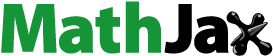
ABSTRACT
Beef production on semi-natural grassland provides meat and nature conservation of biodiverse habitats. In this study, heifers born to a dairy cow and an Angus (ANG) or Charolais (CHA) sire were allocated to a HIGH or LOW production system, varying in indoor feed intensity, number of summers on grass and slaughter age. CHA crosses had higher liveweight gain, conformation score, and proportion of high-value retail cuts than ANG crosses, which had higher scores for fatness and marbling. The LOW system gave lower liveweight gain and carcasses with lower fatness scores than the HIGH system. CHA-LOW had leaner carcasses than the other groups. Per kilogram salable meat produced, ANG-HIGH, CHA-HIGH, ANG-LOW, and CHA-LOW resulted in 18, 15, 58, and 46 m2 grazed semi-natural grassland. These results indicate that dairy × beef heifers can be used for nature conservation grazing of semi-natural grassland while still achieving acceptable performance and market-oriented carcass characteristics.
Introduction
Biodiversity is in critical condition globally (Brondizio et al., Citation2019), including in the European Union (EU) (European Environment Agency, Citation2020). Natural grassland is among the EU habitat types showing a strong deteriorating trend, with 75% of all habitats in poor or very poor condition (European Environment Agency, Citation2020). In Sweden, grassland is the most threatened natural habitat (Toräng & Jacobson, Citation2019). Grasslands in anthropogenic landscapes are often traditional extensively managed semi-natural areas used for mowing or grazing in low-intensity livestock systems. The greatest threat to grassland biodiversity is cessation of farming and subsequent overgrowth (European Environment Agency, Citation2020). Increasing the area of extensively managed semi-natural grassland has therefore been defined as an important measure to prevent species and habitat losses (European Environment Agency, Citation2020). To counteract loss of biodiversity, the European Commission recently proposed a nature restoration law, passed through the EU Parliament, whereby at least 20% of all grasslands and other habitats must be subjected to restoration measures in coming years (European Commission, Citation2022; European Climate, Infrastructure, and Environment Executive Agency, Citation2023).
Extensive pasture-based animal husbandry is one of few agricultural production systems that can increase biodiversity in a landscape (Eriksson, Citation2022). The concept of High Nature Value Farming has developed from a growing recognition that conservation of biodiversity in Europe depends partly on the continuation of traditional low-intensity farming systems. High Nature Value Farming is carried out in all European countries and delivers unique local products (European Forum on Nature Conservation and Pastoralism, Citation2023). Local concepts of beef produced on semi-natural grassland have been a niche product for decades, including in Sweden (e.g. Plateryd, Citation2004). Business opportunities for Swedish farmers to produce pasture beef have increased dramatically in recent years, and there is now a national third-party certification system for beef and lamb meat (Svenskt Sigill, Citation2023). There also is a competitive customer, as one of the three largest food retailers in Sweden runs a national concept for pasture beef, for which consumer demand is steadily increasing (Coop, Citation2023). In addition to added value of the product, farmers in Sweden, and in many other European countries, can receive economic support to keep grazing livestock on semi-natural grassland (Swedish Board of Agriculture, Citation2023).
In the past, most Swedish dairy cows were crossed with bulls of the same dairy breed, but use of beef breed semen in dairy cows has almost quadrupled in the past decade, from 4.2% to 18.3% of dairy cow inseminations (Växa Sverige, Citation2023). Superior weight gain and carcass traits of dairy × beef crossbred compared to pure-bred dairy beef, often under intensive rearing and/or with maize-based feed, has been demonstrated in previous studies (Jukna et al., Citation2009; Huuskonen et al., Citation2013; Mordenti et al., Citation2019; Eriksson et al., Citation2020; McCabe, Citation2021; Basiel & Felix, Citation2022). More extensive rearing of dairy × beef crossbred steers on forage and semi-natural grasslands has also been evaluated (Hessle et al., Citation2019; Holmström et al., Citation2021). However, heifers comprise half the offspring from dairy cow × beef breed crosses, so studies are needed on dairy × beef heifers in production systems using semi-natural grassland. The aim of this study was therefore to investigate the effects of dam breed, sire breed, and intensity of production system on animal performance and carcass characteristics in dairy × beef heifer production based on forage and well-managed semi-natural grassland.
Materials and methods
Experimental design
The experiment was conducted at the SLU Götala Beef and Lamb Research Centre, Skara, south-western Sweden (58°42′N, 13°21′E; elevation 150 m asl.) from 9 October 2019 to 20 April 2022, where the experimental protocol and execution of the study were approved by the Ethics Committee on Animal Experiments in Gothenburg (ID number 002530). During indoor periods, the animals were kept in groups of six in pens with deep straw bedding, while during grazing periods all animals grazed semi-natural grassland.
The animals were followed from weaning to slaughter in an experiment with a 2 × 2 × 2 factorial design, comparing two sire breeds (Angus (ANG) and Charolais (CHA)), two dam breeds (Swedish Red (SR) and Swedish Holstein (SH)) and two production systems (moderately high (HIGH) and Low (LOW) indoor feed intensity). Apart from indoor feed intensity, the two production systems also differed in terms of slaughter age and number of summers on grass. The two systems were chosen to reflect possible rearing strategies combining grazing for nature conservation and production of market-oriented carcasses. Animals were continuously introduced into the experiment according to birth date and slaughtered individually when they reached their target age.
Animals
A total of 72 heifer calves, acquired from commercial farms, were included in the study. After the colostrum period, they were housed in groups and fed at least 5 L whole milk and/or milk replacer per day plus ad libitum tender forage and concentrate. The calves were dehorned at 6–8 weeks of age and entered the experimental station as weanlings at 11–14 weeks of age. They were of four different breed combinations, with 18 animals in each group: Angus × Swedish Red, Angus × Swedish Holstein, Charolais × Swedish Red, and Charolais × Swedish Holstein. Swedish Red and Swedish Holstein were choosen as these breeds are the two most common ones in Sweden, whereas Angus was choosen to represent an early maturing beef breed, and Charolais to represent a late maturing breed. Each calf had a unique dam descending from 32 SR and 38 SH sires (maternal grandsires of the calves) and they were offspring of a total of eight different Angus sires and eight different Charolais sires with genetic merits close to the Swedish average breeding values at this time. Birth date ranged from 19 April to 27 August 2020 for the HIGH group and from 27 June to 8 October 2019 for the LOW group, where the birth date ranges differed in order to suit the planned slaughter time slots in the two production systems. All calves were in good health during both the pre-experimental and experimental period, and data from all animals were included in statistical analyses.
