ABSTRACT
Malaria control has been one of the defining goals in global health. Recently, strategies that aim to control the insect-borne disease by altering mosquito biology have gained interest. One such strategy currently in development is to engineer mosquitoes equipped with so-called gene drives: elements that increase the likelihood of a specific set of genes to be passed on to the next generation. The strategy encapsulates hopes of technological solutions in global health, as well as expectations for the applications of synthetic biology. Hence, gene drive research operates with the potential of genetically modified mosquitoes: to survive in the wild, to reproduce, and to perform the work expected of them in some future timeline. I examine gene drive research with the concept of potentiality and how establishing it has guided gene drive research. Knowledge and uncertainties about mosquitoes and mosquito ecologies emerged through working with the mosquito as a research animal in the laboratory, as population data, and as a regulatable biotechnology. Mathematical modelling made it possible to establish the potential to move from the lab to the wild. In modelling, the mosquito as a research object could be made to work as part of future projections that assembled different kinds of data. As gene drive research progresses, I suggest that the researchers are also modelling how biotechnology handles uncertainty that is related to the ways producing genetic modification in the lab and producing knowledge about their possible environmental effects and efficacy translates into landscapes and mosquito ecologies in the wild.
Introduction
Malaria prevention has been a central goal within the field of global health. For decades, the field has tended to look towards biotechnological solutions (Cueto, Citation2013). Malaria control programmes have primarily relied on measures such as insecticides and bed nets which target the mosquito as the disease vector (WHO, Citation2022). The association between mosquitoes and malaria has underlain much of the malaria research, leaving a lasting impact on how malaria is understood as an epidemic (Kelly and Beisel, Citation2011). Recently, advances have been made towards developing genetically modified mosquitoes that would replace malaria-transmitting mosquito populations. Discussions about gene drives have highlighted tensions between advancing human health and grappling with lethal disease while considering uncertainties about their environmental effects (cf. Callaway, Citation2016).
This article examines the development of one of the more recent mosquito-focused malaria control strategies: the gene drive mosquito. Genetically modified mosquitoes equipped with ‘gene drives’ are part of the recent interest in researching ways of altering mosquito biology to reduce rates of insect-borne diseases. STS researchers have noted how these transformations in mosquito biology also reverberate as shifts in human–insect relations while also contributing to the logic underlying public health practices (Beisel and Boëte, Citation2013). As Timothy Mitchell (Citation2002) has noted, malaria control in the twentieth century has emerged in an interplay between local institutions and practices, notions of progress and control over nature, and the lives of the mosquitoes themselves.
Gene drives are genetic elements that increase the probability of a specific set of genes being passed on to the next generation. The emergence of CRISPR-Cas9 genome editing technology has enabled engineering such elements into the DNA of a wide variety of organisms, ranging from insects to mammals. Gene drives have been envisioned for various purposes, including the control of so-called ‘invasive species’ and agricultural pests (cf. Esvelt et al., Citation2014). In the case of malaria control, gene drive mosquitoes are being developed in several international research projects that combine microbiological research in the Global North with entomological research from malaria endemic countries which are being planned as target sites. The Bill and Melinda Gates Foundation in particular has taken a central role in funding and advancing biotechnological solutions for malaria control, including supporting gene drive research (WHO, Citation2022).
As Alex Nading (Citation2015a) notes, while technologies in global health are shaped by global health funding structures, they are not solely defined by them. Nading (Citation2015a) argues for approaching global health technologies as ‘cosmopolitical events’ which highlight social and technical differences between understandings of, such as those between the logics of pharmaceutical capital, humanitarianism and biosecurity (cf. Stengers, Citation2005). Gene drives are a product of biotechnologically driven disease control. At the same time, they may also mark shifts in how uncertainties and ecological dynamics are dealt with in developing biotechnology.
Indeed, global health biotechnologies have often represented larger narratives about technological possibilities and risks. Nading (Citation2015b) has also noted that these efforts are underpinned by what he calls ‘scalar narratives’: narratives where both the disease and the proposed solution to it come to represent something larger than the issue at hand. Constructing transferable and universalizable technologies nonetheless requires working with localized concerns. The way these concerns are dealt with in developing biotechnology bear their mark on understandings of public health and technological interventions (Bennett, Citation2019). Likewise, altering insects in order to deal with infectious diseases results in a shift in human–insect relations both within and outside the laboratory (see also Lezaun and Porter, Citation2015; Reis-Castro, Citation2021).
This article asks: how are gene drive mosquitoes crafted research objects? What kinds of transformations does gene drive research go through as the mosquitoes are prepared to be moved from the lab into the wild? I analyse work on gene drives as it engages with mosquitoes on different scales: as a laboratory animal, as population data, and as a potential, regulatable global health biotechnology. The concerns of gene drive researchers worked with emerged in interplay with mosquitoes as research objects and the aims of the technology. First, the goal has been to produce a mosquito carrying a gene drive that would have the desired effect, and for this gene drive to be transmitted through populations. Second, the question is whether this will work in natural environments; how the seasonal, ecological and geographical features of the areas of possible release would affect the dispersal of the mosquitoes. Third, since the ultimate goal is to arrive at a mosquito strain that could be released into the world, the work must also aim for acceptability outside laboratories. The analysis presented here is based on following the gene drive researchers as they work around these aims. In doing this, I suggest, gene drive researcher offers a perspective into how biotechnological development operates with uncertainties and ecological knowledge production.
