-PMR with resistance to Px race 1 was identified, and additional experiments proved its resistance also to Px races 2, 3, and 5 from France using a leaf disk assay (Davis et al., Citation2006).
Davis et al. (Citation2007) evaluated the entire available USDA NPGS collection of Citrullus spp. for resistance to Px race 1 W. The collection consisted of watermelon and citron accessions as well as the wild-type C. colocynthis (L.) Schrad., C. rehmii De Winter, and Praecitrullus fistulosus (Stocks) Pangalo. Wild-type accessions tended to be more resistant, and more often than watermelon accessions. None were resistant (immune), eight of the 1573 accessions exhibited high levels of resistance, and another 86 demonstrated intermediate resistance. Stem and leaf disease severity were weakly correlated (r2 = 0.64, P = 0.001). The majority of accessions having resistance were collected in Zimbabwe. Resistance was found in all four species, though C. rehmii expressed intermediate resistance. Unfortunately, PI 525088-PMR (Davis et al., Citation2006) with resistance to Px race 1 W was not included in this assay for comparison.
Tetteh et al. (Citation2010) screened 1654 watermelon accessions, cultivars and breeding lines, citron, C. colocynthis, and P. fistulosus for their responses to artificial inoculuation with Px race 2 W in greenhouse and field conditions. Resistance was identified in the wild PI accessions. All cultigens showed symptoms of CPM. The most resistant cultigens were PI 632755, PI 386015, PI 189225, PI 346082, PI 525082, PI 432337, PI 386024, and PI 269365. Among them, accessions PI 386015 (C. colocynthis from Iran), PI 525082 (C. colocynthis from Egypt), and PI 270545 (watermelon from Sudan) were resistant to Px race 1 W (Davis et al., Citation2007). The most susceptible cultigens were PI 222775 and PI 269677. Many of the resistant cultigens originated from Nigeria and Zimbabwe. These accessions expressed yellow spots on leaves without developed mycelium; these symptoms were considered hypersensitive-type resistant reactions. The authors suggested that although the majority of the accessions tested were heterogeneous for CPM reaction, they may carry useful resistance for breeding purposes. Inbred lines from promising cultigens can be used to determine inheritance of resistance and to develop resistant cultivars (Tetteh et al., Citation2010).
Kousik et al. (Citation2018c) developed through at least eight years of evaluation and selection four watermelon lines that exhibited high levels of resistance to CPM incited by locally prevailing Px (race identity not reported), namely lines USVL608-PMR, USVL255-PMR, USVL313-PMR, and USVL585-PMR. They were derived from PI 307608, PI 482255, PI 482313, and PI 505585, respectively; these accessions were selected on the basis of the previous research of Davis et al. (Citation2007) and Tetteh et al. (Citation2010). The PMR lines were developed by selection of individual resistant plants within each accession, as these accessions were heterogeneous in response to Px infection. Specifically, the hypocotyls, cotyledons, and true leaves of these four PMR lines are highly resistant to CPM compared with the CPM-susceptible watermelon line USVL677-PMS or cv. Mickey Lee on which severe CPM and abundant development of conidia were observed. The true leaves of these four PMR lines were also resistant to Px isolates (race identities not reported) from California, Florida, Georgia, New York, and South Carolina. Each PMR line is uniform for various growth characteristics demanded for commercial marketability. These lines could be used in breeding programs of watermelon. Based on preliminary experiments, resistance in USVL608-PMR is dominant, and this line would be an appropriate candidate to transfer resistance into commercial cultivars (Kousik et al., Citation2018c).
Interestingly, Ben-Naim and Cohen (Citation2015) reported PI 307608, from which USVL608-PMR was developed, susceptible to CPM in Israel. Tetteh et al. (Citation2010) found PI 307608 resistant compared with the susceptible check, PI 269677, and other commercial varieties in North Carolina. PI 307608 was heterogeneous for resistance in the initial trials, they selected and developed a uniformly resistant source through six generations of phenotyping and selection (Kousik et al., Citation2018c). Further work was advised to evaluate these PMR lines in other countries and states within the U.S.A. to more thoroughly characterize this source of resistance. These findings are consistent with occurrence of numerous physiological races of CPM and their spatio-temporal fluctuations (Lebeda et al., Citation2018a; Citation2021a).
The CPM pathogen, Px, has been known to evolve rapidly and develop resistance to fungicides (Lebeda et al., Citation2010). In addition, there exists the potential for Px races based on interactions with watermelon (Kousik et al., Citation2014). Therefore, even when commercial watermelon cultivars with resistance to CPM are developed from these or other germplasm sources, it will be prudent to use an integrated disease management strategy, including the use of fungicides to manage CPM.
A 2014–2016 study assessed relative susceptibilities of 26 watermelon varieties (seeded and seedless) and three pollenizers to Px race 1 W populations prevailing in Charleston, South Carolina (Kousik et al., Citation2019). During all three years, USVL677-PMS was the most susceptible; in comparison USVL531-MDR was very resistant, and the commercial pollenizers, SP5, SP6 and Lion were all resistant to CPM. Among the red fleshed cultivars, Suprema (seedless), was relatively resistant. Most of the seeded cultivars evaluated (e.g., Malali, Black Mama, Mickey Lee) were highly susceptible to CPM, though some were relatively less susceptible (e.g., Declaration) under field conditions. The authors noted that very few to no cultivars with high levels of CPM resistance were available at the time of the tests, and emphasized the need to develop modern CPM-resistant cultivars as CPM frequency was increasing in commercial production fields.
The commercial cultivars were evaluated under natural conditions in the field where race 1 (also known as race 1 W) was the most prevalent CPM race based on melon differentials that were planted in adjacent rows in the same field in all three years (Kousik et al., Citation2019). It will be interesting to determine how these diploid and triploid watermelon cultivars perform against other CPM races in other locations. Line USVL531-MDR was found in previous laboratory assays resistant to 11 CPM isolates collected from different parts of the U.S.A. (Kousik et al., Citation2014).
Yadav et al. (Citation2021a) screened 107 germplasm and advanced watermelon breeding lines from the watermelon repository of Northwest A & F University, Yangling, Shaanxi (China), against Px race 2 F in plastic house tests. Melon differential lines were utilized to confirm identity of the CPM race in Spring and Autumn growing seasons. Some highly resistant cultigens including M16, M11 and M49 with mean disease severity ratings of 0.58, 1.0 and 1.13, respectively were identified, and can be utilized for resistance mechanism studies and resistance breeding. Ngwepe et al. (Citation2019) reviewed known sources of resistance to CPM among citron accessions.
3. Detection of resistance genes, inheritance studies, applications in breeding
Tetteh et al. (Citation2013a; Citation2013b) analyzed inheritance of resistance to Px race 2 W-US in two watermelon accessions. The cross of resistant PI 270545 with susceptible PI 267677 revealed two genes, a recessive resistance gene, pmr-1, and a dominant gene for moderate resistance, Pmr-2 (Tetteh et al., Citation2013a). Narrow sense (h2) heritabilities for PM resistance in this study were 71% and 79% for leaf and stem reactions, respectively. QTL mapping analysis may contribute to the detection of epistatic effects in this population.
Resistant PI 189225 was crossed with moderately susceptible Charleston Gray and highly susceptible PI 269677 (Tetteh et al., Citation2013b). Segregation patterns for leaf reaction in the Charleston Gray × PI 189225 population fit a single gene inheritance model. Generation means analysis established only additive gene action for leaf resistance, while additive, dominance, and epistatic effects were important for stem resistance; narrow sense heritabilities for leaf and stem reactions were 0.58 and 0.81, respectively. In the PI 269677 × PI 189225 population, epistatic effects combined with low narrow sense heritability (h2) estimates of 0.20 and 0.00 for leaf and stem reactions, respectively, and presence of duplicate epistasis may result in slower progress from selection.
Ben-Naim and Cohen (Citation2015) assayed 291 accessions of Citrullus spp. (var. lanatus, var. mucospermus, var. citroides, and C. colocynthis, C. ecirrhosus, C. rehmii) and Praecitrullus fistulosus from U.S.A. and Israel gene banks for resistance to Px race 1 W. Only eight of the accessions exhibited high level resistance on hypocotyl, cotyledons and leaves: citron PI 189225 (Democratic Republic of the Congo), PI 482312 (Zimbabwe) (recently these accessions are classified as Citrullus amarus (USDA-ARS, 2023)), and BIU 119 (unknown origin); P. fistulosus PI 250145 (Pakistan), PI 381748 (India), and PI 381755 (India); C. colocynthis CC-16 (Israel); C. lanatus var. mucospermus L.W.1298 (Africa). Inheritance of resistance against Px race 1 W was studied in crosses of three CPM-resistant citron accessions BIU 119, PI 189225, and PI 482312 with susceptible watermelons Malali and Sugar Baby. Resistance at the cotyledon stage was controlled by a single, partially dominant gene, whereas at the 4-leaf stage or the 15-leaf stage resistance was controlled by three complementary, partially dominant genes. BIU 119 and PI 189225 carry the same genes for resistance, whereas PI 482312 shares two of the three genes in BIU 119 and PI 189225. A breeding line with high level resistance and good fruit qualities was developed from BIU 119 × HA5500 (Ben-Naim and Cohen, Citation2015).
4. Genetic (molecular) mapping
Comparative genomic analysis showed that 11 watermelon chromosomes are derived from a seven-chromosome paleohexaploid eudicot ancestor, and the watermelon genome sequence draft showed that disease resistance genes were lost during domestication (Guo et al., Citation2013; Yong and Guo, Citation2017).
Kim et al. (Citation2013) and Han et al. (Citation2016) conducted bioassays on a set of watermelon CPM differential genotypes, and concluded that the Px race predominat in South Korea was either race 1 W or another race different from race 2 W. Inheritance of resistance to Px race 1 W was studied with different generations derived from a cross between resistant Arka Manik and susceptible inbred HS3355. Resistance to CPM in Arka Manik to this CPM race was conditioned by the single incompletely dominant gene, Pm1.1. BSA (bulked segregant analysis) identified a RAPD marker tightly linked to CPM resistance in Arka Manik. BSA of F2 plants using random amplification of polymorphic DNA (RAPD) identified one polymorphic polymerase chain reaction (PCR) band, OP-R483; close linkage (3.6 cM) was detected between resistance and OP-483. The marker OP-483 was converted to cleaved amplified polymorphic sequence (CAPS-G/C) and a single nucleotide polymorphism (SNP) marker (MCA-A/G) using melting curve analysis. MCA-A/G marker genotyping of a wide range of susceptible breeding lines revealed that the marker allele of Arka Manik was unique in breeding populations (Kim et al., Citation2013).
An intraspecific genetic map for watermelon was constructed using an F2 population derived from Arka Manik × TS34, and transcript sequence variants and quantitative trait loci (QTL) for resistance to Px race 1 W, seed size (SS), and fruit shape (FS) were analyzed (Kim et al., Citation2015b). Inheritance and QTL analyses indicated that each of the traits was controlled by an incompletely dominant effect of two major QTLs designated pmr2.1, ss2.1, and fsi3.1, respectively. A major QTL for PMR in a cultivated, red flesh watermelon was detected in a genomic region carrying a cluster of NBS-LRR genes on Chr02. A major QTL controlling SS was located near the QTL for PMR on Chr02. The results provide useful information for marker-assisted breeding and gene cloning for PMR and fruit-related traits.
5. Physiological background and resistance to CPM
Mandal et al. (Citation2020) used RNA-seq profiling to elucidate innate and activated molecular defense mechanisms involved during compatible and incompatible CPM-watermelon interactions. They inoculated CPM-susceptible USVL677-PMS and resistant USVL531-MDR plants with a Px conidial suspension (105 condia ml−1 in 0.02% Tween 20) with RNA-seq profiling done on leaf samples collected 0, 1, 3, and 8 days post-inoculation (DPI). A total of 2,566 unique differentially expressed genes (DEGs) were identified between compatible and incompatible Px-watermelon interactions. Compatible interactions resulted in distinct plant gene activations compared with incompatible interactions. Based on SNPs identification and CAPS markers, the resistance gene was identified as ClaPMR2, Citrullus lanatus PM Resistance gene 2 {Chr2: 26750001 .. 26753327 (−)}, a NBS-LRR resistance protein (R) with homology to the Arabidopsis thaliana CPM resistance protein, RPW8. In response to Px-watermelon interaction, the expression of resistance proteins TIR-NBS-LRR and CC-NBS-LRR were significantly changed during compatible and incompatible interactions. The transcriptome data also revealed a complex regulatory network associated with the introgressed junctions mediated by CPM resistance R proteins (R genes) that may involve multiple signal regulators and transducers, carbohydrate metabolism, cell wall modifications and the hormone signaling pathway (Mandal et al., Citation2020).
The role of melatonin in resistance responses has recently received attention (Mandal et al., Citation2018). Research on the animal neurohormone, melatonin, has since the 1950s focused on its multiregulatory effect on patients suffering from insomnia, cancer, and Alzheimer’s disease. Melatonin plays major role in plant growth and development, and is induced in responses to diverse biotic and abiotic stresses, but studies on the direct role of melatonin in disease suppression and as a signaling molecule in host-pathogen defense mechanisms are lacking (Gao et al., Citation2023).
Mandal et al. (Citation2018) provided insight on the predicted biosynthetic pathway of melatonin in watermelon and how application of melatonin can boost plant immunity and suppress pathogen growth where fungicide resistance and lack of genetic resistance are major problems. They evaluated the effect of spray-applied melatonin and also transformed watermelon plants with the melatonin biosynthetic gene SNAT (serotonin N-acetyltransferase) to determine the role of melatonin in plant defense. Increased melatonin levels in plants were found to boost resistance against Px, and the soilborne oomycete Phytophthora capsici in watermelon and other cucurbits. Transcriptomic data from melatonin-sprayed (1 mmol/L) watermelon leaves suggested that melatonin alters the expression of genes involved in both PAMP-mediated (pathogen-associated molecular pattern) and ETI-mediated (effector-triggered immunity) defenses. Twenty-seven upregulated genes were associated with constitutive defense as well as initial priming of the melatonin-induced plant resistance response. These results indicate that strategies to increase melatonin levels in specialty crops such as watermelon can lead to resistance against diverse filamentous pathogens (Mandal et al., Citation2018).
Yadav et al. (Citation2021b) studied dirigent (DIR) proteins, which are induced under various stress conditions and involved in sterio- and regio-selective coupling of monolignol. A striking lack of information about DIR genes in cucurbitaceae plants underscores the need for their functional characterization. DIR genes identified in watermelon (22), melon (22) and cucumber (17) were distributed unevenly on different chromosomes (Yadav et al., Citation2021b). Phylogenetic analysis revealed the existence of six subgroups based on domain and structural characteristics. Furthermore, their findings provided information for the evolution and functional divergence of DIR genes in plants, and in addition, information about the involvement of these genes in activities related to phytohormones, stress responses and lignin biosynthesis based on cis-element and GO analysis. The dominant expression of these genes in root tissue provided evidence for their possible involvement in root-related functions. RNA-seq and qRT-PCR analyses indicated that ClDIR and CmDIR could be defense-responsive genes to CPM in different species of cucurbits, while some genes were explicitly upregulated in resistant cultivars. It seems that DIR genes are critically involved in plant defense, probably by their role in secondary cell wall metabolism and production of defense-related compounds. This study lays the foundation for gene function validation studies in cucurbit crops in order to determine the involvement of DIRs in lignin polymerization and possible function in plant–pathogen interactions (Yadav et al., Citation2021b).
Yadav et al. (Citation2022) determined the precise expression changes induced by Px race 2 F during the early stage of infection by the use of differential expression analysis of two contrasting watermelon genotypes, ZXG1755 (resistant) and ZXG1996 (susceptible). Both genotypes showed significant transcriptional regulation in response to Px race 2 F infection. Moreover, this study portrayed strong evidence indicating the important role of a complex network associated with lignin biosynthesis and downstream phytohormone-related mechanisms responsible for incompatible interactions between CPM and watermelon resistant lines. Mandal et al. (Citation2021) identified a PMR marker in watermelon using metabolomic and genomic approaches.
F. Bitter gourd (Momordica charantia)
1. Sources of resistance and powdery mildew races
Commercial cultivars of bitter gourd resistant to Px are generally not available. East-West Seed (EWS) recently released in India the F1 hybrid Pragati that is tolerant to Px. World Vegetable Center (WorldVeg) initiated a bitter gourd CPM-resistance program in Thailand in 2010 using 150 landraces from 13 countries obtained from the WorldVeg genebank. December-January is the best period for screening bitter gourd against CPM in Kamphaeng Saen (Thailand), as this is when CPM epidemics occur in this part of the Thailand and infections are always severe and uniform. Minimum and maximum temperatures during this period in Kamphaeng Saen range from 20 to 22 °C and 26 to 28 °C, respectively, with elevated levels of relative humidity ranging 70–85% in the absence of rainfall–ideal for CPM development (Dhillon, unpublished).
We observed segregation for CPM resistance in these heterogeneous and heterozygous landraces against various local populations of CPM. Resistant plants were hand-pollinated (controlled pollination) and subsequent selections within and among progeny rows led to development of 24 CPM-resistant bitter gourd inbreds, of which 14 originated from landraces collected in India and Bangladesh, two countries considered primary centers of bitter gourd genetic diversity. Horticultural traits including fruit weight, length, diameter, skin color, bitterness and popular Asian market segment of these CPM-resistant lines were evaluated and a database of fruit pictures was created (Dhillon et al., 2023, unpubl.).
Bitter gourd CPM genetic resistance resources originated from their respective primary centers of diversity (Dhillon et al., Citation2016a; Citation2016b). Evaluation of six WorldVeg bitter gourd CPM resistant lines at 12 locations across Asia led to identification of four Px races on bitter gourd in Asia, namely Mc-1, Mc-2, Mc-3, and Mc-4 (Dhillon et al., Citation2018). Pathotype differences between them and six strains of Px isolated from other cucurbits (Cucumis and Cucurbita) in Europe and the Mediterranean area were also established in this study.
2. Genetics of resistance
Resistance to Px race Mc-1 in the five resistant bitter gourd lines (THMC 113, THMC 143, THMC 153, THMC 167, THMC 170) was reported to be controlled by at least two independent, recessive genes (Dhillon et al., Citation2019). Resistance in THMC 143 was nonallelic to Px race Mc-1 resistance exhibited by the other four lines. Resistance to Px race Mc-1 in THMC 153 was intermediate to that exhibited by the other lines. Intermediate resistance to CPM has been exhibited by bitter gourd cvs. Pragati and Rushaan, released by EWS and Nunhems, respectively in India (K.K. Yadav, personal comunication).
G. Ridge gourd (Luffa acutangula)
1. Sources of resistance
One hundred and nine CPM-resistant inbreds representing different market segments were developed through pedigree selection in the WorldVeg CPM hotspot fields in Thailand (Dhillon, N.P.S., unpublished). The lines were derived from heterogeneous and heterozygous ridge gourd landraces collected from 10 Asian countries and stored at the World Vegetable Center. These CPM-resistant lines are also resistant to tomato leaf curl New Delhi virus, and 28 of them are resistant to CDM. The majority of them were derived from Bangladesh landraces (Dhillon et al., Citation2020a). Luffa spp. need more focus and research aimed on various aspects of CPM resistance.
H. Bottle gourd (Lagenaria siceraria)
1. Sources of resistance
Bottle gourd accessions PI 271353 and PI 381840 exhibited moderate resistance to Px in a screen of the entire USDA, GRIN bottle gourd collections (Kousik et al., Citation2008). Bottle gourd lines USVL351-PMR (derived from accession PI 271351, originated from India) and USVL482-PMR (derived from accession PI 487482, originated from Israel) were highly resistant to Px in greenhouse and field tests (Kousik et al., Citation2018a). USVL482-PMR exhibited complete resistance to a local Px isolate in Thailand (Dhillon, unpublished). These bottle gourd rootstocks provided significantly greater levels of Px resistance to otherwise susceptible watermelon scions compared with numerous Px-resistant watermelon rootstocks (Kousik et al., Citation2018b).
2. Genetics of resistance
A pair of recessive genes reported to control resistance to CPM in bottle gourd (Robinson and Decker-Walters, Citation1997) was positively correlated with the number of favorable alleles carried in the genotype (Wu et al., Citation2020). Wang et al. (Citation2022) developed SCAR marker GPDSATG/CTC75 with a linkage distance of 9.6 cM from the CPM resistance gene reported in bottle gourd breeding line J083. The candidate gene cysteine receptor protein kinase 3 (CRK3) was used to develop PMR bottle gourd through GWAS (Wu et al., Citation2020). Zhang et al. (Citation2023b) proposed that CPM resistance in bottle gourd was enhanced via several pathways, including the antioxidant system, phenylalanine biosynthesis, and cell wall cellulose synthesis, and this information can be useful for screening and breeding CPM resistance in bottle gourd. MLO (mildew locus O) genes have a significant role in CPM defense system. LsMLO13 is likely a major CPM susceptibility factor in bottle gourd (Wang et al., Citation2023b) that could facilitate CPM resistance breeding of bottle gourd.
V. Discussion
A. Introduction
Powdery mildews are one of the most common and easy-to-recognize plant pathogen groups (Glawe, Citation2008). They are obligate plant parasites, usually debilitators and not killers (Burdon, Citation1993). However, they are considered and known as an economically very important group of pathogens that may substantially influence growth of plants, their yield, reproduction and quality (Spencer, Citation1978). Powdery mildews are known on about 10,000 species of angiosperms (Angiospermae) (Braun and Cook, Citation2012; Johnston et al., Citation2019; Kiss et al., Citation2020). They have a cosmopolitan distribution, and they occur in various bioms worldwide (Mieslerová et al., Citation2022), but individual species are usually highly host-specialized pathogens with a rather narow host range (Bélanger et al., Citation2002). Very broad virulence variation on specific host plants and their genotypes was reported for many PM species (Brown, Citation2002). This phenomenon has also been observed for CPM (Lebeda et al., Citation2021a) and it is critically considered in this review.
Recent research has shown that PM fungi are more diverse and their biology more complex than generally realized (Glawe, Citation2008). Powdery mildews are also used as a models for study of ecology, epidemiology and disease control, as well as for basic research on pathogen species diversity, ecology, host-parasite interactions, resistance mechanisms and genetics, molecular aspects of these interactions and their application in resistance breeding (Bélanger et al., Citation2002; Glawe, Citation2008; Kusch and Panstruga, Citation2017; Kiss et al., Citation2020; Mieslerová et al., Citation2022; Mapuranga et al., Citation2022; Zou et al., Citation2023).
One of the most studied host-pathogen interactions are the Cucurbitaceae and their powdery mildews. The study of CPM on cucurbits dates to the beginning of the 20th century (Sitterly, Citation1978), including research of resistance genetics and breeding for resistance (Sitterly, Citation1972, 1978; Jahn et al., Citation2002).
B. Host plants
The Cucurbitaceae family, including 95 genera and ca. 950–980 species, is one of the best-known, diverse, and most commercially used plant families worldwide (Schaefer and Renner, Citation2011). Many cucurbit species are important crops grown for edible fruits, for example melon, cucumber, watermelon, and squash and pumpkin, while some species are also used to make containers and musical instruments (e.g. bottle gourd) or as washing sponges (e.g. sponge gourd), many are also used in medicine (e.g., bitter gourd) (Rubatzky and Yamaguchi, Citation1997; Chomicki et al., Citation2020; Grumet et al., Citation2021). The phylogeny and classification of the Cucurbitaceae family has been studied for a long time, and the studies so far have brought many controversies for many genera. Scientists still argue about the concepts of the species and thus also about the resulting number of species within the genera (e.g. Cucurbita, Cucumis). It is difficult to apply a consistent system of taxonomic ranks across all main Cucurbitaceae genera (Citrullus, Cucumis, Cucurbita) because of differences in biology and patterns of their evolution (Nesom, Citation2011). For example, in the genus Cucurbita the paired taxa could be morphologically distinct but molecularly identical or nearly-identical.
Much attention is, therefore, paid to taxonomic studies and infraspecific variability of cucurbit crop wild relatives (Grumet et al., Citation2021), as crop landraces may be important sources of beneficial traits, including resistance against abiotic and biotic stresses (Grumet et al., Citation2021; Parvathi et al., Citation2022), and can be used for crop improvement in breeding programs (Endl et al., Citation2018). There are also still many puzzles remaining, e.g., the places of domestication of some species, when dual domestication of Lagenaria siceraria in America and Asia was surprisingly confirmed (Clarke et al., Citation2006), and origin of melon from Asian and African populations (Chomicki et al., Citation2020). Such studies are important for better understanding and correct interpretation of interactions between cucurbits and powdery mildews as well as for germplasm collection for use in cucurbit breeding programs. Various levels of genetic variation are known in different genera and species of cucurbits (Grumet et al., Citation2017). This is also evident for interactions between various cucurbits and powdery mildews as presented in this review. More detailed research must be focused on evaluation of germplasm collections for CPM resistance diversity (Grumet et al., Citation2021), as well as on studies of these interactions in cucurbit wild plant pathosystems, their relationships to crop plant pathosystems and their exploitation in plant breeding (Lebeda and Burdon, Citation2023).
C. Causal agents of CPM
Although powdery mildews are easily recognizable as a group of biotrophic pathogens, their precise species determination is quite a challenge even for expert taxonomists (Glawe, Citation2008; Braun and Cook, Citation2012; Braun et al., Citation2019). Determination of CPM species is relatively simple using light microscopy and precise using molecular methods, but beyond the reach of interest and attention of practical growers and many breeders. Knowledge of the exact species of powdery mildews is, however, very important in the case of CPM because they differ in their optimal ecological requirements for development and therefore in their occurrence and distribution in individual climatic regions of the world; they differ in host range, virulence, genetic variability, fungicide resistance and overall in economic impact on crop production (Křístková et al., Citation2009; Braun and Cook, Citation2012; Lebeda et al., Citation2010; Citation2021a).
Three important CPM genera, Golovinomyces, Podosphaera, and Leveillula occur on cucurbits. Current detailed and critical analysis of world literature showed that according to recent knowledge there are known on cucurbits at least seven well taxonomically defined causal agents (i.e., Erysiphales species) of CPM (). The taxonomic study of the genus Golovinomyces has already resulted in the distinction of many previously hidden species (see Braun et al., Citation2019), a more detailed distinction of species within the existing genus Podosphaera xanthii is still expected (see Yeh et al., Citation2021). Our study and conclusions also demonstrated that the taxonomy and denomination of individual CPM species rapidly changed during last decades (), and till now in scientific literature is frequently not correct, sometimes very chaotic, and not clearly unified. Further exacting research of host-pathogen relationships, including and genetics of interaction, and breeding is not possible without correct CPM determination.
The CPM endoparasitic species Leveillula taurica (Lév.) G. Arnaud, is not common (known in Mediterranean basin, Iran, Japan, Mexico, USA, USSR) (Khodaparast et al., Citation2016; Beltrán-Peňa et al., Citation2018), and has marginal economic importance (Keinath et al., Citation2017). Research on this species has, therefore been limited. However, in relation to climate changes, we may expect the spreading of this species to other areas (Pautasso et al., Citation2012; Mieslerová et al., Citation2022) and increase their severity.
The two ectoparasitic CPM genera Golovinomyces (with species G. orontii and G. cucurbitacearum) and Px, are important and very common pathogens of Cucurbitaceae world-wide (Křístková et al., Citation2009; Lebeda et al., Citation2010; Braun and Cook, Citation2012); differing in their geographical and seasonal distribution caused by different ecological requirements (Lebeda et al., Citation2009; Pirondi et al., Citation2015a; Trecate et al., Citation2019). These fungi can infect their hosts together (simultaneously) and cause “mixed infections” even in the same plant, crop, area and time, and cannot be distinguished by their symptoms. In Europe, Px prefers warmer areas or greenhouse crops, while Go occurs in cooler (central and north) areas and under field conditions, though in many parts of Europe they occur together (Křístková et al., Citation2009; Lebeda et al., Citation2009; Pirondi et al., Citation2015a; Lebeda et al., Citation2021a). Climate changes surely influence the changes in distribution and spreading of both genera; permanent monitoring of species occurrence is essential for formulating breeding and crop protection strategies (Lebeda et al., Citation2021a).
