ABSTRACT
Human Milk Oligosaccharides (HMOs) have been proposed to be instrumental in building immune competence. To explore the role of HMOs in allergy prevention, twenty-one HMOs were quantified in breast milk samples and associated with sensitisation in infants. 2′-fucosyllactose (2′-FL) levels were positively associated with an increased risk of sensitisation, atopic dermatitis and recurrent skin rash. Interestingly, 2′-FL levels, ranging from 1.35 to 1.95 g/L, were associated with a higher prevalence of non-allergic and non-sensitised infants. The role of 2′-FL and lacto-N-neotetraose (LNnT) was further investigated in allergic sensitisation models in vivo. Oral administration of HMOs decreased allergic sensitisation. This was associated with gut microbiota and short-chain fatty acid (SCFA) production changes. Aligned with the clinical associations, the decreased sensitisation was not observed with lower and higher tested doses of the HMOs supporting a U-shape association between 2′-FL and LNnT levels and allergic sensitisation risk reduction in humans and mice.
Trial registration: ClinicalTrials.gov identifier: NCT02550236.
Introduction
The incidence of allergic diseases such as atopic dermatitis (AD), food allergy and asthma are increasing globally. Worldwide, 4% of the population (300 million people) suffer from asthma (Braman, Citation2006); in the European Union, 2.5–6% of the population (11–26 million people) have a food allergy (Pawankar et al., Citation2013), and 5–30% of children worldwide suffer from atopic dermatitis or eczema (Pawankar et al., Citation2013; Sigurdardottir et al., Citation2021). While many theories abound regarding the mechanisms by which allergy develops in childhood, understanding how to reduce disease risk or disease progression remains elusive. Environmental factors such as diet (i.e. breastfeeding), pollution, urban lifestyles, cleanliness, pets and mode of delivery have been associated with the developing immune system and allergic disease risk (Annesi-Maesano et al., Citation2021; Pawankar et al., Citation2013; Sigurdardottir et al., Citation2021). In particular, the recognition of breast milk as a uniquely suited, immunologically active fluid that may modulate the development of the immune system and, in turn, the development of allergic disease has gained attention. Breast milk components such as TGF-β, sCD14, exosomes and human milk oligosaccharides (HMOs) are key contributors to this effect (Boix-Amoros et al., Citation2019). There is growing evidence regarding the role of HMOs in early life microbiota composition and activity and its potential impact on the infant immune trajectory and allergy development (Boutin et al., Citation2020). A better understanding of the relationships between the composition of breast milk and, more particularly, its components with a potential prebiotic effect such as HMOs, the gut microbiome and allergy development should help provide novel intervention targets for allergic disease modulation (Boutin et al., Citation2020; Roduit et al., Citation2019).
Synthesised in the mammary glands, HMOs in breast milk can reach up to 20.9 g/L in colostrum and 12.9 g/L in mature milk (Bode, Citation2012). HMOs are structurally varied lactose-based complex glycans, of which over 200 have been identified to date (Bode, Citation2012). The sheer number and structural diversity of HMOs is unique to human breast milk (Boehm & Stahl, Citation2007).
HMO composition is influenced by both environmental and genetic factors and varies greatly across maternal populations. In a study of 410 women across 11 countries, McGuire et al. found that HMO composition differed depending on environmental and geographical factors (McGuire et al., Citation2017). Fucosyltransferase genes drive the structural complexity and quantities of HMOs across populations through the expression of glycosyltransferases. These genes code for enzymes which catalyse the addition of fucose on HMO. As an example, the FUT2 gene, also known as the secretor gene (Se), encodes the enzyme galactoside 2-L-fucosyltransferase 2 (FUT2) and catalyses the addition of Fucose in 1-2-linkage. Mothers carrying inactivating mutations in this gene are referred to as non-secretors (Se-) and consequently, their breast milk is partly deficient in 2'-Fucosyllactose (2′-FL) and Lacto-N-fuctopentaose I (LNFP-I) (Morrow, Citation2011; Smilowitz et al., Citation2014). Similarly, the fucosyltransferase 3 gene (Lewis blood group) (Le) encodes the protein Galactoside 3(4)-L-Fucosyltransferase (FUT3). Individuals with the active Le polymorphism (Le+) express the FUT3 enzyme and can produce α1-3,4-fucosylated HMOs such as LNFP II (Erney et al., Citation2000). Mothers with inactive FUT3 protein are referred to as Lewis negative (Le-) (Maroni et al., Citation2015; Sprenger et al., Citation2017).
Se- carriers account for 15–25% of the European population and conflicting data indicates that Se+ individuals may be more or less sensitive to allergic sensitisation and atopic dermatitis (Maroni et al., Citation2015; Sprenger et al., Citation2017). It is hypothesised that the level of variation in HMO composition has been driven by evolution as pathogens have differing levels of affinity to oligosaccharides (Bode, Citation2015). Yet, its influence on allergy risk is largely unknown.
To date, evidence linking HMOs with allergic manifestations is limited. Some in vitro data suggest that HMOs modulate the allergic response (Bode, Citation2015; Holscher et al., Citation2017). In animal allergy models, 2′-FL, 3′-SL and 6′-SL have been shown to decrease allergic responses (Castillo-Courtade et al., Citation2015; Kang et al., Citation2020; Li et al., Citation2021). While no well-powered intervention study is available as of today to demonstrate the role of HMOs in allergy, human association studies have led to the identification of certain HMOs associated with cow’s milk allergy in infants (Doherty et al., Citation2018; Seppo et al., Citation2017). Although the link between individual HMO levels and atopic dermatitis is still controversial (Marriage et al., Citation2015; Miliku et al., Citation2018; Siziba et al., Citation2022), a combination of 10 HMO levels has been associated with the development of food sensitisation (Miliku et al., Citation2018). Finally, clusters of HMO levels were identified as influencing differently allergic susceptibility (Jiang et al., Citation2021; Lodge et al., Citation2021).
In this study, we aimed to investigate possible associations between 21 HMOs quantified in breastmilk at 3 months postpartum with allergic sensitisation, allergy risk or symptoms in infants at 12 months of age from the LIFE Child Study, a longitudinal cohort study conducted at the Research Center for Civilization Diseases in Leipzig, Germany (Poulain et al., Citation2017). We demonstrated a U-shape association between 2′-FL levels and the absence of sensitisation and clinically diagnosed AD and/or prolonged (>15 days) recurrent itchy rashes in infants. A similar trend was observed for LNnT. This was also observed in vivo, with specific doses of 2′-FL and LNnT influencing allergen sensitisation.
Methods
Study cohort
The samples analysed in this study have been collected from a sub-cohort of the German LIFE Child study (Michel et al., Citation2020; Poulain et al., Citation2017). The study was designed in accordance with the Declaration of Helsinki and under the supervision of the Ethics Committee of the University of Leipzig, Germany, which also approved the study (Reg. No. 264-10-19042010). The LIFE Child study is registered on ClinicalTrials.gov under the clinical trial number: NCT02550236 (Poulain et al., Citation2017). Briefly, 156 mother-infant pairs were selected based on the availability of their responses to a skin symptoms questionnaire in the infant’s first year of life and the availability of breast milk samples at three months of age of the infants. One mother-child pair was excluded due to too many missing values for the breast milk component levels (>50% of measures missing). The third-month postpartum breast milk was expressed and stored at −80°C until analysis, as previously described (Poulain et al., Citation2017).
The “non-allergic non-sensitized” infant group () was identified by excluding infants with clinically diagnosed AD (b), infants diagnosed with food allergy (e), with parents and/or caregivers reporting allergy in the 12 first months and/or infants with parents reporting recurrent (>1 occurrence in the last year) prolonged (>15 days) itchy rashes (c) and/or infants with a risk of allergic sensitisation based on total IgE level at 6 and/or 12 months (a).