Analytical perspectives
Potentiality in biotechnology
In addition to being promoted and justified within the framework of global health solutionism, gene drive mosquitoes also embody hopes surrounding gene editing and synthetic biology (see Esvelt et al., Citation2014). Future orientations, whether conceived as promises and expectations or as risks and uncertainties, play a fundamental role in shaping and legitimating biomedical and biotechnological research (Borup et al., Citation2006; Samimian-Darash and Rabinow, Citation2015). Karen-Sue Taussig, Klaus Hoeyer and Stefan Helmreich (Citation2013) have explored how potentiality serves as both an analytical concept and an object of study in biomedicine. They note how articulations of potentiality both produce and depend on the malleability of life. They trace how the concept takes on three distinct meanings, each implying its own moral and political framework: that of potentiality as a ‘hidden force determined to manifest itself’ in the biological material; the potentiality of the materials’ plasticity and capacity to transform; and that of their openness to human direction and management. Uses of potentiality also often tend to shift and blend into each other, Taussig, Hoeyer and Helmreich (Citation2013, p. S4) note. Helmreich (Citation2007) notes how this potentiality in biotechnology is often seen as a property of the organisms themselves, and at the same time liable to scale up, to do work in the world.
In this way, potentiality implies both negative and positive future expectations, as well as those that are more ambiguous or unformed, while still carrying moral valuations. In the case of gene drive research, as I will discuss, positive and negative future expectations overlap, as the potential of the technology to spread is both the cause of worries raised about it, and the source of its justification and promise.
Ana Delgado (Citation2016) has discussed how an increasing tendency to ground and justify the objects of scientific research as novel innovations entails a shift in the epistemic focus of research in synthetic biology towards an epistemology that is concerned with not only, or mainly with, space and representation, but also with time (Delgado, Citation2016). In synthetic biology, the products of research were understood as ‘living designs’ whose ‘referential anchoring [moved] from ‘back’ in reality to ‘ahead’ in the future or from the natural organism to the possible living thing’ (Delgado, Citation2016, p. 1). As such living designs, their justification is built via their promise to work ‘from afar,’ in some future time (Delgado, Citation2016).
Such a shift means that the way objects of scientific research are made to emerge as ‘precarious configurations’ involves not only spatial, but also temporal, assembling (Delgado, Citation2016, p. 3). In the analysis that follows, I explore how assembling gene drive mosquitoes also entailed configuring and attempting to stabilize shifting articulations of potentiality. Casper Bruun Jensen (Citation2020) has discussed how the way mathematical modelling makes risks known in a specific way, by mediating between ways of knowing the object of modelling. Modelling was likewise an essential part of gene drive research and of configuring articulations of mosquito potentiality.
I explore how mosquito bodies are crafted in different settings to become a viable malaria control tool. Studies in STS have shown how potentiality in biomedical research is often crafted on and produced from animal bodies (Friese, Citation2013; Wanderer, Citation2018). Carrie Friese (Citation2013) notes how ‘potentializing practices’ connect the animal bodies biomedical researchers work with to the experimental systems of which the animals are part, thereby posing questions of standardization in biomedical research. Likewise, when moving outside the laboratory, achieving potential from animal bodies happens in relation to the environments of the expected future use, whether they are human bodies or vulnerable ecosystems, as Emily Wanderer (Citation2018) discusses. As laboratory animals, insects have become usable research tools within research infrastructures that render them both disposable and expendable due to their utility as disease models (Mehrabi, Citation2016).
Scalable, living technologies
Experimenting on mosquitoes as public health technologies is based on laboratory craftwork where mosquitoes are detached from the complexities of their living environments (Dupé, Citation2015). The laboratory insectarium, as Dupé (Citation2015, pp. 24–32) describes, is a laboratory space where aspects of mosquitoes’ lives are reduced to aspects deemed relevant to the research problem. Mosquitoes are made knowable by creating spaces to study them (Kelly and Lezaun, Citation2017; see also Kohler, Citation1994; Nelson, Citation2018).
The potentiality of gene drive mosquitoes is expected to transition from the microscopic, genomic scale to encompass entire mosquito populations and species. At its core, this potentiality hinges on mosquitoes’ capacity to reproduce, thus enabling geographical and temporal spread across landscapes and generations of mosquitoes.
The shifts of potentiality as realized in a laboratory setting and a potential that is ascribed to an organism can be conceptualized as a question of scalability. What I mean by scalability in this article refers to a characteristic of projects that, in the words of Anna Tsing (Citation2012; Citation2015), are designed to have the ability to expand without changing the framing of the project itself. In other words, scalable projects are founded by removing objects from their formative social relations (Tsing, Citation2012, p. 508). Achieving this kind of scalability, however, requires work, since ‘scalability is always incomplete’ (Tsing, Citation2012, p. 515).
Priscilla Bennett (Citation2019) has noted how the development of genetically modified mosquitoes for release in Key West was met with local regulatory challenges and social opposition. Developing a scalable, universalizable technology was incumbent on working through such encounters in a way that, as Bennett (Citation2019, p. 212) states, ‘has shaped […] the regulatory pathway and reception for genetically engineered pest technologies more broadly.’ As Bennett (Citation2019) discusses, these encounters may shape how the transferable and the scalable is realized in such biotechnological projects.