Detailed biogeographic studies on distribution and dynamics of individual CPM species on large-scale level (i.e., countries and continents) are lacking. There are many individual reports (or First Reports) on causal agents of CPM (e.g., Braun et al., Citation2001; Rennberger et al., Citation2018; Labo and Khan, Citation2019; Xu et al., Citation2020; Choi et al., Citation2021; Cui et al., Citation2021), but there are only few comprehensive papers on detailed monitoring of species distribution on a country or continental level (e.g., Lebeda, Citation1983; Křístková et al., Citation2009; Wu and Kirschner, Citation2017). Only two studies detailed longterm observations of CPM species distribution and spatiotemporal dynamics (Lebeda et al., Citation2009; Citation2021a), but such studies must be considered as one of the priorities in future CPM research.
All economically important cucurbits are hosts of CPM (Keinath et al., Citation2017). Only the common name of this disease, “cucurbit powdery mildew” or “powdery mildew” was used for many years from the beginning of the 20th century across all cucurbits without species-level specification/recognition of the causal agent in many research reports, and seed catalogues and grower-level information materials and generated considerable confusion in the CPM literature (Ballantyne, Citation1975). This was understandable in retrospect, as they reflected the state of CPM biology and taxonomy knowledge through that period.
Many contemporary research reports (e.g., Liu et al., 2008; Xu et al., Citation2016a; Citation2017; Wang et al., Citation2018; Citation2023a; Zhang et al., Citation2021a) refer only to “powdery mildew” without species specification, or evidence of methods used to determine species identity. It is our opinion such “black holes” in methodology represent “fatal defects” in the research and in the case of molecular and metabolic studies cannot lead to solid and trustworthy results.
The next problems of great challenge in CPM research are connected to enormous pathogenic variation (i.e., existence of numerous pathotypes and races) that has been known from the 1930s (Jagger et al., Citation1938b). Because of frequent and clear expression of compatibility or incompatibility in interactions between different cucurbits and CPM, this phenomenon created the opportunity for the classification of pathotypes and races. The concept of races is very important from scientific and practical breeding viewpoints (Lebeda et al., Citation2008; Citation2011; Citation2016a). This subject was seriously and broadly analyzed during the last two decades in our previous papers (e.g., Lebeda et al., Citation2008; Citation2011; Citation2016a; Citation2018a; Citation2021a). The leading idea of this research and activity was to elaborate a more precise and objective system of race determination, description, understanding and utilization acceptable and applicable internationally (Lebeda et al., Citation2016b). This process was succesfully finished in 2021/2022 by release of an internationally accepted set of melon differential genotypes for differentiation of virulence variation of CPM species (Lebeda et al., Citation2021b) originating from all cucurbit crops. From an historical viewpoint (Lebeda et al., Citation2008; Citation2011), this is the first unified and genetically homogeneous differential set freely available for use on a broad international scale (Lebeda et al., Citation2021b). It is open for future development (Lebeda et al., Citation2021b), i.e., for adding new differential genotypes as well as for more detailed genetic characterization like that made during last decades in lettuce (Parra et al., Citation2016).
The differential set could primarily be used for characterization of virulence of individual isolates and description of races of all CPM species. It was mostly focused on Px (McCreight, Citation2006), not much in other CPM species (Lebeda et al., Citation2008; Citation2011). Use of the set will reduce chaos and confusion in virulence characterization, description and denomination of races in literature focused on resistance or genetics of resistance (e.g., Zijlstra and Groot, Citation1992; Cao et al., Citation2020; Citation2022) and can lead to their correct interpretation. This differential set also provides the opportunity for detailed analyses of diversity and spatiotemporal fluctuations in the composition of CPM populations (not only on the level of races, but also on the level of virulence (v)-phenotypes and v-factors, their frequencies and diversity) with using appropriate analysis methodology (e.g., Schachtel et al., Citation2012; Lebeda et al., Citation2021a). It can provide crucial information for shaping breeding programs and predicting the most effective sources (or genes) of race-specific resistance (Lebeda et al., Citation2021a). The first population studies of Go and Px made in the Czech Republic showed enormous spatiotemporal virulence variation (Pirondi et al., Citation2016; Lebeda et al., Citation2018a; Citation2021a). We lack similar CPM virulence population studies in other countries where cucurbits are significant parts of horticulture based farming and industry, something that is common for other powdery mildews, e.g. Blumeria graminis (Komínková et al., Citation2016; Cieplak et al., Citation2022).
This review demonstrated that the most important cucurbit host crops are genetically variable on different levels. This variation is expressed also in interactions between genotypes of various cucurbits with different isolates (races) of individual CPM species (summarized in Part 4), mostly showing well defined race-specific reaction patterns. This is an opportunity to create specific differential sets for CPM species originating also from some other cucurbits (e.g., C. lanatus and Cucurbita spp.). However, races of CPM have not been yet clearly classified for other commercially important cucurbits because of the lack of differentials and fully CPM-resistant germplasm (Cohen et al., Citation2004). In this case, it is possible to consider in much more detail the host (genera and species) specificity of CPM isolates that is not possible when the melon differential set is used for CPM species originating from different cucurbit species. This approach could yield much more specific information on host-pathogen interactions and detailed information on virulence variation of CPM species on individual species of cucurbits. For example, Kousik and Ikerd (Citation2014) established a good base for development of a watermelon-Px differential set for characterization of virulence variability. Their study indicated the presence of at least two races based on a line derived from one highly susceptible genotype and the old watermelon cv. Mickey Lee (Kousik and Ikerd, Citation2014). Another example of the application this approach is bitter gourd where genotypes exhibited differentiation capacity for Px isolates (Dhillon et al., Citation2018). Such developments must be based, however, on long-term field observations and experiments, which requires huge amounts of data on host resistance and pathogen virulence variation, and could take decades, as evident from the history of the melon differential set development (Lebeda et al., Citation2008; Citation2011; Citation2016a; Citation2021b).
D. Resistance and host—CPM interactions
1. Cucumber (Cucumis sativus)
Kooistra (Citation1968) highlighted the difficulty in comparing CPM resistance results among various countries due to occurrence of Px and/or Go, and drew three different possible conclusions in this regard: i) resistance observed in U.S.A. and in Russia is erroneously attributed to Go; Kable and Ballantyne (Citation1963) concluded that Px also occurred in the U.S.A. and may be prevalent there (see McCreight, Citation2004); ii) resistances to both fungi can be attributed to the same genes; and iii) resistances to both fungi happen to run parallel (a relation between original source of resistance is not excluded) or are linked. He noted further research may provide the answer.
In light of plant-pathogen interactions research over the past ca. 50 years, we provide these conclusions: i) even in the most recent peer-reviewed papers aimed at genetic mapping and molecular mechanisms of resistance, precise determination of CPM species is often fully omitted; ii) the role of mlo genes in generally developed resistance responses to both Px and Go species must be examined and verified; and iii) from a philosophical point of view, parallel evolution of resistance is natural and acceptable. Our long-term (>30 years) studies of physiological specialization (i.e., races) of Px and Go, revealed significant spatio-temporal fluctuations of virulence in both species (Lebeda et al., Citation2018a; Citation2021a), and contrasting interaction patterns between a set of differential host genotypes of melon and pathogen isolates were observed (Lebeda et al., Citation2016a; Citation2016b; Citation2021b). A differential set of cucumber genotypes has yet to be developed because of low genetic diversity for CPM resistance in cucumber, but now may be an opportune time to develop such differential set.
The first step to understanding cucumber responses to CPM should be the testing of reactions of a set of suitable candidate genotypes (reported as resistant under field conditions) in response to taxonomically determined isolates of Px and Go with well characterized virulence patterns on the melon CPM differential set (Lebeda et al., Citation2016a; Citation2021b). Such activities can only be achieved through a broad international cooperation and coordination (Lebeda et al., Citation2016b; Citation2021b).
A large number of important CPM response genes have been identified in cucumber, including WRKY, NAC, TCP transcription factors, plant hormone biosynthesis, genes related to metabolism and signal transduction, and defense biotic stress response-related genes, which indicates a complex of responses in cucumber to CPM infection.
As we previously mentioned, basic proof of CPM species authenticity is missing even in papers dealing with metabolic pathways during pathogenesis. Zheng et al. (Citation2021) confirmed and elucidated signaling pathways in host tissues during the first stages of pathogenesis, but it is not clear if these pathways are CPM species-specific. The authors assumed Px as the CPM species, though no species identification was performed or reported. The extent to which these processes are species-specific remains an open question.
Chen et al. (Citation2021a) stressed in their review that CPM is one of the most severe foliar diseases of cucumber, but inheritance of CPM resistance remains unclear. The molecular mechanisms underlying CPM resistance have been a bottle-neck in the advancement of cucumber cultivars, though recent findings have provided valuable information on the molecular mechanisms of defense-related genes and signaling pathways. Moreover, several CPM resistance genes have been cloned, and their functions have been verified in cucumbers (Chen et al., Citation2021a).
The above mentioned “weak point,” i.e., lack of CPM species and race determination, in the research of CPM–cucumber interactions is not surprising in light of their statement: “Collectively, the inheritance of cucumber CPM resistance is complex and affected by temperature and region. Increasing numbers of CPM-related genes have been detected, which provide insights into the genetic control of CPM resistance in several cucumber cultivars, but the QTLs identified in these studies are inconsistent and the picture continues to be unclear “(Chen et al., Citation2021a).
Recent findings on the inheritance, molecular markers, and QTL mapping of cucumber CPM, CDM and Fusarium wilt (Fusarium oxysporum f. sp. cucumerinum, FW) resistances were summarized by He et al. (Citation2022). Wang et al. (Citation2020) reviewed candidate genes for CPM, CDM, and FW resistances, with or without functional verification with the aim to reveal cucumber disease resistance molecular mechanisms. They concluded inheritance of cucumber disease resistance varies among resistance sources, and the mechanisms of CPM resistance are not well understood. Many factors affect cucumber resistance responses to these three pathogens, including pathogenic race, plant genotype, pathogen invasion site, environment, and genetic linkage, resulting in a variety of effective molecular markers and QTLs for cucumber disease resistance. Large numbers of candidate genes and immune proteins associated with CDM, CPM, and FW resistances have been identified using mapping, GWAS, RNA sequencing (RNA-seq), and proteomic assay technology, but only a few have been functionally verified in cucumber, e.g., MLO-like genes important in CPM.
He et al. (Citation2022) proposed five aspects of future research to effectively prevent cucumber diseases, and explore the genetic and molecular mechanisms of resistances to CPM, CDM, and FW:
Collect more disease-resistant cucumber germplasm, especially materials that are resistant to multiple pathogens, including wild germplasm resources, cultivars, and mutants.
Identify more effective molecular markers and QTLs associated with CPM, CDM, and FW to be used in selecting germplasms and accelerating resistance breeding.
Analyze more differently expressed DNA, RNA, miRNAs, lncRNAs, or metabolic related to CPM, CDM, or FW, respectively, through omics or multiomics and bioinformatics tools would provide considerable experimental information for mechanistic investigations and understand the regulatory network for cucumber diseases, such as transcriptomics, proteomics, metabolomics, epigenomics, and interactomics.
Improve the efficiency and stability of genetic transformation in cucumber.
Develop persistent and safe preventive measures, including chemical, biological, and physical controls.
2. Melon (Cucumis melo)
Xu et al. (Citation2022) reviewed the advances in genetics, genomics, and the breeding of melon. They stressed that the diverse climatic conditions resulted in differentiation in the dominant physiological races of CPM around the world. Numerous resistance genes and QTL associated with resistance to the many Go and Px races were identified in a diverse array of resistance sources ( and ). They concluded that the different results obtained in inheritance patterns and QTL mapping may be due to the diverse physiological races of CPM and resistant accessions used. Unfortunately, there are few functional genes reported to date. They stressed that most accessions with resistance to CPM belong to Groups Momordica (such as snapmelon) and Acidulus (mostly cultivated in southern parts of India). Most melon accessions with resistance come from the primary and secondary diversity centers (Dhillon et al., Citation2012) and could be considered important germplasm reservoirs for melon breeders. Therefore, exploiting the accessions for resistance improvement in melon is imperative.
Finally, Xu et al. (Citation2022) stressed that traditional breeding based on crossing and selection remains important for crop improvement. Although the efficiency of crossing and selection has been improved using marker-assisted selection, it faces limitations in crops with complex genetics. With the increase of re-sequencing data in melon, more and more polymorphic SNPs have been identified. It is feasible to construct the platform based on whole genome selection. Genome editing is expected to be a powerful tool to create desirable variation using molecular scissors and artificially engineered nucleases. The utilization of CRISPR/Cas editing can accelerate melon improvement through the introduction of genetic variation in a targeted manner (Pan et al., Citation2022). The application of genome editing is based on an effective and stable genetic transformation system. Nevertheless, though some research related to melon genetic transformation has been reported, the efficiency and universality of distinct genotypes is the limiting factor. The breakthrough of genetic transformation assisted by genes encoding developmental regulators in watermelons could provide a good reference for melons (Feng et al., Citation2021).
Cui et al. (Citation2022b) summarized efforts to identify sources of resistance to CPM and CDM in cultivated and wild melon germplasm through a literature review of the last 50+ years. Resistance to PM was identified in 239 melon accessions, including C. melo Group. Cantalupensis accessions PMR 45, PMR 5, PMR 6, and WMR 29. Group Momordica accessions from India PI 124111, PI 124112, and PI 414723 have been considered as the most valuable germplasm because multiple CPM resistance genes have been identified from these accessions and are widely used in PMR breeding since 1932 (Jahn et al., Citation2002). Genetic mapping in a number of resistance sources enabled identification of 25 dominant genes, two recessive genes and seven QTL conferring CPM resistance. Based on the reported sequences of associated markers, Cui et al. (Citation2022b) anchored physically (many of) these genes and QTL to chromosomes of the melon line DHL92 genome. They suggested three strategies aimed at breeding melon with durable and broad-spectrum resistance to pathogens and pests: 1) Host resistance is generally the most favorable control method when considering environmental, economic and social factors; 2) To achieve durable and broad-spectrum resistance in melon, tools that facilitate disease evaluation need to be further developed, e. g., high-throughput phenotyping, including precision controlled inoculation and objective disease scoring in order to unambiguously assess resistance reactions; 3) Utilization of impaired susceptibility genes as an alternative strategy for melon resistance breeding to overcome emerging resistance-breaking variants and lack of dominant resistance sources. They expect that a strategic combination of dominant R genes/QTL with mutant alleles of S genes will aid the sustainability of disease resistance in melon.
Within cucurbit crops, breeding of melon for resistance to the CPM has longest tradition initially started by J. T. Rosa and I. C. Jagger, U.S. Department of Agriculture, Agricultural Research Service (USDA, ARS) (Dhillon et al., Citation2012; Jahn et al., Citation2002); longlasting research activities have led to the elaboration of a differential set of melon genotypes for race determination and denomination (Lebeda et al., Citation2016a; Citation2021b). Researchers and breeders have an excellent tool to combine “classical” and “advanced” approaches, which can result in better and more exact understanding and development in this topic.
3. Cucurbita species
The effort to develop CPM-resistant cultivars in Cucurbita spp. is less intense in comparison to melon, very likely because of lower economic importance of squashes and pumpkins (Lebeda et al., Citation2007a). The screening of resistance should be based on the generally accepted and stable methodology (based on leaf disk technique), and system of CPM race determination and denomination (Lebeda and Sedláková, Citation2010; Lebeda et al., Citation2016a; Citation2021b). Until the end of last decade a unified system of CPM virulence description was not available, it was difficult to compare data from various screenings Cucurbita spp. for resistance to PM.
Because of robust plant habit, and large leaf size, screening of Cucurbita spp. for CPM resistance is technically more complicated and space consuming than screening of Cucumis species. Field observations on responses of Cucurbita spp. to CPM represent the first step in the resistance studies. Reports from field screenings (e.g., Lebeda and Křístková, Citation1994; Citation1996a; Citation1996b; Keinath and DuBose, Citation2000; Caligiore-Gei et al., Citation2022) refer the phenomenon of “field resistance,” which is influenced by various factors, e.g., weather conditions, developmental stage of plants, structure and spatiotemporal changes of CPM species populations and their race spectra (Lebeda et al., Citation2021a). These results must be verified by laboratory/growth chamber testing in well-defined conditions and with exactly identified CPM species and races. Unfortunately this is not common even nowdays.
Wide hybridization in plant breeding is an important approach (Singh et al., Citation2021). Utilization of wild Cucurbita germplasm in resistance breeding is very valuable and attractive (Křístková and Lebeda, Citation1997; Jahn et al., Citation2002; Lebeda et al., Citation2007a; Grumet et al., Citation2021). The strategy to transfer the CPM resistance from wild Cucurbita species (C. lundelliana and C. okeechobeensis ssp. martinezii) (Com) to cultivated species C. pepo and C. moschata via interspecific crossing was documented by early reports (Whitaker, Citation1956; Rhodes, Citation1964; Sitterly, Citation1972). Development of resistant cultivars was “blocked/limited” by the fact that interspecific hybrids with C. lundelliana exhibited unfavorable traits from wild parents (Jahn et al., Citation2002). Paris and Cohen (Citation2002) reported good economic results (high yield) of zucchini, cocozelle, vegetable marrow F1 hybrids derived from CPM-resistant C. okeechobeensis. Other examples are given in the previous part of this review.
Breeders and researchers use extensively the Com-derived major resistance gene Pm-0 in breeding and research (Holdsworth et al., Citation2016). There are no reports to our knowledge of precise screening of a broad collection of wild Cucurbita spp. germplasm for response to CPM, as has been done for CDM (Lebeda et al., Citation2016c; Citation2019).
The exploration of resistance to CPM among wild Cucurbita spp. is very important. Germplasm accessions available in genebanks should be completed by plant (seed) material newly originally collected in the areas of their origin and distribution (Grumet et al., Citation2021). According to our opinion such activities seems to be “old fashioned” but have a primary importance. They can be limited by complicated terrain and political instability in focus regions. Elaboration of species concept within Cucurbita genus, knowledge of species relations and gene pools of cultivated ones, and basic knowledge of individual species determination and distribution is essential for planning collection trips, for plant material collecting and further exploitation, e.g., resistance screening. Another, partly neglected source of valuable resistance genes can be located in old cultivars and landraces, (some of which are kept by genebanks and some are maintained in situ in family farms (Grumet et al., Citation2021).
Obstacles in interspecific hybridization and sterility of produced hybrids could be overcome via application of different biotechnology approaches (Gajdová et al., Citation2004; Lebeda et al., Citation2007a; Navrátilová et al., Citation2011). C. moschata is known as a bridge species in interspecific crossing (Liu et al., Citation2022). Except for recent enormous progress in genomic research of cucurbits (Grumet et al., Citation2017), classical breeding combined with suitable biotechnology approaches are currently broadly used and fully accepted.
Grafting, which is a cultivation method of interest to breeders and growers could influence responses of cucurbit plants to CPM. There was progress in development of rootstocks from C. moschata and C. pepo lines resistant to CPM (Jorkesh et al., Citation2018).
Recent QTL mapping and molecular markers of CPM resistance in pumpkin (C. moschata) confirmed that the CPM resistance was introduced from C. okeechobeensis (Park et al., Citation2020). It was not clear from this report whether the authors dealt with material developed in the 20th century, or if they repeated this interspecific cross.
The Mildew resistance locus o (Mlo) in C. moschata was reported by Win et al. (Citation2018), and in C. moschata, C. maxima and C. pepo by Zhu et al. (Citation2021). The study of the MLO gene family in Cucurbitaceae (Iovieno et al., Citation2015) represents the first step in the practical application of this gene family in breeding cucurbits for resistance to CPM. There are not yet to our knowledge any available cucurbit cultivars based on this gene family.
4. Watermelon (Citrullus lanatus)
The need for breeding watermelon for resistance to the CPM appeared in the end of 20th century (Jahn et al., Citation2002). For a long period, this species was considered resistant to CPM. So, in the comparison to melon, where different approaches to determine races have led to various systems of determination and denomination (and to chaos in race labeling) and resistance genes identification, resistance breeding in watermelon can be based on recent developments (Lebeda et al., Citation2016a; Citation2021a), and misunderstanding in communication can be avoided.
Soon after recognition of watermelon as a host of CPM, screening large collections of C. lanatus and related species for reactions to CPM were realized (e.g., Davis et al., Citation2007; Tetteh et al., Citation2010; Ben-Naim and Cohen, Citation2015), and genotypes resistant to local races in Charleston (USA) were identified. Kousik et al. (Citation2019) suggested testing their resistance in other geographic regions in order to identify variation of resistance and responses to local races, which comports with the concept of spatio-temporal changes of virulence within populations of powdery mildew species (Lebeda et al., Citation2021a).
Comprehensive reviews are reporting only resistance to “powdery mildew” in some accessions (PI 494531, PI 482326, PI 482328, PI 189225, PI 532738) (Levi et al., Citation2017b), without any pathogen details. Thus, we are missing informative details of the reactions of various C. lanatus accessions and their related species to different CPM species and race isolates.
Recent studies revealed physiological reponses of watermelon CPM resistance, e.g., on role of melatonin (Mandal et al., Citation2018; Citation2020), and complex pathways in lignin biosynthesis (Yadav et al., Citation2021b; Citation2022). Mandal et al. (Citation2018) suggested increased melatonin levels can lead to resistance of watermelon against diverse filamentous pathogens, including CPM.
Genetic (molecular) mapping can promote MAS, where resistance to Px race 1 W is combined to fruit traits (e.g., Kim et al., Citation2013; Citation2015b). Many studies aimed at detection of candidate genes involved in resistance use usually two host genotypes with contrasting response to isolate of pathogen (Kim et al., Citation2013; Citation2015b; Yadav et al., Citation2022). Results are unclear, many candidate genes are detected.
A different experimental model could bring more light to the biosynthetic pathways during pathogenesis based on:
use of two well denominated races of verified CMP species;
host genotypes with contrasing responses (resistant vs. susceptible) to the selected races;
study differences in metabolic processes, including production of metabolites, and gene expression during pathogenesis.
5. Momordica, Luffa and Lagenaria
A few sources of resistance to CPM are available in bitter gourd, bottle gourd, and ridge gourd (Kousik et al., Citation2008; Dhillon et al., Citation2018; Kousik et al., Citation2018a). Evolutionary capacity of CPM species of Px is quite high (McDonald and Linde, Citation2002a; Citation2002b) and thus likely to overcome race-specific type of resistance. It is, therefore, essential to secure more sources of CPM resistance (with potentially unique resistance genes) in these species through collection of more landraces from their centers of crop diversity. Their evaluation against Px in multiple locations across Asia will facilitate establishment of informative race differential, as has been for melon. Recessively controlled and intermediate (moderate) level resistance to Px has been reported in bitter gourd (Dhillon et al., Citation2019). Farmers prefer intermediate resistance in bitter gourd because currently available complete/high level resistance has been observed by the commercial seed industry to be associated with late fruiting (M. Anil, 2023, personal communication). Genomic resources for development of molecular markers linked to Px resistance genes in bitter gourd, bottle gourd, and ridge gourd are a high priority for future CPM resistant cultivar development initiatives. In general, these crops need much more intense research to fulfill many gaps in knowledge of host diversity, CPM distribution and virulence variation, host-pathogen interactions and their application in resistance breeding.
E. Concluding comments
Host-pathogen interactions between various cucurbits and their respective CPM species are complicated, diverse and complex on different levels of biological hierarchy. There has been enormous progress in research and resistance breeding in this area since the turn of the century, but many gaps and challenges remain for future research, as evident from this review. In the previous comprehensive review of this topic Jahn et al. (Citation2002) concluded that: “Although nothing is understood about the mechanisms by which plants resist this pathogen and selection has been based entirely on plant phenotype under disease pressure, tremendous progress has occurred in the creation of powdery mildew resistant genotypes of all four major crop species (melon, cucumber, squash and pumpkin, watermelon). It will be of great interest to learn the physiological and molecular genetic basis of this trait, which has had such a significant impact on the production of these crops.”
Though we see significant progress, as evidenced by the many scientific reports summarized in this review, based on phenotypic and genotypic analysis, studies of physiological and structural background of cucurbit resistance to CPM, and generation of many candidate resistance genes, we still lack evidence of their real and broader practical applications in CPM resistance breeding (e.g., Das et al., Citation2022; Matthew, Citation2022). Pedigrees of new cultivars are not publically available, with the exceptions of USDA and universities in the U.S.A. (Brzozowski et al., Citation2016) and state companies in China (Cheng et al., Citation2023), so it is not possible to fully assess the real role and importance of all possible approaches. We understand the aggressive and successful application of breeding know-how, including trade secrets and utility patents for commercial seed companies in various competitive markets. This complicates general progress in understanding plant-pathogen interactions, their background and changes from a scientific viewpoint, and results in a research dichotomy with two bodies of knowledge, one held by private breeding companies without public accessibility the other freely available, e.g., technical and scientific publications. There is “one way” inspiration flow from publically available information sources to the private sector. The enormous pressure in the public sphere to continuously produce scientific papers (Rawat and Meena, Citation2014) leads to “fast papers.” We demonstrate in this review, that in some molecular papers authors are omitting basic and correct biological information on the pathogens (i.e., species specification) and their pathogenic characteristics. This is true also for cucurbit-CPM interactions research. Recent research articles on CPM resistance in cucurbits evidences strong fragmentation of research activities, going deeply to the details, but on the other hand we lack potential synthesis from combining various research approaches. Researchers working with CPM could be more inspired by activities and results dealing with similar host-pathogen interactions (e.g. PM on cereals; Mapuranga et al., Citation2022; Zou et al., Citation2023). Studies of crop wild relatives, their exploitation and use in breeding programs are essential (McCouch et al., Citation2020). Comparative research on interactions between wild and cultivated cucurbit CPM pathosystems may facilitate progress in this area (Lebeda and Burdon, Citation2023).
Authors’ contributions
A.L. conceived and designed the study, and supervised elaboration of the manuscript; A.L., E.K., B.M., J.D.M., and N.P.S.D. processed and interpreted the specific information, wrote and revised the manuscript. A.L. and J.D.M. edited the manuscript. All authors have read and approved the final version of the manuscript.
Acknowledgements
A.L. thanks U. Braun (Martin Luther University, Halle, Germany) for reading and commenting on powdery mildew taxonomy. The authors thank S. A. Khodaparast (University of Guilan, Rasht, Iran), L. Trecate (previously Universita Cattolica del Sacro Cuore, Piacenza Italy), and B. Sedláková (Palacký University in Olomouc, Czech Republic) for providing some photos of cucurbit powdery mildews. The authors thank the reviewers for their helpful and generous comments that have undoubtedly led to an improved manuscript.
Disclosure statement
No potential conflict of interest was reported by the author(s).
Additional information
Funding
References
- Aalbersberg, I. W., and Stolk, J. H. 1995. Evaluation of resistance to and tolerance of powdery mildew by cucumber. Plant Variet. Seeds 8: 119–123.
- Abu-Hammour, K., and Wittmann, D. 2010. Pollination and pollinators of Cucurbita pepo (Cucurbitaceae) in the Jordan Valley to improve seed set. Advan. Hortic. Sci. 24: 249–256.
- Adeniji, A. A., and Coyne, D. P. 1983. Genetics and nature of resistance to powdery mildew in crosses of butternut with calabaza squash and ‘Seminole Pumpkin'. J. Amer. Soc. Hort. Sci. 108: 360–368. doi:10.21273/JASHS.108.3.360
- Adhikari, B. N., Savory, E. A., Vaillancourt, B., Childs, K. L., Hamilton, J. P., Day, B., Buell, C. R. 2012. Expression profiling of Cucumis sativus in response to infection by Pseudoperonospora cubensis. PLoS ONE 7: e34954.