Figure 1. Flow chart for inclusion in the study groups. Questionnaires were filled in at 3, 6, 12 m after birth to assess the occurrence of recurrent prolonged itchy rashes (c), clinically diagnosed atopic dermatitis (AD) (b) and sensitisation (a). Non-allergic infants did not have any of the above nor any diagnosis of allergy at any time point.
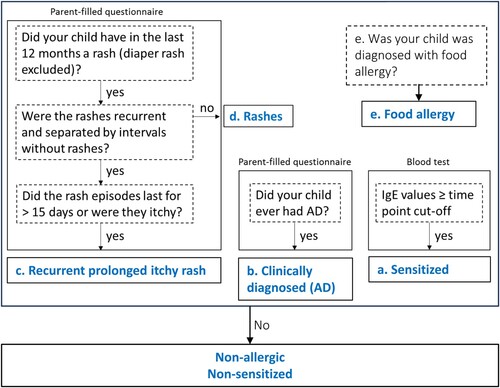
In infants, allergic sensitisation ((a)) was defined as total IgE greater than 35 and 53 kU/L at 6 and 12 months, respectively (Martins et al., Citation2014). Total IgE was quantified using the ImmunoCAP© technology (Phadia, Uppsala, Sweden). Since less than 30% of the children were analysed for total IgE level, this parameter was not considered alone in the analysis. Non-recurrent rashes ((d)), prolonged (> 15 days) and recurring (> 1 occurrence within the last 12 months) skin itchy rashes ((c)) and clinically diagnosed atopic dermatitis ((b)) and food allergy ((e)) were reported by the parents.
Regular diaper rashes and non-recurring, non-persisting, non-itchy rashes ((d)) were excluded from the analysis.
Demographic data for the cohort of 156 mother-infant pairs including some of the leading confounders such as delivery mode, gender, birth weight, breastfeeding exclusivity and duration, economic status, sibling number, parent’s allergy-related questionnaire and atopic diagnosis, were considered in the analyses.
HMO quantification in breast milk
In total, 21 HMOs were quantified using a validated liquid chromatography method (Austin & Benet, Citation2018). Briefly, HMOs were labelled with 2-aminobenzamide and analysed on an Ultimate 3000-RD UHPLC system equipped with an RF-200 fluorimeter, Acquity BEH Glycan and VanGuard BEH amide columns (Waters Corporation, Milford, USA). The temperature of the columns was 55°C, and the flow rate was 0.5 mL min−1. The HMOs 2′-Fucosyllactose (2′-FL), 3-Fucosyllactose (3′-FL), 3′-Sialyllactose (3′-SL), 6′-Sialyllactose (6′-SL), Lacto-N-tetrose (LNT), Lacto-N-neotetraose (LNnT), Lacto-N-fucopentaose I (LNFP I), were quantified by comparison to standard curves with the same HMOs, while 3′-Galactosyllactose (3′-GL), 6′-Galactosyllactose (6′-GL), Lacto-N-fucopentaose II (LNFP II), Lacto-N-fucopentaose III (LNFP III), Lacto-N-fucopentaose V (LNFP V), Lacto-N-neofucopentaose (LNnFP), Lactodifucotetraose (LDF), Lacto-N-tetraose b (LST b), Lacto-N-tetraose c (LST c), difucosyllacto-N-hexaose a (DFLNHa), disialyllacto-N-tetraose (DSLNT), monofucosyllacto-N-hexaose (MFLNH III), Lacto-N-neodifucohexaose (LNnDFH), and Lacto-N-difucohexaose (LNDFH 1) were quantified using maltotriose (Sigma, Buchs, Switzerland) as a surrogate standard assuming equimolar response factors. Samples with 2′-FL levels >50 mg/L were classified as Se positive (Se+) and samples with LNFP II levels >35 mg/L were classified as Le positive (Le+).
Milk FUT2 and FUT3 variant calling
Blood samples from a subset of mothers (n = 118) were collected between 2011 and 2015. DNA was isolated from blood as previously described (Lefebvre et al., Citation2020). The entire FUT2 and FUT3 3′-coding sequences and 5′-regions were PCR amplified as previously described in the same cohort (Lefebvre et al., Citation2020). The quality and quantity of each sample library were checked by gel electrophoresis. Variant calling was performed with the software FreeBayes (Garrison & Marth, Citation2012) using default parameters. To perform the computation of such a large dataset, a script to parallelise the computation was implemented as previously described (Lefebvre et al., Citation2020). Eventually, 23 Single-Nucleotide Polymorphisms (SNPs) and 89 mother-infant pairs samples passed the filters and the QC.
RBL degranulation assay
Beta-lactoglobulin (BLG) allergenicity was measured using an in vitro [3H]serotonin release assay. A rat basophil leukaemia cell line, RBL-2H3 (ATCC, Manassas, VA, United States) was plated at 4 × 104 cells/well in 100 µL. Cells were incubated overnight with HMOs (HMO-2 group) or with medium (control group) and loaded with a rat hyperimmune serum (containing anti-BLG IgE) and radioactive 5-hydroxytryptamine [3H] trifluoroacetate (Anawa, Kloten, Switzerland) at 37 mBq/mL. Cells were then incubated with BLG. Counts per min corresponding to serotonin release, induced by the degranulation of the BLG crosslinking two IgE on the cell surface were quantified. Allergenicity was expressed as the percentage of degranulation considering the control group (no incubation with HMO-2) as 100% degranulation.
Mucosal sensitisation model
The animal study protocol was approved by the “Service Vétérinaire du Canton de Vaud”, Switzerland and the experiments were performed at Ambiotis (Toulouse, France). The model has been previously described in detail (Zuercher et al., Citation2012). Briefly, five-week-old conventional female BALB/cOlaHsd mice from Envigo (Gannat, France) were sensitised for a total of seven consecutive weeks by weekly gavage of 50 mg ovalbumin (OVA, Merck) [sensitised group (S.)] or water [non-sensitised group (N.S.)] in combination with 20 μg cholera toxin (CT) (List Biologicals, purchased from LuBioscience, Lucerne, Switzerland). On day 67 or 68, mice were challenged with an oral administration of 200 mg ovalbumin (OVA; Sigma, Switzerland) and harvested at day 68 or 69 (Supplementary Figure 1A). Two independent experiments were performed with n = 8 animals per group for the exploratory experiment and n = 13 animals per group for the confirmatory experiment.
Epicutaneous sensitisation model
The animal study protocol was approved by the “Service Vétérinaire du Canton de Vaud”, Switzerland. Five-week-old female BALB/c mice (BALB/cByJ JAXTM mice strain 001026 from Charles River, L’Arbresle, France) were epicutaneously sensitised as previously described (Holvoet et al., Citation2016). The back of the mice was shaved with an electric razor and cleaned with 70% isopropanol solution (VWR, Nyon, Switzerland). One hundred μl of Aspergillus fumigatus (Af) protein extract (Greer Laboratories, Lenoir, NC, USA) at 2 mg/mL [sensitised group (S.)] or 100 μL of a 0.9% NaCl solution [non-sensitised group (N.S.)]; (Merck, Schaffhausen, Switzerland) was applied to a 1 × 1 cm patch of sterile gauze (DermaPlast; IVF Hartmann AG, Neuhausen, Switzerland) and secured to the skin with a bio-occlusive transparent dressing (Systagenix, Bioclusive, San Antonio, TX, USA) and a Band-Aid (Mefix, Wasquehal, France) at days 21 and 42, for a minimum of 5 days (Supplementary Figure 1B). Mice were challenged intranasally under isoflurane anaesthesia with 100 μg of Af diluted in 0.9% NaCl on day 51 and euthanized on day 52 (Supplementary Figure 1B). Transepidermal water loss (TEWL) was measured every week in the patch area with a Tewameter (TM300; Courage + Khazaka electronic; Cologne, Germany). Two experiments were performed: one exploratory experiment with n = 8 animals per group and one confirmatory experiment with n = 8–10 animals per group.