An Anopheles research community
I approach the case in terms of a research community centered around working with a particular organism: the gene drive mosquito. Malaria, as a disease affecting humans, is spread by mosquitoes of the genus Anopheles. Arguably the most researched of Anopheles mosquitoes is Anopheles gambiae, which transmits the deadliest form of malaria, caused by the Plasmodium parasites (WHO, Citation2022). Anopheles gambiae is also the focus of gene drive research. STS research on laboratory animals has noted how experimental organisms can act as a connecting node between modes of knowledge production. Robert E. Kohler’s (Citation1994) account of geneticists working on Drosophila fruit flies showed how the creation and standardization of an experimental animal happened alongside, and was made possible by, the creation of a community of drosophilists. In a similar manner, Nicole Nelson (Citation2018) describes research carried out on mice on the genetics of psychiatric disorders, thereby exploring how multifaceted, complex and often interdisciplinary work and work across research projects was enabled by the organism at the center.
Focusing on the research community also reflects the gene drive world. Gene drive mosquitoes are being developed in several separate yet interconnected research projects. These all pursued slightly differing strategies while maintaining frequent contact with each other. Contact between research projects was often facilitated by a major funding institution behind much of gene drive research, Bill and Melinda Gates Foundation. Investing in gene drive research is one of the ways the foundation has chosen to push towards this goal (WHO, Citation2022).
The defining role of institutions such as the Gates Foundation in global health has been contested (Birn, Citation2005). In the field of gene drive research, even if projects were not directly funded by the Gates Foundation, they were able to benefit from networks of collaboration maintained by the foundation. These extended from European and North American universities to collaboration in several African countries where releases were being planned. Nevertheless, the projects were led and funded by European and North American researchers and funding institutions.
My analysis is based on interviews with 16 people who were diversely positioned in gene drive mosquito research: microbiologists, mathematicians, regulatory experts, and those working in communication and stakeholder engagement, as well as observations in two insectaries. The interviewees held varying positions, from PhD students to project PIs. Interlocutors were chosen by first contacting project Pis, and then by asking interlocutors for recommendations on further interviewees. I contacted additional people based on perspectives that were lacking in the material. The interviews were conducted between Autumn 2019 and Autumn 2021; nine were with the interlocutors at their place of work, and nine were conducted virtually. Alongside interviews that were carried out in person, I visited the insectaries and laboratories at which the interlocutors worked. The analysis is supplemented with 270 articles and other text material produced in the projects on which the interlocutors engaged.
I interpreted the data – interviews, text materials, and field notes – as accounts of the work being carried out. I paid attention to how the gene drive mosquito was being made knowable and viable (Mol, Citation2002, 53). The concerns and questions guiding gene drive mosquito development encompass those stemming from laboratory practices in genetics, ecology, and the needs of fitting data into mathematical models, as well as from the anticipated future regulatory networks, and building social acceptance. In my analysis, I pay attention to how divergent concerns emerged and were reconciled with, and to how aspects of potentiality are worked on around them. The goal of this attention is to bring visible the ways in which gene drive mosquitoes are made into being and into usable, a potential technology.
The promise of scalable mosquito work
In the Anopheles research community, the potentiality of the technology was first identified as inherent in the mosquitoes themselves. Mosquito potential structured the research both in terms of creating the community of Anopheles research, as well as in terms of how the novelty of the technology was articulated in relation to other, existing malaria control methods.
Gene drive mosquitoes research projects formed a transnational network of research on Anopheles gene drives. The network encompassed researchers working on developing the gene drive systems and crafting them on mosquito bodies in European and North American universities, and workers in several African countries where eventual mosquito releases were being planned in a still uncertain future. The work of crafting mosquito genetics was done in laboratories far from the malaria-endemic places where the eventual technology was planned to be used. This geographical separation also served as a containment strategy in case mosquitoes were to breach the confines of the insectary (Quinlan et al., Citation2018). The travels of both humans and mosquitoes in and out of the laboratory insectaries were managed within the scope of the gene drive project.
The promise and potential of gene drive mosquitoes relied on two forms of transfer: the transfer of the technology itself from laboratories in the Global North to ecosystems in malaria-endemic countries, and the transfer of how mosquitoes would navigate and reproduce within these ecosystems. In the words of Nading (Citation2015a, 203), the ‘global’ in these kinds of global health technologies asserts that ‘it matters, materially and semiotically, in the same way from place to place.’ Inscribing ‘what mattered’ into mosquito bodies was the goal of gene drive laboratory work. However, at the same time, ‘what mattered’ remained an open question. Yet, their potential to transfer hinged on framing it as an inherent property of mosquito bodies. is an illustration from a research article. It shows how the imagined spread of the genetically edited sequence in mosquito populations.
Figure 1. The spread of RNA guided gene drive systems. Source: Akbari, O. S. et al. (2015) Safeguarding gene drive experiments in the laboratory, Science, 349, 927-929.