Production systems
Half of the calves in each of the four breed groups were reared in the HIGH production system and the other half in the LOW system. The HIGH system involved moderately high feed intensity during two indoor periods with one intermediate grazing period, and slaughter at 20 months of age. The LOW system involved low feed intensity during three indoor periods with two intermediate grazing periods, and slaughter at 27 months of age.
Diets during indoor periods
At the start of the study, all animals were fed ad libitum (defined as 105% of voluntary intake) with total mixed rations consisting of grass-clover silage, rolled barley, rolled peas, and rapeseed meal (). Feed composition for each pen was adjusted when the average liveweight of the two lightest heifers in the pen reached the minimum weight for the next decrease in protein/energy ratio according to Swedish recommendations (Spörndly, Citation2003). For heifers in the LOW system, forage was fed ad libitum as the sole feed from 225 kg liveweight until slaughter. The forage consisted of grass-clover silage during the remaining part of indoor period 1 and the fully indoor periods 2 and 3 (), with pasture herbage during the intermediate grazing periods. The grass-clover silage was taken from the first and second cuts and consisted of 90–95% grass (Lolium perenne, Festuca pratensis, Phleum pratense, Festulolium arundinacea L.) and 5–10% clover (Trifolium repens, Trifolium pratense) and herbage that was pre-wilted and chopped before fermentation. A preservative containing benzoate, nitrite, methenamine, and propionate was used at a rate of 3 L ton−1 herbage (Konsil Ultra, Konsil Scandinavia, Tvååker, Sweden). The herbage was harvested at least four months before feeding. Silage from bunker silos was fed when consumption rate was fast enough to ensure high hygienic quality, whereas silage from round bales was fed when there were few and/or small animals eating the batch.
Table 1. Proportion (% of dry matter) of grass-clover silage, rolled barley, rolled peas, and cold-pressed rapeseed cake in the total mixed ration fed to dairy × beef heifers at different liveweights in production systems with moderately high (HIGH) or low (LOW) indoor feed intensity (IP = indoor period, IP3 for LOW only).
Table 2. Chemical composition of rolled barley, rolled peas, and cold-pressed rapeseed cake (n = 1) fed to dairy × beef heifers in production systems with moderately high (HIGH) or low (LOW) indoor feed intensity (DM = dry matter, ME = metabolizable energy, CP = crude protein, NDF = neutral detergent fiber).
Table 3. Chemical composition of grass-clover silage fed during indoor period (IP) 1, 2, and 3 to dairy × beef heifers in production systems with moderately high (HIGH) or low (LOW) indoor feed intensity (s.d. = standard deviation, DM = dry matter, ME = metabolizable energy, CP = crude protein, NDF = neutral detergent fiber, NH4-N = ammonium-nitrogen).
Table 4. Feed amounts and areas used to dairy × beef heifers with two sire breeds (Angus (ANG) or Charolais (CHA)) in production systems with moderately high indoor feed intensity and 20 months slaughter age (HIGH) or low indoor feed intensity and 27 months slaughter age (LOW), (DM = dry matter, ha = hectare).
During the indoor periods, the heifers were fed once a day. Refusals were weighed and then disposed of three times a week. Daily feed intake was recorded individually and continuously using automatic feed mangers placed on weighing cells (Biocontrol, CRFI, Rakkestad, Norway), and net average feed consumption per animal was calculated weekly. During the entire experiment, the feed rations were supplemented with vitaminized minerals to meet the requirements of the animals (Spörndly, Citation2003), and all animals had free access to water and salt.
Silage samples for analysis of dry matter (DM) content were collected daily and pooled to one sample per week, silage samples for analysis of chemical composition were taken daily and pooled to one sample per month, and silage samples for analysis of fermentation quality were taken weekly and pooled to one sample per silo or batch of round bales. Samples of barley, peas, and rapeseed meal were collected weekly and pooled to one sample per feed and indoor period for analysis of chemical composition.
Grazing periods
The first grazing period for the heifers in the LOW system ran from May 6 to October 23, 2020. All these heifers were turned out again for their second grazing period on May 5, 2021, and brought in and housed on an individual basis eight weeks before their individual slaughter occasion (from August 3 to November 17, 2021). The single grazing period for the heifers in the HIGH system ran from May 5 to September 1, 2021. All heifers in both systems were kept in one group that was rotated between four enclosures every 7–14 days. The heifers were offered water, salt, and a vitaminized mineral supplement ad libitum throughout the grazing periods.
The pasture consisted of permanent, unfertilized semi-natural grassland with approximately 20% dry, 60% mesic, and 20% wet areas, where the grassland area was 19.5 ha in 2020 and 37.3 ha in 2021. The pasture was mainly open, but included small areas of mixed deciduous trees. The dominant plant species was Deschampsia cespitosa (tufted hairgrass), but Festuca rubra (red fescue) was also prominently present. In dry areas, F. ovina (sheep’s fescue), D. flexuosa (wavy hairgrass), Nardus stricta (matgrass), and several herb species were abundant. Besides D. cespitosa and F. rubra, herbs were prevalent in mesic areas, while D. cespitosa and Cyperaceae (sedges and rushes) were dominant in wet areas.
Sward height and chemical composition of the pasture herbage were measured every time the animals changed enclosures, both in the enclosure they left and the enclosure they entered. In each enclosure, sward height measurement followed a W-shaped route according to Frame (Citation1993), with 60–70 recordings performed with a rising plate meter (0.3 × 0.3 m, weight 430 g). To estimate chemical composition, 12–15 herbage samples were cut with a handheld machine in 3-m diameter circles along the route and pooled to one sample per month ().