- Ahamed, K. U., Akhter, B., Islam, M. R., Ara, N., and Humauan, M. R. 2012. An assessment of morphology and yield characteristics of pumpkin (Cucurbita moschata) genotypes in northern Bangladesh. Trop. Agric. Res. Ext. 14: 7–11. doi:10.4038/tare.v14i1.4834
- Akashi, Y., Fukuda, N., Wako, T., Masuda, M., and Kato, K. 2002. Genetic variation and phylogenetic relationships in East and South Asian melons, Cucumis melo L., based on the analysis of five isozymes. Euphytica 125: 385–396. doi:10.1023/A:1016086206423
- Alavilli, H., Lee, J. J., You, C.-R., Poli, Y., Kim, H.-J., Jain, A., and Song, K. 2022. GWAS reveals a novel candidate gene CmoAP2/ERF in pumpkin (Cucurbita moschata) involved in resistance to powdery mildew. Int. J. Mol. Sci. 23: 6524. doi:10.3390/ijms23126524
- Alvarez, J. M., González-Torres, R., Mallor, C., and Gómez-Guillamón, M. L., 2005. Potential sources of resistance to Fusarium wilt and powdery mildew in melons. HortScience 40: 1657–1660. doi:10.21273/HORTSCI.40.6.1657
- An, J. Y., Yin, M. Q., Zhang, Q., Gong, D. T., Jia, X. W., Guan, Y. J., and Hu, J. 2017. Genome survey sequencing of Luffa cylindrica L. and microsatellite high resolution melting (SSR-HRM) analysis for genetic relationship of Luffa genotypes. Int. J. Mol. Sci. 18: 1942. doi:10.3390/ijms18091942
- Anagnostou, K., Jahn, M., and Perl-Treves, R. 2000. Inheritance and linkage analysis of resistance to zucchini yellow mosaic virus, watermelon mosaic virus, papaya ringspot virus and powdery mildew in melon. Euphytica 116: 265–270. doi:10.1023/A:1004005716806
- Andolfo, G., Iovieno, P., Ricciardi, L., Lotti, C., Filippone, E., Pavan, S., and Ercolano, M. R. 2019. Evolutionary conservation of MLO gene promoter signatures. BMC Plant Biol. 19: 150. doi:10.1186/s12870-019-1749-3
- Argyris, J. M., Ruiz-Herrera, A., Madriz-Masis, P., Sanseverino, W., Morata, J., Pujol, M., Ramos-Onsins, S. E., and Garcia-Mas, J. 2015. Use of targeted SNP selection for an improved anchoring of the melon (Cucumis melo L.) scaffold genome assembly. BMC Genomics 16: 4. doi:10.1186/s12864-014-1196-3
- Arnaud, G. 1921. Les Astérinées: II. Études sur les champignons (Parodiellinacées, inclus Erysiphées). Annales Des Épiphyties 7: 1–115.
- Atarashi, H., Sakaguchi, K., Asami, H., Matsui, S., Kano, A., and Kotani, S. 2019. New powdery mildew resistance marker useful for producing powdery-mildew resistance Cucurbita maxima plant and imparting resistance against powdery mildew to plants, comprises powdery mildew resistance locus on chromosome 3. Patent Number(s): JP2019024483-A. Patent Assignee: TAKII SHUBYO KK (TAKI-Non-standard). Derwent Primary Accession Number: 2019-18590E
- Atarashi, H., Sakaguchi, K., Asami, H., Matsui, S., Kano, A., Kotani, S., Hisanori, S., Keitaro, S., Hiroshi, A., Shintaro, M., Akihito, K., Sayaka, K., Shin, H., and Kodani, S. 2018. New powdery mildew resistance Cucurbita maxima plant has powdery mildew resistant locus on chromosome 3 comprising specific polynucleotide. Patent numbers JP6306252-B1; WO2019026924-A1; JP2019024411-A; KR2019045190-A; CN109661478-A; MX2019002442-A1; CA3070721-A1; EP3663402-A1; US2020205362-A1; KR2156692-B1.
- Aust, H. J., and Hoyningen-Huene, J. 1986. Microclimate in relation to epidemics of powdery mildew. Annu. Rev. Phytopathol. 24: 491–510. doi:10.1146/annurev.py.24.090186.002423
- Badri Anarjan, M., Bae, I., and Lee, S. 2021. Marker-assisted evaluation of two powdery mildew resistance candidate genes in Korean cucumber inbred lines. Agronomy 11: 2191. doi:10.3390/agronomy11112191
- Ballantyne, B. J. 1975. Powdery mildew of cucurbitaceae: identity, distribution, host range and sources of resistance. Proc. Linn. Soc. New South Wales 99: 100–120.
- Bardin, M., Carlier, J., and Nicot, P. C. 1999. Genetic differentiation in the French population of Erysiphe cichoracearum, a causal agent of powdery mildew of cucurbits. Plant. Pathol. 48: 531–540. doi:10.1046/j.1365-3059.1999.00380.x
- Barnes, W., and Epps, W. 1956. Powdery mildew resistance in South Carolina cucumbers. Plant Dis. Rep. 40: 1093.
- Barsoum, M., Kusch, S., Frantzeskakis, L., Schaffrath, U., and Panstruga, R. 2020. Ultraviolet mutagenesis coupled with next-generation sequencing as a method for functional interrogation of powdery mildew genomes. Mol. Plant. Microbe Interact. 33: 1008–1021. doi:10.1094/MPMI-02-20-0035-TA
- Behera, T. K., Behera, S., Bharathi, L. K., John, K. J., Simon, P. W., and Staub, J. E. 2010. Bitter gourd: Botany, horticulture, breeding. Hort. Rev. 37: 101–141.
- Behera, T. K., Sureja, A. K., Islam, S., Munshi, A. D., and Sidhu, A. S. 2012. Minor cucurbits. In Genetics, Genomics, and Breeding of Cucurbits; Wang, Y.-H., Behera, T. K., and Kole, C. Eds. CRC Press: New York, NY, pp 17–60.
- Bélanger, R. R., Bushnell, W. R., Dik, A. J., and Carver, T. L. W. Eds. 2002. The Powdery Mildews. A Comprehensive Treatise. APS Press: St. Paul, MN.
- Beltrán-Peña, H., Solano-Báez, A. R., Apodaca-Sánchez, M. Á., Camacho-Tapia, M., Félix-Gastélum, R., and Tovar-Pedraza, J. M. 2018. First report of Leveillula taurica causing powdery mildew on cucumber in Mexico. J. Plant Pathol. 100: 353–353. doi:10.1007/s42161-018-0061-7
- Ben-Naim, Y., and Cohen, Y. 2015. Inheritance of resistance to powdery mildew race 1W in watermelon. Phytopathology 105: 1446–1457. doi:10.1094/PHYTO-02-15-0048-R
- Beraldo-Hoischen, P., Hoefle, C., and López-Sesé, A. I. 2021. Fungal development and callose deposition in compatible and incompatible interactions in melon infected with powdery mildew. Pathogens 10: 873. doi:10.3390/pathogens10070873
- Berg, J. A., Appiano, M., Martínez, M. S., Hermans, F. W. K., Vriezen, W. H., Visser, R. G. F., Bai, Y. L., and Schouten, H. J. 2015. A transposable element insertion in the susceptibility gene CsaMLO8 results in hypocotyl resistance to powdery mildew in cucumber. BMC Plant Biol. 15: 243. doi:10.1186/s12870-015-0635-x
- Bertrand, F. 1991. Les oïdiums des Cucurbitacées: Maintien en culture pure, étude de leur variabilité et de la sensibilité chez le melon. Ph.D. Thesis 225, University Paris XI, Orsay, France (in French).
- Bertrand, F., Pitrat, M., Glandard, A., and Lemaire, J. M. 1992. Diversité et variabilité des champignons responsables de l’oïdium des cucurbitacées. Phytoma 438: 46–49.
- Bharathi, L. K., Munshi, A. D., Behera, T. K., Vinod, Joseph, J. K., Bhat, K.V., Das, A.B., and Sidhu, A. S. 2012. Production and preliminary characterization of novel inter-specific hybrids derived from Momordica species. Curr. Sci. 103: 178–186.
- Bhawna, Abdin, M. Z., Arya, L., Saha, D., Sureja, A. K., Pandey, C., and Verma, M. 2014. Population structure and genetic diversity in bottle gourd (Lagenaria siceraria (Mol.) Standl.) germplasm from India assessed by ISSR markers. Plant Syst. Evol. 300: 767–773. doi:10.1007/s00606-014-1000-5
- Bhawna, Abdin, M. Z., Arya, L., and Verma, M. 2015. Transferability of cucumber microsatellite markers used for phylogenetic analysis and population structure study in bottle gourd (Lagenaria siceraria (Mol.) Standl.). Appl. Biochem. Biotechnol. 175: 2206–2223. doi:10.1007/s12010-014-1395-z
- Block, M., Knaus, B. J., Wiseman, M. S., Grünwald, N. J., and Gent, D. H. 2021. Development of a diagnostic assay for race differentiation of Podosphaera macularis. Plant Dis. 105: 965–971. doi:10.1094/PDIS-06-20-1289-RE
- Block, C. C., and Reitsma, K. R. 2005. Powdery mildew resistance in the U.S. National Plant Germplasm system cucumber collection. HortScience 40: 416–420. doi:10.21273/HORTSCI.40.2.416
- Blumer, S. 1933. Die Erysiphaceen Mitteleuropas unter besonderer Berücksichtigung der Schweiz. Beitr. Kryptogamenflora Schweiz 7: 1–483.
- Bohn, G. W., and Whitaker, T. W. 1964. Genetics of resistance to powdery mildew race 2 in muskmelon. Phytopathology 54: 587–591.
- Bradshaw, M. J., Braun, U., and Pfister, D. H. 2022. Phylogeny and taxonomy of the genera of Erysiphaceae, part 1: Golovinomyces. Mycologia 114: 964–993. doi:10.1080/00275514.2022.2115419
- Branham, S. E., Kousik, C., Mandal, M. K., and Wechter, W. P. 2021. Quantitative trait loci mapping of resistance to powdery mildew race 1 in recombinant inbred line population of melon. Plant Dis. 105: 3809–3815. doi:10.1094/PDIS-12-20-2643-RE
- Braun, U. 1983. Descriptions of new species and combinations in Microsphaera and Erysiphe (IV). Mycotaxon 18: 113–129.
- Braun, U. 1987. A monograph of the Erysiphales (powdery mildews). Beih. Nova Hedwigia 89: 1–700.
- Braun, U. 1999. Some critical notes on the classification and generic concept of the Erysiphaceae. Schlechtendalia 3: 49–55.
- Braun, U., and Cook, R. T. A. 2012. Taxonomic manual of the Erysiphales (Powdery Mildews). CBS Biodiver. Ser. 11: 1–707.
- Braun, U., Kiehr, M., and Delhey, R. 2001. Some new records of powdery mildew fungi from Argentina. Sydowia 53: 34–43.
- Braun, U., Shin, H. D., Takamatsu, S., Meeboon, J., Kiss, L., Lebeda, A., Kitner, M., and Götz, M. 2019. Phylogeny and taxonomy of Golovinomyces orontii revisited. Mycol. Progress 18: 335–357. doi:10.1007/s11557-018-1453-y
- Braun, U., Shishkoff, N., and Takamatsu, S. 2001. Phylogeny of Podosphaera sect. Sphaerotheca subsect. Magnicellulatae (Sphaerotheca fuliginea s. lat.) inferred from rDNA ITS sequences – a taxonomic interpretation. Schlechtendalia 7: 45–52.
- Braun, U., and Takamatsu, S. 2000. Phylogeny of Erysiphe, Microsphaera, Uncinula (Erysipheae) and Cystotheca, Podosphaera, Sphaerotheca (Cystotheceae) inferred from rDNA ITS sequences some taxonomic consequences. Schlechtendalia 4: 1–33.
- Brickell, C. D. (Ed.). 1980. International Code of Nomenclature for Cultivated Plants-1980: Formulated and Adopted by the International Commission for the Nomenclature of Cultivated Plants of the I.U.B.S. Bohn, Scheltema & Holkema: Utrecht, the Netherlands; International Commission for the Nomenclature of Cultivated Plants.
- Brown, J. K. M. 2002. Comparative genetics of avirulence and fungicide resistance in the powdery mildew fungi. In The Powdery Mildews. A Comprehensive Treatise; Bélanger, R. R., Bushnell, W. R., Dik, A. J., and Carver, W. L. T. Eds. APS Press: St. Paul, MN, pp 56–65.
- Brzozowski, L., Holdsworth, W. L., and Mazourek, M. 2016. DMR-NY401: a new downy mildew-resistant slicing cucumber. HortScience 51: 1294–1296. doi:10.21273/HORTSCI10857-16
- Burdon, J. J. 1993. The structure of pathogen populations in natural plant communities. Annu. Rev. Phytopathol. 31: 305–323. doi:10.1146/annurev.py.31.090193.001513
- Burger, Y., Paris, H. S., Cohen, R., Katzir, N., Tadmor, Y., Lewinsohn, E., and Schaffer, A. A. 2010. Genetic diversity of Cucumis melo. Horticult. Rev. 36: 165–198.
- Bushnell, W. R. 2002. The role of powdery mildew research in understanding host-parasite interaction: past, present, and future. In The Powdery Mildews. A Comprehensive Treatise. Bélanger, R. R., Bushnell, W. R., Dik, A. J., and Carver, W. L. T., Eds. APS Press: St. Paul, MN, pp 1–12.
- Cadena-Iñiguez, J., Olguín-Hernández, G., Camacho-Tapia, M., Correia, K. C., Solano-Báez, A. R., Leyva-Mir, S. G., and Tovar-Pedraza, J. M. 2022. First Report of Neoerysiphe sechii causing powdery mildew on Sechium edule in San Luis Potosi, Mexico. Plant Dis. 106: 1069. doi:10.1094/PDIS-07-21-1432-PDN
- Caligiore-Gei, P. F., Della-Gaspera, P., Benitez, E., and Tarnowski, C. 2022. Cucurbit powdery mildew: first insights for the identification of the causal agent and screening for resistance of squash genotypes (Cucurbita moschata (Duchesne ex Lam.) Duchesne ex Poir.) in Mendoza, Argentina. Plant Pathol. J. 38: 296–303. doi:10.5423/PPJ.OA.01.2022.0002
- Cao, Y., Diao, Q., Chen, Y., Jin, H., Zhang, Y., and Zhang, H. 2020. Development of KASP markers and identification of a QTL underlying powdery mildew resistance in melon (Cucumis melo L.) by bulked segregant snalysis and RNA-Seq. Front. Plant Sci. 11: 593207. doi:10.3389/fpls.2020.593207
- Cao, Y., Diao, Q., Lu, S., Zhang, Y., and Yao, D. 2022. Comparative transcriptomic analysis of powdery mildew resistant and susceptible melon inbred lines to identify the genes involved in the response to Podosphaera xanthii infection. Sci. Hortic. 304: 111305. doi:10.1016/j.scienta.2022.111305
- Castagne, L. 1845. Catalogue des plantes qui croissant naturellement aux environs de Marseille. Imprimerie de Nicot et Pardigon, Pont-Moreau: France, p 263.
- Castagne, L. 1851. Supplément au catalogue des plantes qui croissent naturellement aux environs de Marseille. Imprimerie de Nicot et Pardigon, Pont-Moreau, France, p 125.
- Castanera, R., Ruggieri, V., Pujol, M., Garcia-Mas, J., and Casacuberta, J. M. 2019. An improved melon reference genome with single-molecule sequencing uncovers a recent burst of transposable elements with potential impact on genes. Front. Plant Sci. 10: 1815. doi:10.3389/fpls.2019.01815
- Castellanos-Morales, G., Paredes-Torres, L. M., Gamez, N., Hernandez-Rosales, H. S., Sanchez-de, l., Vega, G., Barrera-Redondo, J., Aguirre-Planter, E., Vazquez-Lobo, A., Montes-Hernandez, S., Lira-Saade, R., and Eguiarte, L. E. 2018. Historical biogeography and phylogeny of Cucurbita: Insights from ancestral area reconstruction and niche evolution. Mol. Phylogenet. Evol. 128: 38–54. doi:10.1016/j.ympev.2018.07.016
- Castellanos-Morales, G., Ruiz-Mondragón, K. Y., Hernández-Rosales, H. S., Sánchez-de la Vega, G., Gámez, N., Aguirre-Planter, E., Montes-Hernández, S., Lira-Saade, R., and Eguiarte, L. E. 2019. Tracing back the origin of pumpkins (Cucurbita pepo ssp. pepo L.) in Mexico. Proc. R Soc. B. 286: 20191440. doi:10.1098/rspb.2019.1440
- Cavagnaro, P. F., Senalik, D. A., Yang, L., Simon, P. W., Harkins, T. T., Kodira, C. D., Huang, S., and Weng, Y. 2010. Genome-wide characterization of simple sequence repeats in cucumber (Cucumis sativus L.). BMC Genomics 11: 569. doi:10.1186/1471-2164-11-569
- Chakravarty, H. L. 1990. Cucurbits of India and their role in the development of vegetable crops. In Biology and Utilization of Cucurbitaceae; Bates, D. M., Robinson, R. W., Jeffrey, C., Eds. Cornell University Press: Ithaca, New York, pp 325–334.
- Chen, B. H., Guo, W. L., Yang, H. L., Li, Q. F., Zhou, J. G., and Li, X. Z. 2020. Photosynthetic properties and biochemical metabolism of Cucurbita moschata genotypes following infection with powdery mildew. J. Plant Pathol. 102: 1021–1027. doi:10.1007/s42161-020-00564-9
- Chen, Y., Jing, X., Wang, S., Wang, J., Zhang, S., and Shi, Q. 2021b. Genome-wide analysis of WRKY transcription factor family in melon (Cucumis melo L.) and their response to powdery mildew. Plant Mol. Biol. Rep. 39: 686–699. doi:10.1007/s11105-020-01271-6
- Chen, J. F., and Kirkbride, J. 2000. A new synthetic species of Cucumis (Cucurbitaceae) from interspecific hybridization and chromosome. Brittonia 52: 315–319. doi:10.2307/2666583
- Chen, J. F., Staub, J. E., Tashiro, Y., Isshiki, S., and Miyazaki, S. 1997. Successful interspecific hybridization between Cucumis sativus L. and C. hystrix Chakr. Euphytica 96: 413–419. doi:10.1023/A:1003017702385
- Chen, J. F., Staub, J. E., Qian, C. T., Jiang, J. M., Luo, X. D., and Zhuang, F. Y. 2003. Reproduction and cytogenetic characterization of interspecific hybrids derived from Cucumis hystrix Chakr. to C. sativus L. Theor. Appl. Genet. 106: 688–695. doi:10.1007/s00122-002-1118-7
- Chen, Q., Yu, G., Wang, X., Meng, X., and Lv, C. 2021a. Genetics and resistance mechanism of the cucumber (Cucumis sativus L.) against powdery mildew. J. Plant Growth Regul. 40: 147–153. doi:10.1007/s00344-020-10075-7
- Cheng, H., Kong, W., Hou, D., Lv, J., and Tao, X. 2013. Isolation, characterization, and expression analysis of CmMLO2 in muskmelon. Mol. Biol. Rep. 40: 2609–2615. doi:10.1007/s11033-012-2347-8
- Cheng, H., Kong, W., and Lu, J. 2015. Analysis of powdery mildew resistance in wild melon MLO mutants. Hortic. Plant J. 1: 165–171.
- Cheng, H., Kun, W., Liu, D., Su, Y., and He, Q. 2012. Molecular cloning and expression analysis of CmMlo1 in melon. Mol. Biol. Rep. 39: 1903–1907. doi:10.1007/s11033-011-0936-6
- Cheng, J., Wei, L., Xiang, J., Zheng, T., and Wu, J. 2023. Tiangong Chuxin: an early maturing pumpkin-shaped grape cultivar. HortScience 58: 714–715. doi:10.21273/HORTSCI17134-23
- Chikh-Rouhou, H., Garcés-Claver, A., Kienbaum, L., Ben Belgacem, A., and Gómez-Guillamón, M. L. 2022. Resistance of Tunisian melon landraces to Podosphaera xanthii. Horticulturae 8: 1172. doi:10.3390/horticulturae8121172
- Cho, M. C., Heo, Y. C., Kim, J. S., Om, Y. H., Mok, I. G., Hong, K. H., and Pak, H. G. 2004a. A new oriental squash (Cucurbita moschata) cultivar Mansu, resistant to powdery mildew. Korean J. Breed. Sci. 36: 111–112.
- Cho, M. C., Hong, K. H., Park, H. G., and Heo, Y. C. 2004b. A new oriental squash (Cucurbita moschata) cultivar Chensu, resistant to powdery mildew. Korean J. Breed. Sci. 36: 113–114.
- Cho, M. C., Om, Y. H., Huh, Y. C., Mok, I. G., and Park, H. G. 2003. Two oriental squash varieties resistant to powdery mildew bred through interspecific crosses. Cucurbit Genet. Coop. Rep. 26: 40–41.
- Cho, M.-C., Om, Y.-H., Kim, D.-H., Heo, Y.-C., Kim, J.-S., and Park, H.-G. 2005. Breeding for powdery mildew resistant varieties in Cucurbita moschata. Res. Plant Dis. 11: 106–114. doi:10.5423/RPD.2005.11.2.106
- Cho, M. C., Om, Y. H., Huh, Y. C., Cheong, S. R., Kim, D. H., and Mok, I. G. 2009. Breeding of powdery mildew resistant squash 'Kwangmyeong'. Korean J. Hortic. Sci. Technol. 27: 332–335.
- Choi, I. Y., Ju, H. J., Lee, K. J., and Shin, H. D. 2021. First report of powdery mildew caused by Podosphaera xanthii on Benincasa hispida in Korea. Plant Dis. 105: 3757. doi:10.1094/PDIS-02-21-0253-PDN
- Choi, Y., Lee, J., Hwang, S., and Kim, H. 2020. PCR-based InDel marker associated with powdery mildew resistant MR-1. Agronomy 10: 1274. doi:10.3390/agronomy10091274
- Chomicki, G., and Renner, S. S. 2015. Watermelon origin solved with molecular phylogenetics including Linnaean material: another example of museomics. New Phytol. 205: 526–532. doi:10.1111/nph.13163
- Chomicki, G., Schaefer, H., and Renner, S. S. 2020. Origin and domestication of Cucurbitaceae crops: insights from phylogenies, genomics and archaeology. New Phytol. 226: 1240–1255. doi:10.1111/nph.16015
- Chung, S.-F., Staub, J. E., and Chen, J.-F. 2006. Molecular phylogeny of Cucumis species as revealed by consensus chloroplast SSR marker length and sequence variation. Genome 49: 219–229. doi:10.1139/g05-101
- Cieplak, M., Nucia, A., Ociepa, T., and Okoń, S. 2022. Virulence structure and genetic diversity of Blumeria graminis f. sp. avenae from different regions of Europe. Plants 11: 1358. doi:10.3390/plants11101358
- Clarke, A. C., Burtenshaw, M. K., McLenachan, P. A., Erickson, D. L., and Penny, D. 2006. Reconstructing the origins and dispersal of the Polynesian bottle gourd (Lagenaria siceraria). Mol. Biol. Evol. 23: 893–900. doi:10.1093/molbev/msj092
- Cohen, R., Burger, Y., and Katzir, N. 2004. Monitoring physiological races of Podosphaera xanthii (syn. Sphaerotheca fuliginea), the causal agent of powdery mildew in cucurbits: factors affecting race identification and the importance for research and commerce. Phytoparasitica 32: 174–183. doi:10.1007/BF02979784
- Cohen, R., Hanan, A., and Paris, H. S. 2003. Single-gene resistance to powdery mildew in zucchini squash (Cucurbita pepo). Euphytica 130: 433–441. doi:10.1023/A:1023082612420
- Cohen, R., Leibovich, G., Shtienberg, D., and Paris, H. S. 1993. Variability in the reaction of squash (Cucurbita pepo) to inoculation with Sphaerotheca fuliginea and methodology of breeding for resistance. Plant Pathol. 42: 510–516. doi:10.1111/j.1365-3059.1993.tb01530.x
- Cohen, Y., and Eyal, H. 1988. Pathogenicity of Erysiphe cichoracearum to cucurbits. Cucurbit Genet. Coop. Rep. 11: 87–90.
- Contin, M. 1978. Interspecific transfer of powdery mildew resistance in the genus Cucurbita. Ph.D. Thesis. Cornell University, Ithaca, New York.
- Contreras-Soto, R., Salvatierra, A., Maldonado, C., and Mashilo, J. 2021. The genetic diversity and population structure of different geographical populations of bottle gourd (Lagenaria siceraria) accessions based on genotyping-by-sequencing. Agronomy 11: 1677. doi:10.3390/agronomy11081677
- Corbaz, R., and Taillens, J. 1994. La tolérance des concombres à l´oïdium: une situation confuse. Revue suisse Vitic. Arboric. Hortic 26: 397–398.
- Crosby, K. M., Jifon, J. L., and Leskovar, D. I. 2008. 'Chujuc’, a new powdery mildew-resistant U.S. western-shipper melon with high sugar and β-carotene content. HortScience 43: 1904–1906. doi:10.21273/HORTSCI.43.6.1904
- Cui, H., Ding, Z., Fan, C., Zhu, L., Zhang, H., Gao, P., and Luan, F. 2020. Genetic mapping and nucleotide diversity of two powdery mildew resistance loci in melon (Cucumis melo). Phytopathology 110: 1970–1979.
- Cui, H., Ding, Z., Zhu, Q. L., Wu, Y., Qiu, B. Y., and Gao, P. 2020. Population structure, genetic diversity and fruit-related traits of wild and cultivated melons based on chloroplast genome. Genet. Resour. Crop Evol. 68: 1011–1021. doi:10.1007/s10722-020-01041-z
- Cui, H., Fan, C., Ding, Z., Wang, X., Tang, L., Bi, Y., Luan, F., and Gao, P. 2022a. CmPMRl and CmPMrs are responsible for resistance to powdery mildew caused by Podosphaera xanthii race 1 in melon. Theor. Appl. Genet. 135: 1209–1222. doi:10.1007/s00122-021-04025-4
- Cui, H., Zhu, Z., Ding, Z., Lv, Y., Sun, L., Luan, F., and Wang, X. 2021. First report of powdery mildew caused by Podosphaera xanthii race 1 on watermelon in China. J. Plant Pathol. 103: 1029–1029. doi:10.1007/s42161-021-00843-z
- Cui, L., Siskos, L., Wang, C., Schouten, H. J., Visser, R. G. F., and Bai, Y. 2022b. Breeding melon (Cucumis melo) with resistance to powdery mildew and downy mildew. Hortic. Plant J. 8: 545–561. doi:10.1016/j.hpj.2022.07.006
- Dane, F. E. 1991. Cytogenetics of the Genus Cucumis. In Chromosome Engineering in Plants: Genetics, Breeding, Evolution; Tsuchiya, T., and Gupta P. K., Eds. Springer: Cham, pp 201–214.
- Danin-Poleg, Y., Reis, N., Baudracco-Arnas, S., Pitrat, M., Staub, J. E., Oliver, M., Arus, P., de Vicente, C. M., and Katzir, N. 2000. Simple sequence repeats in Cucumis mapping and map merging. Genome 43: 963–974.
- Danin-Poleg, Y., Reis, N., Tzuri, G., and Katzir, N. 2001. Development and characterization of microsatelite markers in Cucumis. Theor. Appl. Genet. 102: 61–72. doi:10.1007/s001220051618
- Das, A., Singh, S., Islam, Z., Munshi, A. D., Behera, T. K., Dutta, S., Weng, Y., and Dey, S. S. 2022. Current progress in genetic and genomics-aided breeding for stress resistance in cucumber (Cucumis sativus L.). Sci. Hortic. 300: 111059. doi:10.1016/j.scienta.2022.111059
- Davis, A. R., Levi, A., Tetteh, A., Wehner, T., Russo, V., and Pitrat, M. 2007. Evaluation of watermelon and related species for resistance to race 1W powdery mildew. J. Amer. Soc. Hort. Sci. 132: 790–795. doi:10.21273/JASHS.132.6.790
- Davis, A. R., Levi, A., Wehner, T., and Pitrat, M. 2006. PI 525088-PMR, a melon race 1 powdery mildew-resistant watermelon line. HortScience 41: 1527–1528. doi:10.21273/HORTSCI.41.7.1527
- Dean, R., Van Kan, J. A. L., Pretorius, Z. A., Hammond-Kosack, K. E., Di Pietro, A., Spanu, P. D., Rudd, J. J., Dickman, M., Kahmann, R., Ellis, J., and Foster, G. D. 2012. The Top 10 fungal pathogens in molecular plant pathology. Mol. Plant Pathol. 13: 414–430. doi:10.1111/j.1364-3703.2011.00783.x
- De Candolle, A. P. 1805. (In: De Lamarck, J. B. and De Candolle, A. P.) Flore française, Ed 3. 2: 1–600. Paris.