Nutritional intervention
An HMO mix (HMO-2) of 2′-FL and LNnT in a 2:1 ratio was incorporated in the pellets of a low-fibre diet (Kliba 2122, Kliba Nafag, Granovit AG, Kaiseraugst, Switzerland) at different concentrations: 0.2%, 1%, 5% and 10% (g/100 g). An HMO-2 supplemented diet was given to the sensitised groups (S.) at 0.2%, 1%, 5% and 10% from study day 1 to day 68 for the mucosal sensitisation model and from study day 1 to day 52 for the epicutaneous sensitisation model (Holvoet et al., Citation2016). Other groups (S. and N.S.) received a normal diet throughout the experiment. The microbiota composition and SCFA levels were analysed as previously described (Trompette et al., Citation2014).
Tissue processing and staining
Mast cell granules were stained following the Naphthol ASD chloroacetate kit protocol (Sigma Aldrich Darmstadt, Germany). Mast cell counts [maximum mast cell count per high power field (x 400)] were performed in duplicate. The mMCP-1 protein was quantified in mouse serum by ELISA following the manufacturer’s protocol (Thermo Fisher, Zug, Switzerland). Upon harvest, mesenteric lymph nodes were collected and pooled 2 by 2 within groups. Tissues were then dissociated on a 40 µm cell strainer (Merck), and cells were resuspended in RPMI (Merck), 10% FCS (Thermo Fischer, Ecublens, Switzerland), and 1% Penicillin–Streptomycin (Sigma). Cells were centrifuged, washed, and prepared at 6 x 106 cells per mL for flow cytometry staining with the following panel: ViobilityTM-ViogreenTM (Miltenyi Biotech, Paris France), CD3e-Vioblue® (Miltenyi Biotech), CD4-PEVio® 770 (Miltenyi Biotech), CD25-Vio® bright-FITC (Miltenyi Biotech) and Foxp3-PE (Miltenyi Biotech). Cells were fixed and permeabilized with a fixation and permeabilization kit (Thermofischer) according to the manufacturer’s protocol.
Quantification of plasma levels of anti-Aspergillus fumigatus IgG1 and anti-OVA IgG1
Quantification of Aspergillus fumigatus-specific IgG1 and OVA-specific IgG1 was performed by ELISA as previously described in Holvoet et al. (Citation2016) and Zuercher et al. (Citation2012), respectively. Results were expressed in µg/mL.
Quantification of mouse mast cell protease 1 (mMCP1) in plasma
Plasma mouse MCP-1, a mast cell-derived mediator of allergic reaction, was quantified using a mMCP-1 ELISA kit (Moredun Scientific, Penicuik, United Kingdom) according to the manufacturer’s instructions.
Statistical analysis
For the mother-infant cohort data, statistical analysis was performed using JMP Pro 14 (SAS Software, Cary, NC, USA). Figures were drawn with JMP Pro 17 (SAS Software) or GraphPad Prism 6 (GraphPad Software, San Diego, USA). For all tests, a p-value of <0.05 was considered significant.
A subset of the infant population (n = 118) was genotyped for FUT2 and FUT3 SNPs to determine infant and maternal Se and Le status (Samuel et al., Citation2019). Descriptive statistics were completed to describe and compare the baseline characteristics in the cohort. For continuous variables, the data was confirmed as non-normally distributed using the Shapiro–Wilk test.
To test for associations between HMOs, SNPs and atopic dermatitis, logistic regression and Chi-squared testing were completed comparing individual HMO levels against the incidence of atopic dermatitis and the presence of SNPs. The following confounding variables were included in the model: gender, delivery mode, parental allergy, breast milk Se and Le status and pets at home. The non-parametric Mann–Whitney-Wilcoxon test was used to compare the differences in the levels of individual HMOs between infants presenting with recurrent prolonged itchy rashes, AD and/or high levels of IgE and non-allergic non-sensitised infants and to compare the differences in the individual HMO levels between Se and Le status in the allergic and non-allergic groups. Box plots were used to compare individual HMO levels between clinically diagnosed allergic and non-allergic infants in each cohort with Se and Le status also included. SNPs were also analysed using Pearson’s test for their association with allergic phenotypes in infants. No false discovery test was used for this small discovery cohort. Quartile distributions were tested with the Pearson’s χ2 test.
For animal experimentation, the exact Wilcoxon non-parametric statistical test was used to compare groups. Results with a p-value ≤0.05 were considered significant. Statistical analyses were performed using the software R 2.14.1. Data are expressed as interquartile ranges. For each outcome, model validity required a significant difference between the sensitised (S.) and non-sensitised (N.S.) groups. All figures are representative of one experiment out of two independent experiments. For the confirmatory experiment, the sample size for each group was calculated according to the outcomes obtained in the exploratory experiment.
Results
Association of 2′-FL and LNnT with the risk of allergic sensitisation and atopic dermatitis
We identified a significant association between 2′-FL levels and [AD (b) + sensitisation (a)] (2194.72 mg/L, n = 36) compared to non-allergic non-sensitised infants (1585 mg/L, n = 41, OR = 1.444, CI95% (1.063–1.954); p = 0.02). A similar association was observed with a group combining [AD (b) +sensitisation (a) + recurrent prolonged itchy skin rash (c)] (2160.25 mg/L, n = 56, OR = 1.333, CI95% (1.059–1.776); p = 0.01) compared to non-allergic non-sensitised infants ( and (a)). For LNnT, associations were not significant, but a trend was observed for the group combining [AD (b) + sensitisation (a) + recurrent prolonged itchy skin rash (c)] (153.74 mg/L, n = 56, OR = 1.200, CI95% (1.000–1.439); p = 0.06) compared to non-allergic non-sensitised infants (123.41 mg/L, n = 41) ( and (a)). No other trend or significant association was identified for the other 19 HMOs.
A closer examination of 2′-FL levels in a quartile analysis revealed that intermediate levels of 2′-FL ranging from 1.35 to 1.95 g/L (Q2) were protective and that levels above 2.4 g/l were associated with a lower prevalence of non-allergic non-sensitised infant, based on the significantly skewed distribution in the different quartiles compared to expected random distributions ((b,c)). Similar results were observed in the distribution of the population within the LNnT quartile levels, where 30.6% of non-allergic non-sensitised infants were in the second quartile and only 12.2% of the non-allergic infants had LNnT levels corresponding to the fourth quartile ((d)) and were significantly different from a random distribution. These results suggest a non-linear U- association between 2′-FL and LNnT levels in breast milk and the absence of allergy or sensitisation and suggest that intermediate levels of these 2 HMOs may have a protective effect.
Figure 2. HMO levels in breast milk samples obtained from the LIFE Child study. The level of 2′-FL in breast milk is shown for the participants and groups based on a chart of inclusion (a). The 2′-FL levels per quartile (Q1, Q2, Q3, Q4) are shown with the % of non-allergic non-sensitised infants in each quartile (b). Representation of the % of “non-allergic, non-sensitized” infants per quartiles for 2′-FL. *, are significant distributions compared to the expected distribution (c). The LNnT levels per quartile (Q1, Q2, Q3, Q4) are shown with the % of non-allergic non-sensitised infants in each quartile (d). The level of 2′-FL in GG, AA and AG genotypes of the genetic variant rs812936 in the FUT3 gene and association with 2′-FL level in breast milk is shown (e). 2′-FL: 2′-Fucosyllactose; HMO: Human Milk Oligosaccharide; AD: Atopic Dermatitis; LNnT: Lacto-N-Neotetraose; FUT3: Fucosyltransferase 3.