Figure 1. Herbage concentrations of crude protein (CP), metabolizable energy (ME), and neutral detergent fiber (NDF), and sward height in Deschampsia cespitosa-dominated semi-natural grassland grazed from May until October by dairy × beef heifers in production systems with moderately high (HIGH) or low (LOW) feed intensity. 2020 was the first grazing period for heifers in the LOW system, while 2021 was the second grazing period for heifers in the LOW system and the first for heifers in the HIGH system.
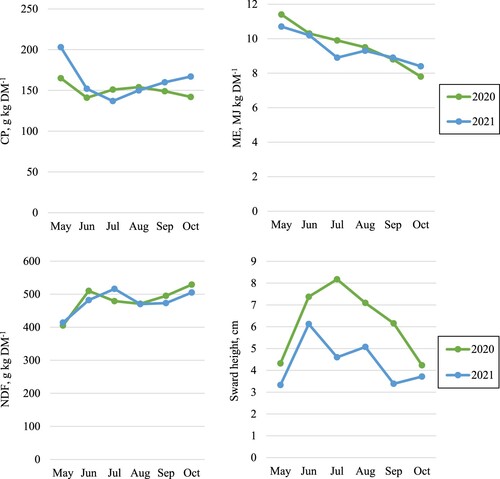
Sward height at the end of the grazing periods was visually determined to be short enough not to accumulate litter, and hence qualified for agri-environmental payments for preserving grassland biodiversity (Swedish Board of Agriculture, Citation2023).
Managed grassland area per heifer was estimated based on pasture area, number of heifers, and individual animal liveweight, weight gain, and breed (Spörndly, Citation2003; ).
Chemical analysis
Silage, barley, peas, rapeseed cake, and pasture herbage samples were analyzed for concentrations of DM, crude protein (CP), neutral detergent fiber (NDF), and in vitro organic matter digestibility. The DM concentration in silage and pasture herbage was determined by drying at 60°C and 130°C, respectively, for 24 h, while ash content was determined by incineration at 550°C for 5 h. Crude protein was determined according to Dumas (Citation1831) and NDF according to Chai and Udén (Citation1998). Metabolizable energy (ME) concentration in silage and pasture herbage was calculated based on in vitro disappearance of rumen organic matter according to Lindgren (Citation1979), and ME concentration in barley, peas, and rapeseed cake was calculated according to Axelsson (Citation1941). Concentrations of starch and crude fat were determined in barley, peas, and rapeseed cake (Åman & Hesselman, Citation1984; EU Council Directive 1998/64/EC, Citation1998). In addition, silage samples were analyzed for pH and concentration of ammonium-nitrogen (NH4-N) (Tecator Kjeltec Auto sample system 1035 Analyzer, Tecator Inc., Höganäs, Sweden; Andersson & Hedlund, Citation1983).
The chemical composition of the grass-clover silages and concentrates fed during indoor periods is shown in and , while the chemical composition of the pasture herbage is shown in .
Carcass measurements
The heifers were transported to a local commercial abattoir for slaughter. After slaughter, the carcasses were divided along the vertebral column and weighed. The cold carcass weight was estimated as 0.98 × hot carcass weight. Carcass conformation and fat cover were graded according to the European Union Carcass Classification Scheme (EUROP; Council of the European Union, No 1234/Citation2007; Commission of the European Union, No 1249/Citation2008). In assessing conformation, i.e. development of the carcass profile, the round, back, and shoulder in particular were taken into consideration according to the EUROP classification system (E: excellent, U: very good, R: good, O: fair, P: poor). In assessing fat cover, the amount of subcutaneous fat and fat deposits in the thoracic cavity were taken into account, using a classification range from 1 to 5 (1: low, 2: slight, 3: average, 4: high, 5: very high). Based on the Swedish system (SJVFS Citation127, Citation1998), each level of the conformation and fatness scales was subdivided into three sub-classes (e.g. O−, O, O+; 3−, 3, 3+) to produce a transformed scale ranging from 1 to 15, with 15 being the best conformation and highest fatness.
After cooling for two days at +2–4°C, the carcasses were split into fore- and hindquarters between the 10th and 11th ribs. Marbling was determined visually on right hindquarters in musculus (M.) longissimus dorsi on a 10-point scale with steps of 0.5 from 1 (no marbling) to 5 (slightly abundant), based on the USDA standard (United States Department of Agriculture, Citation2018). The right hindquarter from each animal was weighed, as were seven high-value retail cuts: strip loin (M. longissimus dorsi), fillet (M. psoas major), topside (M. semimembranosus), outside round (M. biceps femoris), eye of round (M. semitendinosus), top rump (M. quadriceps femoris), and rump steak (M. gluteus medius). Trim fat, bones, and two commercially defined cuts from the hindquarter (grade 2 and grade 3 bone-free mincing and stewing meat, assumed to contain 10% and 23% fat, respectively) were also weighed. Trim fat was defined as subcutaneous and intermuscular fat deposits separable with a knife in a standardized cutting procedure. Bones were weighed together with closely bound connective tissue capsules and without extra cleaning of the bones. Dressing proportion (cold carcass weight:liveweight ratio) and proportion of retail cuts, trim fat, bones, and the two commercial cuts from the hindquarter were calculated.
Statistical analysis
The data were analyzed with the Mixed procedure in SAS (Citation2018), where the individual animal was regarded as nested within its pen. To assess the variation in birth date between the animals, relative age was included as a covariate in the model:
where µ is the population mean, αi is the fixed effect of sire breed, βj is the fixed effect of dam breed, γk is the fixed effect of production system, αβij is the interaction between sire breed and dam breed, αγik is the interaction between sire breed and production system, βγjk is the interaction between dam breed and production system, αβγijk is the interaction between sire breed, dam breed, and production system, cl is the random effect of pen, covijkl is the covariate relative age (i.e. number of days each individual heifer is born later than the oldest heifer in the production system), and eijklm is the error term.