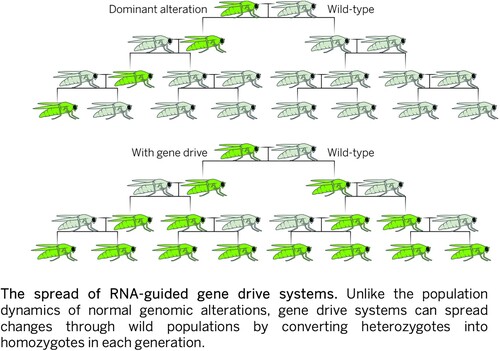
Frederic, a senior microbiologist in a gene drive Anopheles mosquito project, recounted how his career in mosquito genetics had been defined by hopes that research in mosquito genetics could result in a solution for malaria eradication. Frederic spoke of how he saw his research in relation this history:
I think that malaria is a disease of poverty. Because theoretically, or actually not theoretically, practically, there are measures that can be used to eradicate malaria. […] It’s a disease that, in order to eradicate, you need money, resources, tools, political will and stability. Now if any of these fails, then […] the tools that you have dramatically decrease in their efficacy. […] So this given the fact that the major obstacle to eradication of malaria is sustainability, you need to develop a system which is sustainable and possibly independent of resources, political will and logistics. This is why a gene drive for mosquitoes is so appealing. Because the mosquitoes then do the job for you (laughs).
The gene drive element drives the spread; the way the transmission of malaria as a disease would then be reduced or eradicated relies on two alternative strategies. One is to modify mosquito sex-selection genetics in a way that makes them only produce male offspring. Over time, this would lead to the eradication of the Anopheles populations from habitats where gene drive mosquitoes are released. Another alternative modification aims to render the mosquitoes unable to transmit the Plasmodium parasite. This would not result in population eradication but would eventually replace wild-type mosquito populations with ones carrying the modified, malaria-resistant, genome. The benefits of each approach were being weighed in relation to the ecologies they were imagined to be released in. In both cases, however, the gene drive element was what imbued the mosquitoes with a specific potential to eventually spread.
For Frederic, a sustainable solution would be one where gene drive technology is sustained in wild mosquito populations, without human intervention being needed beyond initial release. However, mosquito work does not happen without human intervention, but is incumbent on arrangements both in the laboratory and outside it. Bennett (Citation2019) describes how encounters with non-scalable forms, such as social, political and regulatory challenges, shaped the development of genetically modified mosquitoes in Key West, Florida. In the case of gene drive mosquitoes, the uncertain and open regulatory landscape guides the research in two ways. The projects have to navigate possible pathways for regulatory approval and dealing with environmental concerns.
The uncertainty concerned both the effects of a possible release as well as the feasibility of the strategy. Marcus, a microbiologist in another gene drive mosquito project, enthusiastically compared his approach to biological research with an engineer’s work: ‘It’s just playing, building, see what can be built […] without any necessarily application in mind, and without any fundamental question.’ Marcus’ approach echoed an epistemic focus where research is justified in relation to its future application (Delgado, Citation2016). The same focus contained a tension between the scalar narratives of malaria control on one hand, and on the other hand, the openness that Marcus described.
Stefan Helmreich (Citation2007) has noted how the capacity to do work, articulated as inherent in the organisms themselves, has underpinned biotechnological hopes. The work that gene drive mosquitoes are expected to do was the justification for the technology, as Frederic described, establishing it as separate from what had been done before. Maintaining this potential was how mosquito research in different scales and in different disciplines oriented itself, as I will discuss in the following sections.
Crafting mosquito potentiality in the laboratory
The potentiality of the mosquitoes, however, had to be worked on them in laboratory practices. The gene drive mosquito potential was incumbent on how the mosquitoes became malleable to modification in the laboratory. Studies in STS have discussed how both the research animals themselves and the research infrastructures surrounding them are constitutive in establishing this potential (Friese, Citation2013; Wanderer, 2018).
Unlike experimental animals that were honed and bred for a life in the laboratory however, making the mosquitoes a potential technology required adjustments between making mosquitoes live in the laboratory and equipping them for outside it. In laboratory craftwork, researchers worked on biological materials in order to align their research with the future visions assigned to the technology (cf. Meskus, Citation2018, 218). For the mosquitoes, this included the promise of their ability to reproduce in the wild. Because of this, showing how they worked in standardized laboratory conditions was not enough. Gene drive researchers worked with laboratory mosquitoes by adjusting the living conditions to produce viable strains and usable mosquitoes.
Hannah, a postdoc tending to several Anopheles mosquito strains with a team of research assistants, compared this to working with fruit flies and other Aedes species mosquitoes. As Kelly and Lezaun (Citation2017) have noted, studying mosquitoes in insectaries – facilities that ultimately have the goal of killing the mosquitoes – often the survival of mosquito colonies in fact becomes the most difficult achievement. This achievement was partly possible because of a history of work with other insect species, where infrastructures and skills developed with other species were transferable to Anopheles, and partly by species-specific adjustments in their ways of living (Kohler, Citation1994).
Standardization has been both a central goal and an enduring cause of uncertainty in nurturing experimental life (Kohler, Citation1994; Friese, Citation2013). Kohler (Citation1994) has detailed how modern genetics, as a field of study, developed along with breeding out genetic variability from its model organism, the Drosophila fruit fly. This variability, which ensured the survival of fruit flies in natural environments, posed challenges for their use in the laboratory (Kohler, Citation1994). The potential of gene drive mosquitoes as a malaria control tool, however, is incumbent on them surviving in unpredictable natural environments. Their ability to scale up the malaria control technology, required both transforming them into laboratory tools by reducing the complexities of their living environments, as well as reintroducing aspects of their lives in the wild into the laboratory environment.