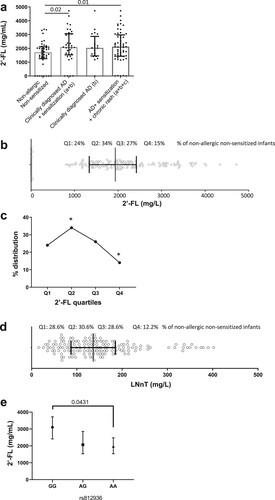
Increased levels of 2′-FL were quantified in Le- breast milk (data not shown), confirming previously published data (Samuel et al., Citation2019). We thus aimed to understand if SNPs in FUT3, driving Le- milk, and higher 2′-FL levels, were associated with a higher prevalence of AD ((b)), sensitisation ((e)) and recurrent itchy skin rash ((c)). Out of the 156 mother-infant pairs, SNPs on FUT2 and FUT3 genes for both mothers and infants were available for 89 pairs. When considered individually, only infant FUT3 SNP rs812936 was found to be significantly associated with the occurrence of AD, sensitisation and recurrent itchy skin rash (p = 0.03, Pearson’s Chi-Squared analysis) (data not shown). Interestingly, the GG homozygote genotype (Le-) for SNP rs812936 in the secretor mothers (secreting 2′-FL) was associated with an increased 2′-FL level (p = 0.0431) compared to AA genotype ((e)). Altogether this result identified a complex interplay between the HMO levels in breast milk, the mother and infant genetics, and the risk for allergy in infants.
Effect of the HMO-2 mix in a murine mucosal sensitisation model
To decipher the specific role of the level of HMOs on allergic sensitisation, we investigated the effect of a blend of 2′-FL and LNnT (HMO-2) at 0.2%, 1%, 5% and 10% in a mouse mucosal sensitisation model, using ovalbumin (OVA) and cholera toxin (CT) administered intragastrically (Supplementary Figure 1a). HMO-2 at a dose of 5% (group S. 5%) significantly inhibited the OVA-specific IgG1 (p = 0.0195) induced by the sensitisation (group S.). No effect was observed when mice were supplemented with HMO-2 at 0.2, 1 and 10% ((a)). This decrease in sensitisation was accompanied by an increase in regulatory T cell (Tregs) counts in the mesenteric lymph nodes, with a maximum increase observed in the 5% HMO-2 group ((b)). Mast cell numbers were also decreased in the jejunum in a dose-dependent manner with a significant effect observed at 5% HMO-2 (p = 0.0013) as compared to the S. group ((c)) and mMCP1 levels in serum were significantly decreased in mice receiving 5% (p = 0.010) and 10% (p = 0.0162) HMO-2 ((d)).
Figure 3. Effect of the 2′-FL and LNnT HMO blend at different concentrations in the drinking water in a mucosal sensitisation model. Specific IgG1 levels in serum (a), Foxp3+ CD4 T cells in mesenteric lymph nodes (b), number of mucosal mast cells (c) and serum mMCPT1 (d) are shown. In faeces, the relative abundance (e), richness (f) and abundance of Parabacteriodes (g) and Akkermensia (h) are also shown for mice supplemented with a mix of 2′-FL and LNnT at 0.2%, 1%, 5% and 10% supplementation in sensitised animal (S.). Data are expressed as median ± interquartile ranges. 2′-FL: 2′-Fucosyllactose; LNnT: Lacto-N-Neotetraose; HMO: Human Milk Oligosaccharides; mMCPT1: mouse Mast Cell Protease-1.
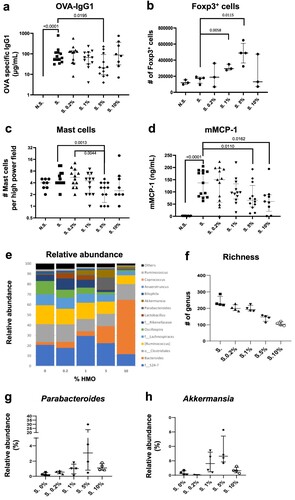
The analysis of the faecal microbiota showed an HMO-2 dose-dependent decrease in the phylogenetic diversity (phylogenetic differences among species, (e)) and richness (the number of observed species) ((f)), underscoring a selective modulation of various organisms such as Bacteroides ((e)). Specifically, the HMO-2 blend led to a significant and expected increase in Bifidobacteria at 10% HMO-2 (p < 0.001) (data not shown) together with a decrease in Bilophila at 1% (p = 0.0014), 5% (p = 0.0001) and 10% (p = 0.0001) HMO-2 in the diet ((e)). The observed decrease in mucosal sensitisation induced by a 5% HMO-2 supplementation was associated with the composition changes in the microbiota, characterised by a peak in abundance of Parabacteroides ((g)) and Akkermansia ((h)). To conclude, HMO-2 at the specific dose of 5% reduces CT-induced OVA mucosal sensitisation. This effect may be partially explained by changes in the microbiota and increased Treg levels.
HMO-2 decreases allergic sensitisation in a murine epicutaneous sensitisation model
To confirm whether intermediate doses of oral administration with 2′-FL and LNnT can protect against allergic sensitisation we used an epicutaneous sensitisation model, as an alternative sensitisation route to the gastrointestinal sensitisation (Supplementary Figure 1b). The oral administration of the HMO-2 blend tended to reduce allergen-specific IgG1 response only at the lower HMO-2 doses tested (0.2% (S. 0.2% group; ns) and 1% (S. 1% group; p = 0.065)) as compared to the sensitised group (S.) ((a)). This was accompanied by a decrease in IL-4 production in restimulated lymph nodes (p = 0.0093 and p = 0.0379 for the S. 0.2% and S. 1% groups, respectively) ((b)). The increase in transepidermal water loss (TEWL), a proxy measure of skin barrier function, observed in the sensitised (S.) compared to the non-sensitised (N.S.) mice (p < 0.001), was not affected by the lower concentrations (0.2 and 1%) of the HMO-2 blend administration. However, we observed an increase in TEWL in mice receiving 5% (ns) and 10% (p = 0.0263) of the HMO-2 blend ((c)) while histological changes were not observed. This TEWL increase was sustained for several days after removal of the patch (data not shown). Caecum propionate was increased significantly by the HMO-2 supplementation in the group receiving 5% and 10% ((d)) and similar microbiota results as in the mucosal sensitisation model were observed in the epicutaneous model (data not shown). Altogether the results suggested that an intermediate dose (1%) of the HMO-2 blend was associated with a decrease in allergic sensitisation while the increased TEWL observed in the groups of mice receiving 10% HMO-2 was not associated with allergic sensitisation. The impact of HMO-2 on allergic sensitisation is possibly occurring through SCFA production and may be counterbalanced by an effect of HMO-2 on skin barrier function possibly favouring sensitisation at a high dose.
Figure 4. Effect of the 2′-FL and LNnT HMO blend at different concentrations in the diet in an epicutaneous sensitisation model. Specific IgG1 (a), IL-4 levels in serum (b), transepidermal water loss (TEWL) individual levels after the second patch (c) caecum propionate (d) are shown, for the 2′-FL and LNnT blend at 0.2, 1, 5 and 10% supplementation in sensitised animal (S.). Data are expressed as median ± interquartile ranges. 2′-FL: 2′-Fucosyllactose; LNnT: Lacto-N-Neotetraose; HMO: Human Milk Oligosaccharides, TEWL: Transepidermal Water Loss.
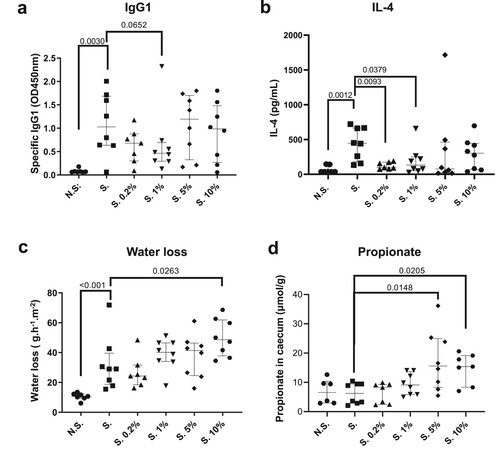
Discussion
The identification of the specific components of breast milk which may contribute to the prevention of allergic disease remains poorly understood (Doherty et al., Citation2018; Kang et al., Citation2020; Lodge et al., Citation2021; Maroni et al., Citation2015; Marriage et al., Citation2015; Miliku et al., Citation2018; Poulain et al., Citation2017; Seppo et al., Citation2017; Siziba et al., Citation2022). The possible beneficial role of HMOs is of special interest since specific HMOs are now available as supplements in infant formulas. Taken together, our results from human association studies, murine sensitisation models and cellular in vitro studies, suggest potential benefits of 2′-FL and LNnT for the prevention of allergy. Both preclinical and human observational studies suggest that consumption of HMO within defined ranges may be beneficial. The data also underscore the interplay between dose-dependent responses of the oral administration of a blend of 2′-FL and LNnT on allergic sensitisation, including direct effects on gut microbiota and indirect effects on Tregs and TEWL.