Dietary intake data were analyzed separately for each indoor period. Liveweight gain data were analyzed for each indoor and grazing period, and also averaged over the rearing period from weaning to slaughter. Means were compared pairwise using LSD0.05-tests, adjusted by the method of Kenward and Roger (Citation2009), and differences were considered significant at p < 0.05 or showing a tendency for significance at 0.05 < p < 0.10.
Results
In general, sire breed and production system had the greatest effects on performance of the beef heifers ( and ). Dam breed effected only a few traits and is therefore presented in text and footnotes only. No two-way interaction with dam breed was observed, but three-way interactions between dam breed, sire breed, and production system were found for energy intake in relation to liveweight gain during indoor period 1 (p = 0.0424) and total liveweight gain from weaning to slaughter (p = 0.0159).
Dietary intake, liveweight gain, and feed efficiency
The CHA crosses had higher weight at the start of indoor period 1 (128 vs. 113 kg) and 2 (420 vs. 387 kg) than ANG crosses (). In relation to liveweight, average daily DM intake in CHA crosses was lower than in ANG crosses during indoor period 1 (2.80 vs. 2.96%) and 2 (2.19 vs. 2.32%) and it also tended to be lower during indoor period 3, although daily intake expressed in kg DM was higher in CHA crosses than in ANG crosses during the latter period (). Aside from a tendency for higher liveweight gain in CHA crosses compared with ANG crosses during grazing period 1, no effect of sire breed on liveweight gain during any specific period could be found. However, for the entire rearing period from weaning until slaughter, CHA crosses had higher daily liveweight gain than ANG crosses (0.93 vs. 0.89 kg).
The SH crosses had higher overall daily liveweight gain from weaning until slaughter than SR crosses (0.93 vs. 0.90 g; p = 0.0156) and also tended to have higher feed efficiency during indoor period 1 (p = 0.0596).
Heifers in the HIGH system had higher DM intake than heifers in the LOW system during indoor period 1 (7.24 vs. 6.13 kg) and 2 (11.71 vs. 11.45 kg). During indoor period 2, intake of DM (2.47 vs. 2.04%) and NDF (1.16 vs. 1.04%) expressed in relation to liveweight were also higher for heifers in the HIGH system than for heifers in the LOW system. Energy intake in relation to weight gain was higher in the HIGH system than in the LOW system during indoor period 1 (75.9 vs. 63.5 MJ ME kg gain day−1), whereas it was lower in the HIGH system during indoor period 2 (101.7 vs. 127.7 MJ ME kg gain day−1). Heifers in the HIGH system tended to show higher liveweight gain during indoor period 1 than heifers in the LOW system. This higher liveweight gain was followed by lower weight gain during the subsequent first grazing period 1 (−0.128 vs. 0.611 kg day−1). For the heifers in the LOW system, this period was in year 2020, when the average sward height was 6.2 cm and the stocking rate was 690 kg liveweight ha−1. For the heifers in the HIGH system, their first grazing period in year 2021 corresponded to an average sward height of 4.3 cm and the stocking rate 740 kg liveweight ha−1 (). During the subsequent indoor period 2, heifers in the HIGH system gained more weight than heifers in the LOW system (1.53 vs. 0.85 kg day−1). During indoor period 2, there was a tendency for an interaction between production system and sire breed (p = 0.0903), with ANG crosses gaining more weight than CHA crosses in the LOW system, but less weight than CHA crosses in the HIGH system (). For the entire rearing period from weaning until slaughter, heifers in the HIGH system gained 1.01 kg day−1, whereas heifers in the LOW system gained 0.82 kg day−1 (p < 0.0001).
Table 5. Daily feed intake (I = intake; DM = dry matter; NDF = neutral detergent fiber), daily liveweight (LW) gain, and feed efficiency (FE; MJ = megajoule metabolizable energy) of dairy × beef heifers (n = 18) with two sire breeds (Angus (ANG) or Charolais (CHA)) in production systems (System) with moderately high indoor feed intensity and 20 months slaughter age (HIGH) or low indoor feed intensity and 27 months slaughter age (LOW). Least square means, pooled standard error of the mean (s.e.), and significance of the main effects of sire breed, production system, and their interaction.
Carcass characteristics
Sire breed influenced almost all carcass characteristics (). Thus CHA crosses were heavier (liveweight at slaughter 708 vs. 670 kg, carcass weight 360 vs. 337 kg) and had higher conformation score (7.2 vs. 6.6), but lower scores of fatness (9.3 vs. 10.1) and marbling (1.7 vs. 3.0), than ANG crosses. At cutting up, CHA crosses had higher proportions of retail cuts (38.9 vs. 35.8%) and bones (19.7 vs. 19.0%), but a lower proportion of trim fat (11.6 vs. 16.1%), than ANG crosses.
Table 6. Carcass characteristics of dairy × beef heifers (n = 18) with two sire breeds (Angus (ANG) or Charolais (CHA)) in production systems with moderately high indoor feed intensity and 20 months slaughter age (HIGH) or low indoor feed intensity and 27 months slaughter age (LOW). Least square mean, pooled standard error of the mean (s.e.), and significance of the main effects of sire breed, production system, and their interaction.
SH crosses had a lower proportion of bones in the hindquarter (19.0 vs. 19.6%, p = 0.0202) than SR crosses. In addition, SH crosses tended to have higher liveweight at slaughter (p = 0.0872), carcass weight (p = 0.0966), conformation score (p = 0.0513), and proportions of retail cuts (p = 0.0993) and grade 2 meat assortment (p = 0.0632), but lower proportion of grade 3 meat assortment (p = 0.0518), than SR crosses.
Heifers in the LOW system, which had a long rearing period and low indoor feed intensity, had heavier carcasses (362 vs. 335 kg) with lower dressing proportion (50.2 vs. 51.0%), and less fatness (9.2 vs. 10.2) and marbling (2.1 vs. 2.6) scores, than the heifers in the HIGH system, which had a shorter rearing and higher indoor feed intensity (). Consequently, the hindquarters of heifers in the LOW system contained less trim fat (12.4 vs. 15.3%) and a tendency for less grade 3 meat assortment, but more retail cuts (38.3 vs. 36.4%), grade 2 meat assortment (20.4 vs. 18.9%), and bones (19.7 vs. 18.9%), than the hindquarters of heifers in the HIGH system.