- Decker-Walters, S. D., Staub, J. E., Chung, S.-M., Naata, E., and Quemada, H. D. 2002. Diversity in free-living populations of Cucurbita pepo (Cucurbitaceae) as assessed by random amplified polymorphic DNA. Syst. Bot. 27: 19–28.
- del Pino, D., Olalla, L., Pérez-García, A., Rivera, M. E., García, S., Moreno, R., de Vicente, A., and Torés, J. A. 2002. Occurrence of races and pathotypes of cucurbit powdery mildew in southeastern Spain. Phytoparasitica 30: 459–466. doi:10.1007/BF02979750
- De Miccolis Angelini, R. M., Pollastro, S., Rotondo, P. R., Laguardia, C., Abate, D., Rotolo, C., and Faretra, F. 2019. Transcriptome sequence resource for the cucurbit powdery mildew pathogen Podosphaera xanthii. Sci. Data 6: 95. doi:10.1038/s41597-019-0107-5
- Demidov, A. 1842. Voyage Dans La Russie Méridionale Et La Crimée, Par La Hongrie, La Valachie Et La Moldavie, 2. Ernest Bourdain and Co.: Paris.
- Dempewolf, H., Baute, G., Anderson, J., Kilian, B., Smith, C., and Guarino, L. 2017. Past and future use of wild relatives in crop breeding. Crop Sci. 57: 1070–1082. doi:10.2135/cropsci2016.10.0885
- de Ruiter, W., Hofstede, R., de Vries, J., and van den Heuvel, H. 2008. Combining QTL for resistance to CYSDV and powdery mildew in a single cucumber line. In Proceedings 9thEUCARPIA Meeting on Genetics and Breeding of Cucurbitaceae, May 21–24, 2008; Pitrat, M., Ed. INRA: Avignon, France, pp 181–188.
- Dey, S. S., Singh, A. K., Chandel, D., and Behera, T. K. 2006. Genetic diversity of bitter gourd (Momordica charantia L.) genotypes revealed by RAPD markers and agronomic traits. Sci. Hortic. 109: 21–28. doi:10.1016/j.scienta.2006.03.006
- Dhillon, N. P. S., Laenoi, S., Srimat, S., Pruangwitayakun, S., Mallappa, A., Kapur, A., Yadav, K. K., Hegde, G., Schafleitner, R., Schreinemachers, P., and Hanson, P. 2020b. Sustainable cucurbit breeding and production in Asia using public–private partnerships by the World Vegetable Center. Agronomy 10: 1171. doi:10.3390/agronomy10081171
- Dhillon, N. P. S., Masud, M. A. T., Pruangwitayakun, S., Natheung, M., Lertlam, S., and Jarret, R. L. 2020a. Evaluation of loofah lines for resistance to tomato leaf curl New Delhi virus and downy mildew, as well as key horticultural traits. Agriculture 10: 298. doi:10.3390/agriculture10070298
- Dhillon, N. P. S., Monforte, A. J., Pitrat, M., Pandy, S., Singh, P. K., Reitsma, K. R., Garcia-Mas, J., Sharma, A., and McCreight, J. D. 2012. Melon landraces of India: contributions and importance. Plant Breed. Rev. 35: 85–150.
- Dhillon, N. P. S., Sanguansil, S., Schafleitner, R., Wang, Y.-W., and McCreight, J. D. 2016a. Diversity among a wide Asian collection of bitter gourd landraces and their genetic relationships with commercial hybrid cultivars. J. Amer. Soc. Hort. Sci. 141: 475–484. doi:10.21273/JASHS03748-16
- Dhillon, N. P. S., Sanguansil, S., Singh, S. P., Masud, M. A. T., Kumar, P., and Bharathi, L. K. 2016b. Gourds: bitter, bottle, wax, snake, sponge and ridge. In Genetics and Genomics of the Cucurbitaceae; Grumet, R., Katzir, N., and Garcia-Mas, J., Eds. Springer Intl Pub AG: Cham, Switzerland, pp 155–172.
- Dhillon, N. P. S., Sanguansil, S., Srimat, S., Laenoi, S., Schafleitner, R., Pitrat, M., and McCreight, J. D. 2019. Inheritance of resistance to cucurbit powdery mildew in bitter gourd. HortScience 54: 1013–1016. doi:10.21273/HORTSCI13906-19
- Dhillon, N. P. S., Sanguansil, S., Srimat, S., Schafleitner, R., Manjunath, B., Agarwal, P., Xiang, Q., Masud, M. A., Myint, T., Hanh, N. T., Cuong, T. K., Balatero, C. H., Salutan-Bautista, V., Pitrat, M., Lebeda, A., and McCreight, J. D. 2018. Cucurbit powdery mildew-resistant bitter gourd breeding lines reveal four races of Podosphaera xanthii in Asia. HortScience 53: 337–341. doi:10.21273/HORTSCI12545-17
- Dijkhuizen, A., Kennard, W. C., Havey, M. J., and Staub, J. E. 1996. RFLP variation and genetic relationships in cultivated cucumber. Euphytica 90: 79–87. doi:10.1007/BF00025163
- Dogimont, C., and Sari, N. 2022. Gene list for melon. Cucurbit Genet. Coop. Rep. 45: 1–47.
- Dreiseitl, A. 2019. Great pathotype diversity and reduced virulence complexity in a Central European population of Blumeria graminis f. sp. hordei in 2015-2017. Eur. J. Plant Pathol. 153: 801–811. doi:10.1007/s10658-018-1593-6
- Dyche, G. H., and Kuijpers, R. J. H. 2013. New plant of Cucurbita genus e.g. Cucurbita maxima and Cucurbita moschata comprising a resistance to powdery mildew, useful for producing plants with resistance to powdery mildew. Patent Number(s): NL2009231-C. Patent Assignee: ENZA ZADEN BEHEER BV(ENZA-C). Derwent Primary Accession Number: 2013-M12581.
- Ellinger, D., Naumann, M., Falter, C., Zwikowics, C., Jamrow, T., Manisseri, C., Somerville, S. C., and Voigt, C. A. 2013. Elevated early callose deposition results in complete penetration resistance to powdery mildew in Arabidopsis. Plant Physiol. 161: 1433–1444. doi:10.1104/pp.112.211011
- El-Sayed, A. A., Mahmoud, S. H., and El-Mohamedy, R. S. R. 2019. Breeding some cantaloupe inbred lines for resistance to powdery mildew. Biosci. Res. 16: 777–792.
- Endl, J., Achigan-Dako, E. G., Pandey, A. K., Monforte, A. J., Pico, B., and Schaefer, H. 2018. Repeated domestication of melon (Cucumis melo) in Africa and Asia and a new close relative from India. Am. J. Bot. 105: 1662–1671. doi:10.1002/ajb2.1172
- Epinat, C., Pitrat, M., and Bertrand, F. 1993. Genetic analysis of resistance of 5 melon lines to powdery mildews. Euphytica 65: 135–144. doi:10.1007/BF00022575
- Erickson, D. L., Smith, B. D., Clarke, A. C., Sandweiss, D. H., and Tuross, N. 2005. An Asian origin for a 10,000-year-old domesticated plant in the Americas. Proc. Natl. Acad. Sci. U S A. 102: 18315–18320. doi:10.1073/pnas.0509279102
- Esquinas-Alcazar, J. T., and Gulick, P. J. 1983. Genetic Resources of Cucurbitaceae - a Global Report. International Board for Plant Genetic Resources (IBPGR): Rome, Italy.
- Fanourakis, N. E. 1984. Inheritance and linkage studies of the fruit epidermis structure and investigation of linkage relations of several traits and of meiosis in cucumber. Ph.D. Diss., University of Wisconsin: Madison, WI.
- Fazza, A. C., Dallagnol, L. J., Fazza, A. C., Monteiro, C. C., Lima, B. M. d., Wassano, D. T., and Camargo, L. E. A. 2013. Mapping of resistance genes to races 1, 3 and 5 of Podosphaera xanthii in melon PI 414723. Crop Breed. Appl. Biotechnol. 13: 349–355. doi:10.1590/S1984-70332013000400005
- Feng, Q., Xiao, L., He, Y., Liu, M., Wang, J., Tian, S., Zhang, X., and Yuan, L. 2021. Highly efficient, genotype-independent transformation and gene editing in watermelon (Citrullus lanatus) using a chimeric ClGRF4-GIF1 gene. J. Integr. Plant Biol. 63: 2038–2042. doi:10.1111/jipb.13199
- Filipowicz, N., and Renner, S. S. 2010. The worldwide holoparasitic Apodanthaceae confidently placed in the Cucurbitales by nuclear and mitochondrial gene trees. BMC Evol. Biol. 10: 219. doi:10.1186/1471-2148-10-219
- Filipowicz, N., Schaefer, H., and Renner, S. S. 2014. Revisiting Luffa (Cucurbitaceae) 25 years after C. Heiser: species boundaries and application of names tested with plastid and nuclear DNA sequences. Syst. Bot. 39: 205–215. doi:10.1600/036364414X678215
- Formisano, G., Paris, H. S., Frusciante, L., and Ercolano, M. R. 2010. Commercial Cucurbita pepo squash hybrids carrying disease resistance introgressed from Cucurbita moschata have high genetic similarity. Plant Genet. Res. 8: 198–203. doi:10.1017/S1479262110000183
- Fu, Y.B. 2017. The vulnerability of plant genetic resources conserved ex situ. Crop. Sci. 57: 2314–2328. doi:10.2135/cropsci2017.01.0014
- Hujieda, K., and Akiya, R. 1962. Inheritance of powdery mildew resistance and spine color on fruit in cucumber. J. Jpn. Soc. Hort. Sci. 31: 30–32. doi:10.2503/jjshs.31.30
- Fukino, N., Kunihisa, M., and Matsumoto, S. 2004. Characterization of recombinant inbred lines derived from crosses in melon (Cucumis melo L.), 'PMAR No. 5' × 'Harukei No. 3. Breed. Sci. 54: 141–145. doi:10.1270/jsbbs.54.141
- Fukino, N., Ohara, T., Monforte, A., Sugiyama, M., Sakata, Y., Kunihisa, M., and Matsumoto, S. 2008. Identification of QTLs for resistance to powdery mildew and SSR markers diagnostic for powdery mildew resistance genes in melon (Cucumis melo L.). Theor. Appl. Genet. 118: 165–175. doi:10.1007/s00122-008-0885-1
- Fukino, N., Yoshioka, Y., Sugiyama, M., Sakata, Y., and Matsumoto, S. 2013. Identification and validation of powdery mildew (Podosphaera xanthii) resistant loci in recombinant inbred lines of cucumber (Cucumis sativus L.). Mol. Breed. 32: 267–277. doi:10.1007/s11032-013-9867-3
- Gajdová, J., Lebeda, A., and Navrátilová, B. 2004. Protoplast cultures of Cucumis and Cucurbita spp. In Progress in Cucurbit Genetics and Breeding Research. Proceedings of Cucurbitaceae 2004, the 8th EUCARPIA Meeting on Cucurbit Genetics and Breeding; Lebeda, A., and Paris, H. S. Eds. Palacký University in Olomouc: Olomouc, Czech Republic, pp 441–454.
- Gao, Y. Y., Chen, H. M., Chen, D. Y., and Hao, G. F. 2023. Genetic and evolutionary dissection of melatonin response signaling facilitates the regulation of plant growth and stress responses. J. Pineal Res. 74:e12850. doi:10.1111/jpi.12850
- Garcia-Mas, J., Monforte, A. J., and Arús, P. 2004. Phylogenetic relationships among Cucumis species based on the ribosomal internal transcribed spacer sequence and microsatellite markers. Plant Syst. Evol. 248: 191–203. doi:10.1007/s00606-004-0170-y
- Garcia-Mas, J., Benjak, A., Sanseverino, W., Bourgeois, M., Mir, G., González, V. M., Hénaff, E., Câmara, F., Cozzuto, L., Lowy, E., Alioto, T., Capella-Gutiérrez, S., Blanca, J., Cañizares, J., Ziarsolo, P., Gonzalez-Ibeas, D., Rodríguez-Moreno, L., Droege, M., Du, L., Alvarez-Tejado, M., Lorente-Galdos, B., Melé, M., Yang, L., Weng, Y., Navarro, A., Marques-Bonet, T., Aranda, M. A., Nuez, F., Picó, B., Gabaldón, T., Roma, G., Guigó, R., Casacuberta, J. M., Arús, P., and Puigdomènech, P. 2012. The genome of melon (Cucumis melo L.). Proc. Natl. Acad. Sci. U S A. 109: 11872–11877. doi:10.1073/pnas.1205415109
- Gent, D. H., Claassen, B. J., Gadoury, D. M., Grünwald, N. J., Knaus, B. J., Radišek, S., Weldon, W., Wiseman, M. S., and Wolfenbarger, S. N. 2020. Population diversity and structure of Podosphaera macularis in the Pacific Northwestern United States and other populations. Phytopathology 110: 1105–1116. doi:10.1094/PHYTO-12-19-0448-R
- Ghebretinsae, A. G., Thulin, M., and Barber, J. C. 2007a. Relationships of cucumbers and melons unraveled: molecular phylogenetics of Cucumis and related genera (Benincaseae, Cucurbitaceae). Am. J. Bot. 94: 1256–1266. doi:10.3732/ajb.94.7.1256
- Ghebretinsae, A. G., Thulin, M., and Barber, J. C. 2007b. Nomenclatural changes in Cucumis (Cucurbitaceae). Novon 17: 176–178. doi:10.3417/1055-3177(2007)17[176:NCICC]2.0.CO;2
- Giuliani, C., Tani, C., and Bini, L. M. 2016. Micromorphology and anatomy of fruits and seeds of bitter melon (Momordica charantia L., Cucurbitaceae). Acta Soc. Bot. Pol. 85: 1–7.
- Glawe, D. A. 2008. The powdery mildews: a review of the world´s most familiar (yet poorly known) plant pathogens. Annu. Rev. Phytopathol. 46: 27–51. doi:10.1146/annurev.phyto.46.081407.104740
- Glazebrook, J. 2005. Contrasting mechanisms of defense against biotrophic and necrotrophic pathogens. Annu. Rev. Phytopathol. 43: 205–227. doi:10.1146/annurev.phyto.43.040204.135923
- Gong, L., Stift, G., Kofler, R., Pachner, M., and Lelley, T. 2008. Microsatellites for the Genus Cucurbita and an SSR-based Genetic Linkage Map of Cucurbita pepo L. Theor. Appl. Genet. 117: 37–48. doi:10.1007/s00122-008-0750-2
- Gong, L., Paris, H. S., Stift, G., Pachner, M., Vollmann, J., and Lelley, T. 2013. Genetic relationships and evolution in Cucurbita as viewed with simple sequence repeat polymorphisms: the centrality of C. okeechobeensis. Genet. Resour. Crop Evol. 60: 1531–1546. doi:10.1007/s10722-012-9940-5
- Gregorio-Cipriano, R., González, D., Félix-Gastélum, R., and Chacón, S. 2020. Neoerysiphe sechii (Ascomycota: Erysiphales): a new species of powdery mildew found on Sechium edule and Sechium mexicanum (Cucurbitaceae) in Mexico. Botany 98: 185–195. doi:10.1139/cjb-2019-0193
- Greuter, W., Burdet, H. M., Chaloner, W. G., Demoulin, V., Grolle, R., Hawksworth, D. L., Nicolson, D. H., Silva, P. C., Stafleu, F. A., Voss, E. G., and McNeill, J., Eds. 1988. International Code of Botanical Nomenclature Adopted by the Fourteenth International Botanical Congress, Berlin, July-August 1987. Regnum Vegetabile 118. Koeltz Scientific Books: Königstein.
- Grumet, R., Katzir, N., and Garcia-Mas, J. Eds. 2017. Genetics and Genomics of Cucurbitaceae. Springer International Publishing AG: Cham, Switzerland.
- Grumet, R., McCreight, J. D., McGregor, C., Weng, Y., Mazourek, M., Reitsma, K., Labate, J., Davis, A., and Fei, Z. 2021. Genetic resources and vulnerabilities of major cucurbit crops. Genes (Basel) 12: 1222. doi:10.3390/genes12081222
- Guner, N., and Wehner, T. C. 2003. Gene list for watermelon. Cucurbit Genet. Coop. Rep. 26: 76–92.
- Guo, W. L., Chen, B. H., Chen, X. J., Guo, Y. Y., Yang, H. L., Li, X. Z., and Wang, G. Y. 2018. Transcriptome profiling of pumpkin (Cucurbita moschata Duch.) leaves infected with powdery mildew. PLoS ONE 13:e0190175. doi:10.1371/journal.pone.0190175
- Guo, W. L., Chen, B. H., Guo, Y. Y., Yang, H. L., Mu, J. Y., Wang, G. Y., Li, X. Z., and Zhou, J. G. 2019. Improved powdery mildew resistance of transgenic Nicotiana benthamiana overexpressing the Cucurbita moschata CmSGT1 gene. Front. Plant Sci. 10: 955. doi:10.3389/fpls.2019.00955
- Guo, W. L., Chen, B. H., Guo, Y. Y., Chen, X. J., Li, Q. F., Yang, H. L., Li, X. Z., Zhou, J. G., and Wang, G. Y. 2020. Expression of pumpkin CmbHLH87 gene improves powdery mildew resistance in tobacco. Front. Plant Sci. 11: 163. doi:10.3389/fpls.2020.00163
- Guo, S., Zhang, J., Sun, H., Salse, J., Lucas, W. J., Zhang, H., Zheng, Y., Mao, L., Ren, Y., Wang, Z., Min, J., Guo, X., Murat, F., Ham, B.-K., Zhang, Z., Gao, S., Huang, M., Xu, Y., Zhong, S., Bombarely, A., Mueller, L. A., Zhao, H., He, H., Zhang, Y., Zhang, Z., Huang, S., Tan, T., Pang, E., Lin, K., Hu, Q., Kuang, H., Ni, P., Wang, B., Liu, J., Kou, Q., Hou, W., Zou, X., Jiang, J., Gong, G., Klee, K., Schoof, H., Huang, Y., Hu, X., Dong, S., Liang, D., Wang, J., Wu, K., Xia, Y., Zhao, X., Zheng, Z., Xing, M., Liang, X., Huang, B., Lv, T., Wang, J., Yin, Y., Yi, H., Li, R., Wu, M., Levi, A., Zhang, X., Giovannoni, J. J., Wang, J., Li, Y., Fei, Z., and Xu, Y. 2013. The draft genome of watermelon (Citrullus lanatus) and resequencing of 20 diverse accessions. Nat. Genet. 45: 51–58. doi:10.1038/ng.2470
- Guo, S., Zhao, S., Sun, H., Wang, X., Wu, S., Lin, T., Ren, Y., Gao, L., Deng, Y., Zhang, J., Lu, X., Zhang, H., Shang, J., Gong, G., Wen, C., He, N., Tian, S., Li, M., Liu, J., Wang, Y., Zhu, Y., Jarret, R., Levi, A., Zhang, X., Huang, S., Fei, Z., Liu, W., and Xu, Y. 2019. Resequencing of 414 cultivated and wild watermelon accessions identifies selection for fruit quality traits. Nat. Genet. 51: 1616–1623. doi:10.1038/s41588-019-0518-4
- Halliwell, R. S., Johnson, J. D., and Cotner, S. D. 1986. Susceptibility of squash cultivars to watermelon mosaic virus-1 and powdery mildew fungus. Texas Agric. Exp. Stat. Miscel. Public. 1617: 1–7.
- Heluta, V. P. 1988. Novi taksonomichni kombinatsyyi v rodini Erysiphaceae. Ukrayins’k Bot. Zhurn. 45: 62–63.
- Hammarlund, C. 1945. Beiträge zur Revision einiger imperfekter Mehltau-Arten. Erysiphe polyphaga nov. sp. Bot. Not. 1945: 101–108.
- Han, B. K., Rhee, S. J., Jang, Y. J., Sim, T. Z., Kim, Y. J., Park, T. S., and Lee, G. P. 2016. Identification of a causal pathogen of watermelon powdery mildew in Korea and development of a genetic linkage marker for resistance in watermelon (Citrullus lanatus). Hort. Sci. Technol. 34: 912–923. (in Korean) doi:10.12972/kjhst.20160095
- Hancock, J. F. 2004. Plant Evolution and the Origin of Crop Species, 2nd ed. CABI Publishing: Wallingford, UK.
- Harwood, R. R., and Markarian, D. 1968a. A genetic survey of resistance to powdery mildew in muskmelon. J. Heredity 59: 213–217. doi:10.1093/oxfordjournals.jhered.a107695
- Harwood, R. R., and Markarian, D. 1968b. The inheritance of resistance to powdery mildew in the cantaloupe variety Seminole. J. Heredity 59: 126–130. doi:10.1093/oxfordjournals.jhered.a107663
- He, X., Li, Y., Pandey, S., Yandell, B. S., Pathak, M., and Weng, Y. 2013. QTL mapping of powdery mildew resistance in WI 2757 cucumber (Cucumis sativus L.). Theor. Appl. Genet. 126: 2149–2161. doi:10.1007/s00122-013-2125-6
- He, Y., Wei, M., Yan, Y., Yu, C., Cheng, S., Sun, Y., Zhu, X., Wei, L., Wang, H., and Miao, L. 2022. Research advances in genetic mechanisms of major cucumber diseases resistance. Front. Plant Sci. 13: 862486. doi:10.3389/fpls.2022.862486
- Henning, M. J., Munger, H. M., and Jahn, M. M. 2005a. 'Hannah’s Choice F-1': a new muskmelon hybrid with resistance to powdery mildew, Fusarium race 2, and potyviruses. HortScience 40: 492–493. doi:10.21273/HORTSCI.40.2.492
- Henning, M. J., Munger, H. M., and Jahn, M. M. 2005b. 'PMR delicious 51': an improved open-pollinated melon with resistance to powdery mildew. HortScience 40: 261–262. doi:10.21273/HORTSCI.40.1.261
- Hirata, T., Cunnington, J. H., Paksiri, U., Limkaisang, S., Shishkoff, N., Grigaliunaite, B., Sato, Y., and Takamatsu, S. 2000. Evolutionary analysis of subsection Magnicellulatae of Podosphaera section Sphaerotheca (Erysiphales) based on the rDNA internal transcribed spacer sequences with special reference to host plants. Can. J. Bot. 78: 1521–1530. doi:10.1139/b00-124
- Hirata, T., and Takamatsu, S. 2001. Phylogeny and cross-infectivity of powdery mildew isolates (Podosphaera fuliginea s. lat.) on Cosmos and Cucumber. J. Gen. Plant Pathol. 67: 1–6. doi:10.1007/PL00012980
- Holdsworth, W. L., LaPlant, K. E., Bell, D. C., Jahn, M. M., and Mazourek, M. 2016. Cultivar-based introgression mapping reveals wild species-derived Pm-0, the major powdery mildew resistance locus in squash. PLoS ONE 11:e0167715. doi:10.1371/journal.pone.0167715
- Holliday, P. 1998. A Dictionary of Plant Pathology, 2nd ed. Cambridge University Press: Cambridge, UK.
- Hong, Y.-J., Hossain, M. R., Kim, H.-T., Park, J.-I., and Nou, I.-S. 2018. Identification of two new races of Podosphaera xanthii causing powdery mildew in melon in South Korea. Plant Pathol. J. 34: 182–190. doi:10.5423/PPJ.OA.12.2017.0261
- Hosoya, K., Kuzuya, M., Murakami, T., Kato, K., Narisawa, K., and Ezura, H. 2000. Impact of resistant melon cultivars on Sphaerotheca fuliginea. Plant Breed. 119: 286–288. doi:10.1046/j.1439-0523.2000.00489.x
- Howlader, J., Hong, Y., Natarajan, S., Sumi, K. R., Kim, H. T., Park, J. I., and Nou, I. S. 2020. Development of powdery mildew race 5-specific SNP markers in Cucumis melo L. using whole-genome resequencing. Hortic. Environ. Biotechnol. 61: 347–357. doi:10.1007/s13580-019-00217-6
- Hu, Y., Gao, Y. R., Yang, L. S., Wang, W., Wang, Y. J., and Wen, Y. Q. 2019. The cytological basis of powdery mildew resistance in wild Chinese Vitis species. Plant Physiol. Biochem. 144: 244–253. doi:10.1016/j.plaphy.2019.09.049
- Infante-Casella, M. L., Wyenandt, C. A., and Henninger, M. R. 2007. Evaluation of zucchini and yellow summer squash breeding lines and varieties for powdery mildew and downy mildew tolerance. HortScience 42: 433.
- Infante-Casella, M., Wyenandt, A., Maxwell, N., and Samulis, R. 2008. Evaluation of pumpkin cultivars for yield and powdery mildew tolerance. HortScience 43: 1255–1256.
- Iovieno, P., Andolfo, G., Schiavulli, A., Catalano, D., Ricciardi, L., Frusciante, L., Ercolano, M. R., and Pavan, S. 2015. Structure, evolution and functional inference on the Mildew Locus O (MLO) gene family in three cultivated Cucurbitaceae spp. BMC Genomics 16: 1112. doi:10.1186/s12864-015-2325-3
- Ito, M., and Takamatsu, S. 2010. Molecular phylogeny and evolution of subsection Magnicellulatae (Erysiphaceae: Podosphaera) with special reference to host plants. Mycoscience 51: 34–43. doi:10.1007/S10267-009-0005-3
- IUCN. 2023. The IUCN Red List of Threatened Species. Version 2022-2. https://www.iucnredlist.org (accessed Aug 9, 2023).
- Jaczewski, A. L. A. 1927. Karmannyi opredelitel’ gribov. II. Muchnisto-rosjanye griby. Leningrad, USSR, pp 1–491.
- Jagger, I. 1926. Powdery mildew of muskmelon in the Imperial Valley of California in 1925. Phytopathology 16: 1009–1010.
- Jagger, I. C., and Scott, G. W. 1937. Development of powdery mildew resistant cantaloupe No. 45. U.S. Dep. Agric. Circ. 441: 1–5.
- Jagger, I. C., Whitker, T. W., and Porter, D. R. 1938a. A new biolologic form of powdery milder on muskmelon in the Imperial Valley in California. Plant Dis. Rep. 22: 275–276.
- Jagger, I. C., Whitaker, T. W., and Porter, D. R. 1938b. Inheritance in Cucumis melo of resistance to powdery mildew (Erysiphe cichoracearum). Phytopathology 28: 761.
- Jahn, M., Munger, H. M., and McCreight, J. D. 2002. Breeding cucurbit crops for powdery mildew resistance. In The Powdery Mildews. A Comprehensive Treatise; Bélanger, R. R., Bushnell, W. R., Dik, A. J., and Carver, T. L. W., Eds. APS Press: St. Paul, MN, pp 239–248.
- Jeffrey, C. 1980. A review of the Cucurbitaceae. Bot. J. Linn. Soc 81: 233–247. doi:10.1111/j.1095-8339.1980.tb01676.x
- Jeffrey, C. 2005. A new system of Cucurbitaceae. Bot. Zhurn. 90: 332–335.
- Jensen, B. D. 2012. African watermelons and their uses. In Cucurbitaceae 2012. Proceedings of the Xth EUCARPIA Meeting on Genetics and Breeding of Cucurbitaceae; October 15-18, 2012, Antalya, Turkey; Sari, N., Solmaz, I., and Aras, V., Eds. Cukurova University: Antalya, Turkey, pp 264–272.
- Jiao, Z., Sun, J., Wang, C., Dong, Y., Xiao, S., Gao, X., Cao, Q., Li, L., Li, W., and Gao, C. 2018. Genome-wide characterization, evolutionary analysis of WRKY genes in Cucurbitaceae species and assessment of its roles in resisting to powdery mildew disease. PLoS ONE 13:e0199851. doi:10.1371/journal.pone.0199851a
- John, K. J., Scariah, S., Nissar, V. A. M., Latha, M., Gopalakrishnan, S., Yadav, S. R., and Bhat, K.V. 2013. On the occurrence, distribution, taxonomy and genepool relationship of Cucumis callosus (Rottler) Cogn., the wild progenitor of Cucumis melo L. from India. Genet. Resour. Crop Evol. 60: 1037–1046. doi:10.1007/s10722-012-9899-2
- John, K. J., Roy, Y. C., Krishnaraj, M. V., Nissar, V. A. M., Latha, M., and Bhat, K. V. 2017. Ecological and morphological characterisation of two rare and endemic wild edible Cucumis species (Cucurbitaceae) of Western Ghats of India. Genet. Resour. Crop Evol. 64: 149–158. doi:10.1007/s10722-015-0340-5
- Johnston, P. R., Quijada, L., Smith, C. A., Baral, H.-O., Hosoya, T., Baschien, C., Pärtel, K., Zhuang, W.-Y., Haelewaters, D., Park, D., Carl, S., López-Giráldez, F., Wang, Z., and Townsend, J. P. 2019. A multigene phylogeny toward a new phylogenetic classification of Leotiomycetes. IMA Fungus 10: 1.