To date, clinical evidence linking HMOs with the development of allergic diseases is limited and relies on a small number of observational studies. Sjogren et al. (Citation2007) were among the first to examine the association of oligosaccharides in breast milk and the development of allergy in a small group of children (n = 20) up to 18 months of age. They observed that, even though not significant, the children with allergies consumed higher amounts of total neutral HMOs from colostrum. In breastfed infants with a high familial risk for allergy, Maroni et al. (Citation2015) observed a beneficial association between IgE-mediated allergic disease, primarily eczema, and the quantity of FUT2-regulated oligosaccharides (including 2′-FL) in breast milk at two years of age in C-section born infants. In another study, Kang et al. (Citation2020) were unable to find a significant association between Se status and cow’s milk allergy (CMA) within the first 18 months of life in a cohort of 80 infants. However, after adjusting for potential confounders, they found significantly lower levels of 6′-SL, LNFP I and DSLNT in the breast milk consumed by infants with CMA than infants without CMA. Furthermore, infants consuming breast milk with <60 µm LNFP III were more likely to develop CMA than infants getting higher LNFP III quantities from breast milk. The authors also noted that levels of LSTc, DSLNT and 6′-SL were lower in the milk of mothers with an infant with AD compared to infants without AD. Marriage et al. (Citation2015) observed that the overall HMO profiles in breast milk at 3–4 months were significantly different between sensitised and non-sensitised infants diagnosed with food allergy by one year of age and suggested, in line with our results, a non-linear association of 2′-FL and LNnT with allergy development. They identified that higher levels of 6 HMOs, including LNnT, and lower levels of 4 HMOs, including 2′-FL, were associated with lower risk of food allergy (Marriage et al., Citation2015). Recently, Chen et al. (Citation2023) also suggested a positive association between atopic dermatitis and 2′-FL levels; while Siziba et al. showed no significant association between AD and HMO levels (Citation2022). The absence of quartile analysis does not allow confirmation whether the association or absence of association in these studies was also driven or not by higher levels of HMO.
The present results provide additional preclinical evidence on the role of HMOs in allergy. The 2′-FL and LNnT blend induced a decrease in allergic sensitisation in 2 different in vivo models. These data support the available literature. In an ovalbumin-sensitised mouse model of food allergy (Castillo-Courtade et al., Citation2015) and a mouse model of house dust mite-induced allergy, mimicking the development of AD (Kang et al., Citation2020), reduced allergic sensitisation and/or symptoms were associated with an induction of Tregs after HMO administration. We confirmed these findings in murine skin and mucosal sensitisation models, using specific doses of the HMO-2 mix. The optimal dose(s) were not the same in the two models suggesting that the dose-effect of HMO-2 is dependent on the route of allergen sensitisation. In the mucosal sensitisation model, the increase in mesenteric Foxp3+ Tregs observed in the groups receiving 1% and 5% HMOs supports the findings of Castillo-Courtade et al. (Citation2015) and Kang et al. (Citation2020). Our data showed an HMO-2 dose-dependent effect on both Tregs and allergen-specific-IgG levels. In vitro data also suggest that the role of 2′-FL and LNnT in dampening the allergic response may, at least partly, be linked with stabilisation of mast cells as shown by a decrease in in vitro degranulation by HMO-2 mix (Supplementary Figure 2). The observed dose-dependent decrease in serum mMCPT1 and the reduction of mast cell numbers in the intestine support the stabilisation of mast cells observed in vitro and demonstrated in vivo and ex vivo by others (Castillo-Courtade et al., Citation2015). Results on TEWL in the skin sensitisation model showed an increase in skin barrier dysfunction with higher doses of HMOs. This is in line with the beneficial impact of lower HMO dose on IL-4, Tregs and mast cell responses, not observed at higher doses. It can be speculated that a similar impairment of the gut intestinal epithelium could occur with higher doses of HMOs and, in accordance with the “barrier regulation hypothesis” (Wesemann & Nagler, Citation2016), this could contribute to a general loss of the protective effect against allergy development at high dose.
HMOs are known to modulate the composition of the gut microbiota, e.g. stimulating the growth of the beneficial Bifidobacterium species (Asakuma et al., Citation2011; Borewicz et al., Citation2020). Healthy breastfed infants exhibit lower faecal microbial diversity than formula-fed infants and this is primarily attributed to a greater proportion of beneficial bacteria including Bifidobacteria and Lactobacilli. Elison et al. (Citation2016) have demonstrated that supplementation with 2′-FL and LNnT, alone or as a mix, leads to an increased relative abundance of Actinobacteria and Bifidobacterium and a decreased relative abundance of Firmicutes and Proteobacteria. In comparison, infants with cow’s milk allergy display lower gut levels of Bifidobacteria (Rachid & Chatila, Citation2016); Gold et al. have demonstrated that 2'-FL and LNnT supplementation of an amino acid-based formula increased abundances of HMO-utilizing Bifidobacteriaceae in infants with cow’s milk allergy (Boulangé et al., Citation2023; Gold et al., Citation2022). Additionally, other data supporting the association of their bifidogenic effect with health outcomes have been proposed (Berger et al., Citation2020). Our in vivo results support a dose-dependent effect of HMO-2 on the gut microbiota composition with an overall decrease in microbiota diversity coupled with increases in specific beneficial bacterial groups. Anti-allergic properties have been attributed to SCFA ability to enhance the induction and function of Tregs and dendritic cells (Tan et al., Citation2016). Notably, the SCFA propionate (and butyrate), for which high levels in feces at one year of age have been associated with decreased atopic dermatitis (Roduit et al., Citation2019), was significantly increased at the two highest HMO concentrations in the skin sensitisation model. Whether this finding is related to differences in the sensitisation pathways remains to be clarified. Yet, this result suggests that HMO-2 fermentation derived-propionate may not fully drive the allergic sensitisation decrease that was previously demonstrated in other allergic models (Hu et al., Citation2022) and suggests that HMO-2 may effect differently than only through a prebiotic or SCFA effect. Finally, a recently published paper showed that a 2′-FL + LNnT supplementation led to the enrichment of Bifidobacteria in the gut microbiome and delayed the shift of the microbiome composition toward an adult-like pattern in infants with CMA, partially correcting the dysbiosis commonly observed in these infants (Tan et al., Citation2016).
In conclusion, the human association data in breast milk collected in mothers of three-month-old infants revealed that intermediate levels of 2′-FL and LNnT are associated with a lower prevalence of cutaneous sensitisation and skin symptoms in infants up to one year of age. We have also provided evidence in preclinical murine models that a blend of 2′-FL and LNnT can influence a number of direct and indirect biological responses contributing to allergic sensitisation reduction. Measurable effects in these murine models of allergy point to effects on sensitisation that are dose-dependent. The in vivo results show a U-shaped response to 2′-FL and LNnT on microbiota, sensitisation and Tregs outcomes, which may at least partly explain the human observation. Different effects depending on the HMO profiles and levels could also explain contradictory reports in the literature as to whether HMOs exert a beneficial effect on allergic disease. The association data between levels of 2′-FL and LNnT and sensitisation and allergy risk reduction observed in this cohort needs to be confirmed in other studies. Also, well-powered, randomised controlled intervention studies are needed to conclude whether HMOs, specifically 2′-FL and LNnT could reduce the development of allergic sensitisation and/or allergic symptoms in infants and children.