Three two-way interactions between sire breed and production system were identified: (i) CHA crosses had lower fatness score than ANG crosses in the LOW system, whereas no sire breed effect was seen in the HIGH system (); (ii) the hindquarters of CHA crosses contained higher proportions of retail cuts and grade 2 meat assortment in the LOW system compared with the HIGH system, whereas there was no effect of production system on the proportion of retail cuts or grade 2 meat assortment in ANG crosses ().
Discussion
This study examined the potential for combining production of high-quality carcasses from dairy x beef heifers with conservation grazing of biodiverse semi-natural grasslands. There are differences in the proportions of muscle and fat deposited in cattle of different breeds, with higher conformation score for Charolais animals (Chapple et al., Citation2000; Huuskonen et al., Citation2013; Basiel & Felix, Citation2022), as also found for CHA crosses in the present study. This was reflected in a greater proportion of valuable retail cuts and a smaller proportion of bones compared with ANG crosses. Thus the greater muscle proportions in CHA crosses compared with ANG crosses gave a more valuable carcass, expressed as kg saleable meat. On the other hand, crossbreds with early-maturing breeds, such as Angus, in general deposit a higher proportion of fat than crossbreds with late-maturing beef breeds (Chapple et al., Citation2000; Huuskonen et al., Citation2013; Eriksson et al., Citation2020; Basiel & Felix, Citation2022). In the present study, this was reflected in higher scores for fatness, trim fat, and visually estimated marbling (intramuscular fat) in M. longissimus dorsi in ANG crosses than in CHA crosses, in accordance with Gault et al. (Citation2015). More marbling could result in higher and less variable eating quality in beef from ANG crosses, but also more tender and juicy meat (Corbin et al., Citation2015). Breed had a more pronounced main effect on the amount of trim fat than on the fatness score (), although fatness scoring is based on estimated amount of trim fat (SJVFS Citation127, Citation1998). This is because the relationship between fatness score and amount of trim fat is not linear, with each unit increase in fatness score up to a value of 7 representing one percentage unit more trim fat in the carcass, whereas each unit increase in fatness score from 8 upwards represents two percentage units more trim fat (SJVFS Citation127, Citation1998).
The LOW system generally resulted in leaner carcasses with lower fatness and marbling (tendency only) scores and lower proportions of trim fat and fat meat assortment (tendency only) than the HIGH system (). The CHA crosses had lower fatness score than ANG crosses in the LOW system, whereas no breed effect was found in the HIGH system. Carcasses from CHA crosses also contained higher proportions of lean meat in the LOW compared with the HIGH system, whereas no such effect of production system was found in ANG crosses (). Hence, CHA crosses in the LOW system differed from the other three animal groups (CHA-HIGH, ANG-LOW, ANG-HIGH) in having low fatness and marbling (tendency only) scores, low proportions of trim fat and fat meat assortment (tendency only), but high proportions of retail cuts, lean meat assortment, and bone. These results indicate that CHA crosses in the LOW system were slaughtered at an earlier stage of maturity than heifers in the other three groups.
The results obtained reflected the difficulty in obtaining a market-oriented carcass regarding weight and fatness score in dairy × beef heifer production, especially at high indoor feed intensity. Carcasses from all heifers reached at least conformation score O−, qualifying them for the premium price in the current Swedish market (Skövde slakteri, Citation2023) and all except one heifer from the HIGH system reached the desired carcass weight range (275–424.9 kg). However, 25% (18 heads) of the heifer carcasses were too fat to qualify for the premium price according to the present payment criteria (fatness score 2 to 4−; Skövde slakteri, Citation2023). Fourteen of the 18 excessively fat carcasses were from the HIGH system and represented the four breed combinations equally. Carcasses graded as excessively fat had an average marbling score of 2.8, which can be compared with the average marbling score in heifers qualifying for the premium price of 2.2. This demonstrates the challenge in producing well-marbled retail cuts without much of the energy in animal feed ending up in excessive fat deposits that are penalized by lower carcass price. However, it should be noted that fatness score up to 4+ qualifies for the premium price in periods of shortage of bovine carcasses, resulting in all heifers in the present study qualifying for the higher price. The extra payment for certified pasture beef, corresponding to 10% of the official price, might also outweigh possible deductions due to fatness (HKScan, Citation2023).
Increased indoor feed intensity (HIGH system) resulted in higher dietary intake expressed as kg DM day−1 during indoor period 1 (18%) and indoor period 2 (28%) than in the LOW system. This difference was partly due to heifers in the HIGH system being born on average 1.6 months earlier in the year, so although the initial age was the same, their first indoor period was longer and the animals were therefore on average older and larger than heifers in the LOW system during this period. Hence, when expressed as a proportion of liveweight, no difference in feed intake was found between the systems. The different initial age and liveweight, and the supposed accomplished increased proportion of liveweight gain as energy-demanding fat deposits and not muscles, also explain why heifers in the HIGH system showed lower feed efficiency than heifers in the LOW system during this period (). In contrast, dietary intake, liveweight gain, and feed efficiency were higher for heifers in the HIGH system than the LOW system during indoor period 2 (). This was due to the different feed rations in the two systems, as the average liveweights at this time were quite similar (525 vs. 505 kg). Breed effects on dietary intake were related to the higher liveweight of CHA crosses compared with ANG crosses. At the start (2.7–3.9 months old), average liveweight of CHA crosses was 18 kg higher than for ANG crosses, and at slaughter this breed difference had increased to 39 kg across the two feed intensities, which is similar to findings in previous studies (Huuskonen et al., Citation2013; Eriksson et al., Citation2020). The heavier CHA crosses had higher dietary intake, expressed as kg day−1 than ANG crosses during indoor period 3, whereas the lighter ANG crosses had, or tended to have, higher intake in relation to liveweight during all three indoor periods. An interaction between sire breed and production system on feed efficiency was anticipated, with ANG crosses being more efficient in the LOW system and CHA crosses being more efficient in the HIGH system (Webster, Citation1989). No such interaction was found, possibly due to effects of the grazing periods between the indoor periods or to too few individuals per group to show an effect. There was however a tendency for a similar interaction during indoor period 2, when ANG crosses tended to gain more than CHA crosses in the LOW system, whereas the CHA crosses tended to gain more than ANG crosses in the HIGH system.