- Jørgensen, I. H. 1992. Discovery, characterization and exploitation of Mlo powdery mildew resistance in barley. Euphytica 63: 141–152. doi:10.1007/BF00023919
- Jorkesh, A., Safaei, M., and Olfati, J.-A. 2018. Resistance of Cucurbita moschata and Cucurbita pepo lines to a powdery mildew. Inter. J. Veget. Sci. 24: 501–505. doi:10.1080/19315260.2018.1440273
- Joseph, J. K., and Antony, V. T. 2008. Ethnobotanical investigations in the genus Momordica L. in the southern Western Ghats of India. Genet. Resour. Crop Evol. 55: 713–721. doi:10.1007/s10722-007-9279-5
- Junell, L. 1966. A revision of Sphaerotheca communis ([Schlecht.] Fr.) Poll s.lat. Sven. Bot. Tidskr. 60: 365–392.
- Kable, P. F., and Ballantyne, B. J. 1963. Observations on the cucurbit powdery mildew in the Ithaca district. Plant Dis. Reptr. 47: 482.
- Karakurt, Y., Güvercin, D., Önder, S., and İşler, Ö. 2020. Assessment of genetic diversity in cucumber (Cucumis sativus L.) genotypes using morphological characters and AFLP analysis. KSU Tarim Ve Doga Dergici – KSU J. Agric. Nature 23: 577–585. doi:10.18016/ksutarimdoga.vi.583844
- Kaur, M., and Sharma, P. 2022. Recent advances in cucumber (Cucumis sativus L.). J. Hort. Sci. Biotech. 97: 3–23. doi:10.1080/14620316.2021.1945956
- Kates, H. R., Soltis, P. S., and Soltis, D. E. 2017. Evolutionary and domestication history of Cucurbita (pumpkin and squash) species inferred from 44 nuclear loci. Mol. Phylogenet. Evol. 111: 98–109. doi:10.1016/j.ympev.2017.03.002
- Keinath, A. P., Baccari, G. V., and DuBose, V. B. 2010. Controlling powdery mildew in the greenhouse on hybrid Cucurbita seedlings used as a rootstocks for grafting watermelon. Phytopathology 100:S60.
- Keinath, A. P., and DuBose, V. B. 2000. Evaluation of pumpkin cultivars for powdery and downy mildew resistance, virus tolerance, and yield. HortScience 35: 281–285. doi:10.21273/HORTSCI.35.2.281
- Keinath, A. P., and Hassel, R. 2014. Control of Fusarium wilt of watermelon by grafting onto bottle gourd or interspecific hybrid squash despite colonization of rootstocks by Fusarium. Plant Dis. 98: 1326–1332. doi:10.1094/PDIS-01-13-0100-RE
- Keinath, A. P., Wintermantel, W. M., and Zitter, T. A. Eds. 2017. Compendium of Cucurbit Diseases and Pests, 2nd ed. APS Press: St. Paul, MN.
- Kenigsbuch, D., and Cohen, Y. 1989. Independent inheritance of resistance to race-1 and race-2 of Sphaerotheca fuliginea in muskmelon. Plant Dis. 73: 206–208. doi:10.1094/PD-73-0206
- Kennard, W. C., Poetter, K., Dijkhuizen, A., Meglic, V., Staub, J. E., and Havey, M. J. 1994. Linkages among RFLP, RAPD, isozyme, disease-resistance, and morphological markers in marrow and wide crosses of cucumber. Theor. Appl. Genet. 89: 42–48. doi:10.1007/BF00226980
- Kesh, H., and Yadav, S. 2023. Recent advances in genetics and breeding of pumpkin (Cucurbita moschata Duch.). J. Hortic. Sci. Biotech. 98: 141–158. doi:10.1080/14620316.2022.2097961
- Khodaparast, S. A., Takamatsu, S., and Hedjaroude, G. A. 2001. Phylogenetic structure of the genus Leveillula (Erysiphales: Erysiphaceae) inferred from the nucleotide sequences of the rDNA ITS region with special reference to the L. taurica species complex. Mycol. Res. 105: 909–918. doi:10.1017/S0953756201004361
- Khodaparast, S. A., Takamatsu, S., Harada, M., Abbasi, M., and Samadi, S. 2012. Additional rDNA ITS sequences and its phylogenetic consequences for the genus Leveillula with emphasis on conidium morphology. Mycol. Progress 11: 741–752. doi:10.1007/s11557-011-0785-7
- Khodaparast, S. A., Takamatsu, S., Shadlou, A., Damadi, M., Pirnia, M., and Jahani, M. 2016. Notes on the genus Leveillula (Erysiphaceae): a new unrecorded species and notes on Leveillula infecting Ficus, Cucurbita and Tropaeolum in Iran. Phytotaxa 260: 267–275. doi:10.11646/phytotaxa.260.3.6
- Khoury, C. K., Carver, D., Kates, H. R., Achicanoy, H. A., van Zonneveld, M., Thomas, E., Heinitz, C., Jarret, R., Labate, J. A., Reitsma, K., Nabhan, G. P., and Greene, S. L. 2020. Distributions, conservation status, and abiotic stress tolerance potential of wild cucurbits (Cucurbita L.). Plants People Planet 2: 269–283. doi:10.1002/ppp3.10085
- Kim, H., Park, J., Ishikawa, T., Kuzuya, M., Horii, M., Yashiro, K., and Nou, I. 2015a. Development of molecular marker to select resistant lines and to differentiate the races related to powdery mildew in melon (Cucumis melo L.). J. Plant Biotechnol. 42: 284–289. doi:10.5010/JPB.2015.42.4.284
- Kim, H., Park, J., and Nou, I. 2016a. Identification of fungal races that cause powdery mildew in melon (Cucumis melo L.) and selection of resistant commercial melon cultivars against the identified races in Korea. J. Plant Biotechnol. 43: 58–65. doi:10.5010/JPB.2016.43.1.58
- Kim, H., Park, J., Robin, A. H. K., Ishikawa, T., Kuzuya, M., Horii, M., Yashiro, K., and Nou, I. 2016b. Identification of a new race and development of DNA markers associated with powdery mildew in melon. Plant Breed. Biotech. 4: 225–233. doi:10.9787/PBB.2016.4.2.225
- Kim, K. H., Ahn, S. G., Hwang, J. H., Choi, Y. M., Moon, H. S., and Park, Y. H. 2013. Inheritance of resistance to powdery mildew in the watermelon and development of a molecular marker for selecting resistant plants. Hortic. Environ. Biotechnol. 54: 134–140. doi:10.1007/s13580-013-0156-1
- Kim, K. H., Hwang, J. H., Han, D. Y., Park, M. K., Kim, S. G., Choi, D., Kim, Y. J., Lee, G. P., Kim, S. T., and Park, Y. H. 2015b. Major quantitative trait loci and putative candidate genes for powdery mildew resistance and fruit-related traits revealed by an intraspecific genetic map for watermelon (Citrullus lanatus var. lanatus). PLoS ONE 10:e0145665. doi:10.1371/journal.pone.0145665
- Kim, S. G., Ro, N.-Y., Hur, O.-S., Gwag, J.-G., Huh, Y.-C., Rhee, J.-H., Sung, J.-S., Jung, H. G., Kwon, T.-R., and Baek, H. J. 2014. Evaluation of powdery mildew resistance in Cucurbita spp. Korean J. Int. Agric. 26: 544–549. doi:10.12719/KSIA.2014.26.4.544
- Kirkbride, J. H. 1993. Biosystematic Monograph of the Genus Cucumis (Cucurbitaceae). Parkway Publishers: Boone, NC, pp 159.
- Kishor, D. S., Song, W. H., Noh, Y., Lee, G. P., Park, Y., Jung, J. K., Shim, E. J., and Chung, S. M. 2020. Development of SNP markers and validation assays in commercial Korean melon cultivars, using Genotyping-bysequencing and Fluidigm analyses. Sci. Hortic. 263: 109113. doi:10.1016/j.scienta.2019.109113
- Kiss, L., Vaghefi, N., Bransgrove, K., Dearnaley, J. D. W., Takamatsu, S., Tan, Y. P., Marston, C., Liu, S.-Y., Jin, D.-N., Adorada, D. L., Bailey, J., Cabrera de Álvarez, M. G., Daly, A., Dirchwolf, P. M., Jones, L., Nguyen, T. D., Edwards, J., Ho, W., Kelly, L., Mintoff, S. J. L., Morrison, J., Németh, M. Z., Perkins, S., Shivas, R. G., Smith, R., Stuart, K., Southwell, R., Turaganivalu, U., Váczy, K. Z., Blommestein, A. V., Wright, D., Young, A., and Braun, U. 2020. Australia: a continent without native powdery mildews? The first comprehensive catalog indicates recent introductions and multiple host range expansion events, and leads to the re-discovery of Salmonomyces as a new lineage of the Erysiphales. Front. Microbiol. 11: 1571. doi:10.3389/fmicb.2020.01571
- Kistler, L., Montenegro, A., Smith, B. D., Gifford, J. A., Green, R. E., Newsom, L. A., and Shapiro, B. 2014. Transoceanic drift and the domestication of African bottle gourds in the Americas. Proc. Natl. Acad. Sci. U S A. 111: 2937–2941. doi:10.1073/pnas.1318678111
- Kistler, L., Newsom, L. A., Ryan, T. M., Clarke, A. C., Smith, B. D., and Perry, G. H. 2015. Gourds and squashes (Cucurbita spp.) adapted to megafaunal extinction and ecological anachronism through domestication. Proc. Natl. Acad. Sci. U S A. 112: 15107–15112. doi:10.1073/pnas.1516109112
- Knerr, L. D., Staub, J. E., Holder, D. J., and May, B. P. 1989. Genetic diversity in Cucumis sativus L. assessed by at 18 allozyme coding loci. Theor. Appl. Genet. 78: 119–128. doi:10.1007/BF00299764
- Komínková, E., Dreiseitl, A., Malečková, E., Doležel, J., and Valárik, M. 2016. Genetic diversity of Blumeria graminis f. sp. hordei in Central Europe and its comparison with Australian population. PLoS ONE 11: e0167099. doi:10.1371/journal.pone.0167099
- Kooistra, E. 1968. Powdery mildew resistance in cucumber. Euphytica 17: 236–244. doi:10.1007/BF00021216
- Kooistra, E. 1971. Inheritance of flesh and skin colors in powdery mildew resistant cucumbers (Cucumis sativus L.). Euphytica 20: 521–523. doi:10.1007/BF00034206
- Kousik, C. S., Donahoo, R. S., Webster, C. G., Turechek, W. W., Adkins, S. T., and Roberts, P. D. 2011. Outbreak of cucurbit powdery mildew on watermelon fruit caused by Podosphaera xanthii in Southwest Florida. Plant Dis. 95: 1586–1586. doi:10.1094/PDIS-06-11-0521
- Kousik, C. S., and Ikerd, J. L. 2014. Evidence for cucurbit powdery mildew pathogen races based on watermelon differentials. In Cucurbitaceae 2014 Proceedings; Havey, M., Weng, Y., Day, B., and Grumet, R. Eds. ASHS: Alexandria, VA, pp 32–34.
- Kousik, C. S., Ikerd, J. L., Mandal, M. K., Adkins, S., Webster, C. G., and Turechek, W. W. 2018a. Powdery mildew–resistant bottle gourd germplasm lines: USVL351-PMR and USVL482-PMR. HortScience 53: 1224–1227. doi:10.21273/HORTSCI13067-18
- Kousik, C. S., Ikerd, J., Mandal, M., Adkins, S., and Turechek, W. W. 2018c. Watermelon germplasm lines USVL608-PMR, USVL255-PMR, USVL313-PMR, and USVL585-PMR with broad resistance to powdery mildew. HortScience 53: 1212–1217. doi:10.21273/HORTSCI12979-18
- Kousik, C. S., Ikerd, J. L., and Mandal, M. 2019. Relative susceptibility of commercial watermelon varieties to powdery mildew. Crop Protect. 125: 104910. doi:10.1016/j.cropro.2019.104910
- Kousik, C. S., Ikerd, J. L., Wechter, W. P., and Levi, A. 2014. Identification and development of multiple disease resistant (Phytophthora fruit rot and Powdery mildew) watermelon germplasm. HortScience S52: 49.
- Kousik, C. S., Levi, A., Ling, K. S., and Wechter, W. P. 2008. Potential sources of resistance to cucurbit powdery mildew in U.S. plant introductions of bottle gourd. HortScience 43: 1359–1364. doi:10.21273/HORTSCI.43.5.1359
- Kousik, C. S., Mandal, M., and Hassel, R. 2018b. Powdery mildew resistant rootstocks that impart tolerance to grafted susceptible watermelon scion seedlings. Plant Dis. 102: 1290–1298. doi:10.1094/PDIS-09-17-1384-RE
- Krawinkel, M. B., and Keding, G.B. 2006. Bitter gourd (Momordica charantia): a dietary approach to hyperglycemia. Nutr. Rev. 64: 331–337. doi:10.1301/nr.2006.jul.331-337
- Křístková, E., and Lebeda, A. 1997. Possibilities of exploration of wild Cucurbita species in the breeding via interspecific hybridization. Zahradnictví (Hort. Sci.) 24: 113–120.
- Křístková, E., and Lebeda, A. 1998. Multiple disease resistance in genetic resources of Cucurbita pepo and C. maxima. Acta Hortic. et Regiotect. 1: 209–210.
- Křístková, E., and Lebeda, A. 1999a. Powdery mildew of cucurbits in the Czech Republic – species, pathotype and race spectra. The First International Powdery Mildew Conference, Programme and Abstracts, Avignon, France, pp 14–15.
- Křístková, E., and Lebeda, A. 1999b. Searching of Cucumis sativus L. genetic resources for field resistance to powdery mildew of cucurbits. Acta Hortic. 492: 371–376. doi:10.17660/ActaHortic.1999.492.51
- Křístková, E., and Lebeda, A. 1999c. Vliv ontogenetického stadia a habitu rostlin Cucurbita pepo L. na polní odolnost k padlí tykvovitých (Influence of developmental stage and plant habit of Cucurbita pepo L. genotypes on their field resistance to the powdery mildew of cucurbits). Zahradnictví (Hort. Sci.) 26: 19–24. (In Czech with English Summary)
- Křístková, E., and Lebeda, A. 1999d. Disease resistance of Cucurbita pepo and C. maxima genetic resources. Cucurbit Genet. Coop. Rep. 22: 53–54.
- Křístková, E., and Lebeda, A. 2000a. Powdery mildew field infection on leaves and stems of Cucurbita pepo accessions. Acta Hortic. 510: 61–66. doi:10.17660/ActaHortic.2000.510.9
- Křístková, E., and Lebeda, A. 2000b. Citrullus lanatus – a potential host of powdery mildew in the Czech Republic. Cucurbit Genet. Coop. Rep. 23: 46–48.
- Křístková, E., and Lebeda, A. 2001. Aggressiveness of powdery mildew isolates on Cucurbita maxima. Cucurbit Genet. Coop. Rep. 24: 73–76.
- Křístková, E., Lebeda, A., and Katovská, J. 2002. Response of Cucumis melo genotypes MR-1 and PI 124112 to Czech isolates of cucurbit powdery mildew. Acta Hortic. 588: 181–184. doi:10.17660/ActaHortic.2002.588.28
- Křístková, E., Lebeda, A., and Sedláková, B. 2004. Virulence of Czech cucurbit powdery mildew isolates on Cucumis melo genotypes MR-1 and PI 124112. Sci. Hortic. 99: 257–265. doi:10.1016/S0304-4238(03)00105-5
- Křístková, E., Lebeda, A., and Sedláková, B. 2009. Species spectra, distribution and host range of cucurbit powdery mildews in the Czech Republic, and in some other European and Middle Eastern countries. Phytoparasitica 37: 337–350. doi:10.1007/s12600-009-0045-4
- Kusch, S., and Panstruga, R. 2017. Mlo–based resistance: an apparently universal "weapon“ to defeat powdery mildew disease. Mol. Plant. Microbe Interact. 30: 179–189. doi:10.1094/MPMI-12-16-0255-CR
- Kuzuya, M., Hosoya, K., Yashiro, K., Tomita, K., and Ezura, H. 2003. Powdery mildew (Sphaerotheca fuliginea) resistance in melon is selectable at the haploid level. J. Exp. Bot. 54: 1069–1074. doi:10.1093/jxb/erg100
- Kuzuya, M., Yashiro, K., Tomita, K., and Ezura, H. 2006. Powdery mildew (Podosphaera xanthii) resistance in melon is categorized into two types based on inhibition of the infection processes. J. Exp. Bot. 57: 2093–2100. doi:10.1093/jxb/erj166
- Labo, A. U., and Khan, A. A. 2019. Incidence of powdery mildew on cucurbit plants in Katsina, Nigeria. J. Phytol. 11: 5–9.
- Laghetti, G., and Hammer, K. 2007. The Corsican citron melon (Citrullus lanatus (Thunb.) Matsum. et Nakai subsp. lanatus var. citroides (Bailey) Mansf. ex Greb.) a traditional and neglected crop. Genet. Resour. Crop Evol. 54: 913–916. doi:10.1007/s10722-007-9220-y
- Lai, W., Zhu, C., Yang, S., Hu, Z., Liu, S., and Zhou, Y. 2022. Comprehensive identification of the VQ family genes in cucumber and their roles in response to abiotic and biotic stresses. Sci. Hortic. 295: 110874. doi:10.1016/j.scienta.2021.110874
- LaPlant, K. E., Wyatt, L. E., Moriarty, G., Fink-Brodnicki, M., Jahn, M., and Mazourek, M. 2016. Powdery mildew–resistant pumpkin inbred lines. HortScience 51: 1297–1300. doi:10.21273/HORTSCI10866-16
- Lebeda, A. 1983. The genera and species spectrum of cucumber powdery mildew in Czechoslovakia. Phytopath. Z. 108: 71–79. doi:10.1111/j.1439-0434.1983.tb00565.x
- Lebeda, A. 1984a. A contribution to the general theory of host-parasite specificity. Phytopath. Z. 110: 226–234. doi:10.1111/j.1439-0434.1984.tb00753.x
- Lebeda, A. 1984b. Screening of wild Cucumis species for resistance to cucumber powdery mildew (Erysiphe cichoracearum and Sphaerotheca fuliginea). Sci. Hortic. 24: 241–249. doi:10.1016/0304-4238(84)90107-9
- Lebeda, A. 1986. Padlí okurkové (Cucumber powdery mildew), Erysiphe cichoracearum, Sphaerotheca fuliginea. In Metody Testování Rezistence Zelenin Vůči Rostlinným Patogenům (Methods of Testing Vegetable Crops for Resistance to Plant Pathogens); Lebeda, A., Ed. VHJ Sempra, VŠÚZ Olomouc: Olomouc, Czechoslovakia, pp 87–91.
- Lebeda, A., and Burdon, J. J. 2023. Studying wild plant pathosystems to understand crop plant pathosystems: status, gaps, challenges and perspectives. Phytopathology 113: 365–380. doi:10.1094/PHYTO-01-22-0018-PER
- Lebeda, A., and Cohen, Y. 2011. Cucurbit downy mildew (Pseudoperonospora cubensis) – biology, ecology, epidemiology, host-pathogen interaction and control. Eur. J. Plant Pathol. 129: 157–192. doi:10.1007/s10658-010-9658-1
- Lebeda, A., Kitner, M., Mieslerová, B., Křístková, E., and Pavlíček, T. 2019. The occurrence of Leveillula lactucae-serriolae on Lactuca serriola in Jordan. Phytopathol. Mediter. 58: 359–367.
- Lebeda, A., and Křístková, E. 1994. Field resistance of Cucurbita species to powdery mildew (Erysiphe cichoracearum). J. Plant Dis. Prot. 101: 598–603.
- Lebeda, A., and Křístková, E. 1996a. Genotypic variation in field resistance of Cucurbita pepo assortment to powdery mildew (Erysiphe cichoracearum). Genet. Resour. Crop Evol. 43: 79–84. doi:10.1007/BF00126944
- Lebeda, A., and Křístková, E. 1996b. Variation in Cucurbita ssp. for field resistance to powdery mildew. In Cucurbits Towards 2000 - Proceedings of the VIth Eucarpia Meeting on Cucurbit Genetics and Breeding, Málaga, Spain, 28-30 May 1996; Gómez-Guillamón, M. L., Soria, C., Cuartero, J., Torés, J. A., and Fernández-Muňoz, R. Eds. Estación Experimental "La Mayora", C.S.I.C.: Málaga, Spain, pp 235–240.
- Lebeda, A., and Křístková, E. 1997. Evaluation of Cucumis sativus L. germplasm for field resistance to the powdery mildew. Acta Phytopath. Entom. Hungarica 32: 299–305.
- Lebeda, A., and Křístková, E. 2000. Interaction between morphotypes of Cucurbita pepo and obligate biotrophs (Pseudoperonospora cubensis, Erysiphe cichoracearum and Sphaerotheca fuliginea). Acta Hortic. 510: 219–226. doi:10.17660/ActaHortic.2000.510.35
- Lebeda, A., Křístková, E., and Doležal, K. 1999. Peroxidase isozyme polymorphism in Cucurbita pepo cultivars with various morphotypes and different level of field resistance to powdery mildew. Sci. Hortic. 81: 103–112. doi:10.1016/S0304-4238(98)00261-1
- Lebeda, A., Křístková, E., Kitner, M., Mieslerová, B., Jemelková, M., and Pink, D. A. C. 2014. Wild Lactuca species, their genetic diversity, resistance to diseases and pests, and exploitation in lettuce breeding. Eur. J. Plant Pathol. 138: 597–640. doi:10.1007/s10658-013-0254-z
- Lebeda, A., Křístková, E., Roháčková, J., Sedláková, B., Widrlechner, M. P., and Paris, H. S. 2016c. Race-specific resistance of Cucurbita germplasm to Pseudoperonospora cubensis. Euphytica 212: 145–156. doi:10.1007/s10681-016-1783-2
- Lebeda, A., Křístková, E., Sedláková, B., Coffey, M. D., and McCreight, J. D. 2011. Gaps and perspectives of pathotype and race determination in Golovinomyces cichoracearum and Podosphaera xanthii. Mycoscience 52: 159–164. doi:10.1007/S10267-010-0098-8
- Lebeda, A., Křístková, E., Sedláková, B., Kitner, M., and Widrlechner, M. P. 2019. Status of research, breeding and protection of cucurbits in relation to cucurbit downy mildew: their limits and perspectives. Acta Hortic. 1242: 421–426. doi:10.17660/ActaHortic.2019.1242.60
- Lebeda, A., Křístková, E., Sedláková, B., McCreight, J. D., and Coffey, M. D. 2016a. Cucurbit powdery mildews: methodology for objective determination and denomination of races. Eur. J. Plant Pathol. 144: 399–410. doi:10.1007/s10658-015-0776-7
- Lebeda, A., Křístková, E., Sedláková, B., and McCreight, J. D. 2016b. Initiative for international cooperation of researchers and breeders related to determination and denomination of cucurbit powdery mildew races. In Proceedings of Cucurbitaceae 2016, The XIth Eucarpia Meeting on Cucurbit Genetics & Breeding, July 24-28, 2016, Warsaw, Poland; Kozik, E. U. and Paris, H. S. Eds. Wydawnictvo SIGMA Sp. J.: Skierniewice, Poland, pp 148–152.
- Lebeda, A., Křístková, E., Sedláková, B., and McCreight, J. D. 2018b. Initiative for uniform cucurbit powdery mildew race determination and denomination: status of race differentials. Cucurbit Genet. Coop. Rep. 41: 17–19.
- Lebeda, A., Křístková, E., Sedláková, B., McCreight, J. D., and Coffey, M. D. 2008. New concept for determination and denomination of pathotypes and races of cucurbit powdery mildew. In Proceedings of Cucurbitaceae 2008, IXth EUCARPIA Meeting on Genetics and Breeding of Cucurbitaceae, Pitrat, M. Ed. INRA: Avignon, France, pp 125–134.
- Lebeda, A., Křístková, E., Sedláková, B., McCreight, J. D., and Kosman, E. 2018a. Virulence variation of cucurbit powdery mildews in the Czech Republic – population approach. Eur. J. Plant Pathol. 152: 309–326. doi:10.1007/s10658-018-1476-x
- Lebeda, A., Navrátilová, B., Kubaláková, M., Doležal, K., Křístková, E., Doležel, J., and Lysák, M. 1999. Morphological and physiological characteristics of plants issued from an interspecific hybridization of Cucumis sativus × Cucumis melo. Acta Hortic. 492: 149–156. doi:10.17660/ActaHortic.1999.492.17
- Lebeda, A., McGrath, M. T., and Sedláková, B. 2010. Fungicide resistance in cucurbit powdery mildew fungi. In Fungicides; Carisse, O. Ed. InTech: Rijeka, Croatia, pp 221–246.
- Lebeda, A., Mieslerová, B., Huszár, J., and Sedláková, B. 2017. Padlí Kulturních a Planě Rostoucích Rostlin. Taxonomie, Biologie, Ekologie a Epidemiologie, Mechanismy Rezistence, Šlechtění Na Odolnost, Metody Experimentální Práce, Diagnostika a Ochrana Rostlin (Powdery Mildews of Crop and Wild Plants. Taxonomy, Biology, Ecology and Epidemiology, Mechanisms of Resistance, Breeding for Resistance, Methods of Experimental Work, Diagnostics and Plant Protection). Vydavatelství Agriprint, Olomouc, p 368.
- Lebeda, A., and Sedláková, B. 2004. Disease impact and pathogenicity variation in Czech populations of cucurbit powdery mildews. In Progress in Cucurbit Genetics and Breeding Research; Proceedings Cucurbitaceae 2004: 8th EUCARPIA Meeting on Cucurbit Genetics and Breeding; Lebeda, A., and Paris, H. S. Eds. Palacký University in Olomouc: Olomouc, Czech Republic, pp 281–287.
- Lebeda, A., and Sedláková, B., 2006. Identification and survey of cucurbit powdery mildew races in Czech populations. In Proceedings Cucurbitaceae 2006; Holmes, G. J. Ed. Universal Press: Raleigh, NC, pp 444–452.
- Lebeda, A., and Sedláková, B. 2010. Screening for resistance to cucurbit powdery mildews (Golovinomyces cichoracearum, Podosphaera xanthii). In Mass Screening Techniques for Selecting Crops Resistant to Disease; Spencer, M. M. and Lebeda, A. Eds. International Atomic Energy Agency (IAEA): Vienna, Austria, pp 295–307.
- Lebeda, A., Sedláková, B., and Křístková, E. 2004. Distribution, harmfulness and pathogenic variability of cucurbit powdery mildew in the Czech Republic. Acta Fytotech. Zootech 7: 174–176.
- Lebeda, A., Sedláková, B., and Křístková, E. 2007b. Temporal changes in pathogenicity structure of cucurbit powdery mildews populations. Acta Hortic. 731: 381–388. doi:10.17660/ActaHortic.2007.731.53
- Lebeda, A., Sedláková, B., Křístková, E., McCreight, J. D., den Hertog, M., and Reitsma, K. 2021b. Development and availability of melon differential set for determination of virulence variation of cucurbit powdery mildews (Podosphaera xanthii and Golovinomyces orontii). Cucurbit Genet. Coop. Rep. 44: 15–19.