A gene drive mosquito starts its life as a genetic construct, transferred into mosquito eggs by sharp quartz needles. When a usable mosquito strain has been established, mosquitoes are then grown under laboratory conditions to examine their biological features and dynamics of reproduction and population growth (). Dupé (Citation2015) has discussed how the insectarium is a central space creating an insect technology: in the laboratory, mosquitoes become unproblematic work tools, as long as they stay in the laboratory. The laboratory was a space where complexities of mosquito life-worlds were reduced; this allowed the scientists working with the mosquitoes to develop skills to work with the insects (Dupé, Citation2015, p. 36).
At the same time, for gene drive mosquitoes, the question of what aspects of mosquito lives were relevant in order to establish their potential to live in the wild was an object of experimentation in itself. Hannah talked about how feeding the laboratory mosquitoes entailed such considerations about what the mosquito populations might become. As she explained, to lay their eggs, Anopheles females require a feed of blood. In their native habitats, Anopheles prefer feeding their young with human blood, which, when carrying the Plasmodium parasite, causes malaria transmission to humans.
Some mosquito researchers use anaesthetized laboratory mice to provide blood to reproducing mosquitoes. Hannah, however, explained how it was important to use human blood: donated blood that was deemed no longer needed by the health services. In this way, the mosquito lines that she works with would not become familiar with animal blood but instead feed on humans, which would make them usable for later experiments with malaria transmission. Feeding human blood to mosquitoes from blood bags is not equivalent to mosquito–human relations in the wild. However it was a way to reconcile the borders of the laboratory to incorporate the question of future mosquito lives in the wild into the insectary. This involved the traffic between both human and mosquito bodies across the laboratory walls.
The scientists’ concerns in laboratory work are related to both creating mosquitoes and mosquito strains that would fare well in laboratory conditions, and to producing data that would be of use in creating the gene drive technology. In Hannah’s work, the potential that was first articulated as a latent force in the mosquito bodies had to be established in laboratory practices. This was done by introducing elements from mosquitoes lives that were considered relevant to their future use. In this way, mosquitoes were crafted to retain in their genome the potential for a life outdoors to carry the gene edit drive into wild-type mosquito populations. The result would not be the genetically engineered mosquito bodies themselves, but an eventual population of mosquitoes capable of producing more mosquitoes. Gene drive laboratory craftwork was oriented towards transforming the potentiality of modifiable and reproducing mosquito bodies into something that emerges in mosquito ecologies.
Population models between the lab and the wild
Another shift in scale and in modes of potentiality occurred in making data from laboratory work and field studies fit together. I have so far explored how that potentiality to work needed to be articulated as a hidden force inherent in mosquito reproduction dynamics. The ‘hidden force’ of mosquito reproduction, the scalability of the technology as a foundational articulation of gene drive potentiality, has to be shown to work at levels of mosquito populations in varying sizes and living environments (cf. Taussig, Hoeyer, and Helmreich, Citation2013, p. S4). Mathematical modelling was used to navigate between microbiologists’ work and that of field biologists.
The potential to spread that the researchers emphasized to be made gene drive mosquitoes a novel technology. The potential of gene drives lies in how the alterations in the mosquito genome get transferred from one mosquito to another. Yet, mosquitoes as organisms as well are not simply products of their genome; nor is a living mosquito the same thing as a mosquito population. A mosquito population living in a laboratory cage is a different population to that living in an arid Sahel environment, or to that living in a tropical rainforest.
Colin, a mathematician in a gene drive mosquito project, spoke of his work as adjusting and comparing data together:
[W]e try to construct models of mosquito population dynamics from different scales and explore the consequences of simulating the releases of the genetically modified mosquitoes and see how different aspects of the environment and the technology will affect the impact it will have. The other parts of my work are working with the field biologists who are collecting data on mosquito populations in the study sites and helping to analyse the data that they get, which will feed into the models as well because we understand more about the mosquitoes from doing that fieldwork. And another part is working with the molecular biologists who are developing the technologies and they’re doing cage experiments with the mosquitoes and doing modelling for those experiments to support them. […] We model the experiments that they do and then we compare the simulated results to the data that they get and if it’s aligning, then that suggests that we understand the system, correctly and that’s encouraging, and if the model results don’t align with the data then that means there’s something more that we have to try to understand.
By adjusting these together, modelling in gene drive research results in a ‘partially connected and comparatively motivated’ (Jensen, Citation2020, p. 81) set of models. Caspar Bruun Jensen (Citation2020) has shown how ecosystem modelling in flood risk forecasting mediates between different sets of data and different concerns in environmental policy. The partial connections in gene drive mosquito models bring together the sites of mosquito population encounters. In this way, it is a way to operate with uncertainties stemming from the ambition to scale up a mosquito population with knowable characteristics from a gene sequence. These uncertainties were placed as part of the future work that underlaid mosquito potential.
In microbiological understanding, the modified element in the mosquito genome will persist and get transferred into each coming generation of mosquitoes. In a caged mosquito population, the mosquito genome encounters evolutionary selection pressures, like all living tools that aim for population suppression. In a population living in less controlled environments, i.e. in locations where they would eventually be released, how mosquitoes migrate and how they aestivate through dry seasons, as well as what affects the survival of eggs and which mosquitoes get to reproduce, all lay increasingly uncertain features into calculations of how the gene drive works.