Heifers in the HIGH system did not gain any weight during their single grazing period, whereas 24–27% of the lifetime weight gain was obtained from pasture for heifers in the LOW system. Heifers in the LOW system had 100 kg lower liveweight at turn-out to pasture in grazing period 1 compared with heifers in the HIGH system, but showed compensatory growth and had similar weight as heifers in the HIGH system at housing (). In previous studies, young cattle with low indoor feed intensity have been found to regain about half of their lost liveweight gain during their recovery period on pasture (Wright et al., Citation1989; Hessle et al., Citation2007; Hessle et al., Citation2011). Heifers in the LOW system in the present study regained all their lost weight on pasture compared with heifers in the HIGH system, but as the heifers in the two systems did not have their first period of grazing during the same year, direct comparison is difficult.
Swedish Holstein is a larger-sized breed than Swedish Red, resulting in SH crosses in the present study having higher liveweight gain and a tendency for higher carcass weight than SR crosses (), which is in accordance with findings in a study on all Swedish cattle of dairy × beef breed slaughtered 2000–2015 (Eriksson et al., Citation2020). Swedish Red is regarded as a dual-purpose breed, traditionally bred for both milk and beef production. Accordingly, Eriksson et al. (Citation2020) found that Swedish Red heifers in their dataset had on average higher conformation score than Holstein heifers (score 4.5 vs. 3.8), which also was the case for dairy × beef crosses of the two breeds. Although there is an ongoing negative trend for conformation score in both Swedish Holstein and Swedish Red, one year ago the average score for female dairy × beef crosses with Swedish Red (6.6) was still higher than that for female dairy × beef crosses with Holsteins (6.2) (Nordic Cattle Genetic Evaluation, Citation2023). The opposing results found in the present study, where SH crosses tended to have higher conformation score and more lean meat (retail cuts and meat assortment 2) than SR crosses (), cannot be explained. No difference in marbling score was found between SR and SH crosses, which is in agreement with results in a previous study on maternal grandsire effects (Casas & Cundiff, Citation2006).
Although the heifer groups did not all gain weight on pasture, all contributed to management of semi-natural grasslands. Managed grassland area per heifer was 0.36, 0.33, 1.32, and 1.14 ha for ANG-HIGH, CHA-HIGH, ANG-LOW, and CHA-LOW, respectively (). Saleable meat was measured directly in the hind quarters (sum of retail cuts and meat assortments; ) and estimated for the fore quarters based on Hansson (Citation1989). Combining the data on grassland area and amount of saleable meat, production of one kg meat resulted in management of 16 m2 semi-natural grassland in the HIGH system and 52 m2 in the LOW system, and ANG crosses gave a slightly larger grazed area than CHA crosses (18 vs. 15 m2 per kg in the HIGH system, 58 vs. 46 m2 per kg in the LOW system).
Liveweight gain was lower during the grazing periods than during both indoor periods, especially in year 2021, which was the first grazing period for heifers in the HIGH system and the second grazing period for heifers in the LOW system (). The energy concentration in herbage on semi-natural grasslands is often low, particularly for Deschampsia cespitosa (Andersson, Citation1999), the grass species dominating pasture in this study. However, the concentration of metabolizable energy was roughly the same in the pasture herbage and in the grass-clover silage, at least during early summer, and the concentration of NDF was also similar (, ). Quantity, and not quality, was probably the limiting factor for the low weight gain in 2021, as average sward height on the pasture was 4.3 cm, compared with 6.2 cm in 2020, when the weight gain was higher. Low sward height (<6 cm) on semi-natural grasslands has previously been shown to restrict weight gain in growing cattle (Spörndly et al., Citation2000). Similar results have been reported in earlier studies of growing cattle grazing the same pastures as in the present study (Hessle et al., Citation2007; Hessle et al., Citation2011; Hessle et al., Citation2019). Although weight gain on pasture was most likely restricted by insufficient herbage mass, decreasing the stocking rate would not improve the production system as a whole since short sward height at the end of the grazing period is required to obtain the agri-environmental payment for semi-natural grassland (Swedish Board of Agriculture, Citation2023). Although sward height was unnecessarily low at the end of the grazing periods (4 cm), the optimal sward height on semi-natural grasslands is generally a trade-off between beef production and nature conservation aspects, which has to be considered in multifunctional grazing aiming at both human food and nature conservation.
This study showed that high carcass quality and preservation of semi-natural grasslands can be achieved successfully in a beef production system. The results also indicated that low indoor feed intensity is most appropriate in production systems using cattle for nature conservation grazing on semi-natural grasslands, because it (i) results in higher weight gain on pasture, (ii) reduces the risk of excessively fat carcasses, and (iii) results in significantly larger grazed grassland area per animal. Special care must be taken when using crossbreds of late-maturing breeds in low-intensity systems, as they might need a longer rearing period than early-maturing breeds to express their genetic growing potential and produce sufficient fat deposits to give high eating quality.
Conclusions
In beef production from dairy × beef heifers on semi-natural grassland, lower indoor feed intensity combined with higher slaughter age results in a larger grazed area and leaner carcasses than higher indoor feed intensity combined with lower slaughter age, where the carcasses may be too fat. However, at low feed intensity crossbred heifers of late-maturing beef breeds, such as Charolais, may need to be kept to higher slaughter age than in this study (27 months) in order to fully utilize their growing potential and deposit fat. Overall, beef production with dairy × beef heifers based on forage and semi-natural grasslands is a multifunctional system where nature conservation effects of grazing and market-oriented carcasses simultaneously can be achieved.
Acknowledgements
We thank Jonas Dahl and David Johansson for their practical work during the rearing, Karin Wallin for her work in the slaughter and cutting up procedures, the abattoir staff at Skövde slakteri for their help, and Hanna Rehnström for data compilation. We are also grateful to the funding bodies and the Swedish University of Agricultural Sciences for its base support.