Ethics statement
The samples analysed in this study have been collected from a sub-cohort of the German LIFE Child study (Michel et al., Citation2020; Poulain et al., Citation2017). To participate in the study, written informed consent was obtained from breast milk donors; for samples and/or medical information from infants, consent was obtained from their parents or legal guardians. The German LIFE Child study was designed in accordance with the Declaration of Helsinki and under the supervision of the Ethics Committee of the University of Leipzig, Germany, which also approved the study (Reg. No. 264-10-19042010). The LIFE Child study is registered on ClinicalTrials.gov under the clinical trial number: NCT02550236. The animal study protocols were approved by the “Service Vétérinaire du Canton de Vaud”, Switzerland under the authorisation VD2382.
Supplemental Material
Download MS Word (141.4 KB)Acknowledgements
The authors would like to thank Eline van der Beek and Giles Major for their critical review of the manuscript. Authors’ Contribution: Sébastien Holvoet, Cheong Kwong Chung, Tristan Bourdeau, and Dominique Donnicola designed and performed the in vivo, ex vivo, and in vitro experiments, analysed the results, drafted the manuscript and the figures, reviewed and agreed with the manuscript. Francis Foata and Severine Combremont performed the microbiota analysis, analysed the data, drafted the figures for the manuscript, reviewed and agreed with the final manuscript; Ellen Ní Cléirigh and Maya Shevlyakova analysed the human data and generated the statistical reports linking HMO and infant health outcomes, drafted the manuscript, reviewed and agreed with the final manuscript; Gregory Lefevre, and Aristea Binia analysed the data on variant analysis, drafted the manuscript, reviewed and agreed with the final manuscript; Chiara Nembrini, Mandy Vogel, and Wieland Kiess designed and managed the human clinical data acquisition and HMO quantification, designed the analytical plan, drafted the manuscript, reviewed and agreed with the final manuscript; Norbert Sprenger, Mario Noti, and Sophie Nutten advised on scientific strategy, provided resources, reviewed and agreed with the manuscript; Carine Blanchard ideated the work, designed experiments, analysed the statistical reports, drafted the manuscript, reviewed and agreed with the final manuscript and serves as the corresponding author.
Disclosure statement
No potential conflict of interest was reported by the author(s).
Data availability statement
The statistical plan and reports of the human clinical data as well as the protocol, statistical plan and statistical report of the animal studies are available upon request.
Additional information
Funding
References
- Annesi-Maesano, I., Fleddermann, M., Hornef, M., von Mutius, E., Pabst, O., Schaubeck, M., & Fiocchi, A. (2021, November 12). Allergic diseases in infancy: I – epidemiology and current interpretation. World Allergy Organization Journal, 14(11), 100591. PMID: 34820047; PMCID: PMC8593659. https://doi.org/10.1016/j.waojou.2021.100591
- Asakuma, S., Hatakeyama, E., Urashima, T., Yoshida, E., Katayama, T., Yamamoto, K., Kumagai, H., Ashida, H., Hirose, J., & Kitaoka, M. (2011). Physiology of consumption of human milk oligosaccharides by infant gut-associated Bifidobacteria. Journal of Biological Chemistry, 286(40), 34583–34592. https://doi.org/10.1074/jbc.M111.248138
- Austin, S., & Benet, T. (2018). Quantitative determination of non-lactose milk oligosaccharides. Analytica Chimica Acta, 1010, 86–96. Epub 2018/02/16. PubMed PMID: 29447675. https://doi.org/10.1016/j.aca.2017.12.036
- Berger, B., Porta, N., Foata, F., Grathwohl, D., Delley, M., Moine, D., Charpagne, A., Siegwald, L., Descombes, P., Alliet, P., Puccio, G., Steenhout, P., Mercenier, A., & Sprenger, N. (2020). Linking human milk oligosaccharides, infant fecal community types, and later risk to require antibiotics. mBio, 11(2), Epub 2020/03/19. PubMed PMID: 32184252; PubMed Central PMCID: PMCPMC7078481. https://doi.org/10.1128/mBio.03196-19
- Bode, L. (2012). Human milk oligosaccharides: Every baby needs a sugar mama. Glycobiology, 22(9), 1147–1162. Epub 2012/04/20. PubMed PMID: 22513036; PubMed Central PMCID: PMCPMC3406618. https://doi.org/10.1093/glycob/cws074
- Bode, L. (2015). The functional biology of human milk oligosaccharides. Early Human Development, 91(11), 619–622. Epub 2015/09/17. PubMed PMID: 26375354. https://doi.org/10.1016/j.earlhumdev.2015.09.001
- Boehm, G., & Stahl, B. (2007). Oligosaccharides from milk. The Journal of Nutrition, 137(3 Suppl 2), 847S–849S. Epub 2007/02/22. PubMed PMID: 17311985. https://doi.org/10.1093/jn/137.3.847S
- Boix-Amoros, A., Collado, M. C., Van't Land, B., Calvert, A., Le Doare, K., Garssen, J., et al. (2019). Reviewing the evidence on breast milk composition and immunological outcomes. Nutrition reviews, 77(8) , 541–556. https://doi.org/10.1093/nutrit/nuz019
- Borewicz, K., Gu, F., Saccenti, E., Hechler, C., Beijers, R., de Weerth, C., van Leeuwen, S. S., Schols, H. A., & Smidt, H. (2020). The association between breastmilk oligosaccharides and faecal microbiota in healthy breastfed infants at two, six, and twelve weeks of age. Scientific Reports, 10(1), 4270. https://doi.org/10.1038/s41598-020-61024-z
- Boulangé, C. L., Pedersen, H. K., Martin, F. P., Siegwald, L., Pallejà Caro, A., Eklund, A. C., Jia, W., Zhang, H., Berger, B., Sprenger, N., & Heine, R. G. (2023, July 13). An extensively hydrolyzed formula supplemented with two human milk oligosaccharides modifies the fecal microbiome and metabolome in infants with cow’s milk protein allergy. International Journal of Molecular Sciences, 24(14), 11422. PMID: 37511184; PMCID: PMC10379726. https://doi.org/10.3390/ijms241411422
- Boutin, R. C. T., Sbihi, H., Dsouza, M., Malhotra, R., Petersen, C., Dai, D., Sears, M. R., Moraes, T. J., Becker, A. B., Azad, M. B., Mandhane, P. J., Subbarao, P., Finlay, B. B., & Turvey, S. E. (2020). Mining the infant gut microbiota for therapeutic targets against atopic disease. Allergy, 75(8), 2065–2068. Epub 2020/02/23. PubMed PMID: 32086944. https://doi.org/10.1111/all.14244
- Braman, S. S. (2006). The global burden of asthma. Chest, 130(1 Suppl), 4S–12S. Epub 2006/07/15. PubMed PMID: 16840363. https://doi.org/10.1378/chest.130.1_suppl.4S
- Castillo-Courtade, L., Han, S., Lee, S., Mian, F. M., Buck, R., & Forsythe, P. (2015). Attenuation of food allergy symptoms following treatment with human milk oligosaccharides in a mouse model. Allergy, 70(9), 1091–1102. Epub 2015/05/15. PubMed PMID: 25966668. https://doi.org/10.1111/all.12650
- Chen, Y., Chiou, A. J., Leung, A. S. Y., Chan, K. C. C., Chang, M. K., Cheng, N. S., Chan, P. K. S., Wong, M. S., Tam, W. H., & Leung, T. F. (2023, April 17). Human milk oligosaccharides in Chinese lactating mothers and relationship with allergy development in offspring. Asian Pacific Journal of Allergy and Immunology, Epub ahead of print. PMID: 37061931. https://doi.org/10.12932/AP-110922-1453
- Doherty, A. M., Lodge, C. J., Dharmage, S. C., Dai, X., Bode, L., & Lowe, A. J. (2018). Human milk oligosaccharides and associations with immune-mediated disease and infection in childhood: A systematic review. Frontiers in Pediatrics, 6, 91. Epub 2018/05/08. PubMed PMID: 29732363; PubMed Central PMCID: PMCPMC5920034. https://doi.org/10.3389/fped.2018.00091
- Elison, E., Vigsnaes, L. K., Rindom Krogsgaard, L., Rasmussen, J., Sørensen, N., McConnell, B., Hennet, T., Sommer, M. O. A., & Bytzer, P. (2016). Oral supplementation of healthy adults with 2′-O-fucosyllactose and lacto-N-neotetraose is well tolerated and shifts the intestinal microbiota. British Journal of Nutrition, 116(8), 1356–1368. Epub 2016/10/10. PMID: 27719686. https://doi.org/10.1017/S0007114516003354
- Erney, R. M., Malone, W. T., Skelding, M. B., Marcon, A. A., Kleman-Leyer, K. M., O'Ryan, M. L., Ruiz–Palacios, G., Hilty, M. D., Pickering, L. K., & Prieto, P. A. (2000). Variability of human milk neutral oligosaccharides in a diverse population. Journal of Pediatric Gastroenterology and Nutrition, 30(2), 181–192. PMID: 10697138. https://doi.org/10.1097/00005176-200002000-00016
- Garrison, E., & Marth, G. (2012). Haplotype-based variant detection from short-read sequencing. arXiv. 1207.