Uncertainties stem from both the characteristics of mosquitoes as organisms and their reproduction patterns, as well as the environments in which they live. While they are ubiquitous in their endemic landscapes and a long-term focus of malaria research, there are aspects of the lives of Anopheles in the wild affecting their reproduction and death that elude entomologists. In their native habitats, the breeding patterns of Anopheles mosquitoes are seasonal. During the rainy season, they breed in small, ephemeral pools wherever these form, or they can also make use of more permanent bodies of water, such as around a water pump. How and when the eggs hatch and mating swarms are formed follow the movements of people, human habitation, and seasonal rainfall. Anopheles mosquitoes seem to behave as skilled opportunists, able to make use of blood and water as they become available. Colin recounted what he tried to grapple with in his modelling work:
One of the most uncertain things is [that during the dry season] there’s no mosquitoes to be found. And when the rains begin, the mosquitoes come very quickly, so exactly how they’re persisting in the dry season we don’t know. And then there’s just the parameters of mosquito biology that are really hard to measure, like the dispersal, how much are they moving between villages.
In this way, modelling is put in use to make knowable the features of mosquito population dynamics that lie at the core of the gene drive technology. Mediating between the laboratory and the field, between controlled knowledge and ecological uncertainties, and between different sizes of mosquito populations living together, enables the transformation and domestication uncertainties into a calculable population object (Reinert, Citation2012). The potentiality of gene drive mosquito technology emerged in this mediating work between laboratory experiments and field entomological data.
Modelling served to transpose the potential of gene drive mosquitoes from the plasticity inherent in the individual mosquito bodies into a characteristic of the mosquito populations. In a sense, this also established the referential point for evidence of the technology working in an uncertain future. This way of making mosquito population dynamics knowable and usable in research, I argue, was a way to transpose the potential crafted onto individual mosquito bodies in the lab into potential to ‘work in a future time,’ in the words of Delgado (Citation2016).
Regulation and mosquito work
Laboratory craftwork and mathematical modelling, which mediated between mosquitoes as laboratory animals and mosquito ecologies in the wild, worked towards mosquito potentiality in terms of showing how the technology would be efficient in malaria control if released. Another aspect of making the technology usable however concerned its regulation. The complications of making genetically modified organisms governable has been a source of interplay between newly created regulatory entities and the organisms this regulation is expected to oversee (Lezaun, Citation2006).
The gene drive researchers I talked with tended to have two kinds of concerns about the effects of possible gene drive mosquito releases. Firstly, a central issue in their work was showing that modified mosquito populations would have the desired effect in either replacing or reducing mosquito populations. Secondly, questions about the possible environmental effects of these releases, referred to anticipating coming regulation. Gene drive mosquito research had to contend with aiming for a technology that would be governable. While modelling served as a mediator between laboratory work and field biology, concerns about how mosquito ecologies and laboratory work on mosquitoes were assessed were formed differently in relation to the question about possible regulation.
A need for closures that assume stability, uniformity and continuity of complex biological processes has been foundational to enabling regulation of genetically modified organisms (Valve and Kauppila, Citation2008). The potential to work was articulated as if inherent in the mosquitoes themselves. In this way, it also called into question what about them could be stable and continuing in time. Helmreich (Citation2007) has discussed how the generative potential of organisms enrolled in biotechnological projects was solidified as fixed and transferable species. In the case of gene drive mosquitoes category of species and its place in ecologies however was complicated by both the scope of the gene drive project as well as the characteristics of the mosquitoes themselves. Furthermore, Anopheles gambiae is not a species of mosquito, but a species complex. This denotes a group of organisms where the boundaries of a species are unclear and shifting (Besansky et al., Citation1994). The status of modified Anopheles gambiae as a regulatory object was assessed in relation to modelled and abstracted ecologies.
Frederic, the microbiologist who earlier had pinpointed mosquito work to be at the centre of gene drive strategies, reflected on how the research would proceed in anticipation of regulatory demands. Frederic detailed what kind of material they would provide to regulators in anticipation of a release. A scalable mosquito needed abstracted natural environments to accompany it:
Frederic: [The regulatory dossier] will include […] documenting the genetic modification, documenting how the genetic modification spread, documenting its stability, documenting how it will spread, modelling its population, documenting the, assessing the ecological risk, and the environmental risk, providing data on the food web, and modelling how the elimination of the mosquito will impact on other species.
I: Is it going to be specific to one particular place where you would be ready..
Frederic: It’s interesting that you say that because in principle, you should contextualise because of course each environment is different. And this is a kind of limitation of the current approach, also because if the genetic modification spreads, [the mosquito] will spread across different environments. But then you have to make some let’s say assumption, what happens in different environments. You cannot micromanage, but you can identify three or four major environments and model what will happen there.
An alternative strategy to sex-biased mosquito strains is to genetically engineer the Anopheles to be resistant to the malaria parasite. In this way, the mosquitoes could remain part of the ecosystem. Joseph, a PI in a gene drive mosquito project whose mosquitoes were rendered malaria resistant, reflected on the decision to pursue that strategy. As has been noted with already existing malaria control methods, the risk is that other potential malaria transmitting mosquitoes will take over the ecological niche that becomes available after the removal of the mosquitoes targeted by the gene drive approach:
For example, Anopheles gambiae, which is a principal vector, bites while people are sleeping in the middle of the night and then it rests inside people’s houses. So then insecticide, bed nets, and spraying the inside walls of people’s houses with a residual insecticide are very effective against them. Other vectors though, they don’t come inside, they bite earlier in the day. So then bed nets and indoor spraying don’t work against them. We’re not talking about removing these mosquitoes from the environment, and so we’re not going to have that issue of secondary vectors moving into an empty niche.