Disclosure statement
No potential conflict of interest was reported by the author(s).
Additional information
Funding
References
- Åman, P. & Hesselman, K. (1984). Analysis of starch and other main constituents of cereal grains. Swedish Journal of Agricultural Research, 14, 135–139.
- Andersson, A. (1999). Nutritional value in grasses from semi-natural pastures. Masters thesis, Department of Animal Nutrition, Swedish University of Agricultural Sciences.
- Andersson, R. & Hedlund, B. (1983). HPLC analysis of organic acids in lactic acid fermented vegetables. Zeitschrift Fur Lebensmittel-Untersuchung und -Forschung, 176(6), 440–443. doi:10.1007/BF01042558
- Axelsson, J. (1941). Der Gehalt des Futters an umsetzbarer Energie. Züchtungskunde, 16, 337–347.
- Basiel, B. L. & Felix, T. L. (2022). Board invited review: Crossbreeding beef × dairy cattle for the modern beef production system. Translational Animal Science, 6, 1–12.
- Brondizio, E. S., Settele, J., Díaz, S. & Ngo, H. T. (2019). Global assessment report on biodiversity and ecosystem services of the Intergovernmental Science-policy Platform on Biodiversity and Ecosystem Services. Intergovernmental Science-Policy Platform on Biodiversity and Ecosystem Services. Accessed 6 September 2023, available at: doi:10.5281/zenodo.3831673.
- Casas, E. & Cundiff, L. V. (2006). Postweaning growth and carcass traits in crossbred cattle from Hereford, Angus, Norwegian Red, Swedish Red and White, Friesian, and Wagyu maternal grandsires. Journal of Animal Science, 84(2), 305–310. doi:10.2527/2006.842305x
- Chai, W. & Udén, P. (1998). An alternative oven method combined with different detergent strengths in the analysis of neutral detergent fibre. Animal Feed Science and Technology, 74(4), 281–288. doi:10.1016/S0377-8401(98)00187-4
- Chapple, D. G., Grundy, H. F., Keatinge, R. & Mann, G. (2000). Comparative performance of Simmental * Holstein/Friesian and Belgian Blue * Holstein/Friesian cows in an autumn calving suckler herd. In D. Pullar (ed.), Beef from Grass and Forage, ADAS Rosemaund (Wynne: Preston Publications), pp. 175–178.
- Commission of the European Union (2008). Commission regulation (EC) no 1249/2008. Accessed 6 September 2023, available at: https://eur-lex.europa.eu/LexUriServ/LexUriServ.do?uri=OJ:L:2008:337:0003:0030:EN:PDF
- Coop (2023). Coop naturbeteskött. Accessed 6 September 2023, available at: https://www.coop.se/hallbarhet/hallbarhetsarbete/kott/naturbeteskott/.
- Corbin, C. H., O’Quinn, T. G., Garmyn, A. J., Legako, J. F., Hunt, M. R., Dinh, T. T. N., Rathmann, R. J., Brooks, J. C. & Miller, M. F. (2015). Sensory evaluation of tender beef strip loin steaks of varying marbling levels and quality treatments. Meat Science, 100, 24–31. doi:10.1016/j.meatsci.2014.09.009
- Council of the European Union (2007). Council regulation (EC) no 1234/2007. Accessed 6 September 2023, available at: https://faolex.fao.org/docs/pdf/eur75432.pdf.
- Dumas, J. B. A. (1831). Proceeds de l’analyse organique. Annales de Chimie et de Physique, 247, 198–213.
- Eriksson, O. (2022). Coproduction of food, cultural heritage and biodiversity by livestock grazing in Swedish semi-natural grasslands. Frontiers in Sustainable Food Systems, 6, 801327. doi:10.3389/fsufs.2022.801327
- Eriksson, S., Ask-Gullstrand, P., Fikse, W. F., Jonsson, E., Eriksson, J.-Å., Stålhammar, H., Wallenbeck, A. & Hessle, A. (2020). Different beef breed sires used for crossbreeding with Swedish dairy cows – Effects on calving performance and carcass traits. Livestock Science 232, 8 p.p. doi:10.1016/j.livsci.2019.103902.
- EU Council Directive 1998/64/EC (1998). Official Journal of European Communities. Commission of the European Communities. L257/14. Accessed 6 September 2023, available at: https://eur-lex.europa.eu/LexUriServ/LexUriServ.do?uri=OJ:L:1998:257:0014:0028:En:PDF.
- European Climate, Infrastructure, and Environment Executive Agency (2023). New Nature Restoration Law boosts biodiversity and climate action across Europe. Accessed 6 September 2023, available at: https://cinea.ec.europa.eu/news-events/news/new-nature-restoration-law-boosts-biodiversity-and-climate-action-across-europe-2023-07-12_en.
- European Commission (2022). Proposal for a regulation of the European Parliament and of the Council on nature restoration. 2022/0195 (cod). Accessed 6 September 2023, available at: https://environment.ec.europa.eu/publications/nature-restoration-law_en
- European Environment Agency (2020). State of the nature in EU. Report 10. Accessed 6 September 2023, available at: https://www.eea.europa.eu/publications/state-of-nature-in-the-eu-2020.
- European Forum on Nature Conservation and Pastoralism. (2023). High nature value farming. Accessed 6 September 2023, available at: http://www.high-nature-value-farming.eu/.
- Frame, J. (1993). Herbage mass. In A. Davis, R. D. Baker, S. A. Grant & A. S. Laidlaw (Eds.), Sward Measurement Handbook (Dunston: British Grassland Society), pp. 39–67).
- Gault, N. F. S., Farrell, D. T., Devlin, D., Majury, L., Lewis, H., Gordon, A. W., Lively, F. & Farmer, L. J. (2015). Beef from dairy breeds versus dairy × beef breed crosses: A comparison of production factors and meat eating quality. Proceedings of the 61st International Congress of Meat Science and Technology, Clermont-Ferrand, France.