- Gold, M. S., Quinn, P. J., Campbell, D. E., Peake, J., Smart, J., Robinson, M., O’Sullivan, M., Vogt, J. K., Pedersen, H. K., Liu, X., Pedersen, H. K., Liu, X., Pazirandeh-Micol, E., & Heine, R. G. (2022). Effects of an amino acid-based formula supplemented with two human milk oligosaccharides on growth, tolerability, safety, and gut microbiome in infants with cow’s milk protein allergy. Nutrients, 14(11), 2297. https://doi.org/10.3390/nu14112297
- Holscher, H. D., Bode, L., & Tappenden, K. A. (2017). Human milk oligosaccharides influence intestinal epithelial cell maturation in vitro. Journal of Pediatric Gastroenterology & Nutrition, 64(2), 296–301. Epub 2017/01/24. PubMed PMID: 28114245. https://doi.org/10.1097/MPG.0000000000001274
- Holvoet, S., Doucet-Ladeveze, R., Perrot, M., Barretto, C., Nutten, S., & Blanchard, C. (2016). Beneficial effect of Lactococcus lactis NCC 2287 in a murine model of eosinophilic esophagitis. Allergy, 71(12), 1753–1761. Epub 2016/06/05. PubMed PMID: 27259693. https://doi.org/10.1111/all.12951
- Hu, M., Alhamwe, B. A., Santner-Nanan, B., Miethe, S., Harb, H., Renz, H., Potaczek, D. P., & Nanan, R. K. (2022). Short-chain fatty acids augment differentiation and function of human induced regulatory T cells. International Journal of Molecular Sciences, 23(10), 5740. PMCID: PMC9143307. https://doi.org/10.3390/ijms23105740
- Jiang, S., Pan, J., Li, Y., Ju, M., Zhang, W., Lu, J., Lv, J., & Li, K. (2021). Comprehensive human milk patterns are related to infant growth and allergy in the CHMP study. Molecular Nutrition & Food Research, 65(17), e2100011. Epub 2021/07/07. PubMed PMID: 34227225. https://doi.org/10.1002/mnfr.202100011
- Kang, L. J., Oh, E., Cho, C., Kwon, H., Lee, C. G., Jeon, J., Lee, H., Choi, S., Han, S. J., Nam, J., Song, C.-u., Jung, H., Kim, H. Y., Park, E.-J., Choi, E.-J., Kim, J., Eyun, S.-i., & Yang, S. (2020). 3′-Sialyllactose prebiotics prevents skin inflammation via regulatory T cell differentiation in atopic dermatitis mouse models. Scientific Reports, 10(1), 5603. Epub 2020/03/30. PubMed PMID: 32221370; PubMed Central PMCID: PMCPMC7101440. https://doi.org/10.1038/s41598-020-62527-5
- Lefebvre, G., Shevlyakova, M., Charpagne, A., Marquis, J., Vogel, M., Kirsten, T., Kiess, W., Austin, S., Sprenger, N., & Binia, A. (2020). Time of lactation and maternal fucosyltransferase genetic polymorphisms determine the variability in human milk oligosaccharides. Frontiers in Nutrition, 7, 574459. Epub 2020/11/17. PubMed PMID: 33195368; PubMed Central PMCID: PMCPMC7658960. https://doi.org/10.3389/fnut.2020.574459
- Li, A., Li, Y., Zhang, X., Zhang, C., Li, T., Zhang, J., & Li, C. (2021). The human milk oligosaccharide 2′-fucosyllactose attenuates β-lactoglobulin–induced food allergy through the miR-146a–mediated toll-like receptor 4/nuclear factor-κB signaling pathway. Journal of Dairy Science, 104(10), 10473–10484. Epub 2021/08/03. PubMed PMID: 34334202. https://doi.org/10.3168/jds.2021-20257
- Lodge, C. J., Lowe, A. J., Milanzi, E., Bowatte, G., Abramson, M. J., Tsimiklis, H., Axelrad, C., Robertson, B., Darling, A. E., Svanes, C., Wjst, M., Dharmage, S. C., & Bode, L. (2021). Human milk oligosaccharide profiles and allergic disease up to 18 years. Journal of Allergy and Clinical Immunology, 147(3), 1041–1048. Epub 2020/07/11. PubMed PMID: 32650022. https://doi.org/10.1016/j.jaci.2020.06.027
- Maroni, L., van de Graaf, S. F., Hohenester, S. D., Oude Elferink, R. P., & Beuers, U. (2015). Fucosyltransferase 2: A genetic risk factor for primary sclerosing cholangitis and Crohn's disease—a comprehensive review. Clinical Reviews in Allergy & Immunology, 48(2-3), 182–191. Epub 2014/05/16. PubMed PMID: 24828903. https://doi.org/10.1007/s12016-014-8423-1
- Marriage, B. J., Buck, R. H., Goehring, K. C., Oliver, J. S., & Williams, J. A. (2015). Infants fed a lower calorie formula with 2′FL show growth and 2′FL uptake like breast-fed infants. Journal of Pediatric Gastroenterology & Nutrition, 61(6), 649–658. Epub 2015/07/15. PubMed PMID: 26154029; PubMed Central PMCID: PMCPMC4645963. https://doi.org/10.1097/MPG.0000000000000889
- Martins, T. B., Bandhauer, M. E., Bunker, A. M., Roberts, W. L., & Hill, H. R. (2014). New childhood and adult reference intervals for total IgE. Journal of Allergy and Clinical Immunology, 133(2), 589–591. PubMed PMID: 24139495. https://doi.org/10.1016/j.jaci.2013.08.037
- McGuire, M. K., Meehan, C. L., McGuire, M. A., Williams, J. E., Foster, J., Sellen, D. W., Kamau-Mbuthia, E. W., Kamundia, E. W., Mbugua, S., Moore, S. E., Prentice, A. M., Kvist, L. J., Otoo, G. E., Brooker, S. L., Price, W. J., Shafii, B., Placek, C., Lackey, K. A., Robertson, B., … Bode, L. (2017). What's normal? Oligosaccharide concentrations and profiles in milk produced by healthy women vary geographically. The American Journal of Clinical Nutrition, 105(5), 1086–1100. Epub 2017/03/31. PubMed PMID: 28356278; PubMed Central PMCID: PMCPMC5402033. https://doi.org/10.3945/ajcn.116.139980
- Michel, L., Shevlyakova, M., Ni Cleirigh, E., Eckhardt, E., Holvoet, S., Nutten, S., Sprenger, N., Körner, A., Vogel, M., Nembrini, C., Kiess, W., & Blanchard, C. (2020). Novel approach to visualize the inter-dependencies between maternal sensitization, breast milk immune components and human milk oligosaccharides in the LIFE Child cohort. PLoS One, 15(4), e0230472. Epub 2020/04/22. PubMed PMID: 32315306; PubMed Central PMCID: PMCPMC7173766. https://doi.org/10.1371/journal.pone.0230472
- Miliku, K., Robertson, B., Sharma, A. K., Subbarao, P., Becker, A. B., Mandhane, P. J., Turvey, S. E., Lefebvre, D. L., Sears, M. R., Bode, L., & Azad, M. B. (2018). Human milk oligosaccharide profiles and food sensitization among infants in the CHILD study. Allergy, 73(10), 2070–2073. PubMed PMID: 29775217. https://doi.org/10.1111/all.13476
- Morrow, A. L. (2011). Infant feeding in the 21st century. Journal of Pediatric Health Care, 25(3), 195–197. Epub 2011/04/26. PubMed PMID: 21514496. https://doi.org/10.1016/j.pedhc.2011.02.011
- Pawankar, R., Canonica, G. W., Holgate, S. T., Lockey, R. F., & Blaiss, M. S. (2013). The WAO white book on allergy (Update 2013).