As a potential and potentially regulatable technology, the potential of gene drive mosquitoes was shifting away from being solely articulated as a characteristic of mosquito bodies. The mosquito bodies and mosquito population data was assessed in relation to abstracted ecosystems and abstracted ecologies. In a manner, this was reminiscent of how Dupé (Citation2015) described the function of the laboratory in manufacturing mosquito tools. A process of fixing and simplifying some properties in space and opening them up again characterized how laboratory work, field entomology and modelling oriented towards each other. This was not a static system or a set goal. Instead, the ephemeral in a future time provided gene drive researchers a space of ambiguity in which to operate.
Conclusion
Gene drive mosquitoes can be seen as both a rearticulation and an intensification of some of the aspirations associated with insect-focused malaria prevention strategies. The concerns surrounding their development both echo and transform existing ones. Gene drive mosquitoes emerge from a field where significant funding and attention have been directed towards technological solutions aimed at transferability and scalability (Cueto, Citation2013).
I have explored how potentiality is crafted in biotechnological research. For researchers working with gene drive mosquitoes, their goal is, I argue, a precarious achievement of assembling articulations of potentiality. What orients these different streams of research is not the aim to refer to some form of a mosquito, out there, but mosquito work that might happen in some future timeline. Establishing this promise orients ways of producing evidence and enacting the mosquito as a research object in the interdisciplinary work in gene drive development.
Using potentiality as an analytic lens to examine what is expected of mosquitoes, and how this is crafted on them, has pointed towards how these are produced and reconciled. Taussig, Hoyer and Helmreich (Citation2013) note this ambiguity of potentiality as a concept and as an analytic. On a similar note, Delgado (Citation2016) has described a shift in the epistemic focus in synthetic biology. Delgado notes how the research was justified not in relation to a reference point in the present time but to work it was expected to do in the future.
Arranging potentiality to scale up into a viable malaria control technology required managing mosquitoes as research objects in different scales. Tsing (Citation2012) has noted how ‘encounters with non-scalable forms’ bear their mark on projects whose defining feature is a goal towards universality. Such biotechnological solutions in global health are, in the words of Nading (Citation2015), ‘leaky objects’: they transcend the boundaries of the categories they are based on. The local encounters and problems universalizable technologies encounter bear their mark on how this universality is achieved and understood (Bennett, Citation2019). I have analysed how mosquito potential is articulated and transposed in using the mosquito as a research object. In making gene drive mosquitoes a viable technology in the lab, bioscientists also produce understandings of biotechnology and how it manages uncertainty.
Working with laboratory mosquitoes required reconciling between standardized laboratory mosquitoes and how aspects of their lives affect their reproduction and malaria transmission. If the potential in the mosquitoes themselves was located in the mosquito bodies’ plasticity and openness to transform, the potential had to be transposed from the malleable mosquito bodies into the population, and eventually the ecological dynamics of the site of transmission.
The question of managing uncertainties that stemmed from the mosquitoes’ ecological existence was a central tension animating much of gene drive research. In gene drive development however, the concern dealt with whether the technology would work: whether the genetic modification in individual mosquito bodies would transform into an intervention that would persist outside the laboratory. Their potential as something modifiable had to be transposed to implicate a force that would sustain beyond laboratory walls. In laboratory work, this meant adjusting with aspects of mosquito lives that were not scalable from the mosquito genome. Likewise, the interplay between microbiology, mathematical modeling and fieldwork served to mediate between how mosquitoes came to exist as research objects in each of them. While the potential to scale up the technology derived from a purified, standardized laboratory mosquito and its genome, open to modification, the concerns involved in turning gene drive mosquitoes into a viable technology also required questioning this foundation. This made the gene drive mosquito a precarious achievement.
Mosquito gene drives aim to be at the same time a universalizable solution that nevertheless encounters the particularities of how mosquitoes’ lives unfold in the laboratory and outside of it. The environmental concerns that were accounted for in research emerged in these encounters and were positioned in relation to the potential of a future malaria control tool. In doing so, I suggest that the gene drive researchers were also modelling how biotechnology conceptualizes and manages uncertainties. In the case I have explored in this article, managing uncertainty is related to the ways in which the mosquitoes are expected to affect the local ecologies they are released in. I have explored gene drive research with the concept of potentiality as it has been used to shed light on knowledge production on experimental animals. The case of gene drive research enrichens this scholarship with an account of how the potentiality that is worked on and out of insect bodies is eventually being made to transcend these bodies.
Acknowledgements
I thank the editors, anonymous referees, and Mianna Meskus and Lotta Hautamäki for their helpful comments on various versions of this manuscript.
Disclosure Statement
No potential conflict of interest was reported by the author(s).
Additional information
Funding
Notes on contributors
Marianne Mäkelin
Marianne Mäkelin is a doctoral researcher at University of Helsinki. She has a background in sociology, and works on human–animal relations and knowledge production in biosciences.
References
- Beisel, U. and Boëte, C. (2013) The flying public health tool: genetically modified mosquitoes and malaria control, Science as Culture, 22(1), pp. 38–60.
- Bennett, P. (2019) Reinventing mosquito control. experimental trials and nonscalable relations in the Florida keys, in: K. Bardosh (Eds) Locating Zika. Social Change and Governance in an Age of Mosquito Pandemics, pp. 195–213 (London: Routledge).