- Lebeda, A., Sedláková, B., Křístková, E., and Vysoudil, M. 2009. Long-lasting changes in the species spectrum of cucurbit powdery mildew in the Czech Republic – influence of air temperature changes or random effect? In Climate Change and Plant Pathogens, Pests and Weeds; Pokorný, R., and Lebeda, A. Eds. Plant Prot. Sci. 45:S41–S47. doi:10.17221/2807-PPS
- Lebeda, A., Sedláková, B., Křístková, E., Widrlechner, M. P., and Kosman, E. 2021a. Understanding pathogen population structure and virulence variation for efficient resistance breeding to control cucurbit powdery mildews. Plant Pathol. 70: 1364–1377. doi:10.1111/ppa.13379
- Lebeda, A., Widrlechner, M. P., Staub, J., Ezura, H., Zalapa, J., and Křístková, E. 2007a. Cucurbits (Cucurbitaceae; Cucumis spp., Cucurbita spp., Citrullus spp.), Chapter 8. In Genetic Resources, Chromosome Engineering, and Crop Improvement Series; Volume 3–Vegetable Crops; Singh, R. Ed. CRC Press: Boca Raton, FL, pp 271–376.
- Lee, J. H., Jang, K. S., Lee, W. J., Choi, Y. H., and Choi, G. J. 2014. Resistance of Cucurbits to Podosphaera xanthii race 1. Hort. Sci. Technol. 32: 673–683. (in Korean) doi:10.7235/hort.2014.14027
- Lee, H. Y., Kim, J. G., Kang, B. C., and Song, K. W. 2020. Assessment of the genetic diversity of the breeding lines and a genome wide association study of three horticultural traits using worldwide cucumber (Cucumis spp.) germplasm collection. Agronomy 10: 1736. doi:10.3390/agronomy10111736
- Leibovich, G., Cohen, R., and Paris, R. S. 1996. Shading of plants facilitates selection for powdery mildew resistance in squash. Euphytica 90: 289–292. doi:10.1007/BF00027478
- Levi, A., Jarret, R., Kousik, S., Wechter, P., Nimmakayala, P., and Reddy, U. K. 2017a. Genetic resources of watermelon. In Genetics and Genomics of Cucurbitaceae; Grumet, R., Katzir, N., Garcia-Mas, J. Eds. Springer International Publishing AG: Cham, Switzerland, pp 87–110.
- Levi, A., Simmons, A. M., Massey, L., Coffey, J., Wechter, W. P., Jarret, R. L., Tadmor, Y., Nimmakayala, P., and Reddy, U. K. 2017b. Genetic diversity in the desert watermelon Citrullus colocynthis and its relationship with Citrullus species as determined by high-frequency oligonucleotides-targeting active gene markers. J. Amer. Soc. Hort. Sci. 142: 47–56. doi:10.21273/JASHS03834-16
- Levi, A., Thies, J. A., Wechter, W. P., Harrison, H. F., Simmons, A. M., Reddy, U. K., Nimmakayala, P., and Fei, Z. 2013. High frequency oligonucleotides: targeting active gene (HFO–TAG) markers revealed wide genetic diversity among Citrullus spp. accessions useful for enhancing disease or pest resistance in watermelon cultivars. Genet. Resour. Crop Evol. 60: 427–440. doi:10.1007/s10722-012-9845-3
- Levi, A., Thomas, C. E., Keinath, A. P., and Wehner, T. C. 2000. Estimation of genetic diversity among Citrullus accessions using RAPD markers. Acta Hortic. 510: 385–390. doi:10.17660/ActaHortic.2000.510.61
- Levi, A., Thomas, C. E., Keinath, A. P., and Wehner, T. C. 2001a. Genetic diversity among watermelon (Citrullus lanatus and Citrullus colocynthis) accessions. Genet. Resour. Crop Evol. 48: 559–566. doi:10.1023/A:1013888418442
- Levi, A., Thomas, C. E., Wehner, T. C., and Zhang, X. 2001b. Low genetic diversity indicates the need to broaden the genetic base of cultivated watermelon. HortScience 36: 1096–1101. doi:10.21273/HORTSCI.36.6.1096
- Li, B., Zhao, Y., Zhu, Q., Zhang, Z., Fan, C., Amanullah, S., Gao, P., and Luan, F. 2017. Mapping of powdery mildew resistance genes in melon (Cucumis melo L.) by bulked segregant analysis. Sci. Hortic. 220: 160–167. doi:10.1016/j.scienta.2017.04.001
- Lija, M., and Beevy, S. S. 2021. A review on the diversity of Melon. Plant Sci. Today 8: 995–1003. doi:10.14719/pst.1300
- Lira, R., Eguiarte, L., Montes, S., Zizumbo-Villarreal, D., Marín, P. C.-G., and Quesada, M. 2016. Homo sapiens–Cucurbita interaction in Mesoamerica: domestication dissemination, and diversification. In Ethnobotany of Mexico, Lira, R., Casas, A., and Blancas, J. Eds. Springer: New York, NY, pp 389–401.
- Liu, L. Z., Chen, Y. Y., Su, Z. H., Zhang, H., and Zhu, W. M. 2010. A sequence-amplified characterized region marker for a single, dominant gene in melon PI 134198 that confers resistance to a unique race of Podosphaera xanthii in China. HortScience 45: 1407–1410. doi:10.21273/HORTSCI.45.9.1407
- Liu, Z., Jiang, Y., Yang, X., Deng, X., Dang, J., Wang, Z., Yusop, M. R., and Abdullah, S. 2022. Characteristics of interspecific hybridization and inbred progeny of pumpkin (Cucurbita moschata Duch.) and winter squash (Cucurbita maxima Duch.). Horticulturae 8: 596. doi:10.3390/horticulturae8070596
- Liu, C. M., Li, X. L., Yang, R. P., Mo, Y. L., Wang, Y. Q., Xian, F., Zhang, X., and Wan, F. 2014. The protective roles of S-adenosylmethionine decarboxylase (SAMDC) gene in melon resistance to powdery mildew infection. Hortic. Environ. Biotechnol. 55: 557–567. doi:10.1007/s13580-014-0026-5
- Liu, P. N., Miao, H., Lu, H. W., Cui, J. Y., Tian, G. L., Wehner, T. C., Gu, X. F., and Zhang, S. P. 2017. Molecular mapping and candidate gene analysis for resistance to powdery mildew in Cucumis sativus stem. Genet. Mol. Res. 16:gmr16039680. doi:10.4238/gmr16039680
- Liu, L., Yuan, X., Cai, R., Pan, J., and Zhu, L. 2008. Quantitative Trait Loci for resistance to powdery mildew in cucumber under seedling spray inoculation and leaf disc infection. J Phytopathol 156: 691–697.
- Liu, X., Gu, X., Lu, H., Liu, P., Miao, H., Bai, Y., and Zhang, S. 2021. Identification of novel loci and cadidate genes for resistance to powdery mildew in a resequenced cucumber germplasm. Genes 12: 584. doi:10.3390/genes12040584
- Lombello, R.A. 2020. Cytogenetical Analysis of Bitter Gourd Genome. In The Bitter Gourd Genome; Kole, C., Matsumura, H., and Behera, T. Eds. Springer: Cham, Switzerland, pp 61–72.
- Longzhou, L., Xiaojun, Y., Run, C., Junsong, P., Huanle, H., Lihua, Y., Yuan, G., and Lihuang, Z. 2008. Quantitative trait loci for resistance to powdery mildew in cucumber under seedling spray inoculation and leaf disc infection. J. Phytopathol. 156: 691–697. doi:10.1111/j.1439-0434.2008.01427.x
- López-Martín, M., Pérez-de-Castro, A., Picó, B., and Gómez-Guillamón, M. L. 2022. Advanced genetic studies on powdery mildew resistance in TGR-1551. Int. J. Mol. Sci. 23: 12553. doi:10.3390/ijms232012553
- Luhová, L., Jančová, D., Frébort, I., Lebeda, A., Šebela, M., Křístková, E., and Peč, P. 1999. Amine oxidase, peroxidase, catalase and acid phosphatase activities in powdery mildew infected plants of Cucumis sativus. Phyton 39: 235–241.
- Luitel, B. P., Kim, S. G., Sung, J. S., Hur, O. S., Yoon, M. S., Rhee, J. H., Baek, H. J., Ryu, K. Y., and Ko, H. C. 2016. Screening of pumpkin (Cucurbita spp.) germplasm for resistance to powdery mildew at various stages of seedlings growth. Res. Plant Sci. 22: 133–144. doi:10.5423/RPD.2016.22.3.133
- Lv, J., Qi, J., Shi, Q., Shen, D., Zhang, S., Shao, G., Li, H., Sun, Z., Weng, Y., Shang, Y., Gu, X., Li, X., Zhu, X., Zhang, J., van Treuren, R., van Dooijeweert, W., Zhang, Z., and Huang, S. 2012. Genetic diversity and population structure of cucumber (Cucumis sativus L.). PLoS ONE 7:e46919. doi:10.1371/journal.pone.0046919
- Mandal, M. K., Chanda, B., and Kousik, C. S. 2021. Identification of powdery mildew resistant marker in watermelon by metabolomics and genomics approach. Phytopathology 10:S26.
- Mandal, M. K., Suren, H., and Kousik, C. 2020. Elucidation of resistanc signaling and identification of powdery mildew resistant mapping loci (ClaPMR2) during watermelon-Podosphaera xanthii interaction using RNA-Seq and whole-genome resequencing approach. Sci. Rep. 10: 14038. doi:10.1038/s41598-020-70932-z
- Mandal, M. K., Suren, H., Ward, B., Boroujerdi, A., and Kousik, C. 2018. Differential roles of melatonin in plant-host resistance and pathogen suppression in cucurbits. J. Pineal Res. 65:e12505. doi:10.1111/jpi.12505
- Mapuranga, J., Chang, J., and Yang, W. 2022. Combating powdery mildew: Advances in molecular interactions between Blumeria graminis f. sp. tritici and wheat. Front. Plant Sci. 13: 1102908. doi:10.3389/fpls.2022.1102908
- Margaritopoulou, T., Kizis, D., Kotopoulis, D., Papadakis, I. E., Anagnostopoulos, C., Baira, E., Termentzi, A., Vichou, A.-E., Leifert, C., and Markellou, E. 2022. Enriched H3K4me3 marks at Pm-0 resistance-related genes prime courgette against Podosphaera xanthii. Plant Physiol. 188: 576–592. doi:10.1093/plphys/kiab453
- Marr, K. L., Bhattarai, N. K., and Xia, Y. M. 2005. Allozymic, morphological, and phenological diversity in cultivated Luffa acutangula (Cucurbitaceae) from China, Laos, and Nepal, and allozyme divergence between L. acutangula and L. aegyptiaca. Econ. Bot. 59: 154–165. doi:10.1663/0013-0001(2005)059[0154:AMAPDI]2.0.CO;2
- Martínez, C., Manzano, S., Megías, Z., Barrera, A., Boualem, A., Garrido, D., Bendahmane, A., and Jamilena, M. 2014. Molecular and functional characterization of CpACS27A gene reveals its involvement in monoecy instability and other associated traits in squash (Cucurbita pepo L). Planta 239: 1201–1215. doi:10.1007/s00425-014-2043-0
- Martínez, C., Manzano, S., Megías, Z., Garrido, D., Picó, B., and Jamilena, M. 2013. Involvement of ethylene biosynthesis and signalling in fruit set and early fruit development in zucchini squash (Cucurbita pepo L.). BMC Plant Biol. 13: 139. doi:10.1186/1471-2229-13-139
- Martínez-González, C., Castellanos-Morales, G., Barrera-Redondo, J., Sánchez-de la Vega, G., Hernández-Rosales, H. S., Gasca-Pineda, J., Aguirre-Planter, E., Moreno-Letelier, A., Escalante, A. E., Montes-Hernández, S., Lira-Saade, R., and Eguiarte, L. E. 2021. Recent and historical gene flow in cultivars, landraces, and a wild taxon of Cucurbita pepo in Mexico. Front. Ecol. Evol. 9: 656051. doi:10.3389/fevo.2021.656051
- Mashilo, J., Shimelis, H., and Ngwepe, R. M. 2022. Genetic resources of bottle gourd (Lagenaria siceraria (Molina) Standl.] and citron watermelon (Citrullus lanatus var. citroides (L.H. Bailey) Mansf. ex Greb.) - implications for genetic improvement, product development and commercialization: a review. S. Afr. J. Bot. 145: 28–47. doi:10.1016/j.sajb.2021.10.013
- Matsuda, S., and Takamatsu, S. 2003. Evolution of host-parasite relationship of Golovinomyces (Ascomycota: Erysiphaceae) inferred from nuclear rDNA sequences. Mol. Phylogenet. Evol. 27: 314–327. doi:10.1016/s1055-7903(02)00401-3
- Matsumura, H., Hsiao, M.-C., Lin, Y.-P., Toyoda, A., Taniai, N., Tarora, K., Urasaki, N., Anand, S. S., Dhillon, N. P. S., Schafleitner, R., and Lee, C.-R. 2020. Long-read bitter gourd (Momordica charantia) genome and the genomic architecture of nonclassic domestication. Proc. Natl. Acad. Sci. U S A. 117: 14543–14551. doi:10.1073/pnas.1921016117
- Matthew, D. 2022. Omics in vegetable crops: Cucurbitaceae and Amaryllidaceae. In Omics in Horticultural Crops; Rout, G. R. and Peter, K. V. Eds. Elsevier Science and Academic Press: London, UK, pp 239–280.
- Maxted, N., Ford-Lloyd, B. V., Jury, S., Kell, S. P., and Scholten, M. A. 2006. Towards a definition of a crop wild relative. Biodivers. Conserv. 15: 2673–2685. doi:10.1007/s10531-005-5409-6
- McCouch, S., Navabi, Z. K., Abberton, M., Anglin, N. L., Barbieri, R. L., Baum, M., Bett, K., Booker, H., Brown, G. L., Bryan, G. J., Cattivelli, L., Charest, D., Eversole, K., Freitas, M., Ghamkhar, K., Grattapaglia, D., Henry, R., Valadares Inglis, M. C., Islam, T., Kehel, Z., Kersey, P. J., King, G. J., Kresovich, S., Marden, E., Mayes, S., Ndjiondjop, M. N., Nguyen, H. T., Paiva, S. R., Papa, R., Phillips, P. W. B., Rasheed, A., Richards, C., Rouard, M., Amstalden Sampaio, M. J., Scholz, U., Shaw, P. D., Sherman, B., Staton, S. E., Stein, N., Svensson, J., Tester, M., Montenegro Valls, J. F., Varshney, R., Visscher, S., von Wettberg, E., Waugh, R., Wenzl, P., and Rieseberg, L. H. 2020. Mobilizing crop biodiversity. Mol. Plant. 13: 1341–1344. doi:10.1016/j.molp.2020.08.011
- McCreight, J. D. 2003. Genes for resistance to powdery mildew races 1 and 2US in melon PI 313970. HortScience 38: 591–594. doi:10.21273/HORTSCI.38.4.591
- McCreight, J. D. 2004. Notes on the change of the causal species of cucurbit powdery mildew in the U.S. Cucurbit Genet. Coop. Rep. 27: 8–23.
- McCreight, J. D. 2006. Melon–powdery mildew interactions reveal variation in melon cultigens and Podosphaera xanthii races 1 and 2. J. Amer. Soc. Hort. Sci. 131: 59–65. doi:10.21273/JASHS.131.1.59
- McCreight, J. D., and Coffey, M. D. 2011. Inheritance of resistance in melon PI 313970 to cucurbit powdery mildew incited by Podosphaera xanthii race S. HortScience 46: 838–840. doi:10.21273/HORTSCI.46.6.838
- McCreight, J. D., and Coffey, M. D. 2012. New sources of resistance to cucurbit powdery mildew in melon. HortScience 47:S292.
- McCreight, J. D., Coffey, M. D., Sedláková, B., and Lebeda, A. 2012. Cucurbit powdery mildew of melon incited by Podosphaera xanthii: Global and western U.S. perspectives. In Cucurbitaceae 2012. Proceedings of the Xth EUCARPIA Meeting on Genetics and Breeding of Cucurbitaceae; October 15-18, 2012, Antalya, Turkey; Sari, N., Solmaz, I., and Aras, V., Eds. Cukurova University: Antalya, Turkey, pp 181–189.
- McCreight, J. D., Kishaba, A. N., and Bohn, G. W. 1984. AR Hale’s Best Jumbo, AR 5, and AR Topmark, melon aphid-resistant muskmelon breeding lines. HortScience 19: 309–310. doi:10.21273/HORTSCI.19.2.309
- McCreight, J. D., Pitrat, M., Thomas, C. E., Kishaba, A. N., and Bohn, G. W. 1987. Powdery mildew resistance genes in muskmelon. J. Amer. Soc. Hort. Sci. 112: 156–160. doi:10.21273/JASHS.112.1.156
- McDonald, B. A., and Linde, C. 2002a. The population genetics of plant pathogens and breeding strategies for durable resistance. Euphytica 124: 163–180. doi:10.1023/A:1015678432355
- McDonald, B. A., and Linde, C. 2002b. Pathogen population genetics, evolutionary potential, and durable resistance. Annu. Rev. Phytopathol. 40: 349–379. doi:10.1146/annurev.phyto.40.120501.101443
- Meeboon, J., and Takamatsu, S. 2016. Notes on powdery mildews (Erysiphales) in Thailand II. Erysiphe species on Adoxaceae Anacardiaceae, Apocynaceae, Araliaceae, Aristolochiaceae, Bixaceae, Brassicaceae, Cleomaceae, Convolvulaceae, Cucurbitaceae and Euphorbiacea. Trop. Plant Pathol. 41: 357–369. doi:10.1007/s40858-016-0111-7
- Meglic, V., and Staub, J. E. 1996. Inheritance and linkage relationships of isozyme and morphological loci in cucumber (Cucumis sativus L.). Theor. Appl. Genet. 92: 865–872. doi:10.1007/BF00221899
- Meglic, V., Serquen, F., and Staub, J. E. 1996. Genetic diversity in cucumber (Cucumis sativus L.): I. A reevaluation of the U.S. germplasm collection. Genet. Genet. Resour. Crop Evol. 43: 533–546. doi:10.1007/BF00138830
- Mercier, J., Muscara, M. J., and Davis, A. R. 2014. First report of Podosphaera xanthii race 1W causing powdery mildew of watermelon in California. Plant Dis. 98: 158–158. doi:10.1094/PDIS-05-13-0552-PDN
- Miazzi, M., Laguardia, C., and Faretra, F. 2011. Variation in Podosphaera xanthii on cucurbits in Southern Italy. J. Phytopathol. 159: 538–545. doi:10.1111/j.1439-0434.2011.01801.x
- Mieslerová, B., Cook, R. T. A., Wheater, C. P., and Lebeda, A. 2022. Ecology of powdery mildews – influence of abiotic factors on their development and epidemiology. Critic. Rev. Plant Sci. 41: 365–390. doi:10.1080/07352689.2022.2138044
- Minocha, S. 2015. An overview of Lagenaria siceraria (Bottle gourd). J. Biomed. Pharm. Res. 4: 4–10.
- Misra, S., Srivastava, A. K., Verma, S., Pandey, S., Bargali, S. S., Rana, T. S., and Nair, K. N. 2017. Phenetic and genetic diversity in Indian Luffa (Cucurbitaceae) inferred from morphometric, ISSR and DAMD markers. Genet. Resour. Crop Evol. 64: 995–1010. doi:10.1007/s10722-016-0420-1
- Montero-Pau, J., Esteras, C., Banca, J., Ziarsolo, P., Cañizares, J., and Picó, B. 2017. Genetics and genomics of Cucurbita spp. In Genetics and Genomics of Cucurbitaceae; Grumet, R., Katzir, N., and Garcia-Mas, J. Eds. Springer International Publishing AG: Cham, Switzerland, pp 211–228.
- Montes-Hernandez, S., and Eguiarte, L. E. 2002. Genetic structure and indirect estimates of gene flow in three taxa of Cucurbita (Cucurbitaceae) in western Mexico. Am. J. Bot. 89: 1156–1163. doi:10.3732/ajb.89.7.1156
- Morimoto, Y., Maundu, P., Fujimaki, H., and Morishima, H. 2005. Diversity of landraces of the white-flowered gourd (Lagenaria siceraria) and its wild relatives in Kenya: fruit and seed morphology. Genet. Resour. Crop Evol. 52: 737–747. doi:10.1007/s10722-004-6119-8
- Morimoto, Y., Maundu, P., Kawase, M., Fujimaki, H., and Morishima, H. 2006. RAPD polymorphism of the white-flowered gourd (Lagenaria siceraria (Molina) Standl. landraces and its wild relatives in Kenya. Genet. Resour. Crop Evol. 53: 963–974. doi:10.1007/s10722-004-7070-4
- Morishita, M., Sugiyama, K., Saito, T., and Sakata, Y. 2002. An improved evaluation method for screening and selecting powdery mildew resistant cultivars and lines of cucumber (Cucumis sativus L.). J. Jpn. Hort. Sci. 71: 94–100. doi:10.2503/jjshs.71.94
- Morishita, M., Sugiyama, K., Saito, T., and Sakata, Y. 2003. Powdery mildew resistance in cucumber. JARQ 37: 7–14. doi:10.6090/jarq.37.7
- Naegele, R. P., and Wehner, T. C. 2017. Genetic resources of cucumber. In Genetics and Genomics of Cucurbitaceae; Grumet, R., Katzir, N., and Garcia-Mas, J. Eds. Springer International Publishing AG: Cham, Switzerland, pp 61–86.
- Natarajan, S., Kim, H. T., Thamilarasan, S. K., Veerappan, K., Park, J. I., and Nou, I. S. 2016. Whole genome re-sequencing and characterization of powdery mildew disease-associated allelic variation in melon. PLoS ONE 11:e0157524. doi:10.1371/journal.pone.0157524
- NatureServe Explorer. 2023. www.natureserve.org (accessed Aug. 9, 2023).
- Navrátilová, B., Skálová, D., Ondřej, V., Kitner, M., and Lebeda, A. 2011. Biotechnological methods utilized in Cucumis sp. research – A review. Hortic. Sci. 38: 150–158. doi:10.17221/143/2010-HORTSCI
- Nee, M. 1990. The Domestication of Cucurbita (Cucurbitaceae). Econ. Bot. 44: p. 56–68.
- Nesom, G. L. 2011. Toward consistency of taxonomic rank in wild/domesticated Cucurbitaceae. Phytoneuron 13: 1–33.
- Neuhausen, S. L. 1992. Evaluation of restriction fragment length polymorphism in Cucumis melo. Theor. Appl. Genet. 83: 379–384. doi:10.1007/BF00224286
- Ngwepe, R. M., Mashilo, J., and Shimelis, H. 2019. Progress in genetic improvement of citron watermelon (Citrullus lanatus var. citroides): a review. Genet. Resour. Crop Evol. 66: 735–758. doi:10.1007/s10722-018-0724-4
- Ngwerume, F. C. 2004. Cucurbita moschata. In PROTA 2. Plant Resources of Tropical Africa; Grubben, G. J. H., and Denton, O. A. Eds. PROTA Foundation–Backhuys–CTA: Wageningen, the Netherlands, p 668.
- Nie, J. T., He, H. L., Peng, J. L., Yang, X. Q., Bie, B. B., Zhao, J. L., Wang, Y. L., Si, L. T., Pan, J. S., and Cai, R. 2015a. Identification and fine mapping of pm5.1: a recessive gene for powdery mildew resistance in cucumber (Cucumis sativus L.). Mol. Breed. 35: 7.
- Nie, J. T., Wang, Y. L., He, H. L., Guo, C. L., Zhu, W. Y., Pan, J., Li, D. D., Lian, H. L., Pan, J. S., and Cai, R. 2015b. Loss-of-function mutations in CsMLO1confer durable powdery mildew resistance in cucumber (Cucumis sativus L.). Front. Plant Sci. 6: 1155. doi:10.3389/fpls.2015.01155
- Nie, J. T., Wang, H. Z., Zhang, W. H., Teng, X., Yu, C., Cai, R., and Wu, G. 2021. Characterization of lncRNAs and mRNAs involved in powdery mildew resistance in cucumber. Phytopathology 111: 1613–1624. doi:10.1094/PHYTO-11-20-0521-R
- Niks, R. E., and Marcel, T. C. 2009. Nonhost and basal resistance: how to explain specificity? New Phytol. 182: 817–828. doi:10.1111/j.1469-8137.2009.02849.x
- Ning, X. F., Wang, X. L., Gao, X. W., Zhang, Z. Q., Zhang, L. H., Yan, W. L., and Li, G. 2014. Inheritances and location of powdery mildew resistance gene in melon Edisto 47. Euphytica 195: 345–353. doi:10.1007/s10681-013-1000-5
- Norton, J. D., Boyhan, G. E., Smith, D. A., and Abrahams, D. R. 1995. Au-sweet scarlet watermelon. HortScience 30: 393–394. doi:10.21273/HORTSCI.30.2.393
- Nunes, E. W. L. P., Esteras, C., Ricarte, A. O., Martínez-Perez, E., Gómez-Guillamón, M. L., Nunes, G. H. S., and Picó, M. B. 2017a. Brazilian melon landraces resistant to Podosphaera xanthii are unique germplasm resources. Ann. Appl. Biol. 171: 214–228. doi:10.1111/aab.12370
- Nunes, E.W.L.P., Martínez, E.M., Aragão, F.A.S., Esteras, C., Nunes, G.H.S., and Picó, M.B. 2017b. Inheritance of resistance to Podosphaera xanthii in melon accessions AM-55 and AC-15. Acta Hortic. 1151: 63–68. doi:10.17660/ActaHortic.2017.1151.11
- Nunes, E.W.L.P., Ricarte, A.O., Martínez, E.M., Esteras, C., Nunes, G.H.S., and Picó, M.B. 2017c. Morphological and molecular characterization of new melon germplasm resistant to Podosphaera xanthii. Acta Hortic. 1151: 69–74. doi:10.17660/ActaHortic.2017.1151.12
- Palomares-Rius, F. J., Garcés-Claver, A., Picó, M. B., Esteras, C., Yuste-Lisbona, F. J., and Gómez-Guillamón, M. L. 2018. 'Carmen’, a yellow canary melon breeding line resistant to Podosphaera xanthii, Aphis gossypii, and Cucurbit Yellow Stunting Disorder Virus. HortScience 53: 1072–1075. doi:10.21273/HORTSCI13013-18
- Palti, J. 1961. Problems of plant disease forecasting in an arid zone. Plant Dis. Rep. 45: 31–37.
- Pan, W., Cheng, Z., Han, Z., Yang, H., Zhang, W., and Zhang, H. 2022. Efficient genetic transformation and CRISPR/Cas9-mediated genome editing of watermelon assisted by genes encoding developmental regulators. J. Zhejiang Univ. Sci. B 23: 339–344. doi:10.1631/jzus.B2200119
- Pandey, S., Ansari, W. A., Choudhary, B. R., Pandey, M., Jena, S. N., Singh, A. K., Dubey, R. K., and Singh, B. 2017. Microsatellite analysis of genetic diversity and population structure of hermaphrodite ridge gourd (Luffa hermaphrodita). 3 Biotech 8: 17. doi:10.1007/s13205-017-1030-0
- Pandey, A., and Rajkumar, S. 2021. A new potential variety of cultivated melon (Cucumis melo L.) from north western India. Genet. Resour. Crop Evol. 68: 785–794. doi:10.1007/s10722-020-00997-2
- Panstruga, R., and Moscou, M. J. 2020. What is the molecular basis of nonhost resistance? Mol. Plant. Microbe Interact. 33: 1253–1264. doi:10.1094/MPMI-06-20-0161-CR
- Panstruga, R., and Schulze-Lefert, P. 2002. Live and let live: insights into powdery mildew disease and resistance. Mol. Plant Pathol. 3: 495–502. doi:10.1046/j.1364-3703.2002.00145.x
- Paris, H. S. 2015. Origin and emergence of the sweet dessert watermelon, Citrullus lanatus. Ann. Bot. 116: 133–148. doi:10.1093/aob/mcv077
- Paris, H. S. 2016. Germplasm enhancement of Cucurbita pepo (pumpkin, squash, gourd: Cucurbitaceae): progress and challenges. Euphytica 208: 415–438. doi:10.1007/s10681-015-1605-y
- Paris, H. S. 2017. Genetic resources of pumpkins and squash, Cucurbita spp. In Genetics and Genomics of Cucurbitaceae; Grumet, R., Katzir, N., and Garcia-Mas, J. Eds. Springer International Publishing AG: Cham, Switzerland, pp 111–154.