- Hansson, I. (1989). Composition and characteristics of bovine carcasses. Report 89. Department of Animal Breeding and Genetics, Swedish University of Agricultural Sciences.
- Hessle, A., Dahlström, F. & Wallin, K. (2011). Alternative production systems for male Charolais cross-bred cattle using semi-natural grasslands. Acta Agriculturae Scandinavica, Section A – Animal Science, 61(1), 21–33. doi:10.1080/09064702.2010.543427
- Hessle, A., Nadeau, E. & Johnsson, S. (2007). Beef heifer production as affected by indoor feed intensity and slaughter age when grazing semi-natural grasslands in summer. Livestock Science, 111(1–2), 124–135. doi:10.1016/j.livsci.2006.12.014
- Hessle, A., Therkildsen, M. & Arvidsson-Segerkvist, K. (2019). Beef production systems with steers of dairy and dairy × beef breeds based on forage and semi-natural pastures. Animals: An Open Access Journal from MDPI, 9(12), 1064. doi:10.3390/ani9121064
- HKScan. (2023). Payoff. Accessed 6 September 2023, available at: https://www.hkscanagri.se/notering/.
- Holmström, K., Kumm, K.-I., Andersson, H., Nadeau, E., Segerkvist, K. A. & Hessle, A. (2021). Economic incentives for preserving biodiverse semi-natural pastures with calves from dairy cows. Journal for Nature Conservation, 62, 126010. doi:10.1016/j.jnc.2021.126010
- Huuskonen, A., Pesonen, M., Kämäräinen, H. & Kauppinen, R. (2013). A comparison of the growth and carcass traits between dairy and dairy × beef breed crossbred heifers reared for beef production. Journal of Animal and Feed Sciences, 22(3), 188–196. doi:10.22358/jafs/65987/2013
- Jukna, V., Jukna, C. & Pečiulaitienė, N. (2009). The beef production efficiency of milk cattle used crossed with different intensive beef cattle breeds. Biotechnology in Animal Husbandry, 25(5–6), 293–300. doi:10.2298/BAH0906293J
- Kenward, M. G. & Roger, J. H. (2009). An improved approximation to the precision of fixed effects from restricted maximum likelihood. Computational Statistics and Data Analysis, 53(7), 2583–2595. doi:10.1016/j.csda.2008.12.013
- Lindgren, E. (1979). The nutritional value of roughages determined in vivo and by laboratory methods. Report 45. Department of Animal Nutrition, Swedish University of Agricultural Sciences.
- McCabe, E. D. (2021). Beef cattle data analytics: Trends in feedlot cattle health, performance, and carcass traits, and effects of beef on dairy programs. Dissertation for the degree of Doctor of Philosophy. Kansas State University.
- Mordenti, A. L., Brogna, N., Canestrari, G., Bonfante, E., Eusebi, S., Mammi, L. M. E., Giaretta, E. & Formigoni, A. (2019). Effects of breed and different lipid dietary supplements on beef quality. Animal Science Journal, 90(5), 619–627. doi:10.1111/asj.13177
- Nordic Cattle Genetic Evaluation. (2023). Accessed 5 October 2023, available at: https://nordicebv.info/.
- Plateryd, L. (2004). Consumers and pasture beef. Motives for purchases of Kaprifolkött and views of rural landscapes. Occasional Papers. Department of Human Geography, University of Gothenburg, 2004:4.
- SAS Institute. (2018). Inc. SAS version 9.4 (SAS Institute, Inc.).
- SJVFS 127. (1998). Directions of classifications of carcasses from the Swedish Board of Agriculture (Swedish Board of Agriculture). Accessed 6 September 2023, available at: https://lagen.nu/sjvfs/1998:127.
- Skövde slakteri. (2023). Payoff. Accessed 6 September 2023, available at: https://www.skovdeslakteri.com/noteringssidan.php.
- Spörndly, R. (ed.). (2003). Feed tables for ruminants. Report 257. Department of Animal Nutrition and Management, Swedish University of Agricultural Sciences.
- Spörndly, E., Olsson, I. & Burstedt, E. (2000). Grazing by steers at different sward surface heights on extensive pastures: A study of weight gain and fat deposition. Acta Agriculturae Scandinavica, Section A – Animal Science, 50(3), 184–192. doi:10.1080/090647000750014313
- Svenskt Sigill. (2023). Svenskt Sigill pasture beef for biodiversity. Accessed 5 September 2023, available at: https://www.svensktsigill.se/hallbarhet/vara-marken/naturbeteskott/.
- Swedish Board of Agriculture. (2023). Agri-environmental payments for management of pastureland and meadows 2023. Accessed 6 September 2023, available at: https://jordbruksverket.se/stod/jordbruk-tradgard-och-rennaring/jordbruksmark/betesmarker-och-slatterangar/skotsel-av-betesmarker-och-slatterangar.
- Toräng, P. & Jacobson, A. (2019). An assessment of favorable reference areas for grassland habitat types in the 2019 reporting under Article 17 of the Habitats Directive. PM SLU.dha.2019.5.5-16 (Swedish Species Information Centre).
- United States Department of Agriculture. (2018). Official USDA marbling photographs. Accessed 7 December 2018, available at: https://swfulfillment.1touchpoint.com/sv5fmsnet/OeCart/OEFrame.asp?Action=NEWORDER&cmenunodseq=&FromFav=&PmSess1=58859&pos=006142&v=2NCBA/Secure/StoreItem.aspx?ID=16313&ITEMS=CATALOG&CAT=165&TP=180.
- Växa Sverige. (2023). Cattle statistics 2023. Accessed 6 September 2023, available at: https://vxa.qbank.se/mb/?h=c7a1d64e698d8df91094699ba3ffd110&p=dccda36951e6721097a93eae5c593859&display=feature&s=name&d=desc.
- Webster, A. J. F. (1989). Bioenergetics, bioengineering and growth. Animal Science, 48(2), 249–269. doi:10.1017/S0003356100040265
- Wright, I. A., Russel, A. J. F. & Hunter, E. A. (1989). Compensatory growth in cattle grazing different vegetation types. Animal Production, 48(1), 43–50. doi:10.1017/S0003356100003779