- Besansky, N. J., et al. (1994) Molecular phylogeny of the anopheles gambiae complex suggests genetic introgression between principal malaria vectors, Proceedings of the National Academy of Sciences of the United States of America, 91(15), pp. 6885–6888.
- Birn, A. (2005) Gates’s grandest challenge: transcending technology as public health ideology, The Lancet, 366(9484), pp. 514–519.
- Borup, M., Brown, N., Konrad, K. and Van Lente, H. (2006) The sociology of expectations in science and technology, Technology Analysis & Strategic Management, 18(3–4), pp. 285–298.
- Callaway, E. (2016) “Gene drive” Moratorium Shot Down at UN Biodiversity Meeting, Nature, December 21.
- Cueto, M. (2013) A return to the magic bullet?, in: J. Biehl, and A. Petryna (Eds) When People Come First: Critical Studies in Global Health, pp. 30–53. (Princeton: Princeton University Press).
- Delgado, A. (2016) Assembling desires: synthetic biology and the wish to Act at a distant time, Environment and Planning D: Society and Space, 34(5), pp. 914–934.
- Dupé, S. (2015) Transformer pour contrôler, Revue D’anthropologie des Connaissances, 9(2), pp. 213–236.
- Esvelt, K. M., Smidler, A. L., Catteruccia, F. and Church, G. M. (2014) Emerging technology: concerning RNA-guided gene drives for the alteration of wild populations, eLife, 3, pp. e03401.
- Friese, C. (2013) Realizing potential in translational medicine: The uncanny emergence of care as science, Current Anthropology, 54(S7), pp. S129–S138.
- Helmreich, S. (2007) Blue-Green capital, biotechnological circulation and an oceanic imaginary: A critique of biopolitical economy, BioSocieties, 2(3), pp. 287–302.
- Jensen, C. B. (2020) A flood of models: Mekong ecologies of comparison, Social Studies of Science, 50(1), pp. 76–93.
- Kelly, A. H. and Beisel, U. (2011) Neglected malarias: The frontlines and back alleys of global health, BioSocieties, 6(1), pp. 71–87.
- Kelly, A. H. and Lezaun, J. (2017) The wild indoors: room-spaces of scientific inquiry, Cultural Anthropology, 32(3), pp. 367–398.
- Kohler, R. (1994) Lords of the Fly. Drosophila Genetics and the Experimental Life (Chicago: University of Chicago Press).
- Kohler, R. (2002) Landscapes and Labscapes. Exploring the Lab-Field Border in Biology (Chicago: University of Chicago Press).
- Lezaun, J. (2006) Creating a New object of government: making genetically modified organisms traceable, Social Studies of Science, 36(4), pp. 499–531.
- Lezaun, J. and Porter, N. (2015) Containment and competition: transgenic animals in the One health agenda, Social Science & Medicine, 129, pp. 96–105.
- Mehrabi, T. 2016. Making Death Matter : A Feminist Technoscience Study of Alzheimer’s Sciences in the Laboratory, Doctoral Dissertation, Linköping University, 2016.
- Meskus, M. (2018) Craft in Biomedical Research (New York: Palgrave Macmillan).
- Mitchell, T. (2002) Rule of Experts: Egypt, Techno-Politics, Modernity (Berkeley: University of California Press).
- Mol, A. (2002) The Body Multiple: Ontology in Medical Practice (Durham: Duke University Press).
- Nading, A. M. (2015a) Chimeric globalism: global health in the shadow of the dengue vaccine: chimeric globalism, American Ethnologist, 42(2), pp. 356–370.
- Nading, A. M. (2015b) The lively ethics of global health GMOs: The case of the oxitec mosquito, BioSocieties, 10(1), pp. 24–47.
- Nelson, N. C. (2018) Model Behavior. Animal Experiments, Complexity, and the Genetics of Psychiatric Disorders (Chicago: The University of Chicago Press).
- Quinlan, M., et al. (2018) Studies of transgenic mosquitoes in disease-endemic countries: preparation of containment facilities, Vector Borne and Zoonotic Diseases, 18(1), pp. 21–30.
- Reinert, H. (2012) The disposable surplus: notes on waste, reindeer, and biopolitics, Laboratorium, 4(3), pp. 67–83.
- Reis-Castro, L. (2021) Becoming without, Environmental Humanities, 13(2), pp. 323–347.
- Samimian-Darash, L. and Rabinow, P. (2015) Modes of Uncertainty: Anthropological Cases (Chicago: The University of Chicago Press).
- Stengers, I. (2005) The cosmopolitical proposal, in: B. Latour, and P. Weibel (Eds) Making Things Public, pp. 994–1003 (Cambridge: MIT Press).
- Taussig, K., Hoeyer, K. and Helmreich, S. (2013) The anthropology of potentiality in biomedicine, Current Anthropology, 54(S7), pp. S3–14.
- Tsing, A. L. (2012) On nonscalability, Common Knowledge, 18(3), pp. 505–524.
- Tsing, A. (2015) The Mushroom at the End of the World (Princeton: Princeton University Press).
- Valve, H. and Kauppila, J. (2008) Enacting closure in the environmental control of genetically modified organisms, Journal of Environmental Law, 20(3), pp. 339–362.
- Wanderer, E. (2018) The axolotl in global circuits of knowledge production: Producing multispecies potentiality, Cultural Anthropology, 33(4), pp. 650–667.
- World Health Organization. (2022) World Malaria Report 2022 (Geneva: World Health Organization).