- Poulain, T., Baber, R., Vogel, M., Pietzner, D., Kirsten, T., Jurkutat, A., Hiemisch, A., Hilbert, A., Kratzsch, J., Thiery, J., Fuchs, M., Hirsch, C., Rauscher, F. G., Loeffler, M., Körner, A., Nüchter, M., & Kiess, W. (2017). The LIFE child study: A population-based perinatal and pediatric cohort in Germany. European Journal of Epidemiology, 32(2), 145–158. Epub 2017/02/02. PubMed PMID: 28144813. https://doi.org/10.1007/s10654-016-0216-9
- Rachid, R., & Chatila, T. A. (2016). The role of the gut microbiota in food allergy. Current Opinion in Pediatrics, 28(6), 748–753. https://doi.org/10.1097/MOP.0000000000000427
- Roduit, C., Frei, R., Ferstl, R., Loeliger, S., Westermann, P., Rhyner, C., Schiavi, E., Barcik, W., Rodriguez-Perez, N., Wawrzyniak, M., Chassard, C., Lacroix, C., Schmausser-Hechfellner, E., Depner, M., von Mutius, E., Braun-Fahrländer, C., Karvonen, A. M., Kirjavainen, P. V., Pekkanen, J., … O'Mahony, L. (2019). High levels of butyrate and propionate in early life are associated with protection against atopy. Allergy, 74(4), 799–809. Epub 2018/11/06. PubMed PMID: 30390309. https://doi.org/10.1111/all.13660
- Samuel, T. M., Binia, A., de Castro, C. A., Thakkar, S. K., Billeaud, C., Agosti, M., Al-Jashi, I., Costeira, M. J., Marchini, G., Martínez-Costa, C., Picaud, J.-C., Stiris, T., Stoicescu, S.-M., Vanpeé, M., Domellöf, M., Austin, S., & Sprenger, N. (2019). Impact of maternal characteristics on human milk oligosaccharide composition over the first 4 months of lactation in a cohort of healthy European mothers. Scientific Reports, 9(1), 11767. Epub 2019/08/15. PubMed PMID: 31409852; PubMed Central PMCID: PMCPMC6692355. https://doi.org/10.1038/s41598-019-48337-4
- Seppo, A. E., Autran, C. A., Bode, L., & Jarvinen, K. M. (2017). Human milk oligosaccharides and development of cow's milk allergy in infants. Journal of Allergy and Clinical Immunology, 139(2), 708–711.e5. PubMed PMID: 27702672; PubMed Central PMCID: PMCPMC5303147. https://doi.org/10.1016/j.jaci.2016.08.031
- Sigurdardottir, S. T., Jonasson, K., Clausen, M., Lilja Bjornsdottir, K., Sigurdardottir, S. E., Roberts, G., Grimshaw, K., Papadopoulos, N. G., Xepapadaki, P., Fiandor, A., Quirce, S., Sprikkelman, A. B., Hulshof, L., Kowalski, M. L., Kurowski, M., Dubakiene, R., Rudzeviciene, O., Bellach, J., Yürek, S., … Keil, T. (2021). Prevalence and early-life risk factors of school-age allergic multimorbidity: The EuroPrevall-iFAAM birth cohort. Allergy, 76(9), 2855–2865. Epub 2021/05/03. PubMed PMID: 33934363; PMCID: PMCPMC8453757. https://doi.org/10.1111/all.14857
- Siziba, L. P., Mank, M., Stahl, B., Kurz, D., Gonsalves, J., Blijenberg, B., Rothenbacher, D., & Genuneit, J. (2022). Human milk oligosaccharide profiles and child atopic dermatitis up to 2 years of age: The Ulm SPATZ health study. Pediatric Allergy and Immunology, 33(2), e13740. https://doi.org/10.1111/pai.13740
- Sjogren, Y. M., Duchen, K., Lindh, F., Bjorksten, B., & Sverremark-Ekstrom, E. (2007). Neutral oligosaccharides in colostrum in relation to maternal allergy and allergy development in children up to 18 months of age. Pediatric Allergy and Immunology, 18(1), 20–26. Epub 2007/02/14. PubMed PMID: 17295795. https://doi.org/10.1111/j.1399-3038.2006.00486.x
- Smilowitz, J. T., Lebrilla, C. B., Mills, D. A., German, J. B., & Freeman, S. L. (2014). Breast milk oligosaccharides: Structure-function relationships in the neonate. Annual Review of Nutrition, 34(1), 143–169. Epub 2014/05/23. PubMed PMID: 24850388; PubMed Central PMCID: PMCPMC4348064. https://doi.org/10.1146/annurev-nutr-071813-105721
- Sprenger, N., Odenwald, H., Kukkonen, A. K., Kuitunen, M., Savilahti, E., & Kunz, C. (2017). FUT2-dependent breast milk oligosaccharides and allergy at 2 and 5 years of age in infants with high hereditary allergy risk. European Journal of Nutrition, 56(3), 1293–1301. Epub 2016/02/26. PubMed PMID: 26907090. https://doi.org/10.1007/s00394-016-1180-6
- Tan, J., McKenzie, C., Vuillermin, P. J., Goverse, G., Vinuesa, C. G., Mebius, R. E., Macia, L., & Mackay, C. R. (2016). Dietary fiber and bacterial SCFA enhance oral tolerance and protect against food allergy through diverse cellular pathways. Cell Reports, 15(12), 2809–2824. Epub 2016/06/23. PubMed PMID: 27332875. https://doi.org/10.1016/j.celrep.2016.05.047
- Trompette, A., Gollwitzer, E. S., Yadava, K., Sichelstiel, A. K., Sprenger, N., Ngom-Bru, C., Blanchard, C., Junt, T., Nicod, L. P., Harris, N. L., & Marsland, B. J. (2014). Gut microbiota metabolism of dietary fiber influences allergic airway disease and hematopoiesis. Nature Medicine, 20(2), 159–166. Epub 2014/01/07. PubMed PMID: 24390308. https://doi.org/10.1038/nm.3444
- Wesemann, D. R., & Nagler, C. R. (2016). The microbiome, timing, and barrier function in the context of allergic disease. Immunity, 44(4), 728–738. Epub 2016/04/21. PubMed PMID: 27096316; PubMed Central PMCID: PMCPMC4869964. https://doi.org/10.1016/j.immuni.2016.02.002
- Zuercher, A. W., Weiss, M., Holvoet, S., Moser, M., Moussu, H., van Overtvelt, L., Horiot, S., Moingeon, P., Nutten, S., Prioult, G., Singh, A., & Mercenier, A. (2012). Lactococcus lactis NCC 2287 alleviates food allergic manifestations in sensitized mice by reducing IL-13 expression specifically in the ileum. Clinical and Developmental Immunology, 2012, 1–10. Epub 2011/10/01. PubMed PMID: 21961022; PubMed Central PMCID: PMCPMC3179883. https://doi.org/10.1155/2012/485750