- Paris, H. S. 2018. Consumer-oriented exploitation and conservation of genetic resources of pumpkins and squash, Cucurbita. Israel J. Plant Sci. 65: 202–221. doi:10.1163/22238980-00001036
- Paris, H. S., and Brown, R. N. 2005. The genes of pumpkin and squash. HortScience 40: 1620–1630. doi:10.21273/HORTSCI.40.6.1620
- Paris, H. S., Burger, Y., and Schaffer, A. A. 2006. Genetic variability and introgression of horticulturally valuable traits in squash and pumpkins of Cucurbita pepo. Isr. J. Plant Sci. 54: 223–231. doi:10.1560/IJPS_54_3_223
- Paris, H. S., and Cohen, R. 2002. Powdery mildew-resistant summer squash hybrids having higher yields than their susceptible, commercial counterparts. Euphytica 124: 121–128. doi:10.1023/A:1015623013740
- Paris, H. S., Daunay, M., Ch., and Janick, J. 2012a. Occidental diffusion of cucumber (Cucumis sativus) 500–1300 CE: two routes to Europe. Ann. Bot. 109: 117–126. doi:10.1093/aob/mcr281
- Paris, H. S., and Kabelka, E. 2008-2009. Gene list of Cucurbita species, 2009. Cucurbit Genet. Coop. Rep. 31–32: 44–69.
- Paris, H. S., Lebeda, A., Křístková, E., Andres, T. C., and Nee, M. H. 2012b. Parallel evolution under domestication and phenotypic differentiation of the cultivated subspecies of Cucurbita pepo (Cucurbitaceae). Econ. Bot. 66: 71–90. doi:10.1007/s12231-012-9186-3
- Paris, H. S., and Padley, L. D. Jr., 2014-2015. Gene List for Cucurbita species, 2014. Cucurbit Genet. Coop. Rep. 37–38: 1–14. https://cucurbit.info/wp-content/uploads/2018/ 10/gene14 squash.pdf (accessed Jan. 29, 2023).
- Park, B. S., Jang, S., Yu, Y., Choi, G. J., Kang, B. C., and Seo, S. K. 2020. QTL mapping and molecular markers of powdery mildew resistance in pumpkin (Cucurbita moschata). Hort. Sci. Technol. 38: 717–729. doi:10.7235/HORT.20200065
- Parra, L., Maisonneuve, B., Lebeda, A., Schut, J., Christopoulou, M., Jeuken, M., McHale, L., Truco, M.-J., Crute, I., and Michelmore, R. 2016. Rationalization of genes for resistance to Bremia lactucae in lettuce. Euphytica 210: 309–326. doi:10.1007/s10681-016-1687-1
- Parvathi, M. S., Antony, P. D., and Kutty, M. S. 2022. Multiple stressors on vegetable production: insigths for trait-based crop improvement in cucurbits. Front. Plant Sci. 13: 861637. doi:10.3389/fpls.2022.861637
- Pautasso, M., Döring, T. F., Garbelotto, M., Pellis, L., and Jeger, M. J. 2012. Impacts of climate change on plant diseases – opinions and trends. Eur. J. Plant Pathol. 133: 295–313. doi:10.1007/s10658-012-9936-1
- Pavan, S., Jacobsen, E., Visser, R. G. F., and Bai, Y. 2009. Loss of susceptibility as a novel breeding strategy for durable and broad-spectrum resistance. Mol. Breed. 25: 1–12. doi:10.1007/s11032-009-9323-6
- Perchepied, L., Bardin, M., Dogimont, C., and Pitrat, M. 2005. Relationship between loci conferring downy mildew and powdery mildew resistance in melon assessed by quantitative trait loci mapping. Phytopathology 95: 556–565. doi:10.1094/PHYTO-95-0556
- Pérez-García, A., Romero, D., Fernández-Ortuño, D., López-Ruiz, F., De Vicente, A., and Torés, J. A. 2009. The powdery mildew fungus Podosphaera fusca (synonym Podosphaera xanthii), a constant threat to cucurbits. Mol. Plant Pathol. 10: 153–160. doi:10.1111/j.1364-3703.2008.00527.x
- Perez, G. A., Tongyoo, P., Chunwongse, J., de Jong, H., Wongpraneekul, A., Sinsathapornpong, W., and Chuenwarin, P. 2021. Genetic diversity and population structure of ridge gourd (Luffa acutangula) accessions in a Thailand collection using SNP markers. Sci. Rep. 11: 15311. doi:10.1038/s41598-021-94802-4
- Périn, C., Hagen, S., De Conto, V., Katzir, N., Danin-Poleg, Y., Portnoy, V., Baudracco-Arnas, S., Chadoeuf, J., Dogimont, C., and Pitrat, M. 2002. A reference map of Cucumis melo based on two recombinant inbred line populations. Theor. Appl. Genet. 104: 1017–1034. doi:10.1007/s00122-002-0864-x
- Pierce, L. K., and Wehner, T. C. 1990. Review of genes and linkage groups in cucumber. HortScience 25: 605–615. doi:10.21273/HORTSCI.25.6.605
- Pirondi, A., Kitner, M., Iotti, M., Sedláková, B., Lebeda, A., and Collina, M. 2016. Genetic structure and phylogeny of Italian and Czech populations of the cucurbit powdery mildew fungus Golovinomyces orontii inferred by multilocus sequence typing. Plant Pathol. 65: 959–967. doi:10.1111/ppa.12480
- Pirondi, A., Pérez-García, A., Battistini, G., Muzzi, E., Brunelli, A., and Collina, M. 2015a. Seasonal variations in the occurrence of Golovinomyces orontii and Podosphaera xanthii, causal agents of cucurbit powdery mildew in Northern Italy. Ann. Appl. Biol. 167: 298–313. doi:10.1111/aab.12225
- Pirondi, A., Vela-Corcía, D., Dondini, L., Brunelli, A., Pérez-García, A., and Collina, M. 2015b. Genetic diversity analysis of the cucurbit powdery mildew fungus Podosphaera xanthii suggests a clonal population structure. Fungal Biol. 119: 791–801. doi:10.1016/j.funbio.2015.05.003
- Pitrat, M. 1991. Linkage groups in Cucumis melo L. J. Heredity 82: 406–411. doi:10.1093/oxfordjournals.jhered.a111112
- Pitrat, M. 2013. Phenotypic diversity in wild and cultivated melons (Cucumis melo). Plant Biotech. 30: 273–278. doi:10.5511/plantbiotechnology.13.0813a
- Pitrat, M. 2017. Melon genetic resources: phenotypic diversity and horticultural taxonomy. In Genetics and Genomics of the Cucurbitaceae, Vol. 20; Grumet, R., Katzir, N., Garcia-Mas, J. Eds. Springer: Cham, Switzerland, pp 25–60.
- Pitrat, M., and Besombes, D. 2008. Inheritance of Podosphaera xanthii resistance in melon line ‘90625'. In Cucurbitaceae 2008, IXth EUCARPIA Meeting on Genetics and Breeding of Cucurbitaceae, Pitrat, M. Ed. INRA: Avignon, France, pp 135–142.
- Pitrat, M., and de Vaulx, D. 1979. Recherche de géniteurs de résistance à l´oïdium et aux Virus de la Mosaïque du Concombre et de la Mosaïque de la Pastèque chez Cucurbita sp. Ann. Amélior. Plantes 29: 439–445.
- Pitrat, M., Dogimont, C., and Bardin, M. 1998. Resistance to fungal diseases of foliage in melon. In Cucurbitaceae ´98. Evaluation and Enhancement of Cucurbit Germplasm; McCreight, J. D. Ed. A.S.H.S. Press: Alexandria, VA, pp 167–173.
- Pitrat, M., Hanelt, P., Hammer, K., Katzir, N., and Paris, H. S. 2000. Some comments on infraspecific classification of cultivars of melon. Acta Hortic. 510: 29–36. doi:10.17660/ActaHortic.2000.510.4
- Plants of the World Online. 2023. www.powo.science.kew.org (accessed Aug. 9, 2023).
- Polonio, Á., Pineda, M., Bautista, R., Martínez-Cruz, J., Pérez-Bueno, M. L., Barón, M., and Pérez-García, A. 2019. RNA-seq analysis and fluoroscence imaging of melon powdery mildew disease reveal an orchestrated reprogramming of host physiology. Sci. Rep. 9: 7978. doi:10.1038/s41598-019-44443-5
- Porterfield, R., and Meru, G. 2017. Candidate susceptibility genes for powdery and downy mildew in watermelon and squash. J. Phylogenetics Evol. Biol. 5: 186.
- Prasanth, K., Sadashiva, A.T., Pitchaimuthu, M., and Varalakshmi, B. 2020. Genetic diversity, variability and correlation studies in bitter gourd (Momordica charantia L.). Indian J. Plant Genet. Resour. 33: 179–185. doi:10.5958/0976-1926.2020.00026.1
- Qi, J., Liu, X., Shen, D., Miao, H., Xie, B., Li, X., Zeng, P., Wang, S., Shang, Y., Gu, X., Du, Y., Li, Y., Lin, T., Yuan, J., Yang, X., Chen, J., Chen, H., Xiong, X., Huang, K., Fei, Z., Mao, L., Tian, L., Städler, T., Renner, S. S., Kamoun, S., Lucas, W. J., Zhang, Z., and Huang, S. 2013. A genomic variation map provides insights into the genetic basis of cucumber domestication and diversity. Nat. Genet. 45: 1510–1515. doi:10.1038/ng.2801
- Qie, Y., Sheng, Y., Xu, H., Jin, Y., Ma, F., Li, L., Li, X., and An, D. 2019. Identification of a new powdery mildew resistance gene pmDHT a tor closely linked to the Pm5 locus in the Chinese wheat landrace Dahongtou. Plant Dis. 103: 2645–2651. doi:10.1094/PDIS-02-19-0401-RE
- Qiu, P. L., Qi, X. F., Li, Y., Braun, U., and Liu, S. Y. 2020. Epitypification and molecular confirmation of Erysiphe cucurbitacearum as a synonym of Golovinomyces tabaci. Mycoscience 61: 30–36. doi:10.1016/j.myc.2019.09.002
- Rabelo, H. O., Santos, L. S., Diniz, G. M. M., Marin, M. V., Braz, L. T., and McCreight, J. D. 2017. Cucurbits powdery mildew race identity and reaction of melon genotypes. Pesqui. Agropecu. Trop. 47: 440–447. doi:10.1590/1983-40632017v4749537
- Rawat, S., and Meena, S. 2014. Publish or perish: where are we heading? J. Res. Med. Sci. 19: 87–89.
- Rennberger, G., Kousik, C. S., and Keinath, A. P. 2018. First report of powdery mildew on Cucumis zambianus, Cucurbita digitata, and Melothria scabra caused by Podosphaera xanthii in the United States. Plant Dis. 102: 246–246. doi:10.1094/PDIS-06-17-0916-PDN
- Renner, S. S. 2017. A valid name for the Xishuangbanna gourd, a cucumber with carotene-rich fruits. PhytoKeys 85: 87–94. doi:10.3897/phytokeys.85.17371
- Renner, S. S., Chomicki, G., and Greuter, W. 2014. Proposal to conserve the name Momordica lanata (Citrullus lanatus) (watermelon, Cucurbitaceae), with a conserved type, against Citrullus battich. Taxon 63: 941–942. doi:10.12705/634.29
- Renner, S. S., and Schaefer, H. 2017. Phylogeny and evolution of the Cucurbitaceae. In Genetics and Genomics of Cucurbitaceae; Grumet, R., Katzir, N., and Garcia-Mas, J. Eds. Springer: Cham, Switzerland, pp 14–23.
- Renner, S. S., Schaefer, H., and Kocyan, A. 2007. Phylogenetics of Cucumis (Cucurbitaceae): Cucumber (C. sativus) belongs in an Asian/Australian clade far from melon (C. melo). BMC Evol. Biol. 7: 58. doi:10.1186/1471-2148-7-58
- Renner, S. S., Sousa, A., and Chomicki, G. 2017. Chromosome numbers, Sudanese wild forms, and classification of the watermelon genus Citrullus, with 50 names allocated to seven biological species. Taxon 66: 1393–1405. doi:10.12705/666.7
- Reyad, N. E.-H A., Azoz, S. N., Ali, A. M., and Sayed, E. G. 2022. Mitigation of powdery mildew disease by integrating biocontrol agents and shikimic acid with modulation of antioxidant defense system, anatomical characterization, and improvement of squash plant productivity. Horticulturae 8: 1145. doi:10.3390/horticulturae8121145
- Rhodes, A. M. 1959. Species hybridization and interspecific gene transfer in the genus Cucurbita. Proc. Am. Soc. Hortic. Sci. 74: 546–551.
- Rhodes, A. M. 1964. Inheritance of powdery mildew resistance in the genus Cucurbita. Plant Dis. Rep. 48: 54–55.
- Richardson, J. B. 1972. The Pre-Columbian distribution of the bottle gourd (Lagenaria siceraria): a re-evaluation. Econ. Bot. 26: 265–273. doi:10.1007/BF02861040
- Robinson, R. W., and Decker-Walters, D. S. 1997. Cucurbits. CAB International: Wallingford, UK.
- Robinson, R. W., Provvidenti, R., and Shail, J. W. 1975. Inheritance of susceptibility to powdery mildew in the watermelon. J. Heredity 66: 310–311. doi:10.1093/oxfordjournals.jhered.a108636
- Romero, D., Eugenia Rivera, M., Cazorla, F. M., Codina, J. C., Fernández-Ortuño, D., Torés, J. A., Pérez-García, A., and de Vicente, A. 2008. Comparative histochemical analyses of oxidative burst and cell wall reinforcement in compatible and incompatible melon-powdery mildew (Podosphaera fusca) interactions. J. Plant Physiol. 165: 1895–1905. doi:10.1016/j.jplph.2008.04.020
- Rubatzky, V. E., and Yamaguchi, M. 1997. World Vegetables. Principles, Production, and Nutritive Values, 2nd ed. Chapman & Hall: New York.
- Ruggieri, V., Alexiou, K. G., Morata, J., Argyris, J., Pujol, M., Yano, R., Nonaka, S., Ezura, H., Latrasse, D., Boualem, A., Benhamed, M., Bendahmane, A., Cigliano, R. A., Sanseverino, W., Puigdomènech, P., Casacuberta, J. M., and Garcia-Mas, J. 2018. An improved assembly and annotation of the melon (Cucumis melo L.) reference genome. Sci. Rep. 8: 8088. doi:10.1038/s41598-018-26416-2
- Sakata, Y., Kubo, N., Morishita, M., Kitadani, E., Sugiyama, M., and Hirai, M. 2006. QTL analysis of powdery mildew resistance in cucumber (Cucumis sativus L). Theor. Appl. Genet. 112: 243–250. doi:10.1007/s00122-005-0121-1
- Sales Júnior, R., Nunes, G. H., Michereff, S. J., Pereira, E. W., and Guimarães, I. M. 2011. Reaction of families and lines of melon to powdery mildew. Hortic. Bras. 29: 382–386. doi:10.1590/S0102-05362011000300021
- Salmon, E. 1900. A monograph of the Erysiphaceae. Mem. Torrey Bot. Club 9: 1–292.
- Salmon, E. S. 1906. On Oidiopsis taurica (Lév.), an endophytic member of the Erysiphaceae. Ann. Bot. os-20: 187–200. doi:10.1093/oxfordjournals.aob.a089091
- Sánchez-de la Vega, G., Castellanos-Morales, G., Gámez, N., Hernández-Rosales, H. S., Vázquez-Lobo, A., Aguirre-Planter, E., Jaramillo-Correa, J. P., Montes-Hernández, S., Lira-Saade, R., and Eguiarte, L. E. 2018. Genetic resources in the “Calabaza Pipiana” squash (Cucurbita argyrosperma) in Mexico: genetic diversity, genetic differentiation and distribution models. Front. Plant Sci. 9: 400. doi:10.3389/fpls.2018.00400
- Sanjur, O. I., Piperno, D. R., Andres, T. C., and Wessel-Beaver, L. 2002. Phylogenetic relationships among domesticated and wild species of Cucurbita (Cucurbitaceae) inferred from a mitochondrial gene: implications for crop plant evolution and areas of origin. Proc. Natl. Acad. Sci. U S A. 99: 535–540. doi:10.1073/pnas.012577299
- Saur, I. M. L., and Hückelhoven, R. 2021. Recognition and defence of plant-infecting fungal pathogens. J. Plant Physiol. 256: 153324. doi:10.1016/j.jplph.2020.153324
- Sawada, K. 1928. Descriptive catalogue of the Formosan fungi IV. Bull. Dept. Agr. Govt. Res. Inst. Formosa 35: 1–123.
- Schachtel, G. A., Dinoor, A., Herrmann, A., and Kosman, E. 2012. Comprehensive evaluation of virulence and resistance data: a new analysis tool. Plant Dis. 96: 1060–1063. doi:10.1094/PDIS-02-12-0114-SR
- Schaefer, H., Heibl, C., and Renner, S. S. 2009. Gourds afloat: A dated phylogeny reveals an Asian origin of the gourd family (Cucurbitaceae) and numerous oversea dispersal events. Proc. Biol. Sci. 276: 843–851. doi:10.1098/rspb.2008.1447
- Schaefer, H., and Renner, S. S. 2010. A three-genome phylogeny of Momordica (Cucurbitaceae) suggests seven returns from dioecy to monoecy and recent long-distance dispersal to Asia. Mol. Phylogenet. Evol. 54: 553–560. doi:10.1016/j.ympev.2009.08.006
- Schaefer, H., and Renner, S. S. 2011. Phylogenetic relationships in the order Cucurbitales and a new classification of the gourd family (Cucurbitaceae). Taxon 60: 122–138. doi:10.1002/tax.601011
- Schnathorst, W. C. 1965. Environmental relationships in powdery mildews. Annu. Rev. Phytopathol. 3: 343–366. doi:10.1146/annurev.py.03.090165.002015
- Schouten, H. J., Krauskopf, J., Visser, R. G. F., and Bai, Y. L. 2014. Identification of candidate genes required for susceptibility to powdery or downy mildew in cucumber. Euphytica 200: 475–486. doi:10.1007/s10681-014-1216-z
- Schweinfurth, G. 1883. The flora of ancient Egypt. Nature 28: 109–114.
- Sebastian, P., Schaefer, H., Telford, I. R. H., and Renner, S. S. 2010. Cucumber (Cucumis sativus) and melon (C. melo) have numerous wild relatives in Asia and Australia, and the sister species of melon is from Australia. Proc. Natl. Acad. Sci. U S A. 107: 14269–14273. doi:10.1073/pnas.1005338107
- Sedlářová, M., Kubienová, L., Drábková-Trojanová, Z., Luhová, L., Lebeda, A., and Petřivalský, M. 2016. The role of nitric oxide in development and pathogenesis of biotrophic phytopathogens – downy and powdery mildews. In Nitric Oxide and Signaling in Plants, Vol. 77; Wendehenne, D., Ed., Academic Press: London, pp 263–283.
- Sedlářová, M., Lebeda, A., Mikšíková, P., Duchoslav, M., Sedláková, B., and McCreight, J. D. 2009. Histological aspects of Cucumis melo PI 313970 resistance to Podosphaera xanthii and Golovinomyces cichoracearum. J. Plant Dis. Prot. 116: 169–176. doi:10.1007/BF03356306
- Seshadri, V. S., and More, T. A. 2009. Cucurbit Vegetables – Biology, Production and Utilization. New Delhi: Studium Press, pp 482.
- Shanmugasundaram, S., Williams, P. H., and Peterson, C. E. 1971a. Inheritance of resistance to powdery mildew in cucumber. Phytopathology 61: 1218–1221. doi:10.1094/Phyto-61-1218
- Shanmugasundaram, S., Williams, P. H., and Peterson, C. E. 1971b. Inheritance of fruit spine color in cucumber-D. HortScience 6: 213–214. doi:10.21273/HORTSCI.6.3.213
- Shanmugasundaram, S., Williams, P. H., and Peterson, C. E. 1972. A recessive cotyledon marker gene in cucumber with pleiotropic effect. HortScience 7: 555–556. doi:10.21273/HORTSCI.7.6.555b
- Sharma, S., Dar, A. A., Gupta, S., and Singh, R. 2021. Evaluation of resistant genotypes and their characterization using molecular markers linked for powdery mildew resistance in cucumber (Cucumis sativus L.). Plant Genet. Resour. 19: 497–502. doi:10.1017/S1479262121000605
- Shimizu, S., Kanazawa, K., and Kato, A. 1963. Studies on the breeding of cucumber for resistance to downy mildew. Difference of resistance to downy mildew among the cucumber varieties and the utility of the cucumber variety resistance to downy mildew. Bul. Hort. Res. Sta. Jpn. 2: 80–81.
- Shimomura, K., Sugiyama, M., Kawazu, Y., and Yoshioka, Y. 2021. Identification of quantitative trait loci for powdery mildew resistance in highly resistant cucumber (Cucumis sativus L.) using ddRAD-seq analysis. Breed. Sci. 71: 326–333. doi:10.1270/jsbbs.20141
- Shin, A. Y., Koo, N., Kim, S., Sim, Y. M., Choi, D., Kim, Y. M., and Kwon, S. Y. 2019. Draft genome sequences of two oriental melons, Cucumis melo L. var. makuwa. Sci. Data 6: 220. doi:10.1038/s41597-019-0244-x
- Shishkoff, N. 2000. The name of the cucurbit powdery mildew: Podosphaera (sect. Sphaerotheca) xanthii (Castag.) U. Braun & N. Shish. comb. nov. Phytopathology 90:S133.
- Simko, I., Jia, M., Venkatesh, J., Kang, B.-C., Weng, Y., Barcaccia, G., Lanteri, S., Bhattarai, G., and Foolad, M. R. 2021. Genomics and marker-assisted improvement of vegetable crops. Crit. Rev. Plant Sci. 40: 303–365. doi:10.1080/07352689.2021.1941605
- Singh, D., Singh, A., and Singh, A. 2021. Wide Hybridization. Plant Breeding and Cultivar Development. Academic Press: London, UK, pp 159–178.
- Sitterly, W. R. 1972. Breeding for disease resistance in cucurbits. Annu. Rev. Phytopathol. 10: 471–490. doi:10.1146/annurev.py.10.090172.002351
- Sitterly, W. R., 1978. Powdery mildews of cucurbits. In The Powdery Mildews; Spencer, D. M. Ed. Academic Press: London, UK, pp 359–379.
- Skálová, D., Lebeda, A., and Navrátilová, B. 2004. Embryo and ovule cultures in Cucumis species and their utilization in interspecific hybridization. In Progress in Cucurbit Genetics and Breeding Research. Proceedings of Cucurbitaceae 2004, the 8th EUCARPIA Meeting on Cucurbit Genetics and Breeding; Lebeda, A. and Paris, H. S. Eds. Palacký University in Olomouc: Olomouc, Czech Republic, pp 415–430.
- Smith, B. D. 2006. Eastern North America as an independent center of plant domestication. Proc. Natl. Acad. Sci. U S A. 103: 12223–12228. doi:10.1073/pnas.0604335103
- Smith, P. 1948. Powdery mildew resistance in cucumber. Phytopathology 38: 1027–1028.
- Spanu, P. D., and Kämper, J. 2010. Genomics of biotrophy in fungi and oomycetes - emerging patterns. Curr. Opin. Plant Biol. 13: 409–414. doi:10.1016/j.pbi.2010.03.004
- Spanu, P. D., and Panstruga, R. 2017. Editorial: biotrophic plant-microbe interactions. Front. Plant Sci. 8: 192. doi:10.3389/fpls.2017.00192
- Spencer, D. M. E. 1978. The Powdery Mildews. Academic Press: London, UK.
- Spring, O., Gomez-Zeledon, J., Hadziabdic, D., Trigiano, R. N., Thines, M., and Lebeda, A. 2018. Biological characteristics and assessment of virulence diversity in pathosystems of economically important biotrophic oomycetes. Crit. Rev. Plant Sci. 37: 439–495. doi:10.1080/07352689.2018.1530848
- Srivastava, D., Khan, N. A., Shamim, M., Yadav, P., Pandey, P., and Singh, K. N. 2014. Assessment of the Genetic Diversity in Bottle Gourd (Lagenaria siceraria [Molina] Standl.) Genotypes Using SDS-PAGE and RAPD Markers. Natl. Acad. Sci. Lett. 37: 155–161. doi:10.1007/s40009-013-0207-2
- Staub, J. E., Box, J., Meglic, V., Horejsi, T. F., and McCreight, J.D. 1997. Comparison of isozyme and random amplified polymorphic DNA data for determining intraspecific variation in Cucumis. Genet. Resour. Crop Evol. 44: 257–269. doi:10.1023/A:1008639616331
- Staub, J. E., Danin-Poleg, Y., Fazio, G., Horejsi, T., Reis, N., and Katzir, N. 2000. Comparative analysis of cultivated melon groups (Cucumis melo L.) using random amplified polymorphic DNA and simple sequence repeat markers. Euphytica 115: 225–241. doi:10.1023/A:1004054014174
- Suh, S. K., and Cho, M. 2017. New Cucurbita pepo variety (CTC12873BP) resistant to powdery mildew and resistant to infection of virus e.g. zucchini yellow mosaic virus and watermelon mosaic virus, does not have thin appearance in neck portion. Patent Number(s): KR2017013006-A. Patent Assignee: SUH S K(SUHS-Individual. Derwent Primary Accession Number: 2017-123583
- Sun, J., Dong, Y., Wang, C., Xiao, S., Jiao, Z., and Gao, C. 2021. Identification and characterization of melon circular RNAs involved in powdery mildew responses through comparative transcriptome analysis. PeerJ 9:e11216. doi:10.7717/peerj.11216
- Sun, J., Lv, D., Chen, Y., Pan, J., Cai, R., and Pan, J. 2022. QTL mapping for disease resistance in cucumber. In The Cucumber Genome; Pandey, S., Weng, Y., Behera, T. K., and Bo, K., Eds. Springer Nature Switzerland AG: Cham, Switzerland, pp 81–92.
- Sun, H., Wu, S., Zhang, G., Jiao, C., Guo, S., Ren, Y., Zhang, J., Zhang, H., Gong, G., Jia, Z., Zhang, F., Tian, J., Lucas, W. J., Doyle, J. J., Li, H., Fei, Z., and Xu, Y. 2017. Karyotype stability and unbiased fractionation in the paleo-allotetraploid Cucurbita genomes. Mol. Plant. 10: 1293–1306. doi:10.1016/j.molp.2017.09.003
- Swamy, K. R. M. 2023. Origin, distribution, taxonomy, botanical description, cytogenetics, genetic diversity and breeding of bitter gourd (Momordica charantia l.). Int. J. Dev. Res. 13: 61294–61306.
- Takamatsu, S., Hirata, T., and Sato, Y. 2000. A parasitic transition from trees to herbs occurred at least twice in tribe Cystotheceae (Erysiphaceae): Evidence from nuclear ribosomal DNA. Mycol. Res. 104: 1304–1311. doi:10.1017/S0953756200003014
- Takamatsu, S., Matsuda, S., and Grigaliunaite, B. 2013. Comprehensive phylogenetic analysis of the genus Golovinomyces (Ascomycota: Erysiphales) reveals close evolutionary relationships with its host plants. Mycologia 105: 1135–1152. doi:10.3852/13-046
- Teixeira, A. M., Barreto, F. A., and Camargo, L. E. 2006. An AFLP marker linked to the Pm-1 gene that confers resistance to Podosphaera xanthii in melon. Phytopathology 96:S113–S114.
- Teixeira, A. P. M. d., Silva Barreto, F. A., and Camargo, L. E. A. 2008. An AFLP marker linked to the Pm-1 gene that confers resistance to Podosphaera xanthii race 1 in Cucumis melo. Genet. Mol. Biol. 31: 547–550. doi:10.1590/S1415-47572008000300023
- Tek, M. I., and Calis, O. 2022. Mechanisms of resistance to powdery mildew in cucumber. Phytopathol. Mediterr. 61: 119–127. doi:10.36253/phyto-13313
- Tek, M. I., Calis, O., Fidan, H., Shah, M. D., Celik, S., and Wani, S. H. 2022. CRISPR/Cas9 based mlo-mediated resistance against Podosphaera xanthii in cucumber (Cucumis sativus L.). Front. Plant Sci. 13: 1081506. doi:10.3389/fpls.2022.1081506
- Telford, R. H., Schaefer, H., Greuter, W., and Renner, S. S. 2011b. A new Australian species of Luffa (Cucurbitaceae) and typification of two Australian Cucumis names, all based on specimens collected by Ferdinand Mueller in 1856. PhytoKeys 5: 21–29. doi:10.3897/phytokeys.5.1395
- Telford, I. R. H., Sebastian, P. M., Bruhl, J. J., and Renner, S. S. 2011a. Cucumis (Cucurbitaceae) in Australia and eastern Malesia, including newly recognized species and the sister species to C. melo. Syst. Bot. 36: 376–389. doi:10.1600/036364411X569561
- Tetteh, A. Y., Wehner, T. C., and Davis, A. R. 2010. Identifying resistance to powdery mildew race 2W in the USDA-ARS watermelon grmplasm collection. Crop Sci. 50: 933–939. doi:10.2135/cropsci2009.03.0135
- Tetteh, A. Y., Wehner, T. C., and Davis, A. R. 2013a. Inheritance of resistance to powdery mildew race 2 in Citrullus lanatus var. lanatus. HortScience 48: 1227–1230. doi:10.21273/HORTSCI.48.10.1227
- Tetteh, A. Y., Wehner, T. C., and Davis, A. R. 2013b. Inheritance of resistance to the new race of powdery mildew in watermelon. Crop Sci. 53: 880–887. doi:10.2135/cropsci2012.07.0453
- Thakur, H., Sharma, S., and Thakur, M. 2019. Recent trends in muskmelon (Cucumis melo L.) research: an overview. J. Hortic. Sci. Biotech. 94: 533–547. doi:10.1080/14620316.2018.1561214
- Thomas, C. E. 1978. A new biological race of powdery mildew of cantaloups. Plant Dis. Rep. 62: 223.
- Thomas, C. E., Levi, A., and Caniglia, E. 2005. Evaluation of U.S. plant introductions of watermelon for resistance to powdery mildew. HortScience 40: 154–156. doi:10.21273/HORTSCI.40.1.154
- Thulin, M., and Al-Gifri, A. N. 1994. Cucumis canoxyi (Cucurbitaceae) – a new species from Yemen. Nordic J. Bot. 14: 315–317. doi:10.1111/j.1756-1051.1994.tb00610.x
- Tian, J., Zhang, G., Zhang, F., Ma, J., Wen, C., and Li, H. 2022. Genome-wide identification of powdery mildew responsive long non-coding RNAs in Cucurbita pepo. Front. Genet. 13: 933022. doi:10.3389/fgene.2022.933022
- Trecate, L., Sedláková, B., Mieslerová, B., Manstretta, V., Rossi, V., and Lebeda, A. 2019. Effect of temperature on infection and development of powdery mildew on cucumber. Plant Pathol. 68: 1165–1178. doi:10.1111/ppa.13038
- Tyagi, R., Sharma, V., Sureja, A. K., Das Munshi, A., Arya, L., and Verma, M. 2016. Deciphering population structure and diversity in Luffa cylindrica (L.) M. Roem. using morphological and sequence-related amplified polymorphism markers. Plant Genet. Resour. 14: 234–237. doi:10.1017/S1479262115000258
- Ünlü, A., Polat, I., Yildirim, A., and Onus, A. N. 2022. Mapping quantitative trait loci and developing first molecular marker for race 5 of Podosphera xanthii resistance in melon (Cucumis melo L.). Turk. J. Bot. 46: 123–133. doi:10.55730/1300-008X.2676
- USDA-ARS Germplasm Resources Information Network (GRIN). 2023. Search Accessions GRIN-Global (ars-grin.gov) (accessed Apr. 21, 2023).
- Vachev, M., Cavatorta, J., and Conrad, L. J. 2022. 'Triton’: a disease-resistant cantaloupe hybrid. HortScience 57: 1106–1109. doi:10.21273/HORTSCI16571-22
- Vakalounakis, D. J., and Klironomou, E. 2001. Taxonomy of Golovinomyces on cucurbits. Mycotaxon 80: 489–491.
- Van Schie, C. C., and Takken, F. L. 2014. Susceptibility genes 101: how to be a good host. Annu. Rev. Phytopathol. 52: 551–581. doi:10.1146/annurev-phyto-102313-045854
- Vicente-Dólera, N., Troadec, C., Moya, M., del Río-Celestino, M., Pomares-Viciana, T., Bendahmane, A., Picó, B., Román, B., and Gómez, P. 2014. First TILLING platform in Cucurbita pepo: a new mutant resource for gene function and crop improvement. PLoS ONE 9:e112743. doi:10.1371/journal.pone.0112743
- Walters, T. W., and Decker-Walters, D. 1988. Balsampear (Momordica charantia, Cucurbitaceae). Econ. Bot. 42: 286–288.
- Walters, S. A., Shetty, N. V., and Wehner, T. C. 2001. Segregation and linkage of several genes in cucumber. J. Amer. Soc. Hort. Sci. 126: 442–450. doi:10.21273/JASHS.126.4.442
- Wang, Y., Bo, K., Gu, X., Pan, J., Li, Y., and Chen, J. 2020. Molecularly tagged genes and quantitative trait loci in cucumber with recommendations for QTL nomenclature. Hort. Res. 7: 3.
- Wang, X., Li, G., Gao, X., Xiong, L., Wang, W., and Han, R. 2011. Powdery mildew resistance gene (Pm-AN) located in a segregation distortion region of melon LGV. Euphytica 180: 421–428. doi:10.1007/s10681-011-0406-1
- Wang, Y., Qi, C., Luo, Y., Zhang, F., Dai, Z., Li, M., and Qu, S. 2021b. Identification and mapping of CpPM10.1, a major gene involved in powdery mildew (race 2 France of Podosphaera xanthii) resistance in zucchini (Cucurbita pepo L.). Theor. Appl. Genet. 134: 2531–2545. doi:10.1007/s00122-021-03840-z
- Wang, Y. H., Van den Langenberg, K., Wen, C. L., Wehner, T. C., and Weng, Z. Q. 2018. QTL mapping of downy and powdery mildew resistances in PI 197088 cucumber with genotyping-by-sequencing in RIL population. Theor. Appl. Genet. 131: 597–611. doi:10.1007/s00122-017-3022-1
- Wang, Y., Wu, D., Huang, J., Tsao, S., Hwu, K., and Lo, H. 2016. Mapping quantitative trait loci for fruit traits and powdery mildew resistance in melon (Cucumis melo). Bot. Stud. 57: 19. doi:10.1186/s40529-016-0130-1
- Wang, L., Wu, X., Xing, Q., Zhao, Y., Yu, B., Ma, Y., Wang, F., and Qi, H. 2023a. PIF8-WRKY42-mediated salicylic acid synthesis modulates red light induced powdery mildew resistance in oriental melon. Plant Cell Environ. 46: 1726–1742. doi:10.1111/pce.14560
- Wang, J., Wu, X., Wang, Y., Wu, X., Wang, B., Lu, Z., and Li, G. 2023b. Genome-wide characterization and expression analysis of the MLO gene family sheds light on powdery mildew resistance in Lagenaria siceraria. Heliyon 9:e14624. doi:10.1016/j.heliyon.2023.e14624
- Wang, L. P., Wu, X., Wang, B., Xu, P., and Li, G. J. 2022. SCAR marker linked to resistance gene of powdery mildew in bottle gourd [Lagenaria siceraria (Molina) Standl] breeding line J083. J. Zhejiang Univ. 37: 119–124.
- Wang, S., Yan, W., Yang, X., Zhang, J., and Shi, Q. 2021a. Comparative methylome reveals regulatory roles of DNA methylation in melon resistance to Podosphaera xanthii. Plant Sci. 309: 110954. doi:10.1016/j.plantsci.2021.110954
- Wani, S. H., Anand, S., Singh, B., Bohra, A., and Joshi, R. 2021. WRKY transcription factors and plant defense responses: latest discoveries and future prospects. Plant Cell Rep. 40: 1071–1085. doi:10.1007/s00299-021-02691-8
- Wechter, W. P., Kousik, C., McMillan, M., and Levi, A. 2012. Identification of resistance to Fusarium oxysporum f. sp. niveum race 2 in Citrullus lanatus var. citroides plant introductions. HortScience 47: 334–338. doi:10.21273/HORTSCI.47.3.334
- Wehner, T. C. 2005-2006. Gene List 2005 for Cucumber. Cucurbit Genet. Coop. Rep. 28–29: 105–141.
- Weng, Y. 2017. The cucumber genome. In Genetics and Genomics of Cucurbitaceae; Grumet, R., Katzir, N., and Garcia-Mas, J. Eds. Springer International Publishing AG: Cham, Switzerland, pp 183–198.
- Weng, Y., and Wehner, T. C. 2016. Cucumber Gene Catalog 2017. Cucurbit Genet. Coop. Rep. 39 & 40: 17–27.
- Whitaker, T. W. 1956. The origin of the cultivated Cucurbita. Am. Nat. 90: 171–176. doi:10.1086/281923
- Whitaker, T. W., and Bemis, W. P. 1975. Origin and evolution of the cultivated Cucurbita. Bull. Torrey Bot. Club 102: 362–368. doi:10.2307/2484762
- Wilde de, W. J. J. O., and Duyfjes, B. E. E. 2010. Cucumis sativus L. forma hardwickii (Royle) W.J. de Wilde & Duyfjes and feral forma sativus. Thai Forest Bull. (Bot.) 38: 98–107.
- Wilson, J., John, C., Wohler, H., and Hoover, M. 1956. Two foreign cucumbers resistant to bacterial wilt and powdery mildew. Plant Dis. Rep. 40: 437–438.
- Win, K. T., Zhang, C., and Lee, S. 2018. Genome-wide identification and description of MLO family genes in pumpkin (Cucurbita maxima Duch.). Hortic. Environ. Biotechnol. 59: 397–410. doi:10.1007/s13580-018-0036-9
- Wu, T. Y., and Kirschner, R. 2017. A brief global review on the species of cucurbit powdery mildew fungi and new records in Taiwan. Mycol. Iran 4: 85–91.
- Wu, X., Wang, Y., Wu, X., Xu, P., Wang, B., Lu, F. Z., and Li, G. J. 2020. Genome-wide association analysis of powdery mildew resistance in bottle gourd (Lagenaria siceraria). Mol. Plant Breed. 18: 759–764.
- Wyand, R. A., and Brown, J. K. M. 2003. Genetic and forma specialis diversity in Blumeria graminis of cereals and its implications for host–pathogen co-evolution. Mol. Plant Pathol. 4: 187–198. doi:10.1046/j.1364-3703.2003.00167.x
- Xian, F., Zhang, Y., Ma, J., Zhang, X., and Yang, J. 2011. Genetic analysis of resistance to eace 2F. of Podosphaera xanthii in wild melon material 'Yuntian-930'. Acta Hortic. 918: 595–602. doi:10.17660/ActaHortic.2011.918.74
- Xiang, Y., Miller, A. N., McGrath, M., and Babadoost, M. 2020. Genotyping-by-sequencing for analysis of the genetic variation of Podosphaera xanthii, incitant of cucurbit powdery mildew. Plant Dis. 104: 951–957. doi:10.1094/PDIS-03-19-0513-RE
- Xin, M., Wang, Y., Yao, Y., Song, N., Hu, Z., Qin, D., Xie, C., Peng, H., Ni, Z., and Sun, Q. 2011. Identification and characterization of wheat long nonprotein coding RNAs responsive to powdery mildew infection and heat stress by using microarray analysis and SBS Sequencing. BMC Plant Biol. 11: 61. doi:10.1186/1471-2229-11-61
- Xu, L., He, Y., Tang, L., Xu, Y., and Zhao, G. 2022. Genetics, genomics, and breeding in melon. Agronomy 12: 2891. doi:10.3390/agronomy12112891
- Xu, X., Liu, X., Tan, M., Qi, X., Xu, Q., and Chen, X. 2020. First report of powdery mildew caused by Podosphaera xanthii on Cucumis dipsaceus in China. Plant Dis. 104: 985–985. doi:10.1094/PDIS-08-19-1577-PDN
- Xu, X., Liu, X., Yan, Y., Wang, W., Gebretsadik, K., Qi, X., Xu, Q., and Chen, X. 2019. Comparative proteomic analysis of cucumber powdery mildew resistance between a single-segment substitution line and its recurrent parent. Hortic. Res. 6: 115. doi:10.1038/s41438-019-0198-3
- Xu, Q., Shi, Y., Yu, T., Xu, X., Yan, Y., Qi, X., and Chen, X. 2016a. Whole-genome resequencing of a cucumber chromosome segment substitution line and its recurrent parent to identify candidate genes governing powdery mildew resistance. PLoS ONE 11:e 0164469. doi:10.1371/journal.pone.0164469
- Xu, K., and Wang, P. 2022. Genome-wide identification and expression analysis of the VQ gene family in Cucurbita pepo L. PeerJ 10:e12827. doi:10.7717/peerj.12827
- Xu, Q., Xu, X., Shi, Y., Qi, X., and Chen, X. 2017. Elucidation of the molecular responses of a cucumber segment substitution linecarrying Pm5.1 and its recurrent parent triggered by powdery mildew by comparative transcriptome profiling. BMC Genomics 18: 21. doi:10.1186/s12864-016-3438-z
- Xu, X., Yu, T., Xu, R., Shi, Y., Lin, X., Xu, Q., Qi, X., Weng, Y., and Chen, X. 2016b. Fine mapping of a dominantly inherited powdery mildew resistance major-effect QTL, Pm1.1, in cucumber identifies a 41.1 kb region containing two tandemly arrayed cysteine-rich receptor-like protein kinase genes. Theor. Appl. Genet. 129: 507–516. doi:10.1007/s00122-015-2644-4
- Yadav, V., Wang, Z. Y., Lu, G., Sikdar, A., Yang, X. Z., and Zhang, X. 2021a. Evaluation of watermelon germplasm and advance breeding lines against powdery mildew race ´2F'. Pak. J. Agri. Sci. 58: 321–330.
- Yadav, V., Wang, Z. Y., Yang, X. Z., Wei, C. H., Changqing, X., and Zhang, X. 2021b. Comparative analysis, characterization and evolutionary study of dirigent gene family in Cucurbitaceae and expression of novel dirigent peptide against powdery mildew stress. Genes 12: 326. doi:10.3390/genes12030326
- Yadav, V., Wang, Z., Guo, Y., and Zhang, X. 2022. Comparative transcriptome profiling reveals the role of phytohormones and phenylpropanoid pathway in early-stage resistance against powdery mildew in watermelon (Citrullus lanatus L.). Front. Plant Sci. 13: 1016822. doi:10.3389/fpls.2022.1016822
- Yang, J., Deng, G., Lian, J., Garraway, J., Niu, Y., Hu, Z., Yu, J., and Zhang, M. 2020. The chromosome-scale genome of melon dissects genetic architecture of important agronomic Traits. iScience 23: 101422. doi:10.1016/j.isci.2020.101422
- Yang, L., Koo, D. H., Li, Y., Zhang, X., Luan, F., Havey, M. J., Jiang, J., and Weng, Y. 2012. Chromosome rearrangements during domestication of cucumber as revealed by high density genetic mapping and draft genome assembly. Plant J. 71: 895–906. doi:10.1111/j.1365-313X.2012.05017.x
- Yang, S., Zhu, C., Chen, J., Zhao, J., Hu, Z., Liu, S., and Zhou, Y. 2022. Identification and expression profile analysis of the OSCA gene family related to abiotic and biotic stress response in cucumber. Biology 11: 1134. doi:10.3390/biology11081134
- Yashiro, K., Iwata, H., Akashi, Y., Tomita, K. O., Kuzuya, M., Tsumura, Y., and Kato, K. 2005. Genetic relationship among East and South Asian melon (Cucumis melo L.) revealed by AFLP analysis. Breed. Sci. 55: 197–206. doi:10.1270/jsbbs.55.197
- Yeh, Y. W., Wu, T. Y., Wen, H. L., Jair, H. W., Lee, M. Z., and Kirschner, R. 2021. Host plants of the powdery mildew fungus Podosphaera xanthii in Taiwan. Trop. Plant Pathol. 46: 44–61. doi:10.1007/s40858-020-00393-2
- Yetişir, H., Şakar, M., and Serçe, S. 2008. Collection and morphological characterization of Lagenaria siceraria germplasm from the Mediterranean region of Turkey. Genet. Resour. Crop Evol. 55: 1257–1266. doi:10.1007/s10722-008-9325-y
- Yong, X., and Guo, S. 2017. The watermelon genome. In Genetics and Genomics of Cucurbitaceae; Grumet, R., Katzir, N., and Garcia-Mas, J. Eds. Springer International Publishing AG.: Cham, Switzerland, pp 199–210.
- Yuste-Lisbona, F. J., Capel, C., Gómez-Guillamón, M. L., Capel, J., López-Sesé, A. I., and Lozano, R. 2011a. Codominant PCR-based markers and candidate genes for powdery mildew resistance in melon (Cucumis melo L.). Theor. Appl. Genet. 122: 747–758. doi:10.1007/s00122-010-1483-6
- Yuste-Lisbona, F.J., Capel, C., Sarria, E., Gómez-Guillamón, M.L., Capel, J., Lozano, R., and López-Sesé, A.I. 2010b. Construction of a genetic linkage map and identification of a major QTL conferring resistance to powdery mildew in the melon genotype TGR-1551. Acta Hortic. 871: 179–186. doi:10.17660/ActaHortic.2010.871.22
- Yuste-Lisbona, F. J., Capel, C., Sarria, E., Torreblanca, R., Gómez-Guillamón, M. L., Capel, J., Lozano, R., and López-Sesé, A. I. 2011b. Genetic linkage map of melon (Cucumis melo L.) and localization of a major QTL for powdery mildew resistance. Mol. Breeding 27: 181–192. doi:10.1007/s11032-010-9421-5
- Yuste-Lisbona, F. J., López-Sesé, A. I., and Gómez-Guillamón, M. L. 2010a. Inheritance of resistance to races 1, 2 and 5 of powdery mildew in the melon TGR-1551. Plant Breed. 129: 72–75. doi:10.1111/j.1439-0523.2009.01655.x
- Zhang, Q. 2011. New seed of Cucurbita pepo useful for producing plants with improved desired traits e.g. disease resistance, herbicide resistance and insect or pest resistance. Patent Number(s): US2011004954-A1; US8558069-B2. Patent Assignee: HOLLAR SEEDS (HOLL-Non-standard). Derwent Primary Accession Number: 2011-A34664
- Zhang, Q. 2013a. Inbred Cucurbita pepo pumpkin HSPMR7B1 having a mutant allele for powdery mildew resistance. Patent Number: US 08558069. Patent Assignee: Hollar Seeds. Official Gazette of the United States Patent and Trademark Office Patents Published: OCT 15 2013.
- Zhang, Q. 2013b. New Cucurbita pepo plant containing mutant allele Pm2, useful for developing further pumpkin hybrids with desired traits, e.g. resistance to powdery mildew, herbicide resistance, and insect resistance. Patent Number(s): US2013283463-A1, Patent Assignee: HOLLAR SEEDS (HOLL-Non-standard). Derwent Primary Accession Number: 2013-S68132
- Zhang, C. Y., Anarjan, M. B., Win, K. T., Begum, S., and Lee, S. H. 2021a. QTL-seq analysis of powdery mildew resistance in a Korean cucumber inbred line. Theor. Appl. Genet. 134: 435–451. doi:10.1007/s00122-020-03705-x
- Zhang, T., Cui, H., Luan, F., Liu, H., Ding, Z., Amanullah, S., Zhang, M., Ma, T., and Gao, P. 2023a. A recessive gene Cmpmr2F confers powdery mildew resistance in melon (Cucumis melo L.). Theor. Appl. Genet. 136: 4. doi:10.1007/s00122-023-04269-2
- Zhang, H., Guo, S., Gong, G., Ren, Y., Davis, A. R., and Xu, Y. 2011b. Sources of resistance to race 2WF powdery mildew in US watermelon plant introductions. HortScience 46: 1349–1352. doi:10.21273/HORTSCI.46.10.1349
- Zhang, H., Hu, W., Hao, J., Lv, S., Wang, C., Tong, W., Wang, Y., Wang, Y., Liu, X., and Ji, W. 2016. Genomewide identification and functional prediction of novel and fungi-responsive lincRNAs in Triticum aestivum. BMC Genomics 17: 238. doi:10.1186/s12864-016-2570-0
- Zhang, H., Li, X., Yu, H., Zhang, Y., Li, M., Wang, H., Wang, D., Wang, H., Fu, Q., Liu, M., Ji, C., Ma, L., Tang, J., Li, S., Miao, J., Zheng, H., and Yi, H. 2019. A high-quality melon genome assembly provides insights into genetic basis of fruit trait improvement. iScience 22: 16–27. doi:10.1016/j.isci.2019.10.049
- Zhang, S., Liu, M., Miao, H., Zhang, S., Yang, Y., Xie, B., and Gu, X. 2011a. QTL mapping of resistance genes to powdery mildew in cucumber (Cucumis sativus L.). Sci. Agric. Sin. 44: 3584–3593.
- Zhang, S., Liu, J., Xu, B., and Zhou, J. 2021c. Differential responses of Cucurbita pepo to Podosphaera xanthii reveal the mechanism of powdery mildew disease resistance in pumpkin. Front. Plant Sci. 12: 633221. doi:10.3389/fpls.2021.633221
- Zhang, Q., Medina, A., and Lyerly, C. 2016. 'Eight BallPlus’, ‘Six Ball’, ‘Cue BallPlus’, and ‘One BallPlus’: new ball summer squash cultivars. HortScience 51: 459–461. doi:10.21273/HORTSCI.51.4.459
- Zhang, C., Ren, Y., Guo, S., Zhang, H., Gong, G., Du, Y., and Xu, Y. 2013. Application of comparative genomics in developing markers tightly linked to the Pm-2F gene for powdery mildew resistance in melon (Cucumis melo L.). Euphytica 190: 157–168. doi:10.1007/s10681-012-0828-4
- Zhang, L. B., Simmons, M. P., Kocyan, A., and Renner, S. S. 2006. Phylogeny of the Cucurbitales based on DNA sequences of nine loci from three genomes: implications for morphological and sexual system evolution. Mol. Phylogenet. Evol. 39: 305–322. doi:10.1016/j.ympev.2005.10.002
- Zhang, H., Wang, Z., Mao, A., Zhang, F., Wang, Y., and Xu, Y. 2008. SSR markers linked to the resistant gene of cucumber powdery mildew. Acta Agri. Boreali. Sin. 23: 77–80.
- Zhang, K., Wang, X., Zhu, W., Qin, X., Xu, J., Cheng, C., Lou, Q., Li, J., and Chen, J. 2018b. Complete resistance to powdery mildew and partial resistance to downy mildew in a Cucumis hystrix introgression line of cucumber were controlled by a co-localized locus. Theor. Appl. Genet. 131: 2229–2243. doi:10.1007/s00122-018-3150-2
- Zhang, Z., Wang, K., Chen, C., Tian, S., Wu, J., Li, J., Kong, L., Yang, X., Zhang, C., Li, Y., Zhu, H., and Xiao, D. 2023b. Transcriptome sequence analysis of defense response of resistant and susceptible bottle gourd to powdery mildew. Agronomy 13: 1406. doi:10.3390/agronomy13051406
- Zhang, C., Zhu, Q., Liu, S., Gao, P., Zhu, Z., Wang, X., and Luan, F. 2018a. The complete chloroplast genome sequence of the Cucurbita pepo L. (Cucurbitaceae). Mitochondrial DNA B Resour. 3: 717–718. doi:10.1080/23802359.2018.1483766
- Zhang, P., Zhu, Y., and Zhou, S. 2020. Comparative transcriptomic analyses of powdery mildew resistant and susceptible cultivated cucumber (Cucumis sativus L.) varieties to identify the genes involved in the resistance to Sphaerotheca fuliginea infection. PeerJ 8:e8250. doi:10.7717/peerj.8250
- Zhang, P., Zhu, Y., and Zhou, S. 2021b. Comparative analysis of powdery mildew resistant and susceptible cultivated cucumber (Cucumis sativus L.) varieties to reveal the metabolic responses to Sphaerotheca fuliginea infection. BMC Plant Biol. 21: 24. doi:10.1186/s12870-020-02797-3
- Zhao, G., Lian, Q., Zhang, Z., Fu, Q., He, Y., Ma, S., Ruggieri, V., Monforte, A. J., Wang, P., Julca, I., Wang, H., Liu, J., Xu, Y., Wang, R., Ji, J., Xu, Z., Kong, W., Zhong, Y., Shang, J., Pereira, L., Argyris, J., Zhang, J., Mayobre, C., Pujol, M., Oren, E., Ou, D., Wang, J., Sun, D., Zhao, S., Zhu, Y., Li, N., Katzir, N., Gur, A., Dogimont, C., Schaefer, H., Fan, W., Bendahmane, A., Fei, Z., Pitrat, M., Gabaldón, T., Lin, T., Garcia-Mas, J., Xu, Y., and Huang, S. 2019. A comprehensive genome variation map of melon identifies multiple domestication events and loci influencing agronomic traits. Nat. Genet. 51: 1607–1615. doi:10.1038/s41588-019-0522-8
- Zheng, Y.-H., Alverson, A. J., Wang, Q. F., and Palmer, J. D. 2013. Chloroplast phylogeny of Cucurbita: Evolution of the domesticated and wild species. J. Syst. Evol. 51: 326–334. doi:10.1111/jse.12006
- Zheng, R. Y., and Chen G. Q., 1981. The genus Erysiphe in China. Sydowia 34: 214–327.
- Zheng, L., Zhang, M., Zhuo, Z., Wang, Y., Gao, X., Li, Y., Liu, W., and Zhang, W. 2021. Transcriptome profiling analysis reveals distinct resistance response of cucumber leaves infected with powdery mildew. Plant Biol. (Stuttg) 23: 327–340. doi:10.1111/plb.13213
- Zhou, X., Cui, J., Cui, H., Jiang, N., Hou, X., Liu, S., Gao, P., Luan, Y., Meng, J., and Luan, F. 2020. Identification of lncRNAs and their regulatory relationships with target genes and corresponding miRNAs in melon response to powdery mildew fungi. Gene 735: 144403. doi:10.1016/j.gene.2020.144403
- Zhou, J. G., Hu, H. L., Li, X. Z., Zhou, R. J., and Zhang, H. R. 2010. Identification of a resource of powdery mildew resistance in Cucurbita moschata. Acta Hortic. 871: 141–146. doi:10.17660/ActaHortic.2010.871.17
- Zhu, Q., Gao, P., Wan, Y., Cui, H., Fan, C., Liu, S., and Luan, F. 2018. Comparative transcriptome profiling of genes and pathways related to resistance against powdery mildew in two contrasting melon genotypes. Sci. Hortic. 227: 169–180. doi:10.1016/j.scienta.2017.09.033
- Zhu, L., Li, Y., Li, J., Wang, Y., Zhang, Z., Wang, Y., Wang, Z., Hu, J., Yang, L., and Sun, S. 2021. Genome-wide identification and analysis of the MLO gene families in three Cucurbita species. Czech J. Genet. Plant Breed. 57: 119–123. doi:10.17221/99/2020-CJGPB
- Zhuang, F.-Y., Chen, J.-F., Staub, J. E., and Qian, C.-T. 2006. Taxonomic relationships of a rare Cucumis species (C. hystrix Chakr.) and its interspecific hybrid with cucumber. HortScience 41: 571–574. doi:10.21273/HORTSCI.41.3.571
- Zijlstra, S., and Groot, S. P. C. 1992. Search for novel genes for resistance to powdery mildew (Sphaerotheca fuliginea) in cucumber (Cucumis sativus). Euphytica 64: 31–37. doi:10.1007/BF00023535
- Zijlstra, S., Jansen, R. C., and Groot, S. P. C. 1995. The relationship between powdery mildew (Sphaerotheca fuliginea) resistance and leaf chlorosis sensitivity in cucumber (Cucumis sativus) studied in sigle seed descent lines. Euphytica 81: 193–198. doi:10.1007/BF00025433
- Zou, S., Xu, Y., Li, Q., Wei, Y., Zhang, Y., and Tang, D. 2023. Wheat powdery mildew resistance: from gene identification to immunity deployment. Front. Plant Sci. 14: 1269498. doi:10.3389/fpls.2023.1269498