ABSTRACT
This study aimed to elucidate the causal genetic relationships between iron, copper, zinc, magnesium, and rheumatoid arthritis (RA). A two-sample Mendelian randomization (MR) analysis was conducted using the “TwoSampleMR” and “MendelianRandomization” packages in R. The random-effects inverse variance-weighted (IVW) method was used as the primary approach. We performed sensitivity analyses to test the reliability of the results. The random-effects IVW analysis revealed that there was no genetic causal relationship between iron (P = 0.429, odds ratio [OR] 95% confidence interval [CI] = 0.919 [0.746–1.133]), copper (P = 0.313, OR 95% CI = 0.973 [0.921–1.027]), zinc (P = 0.633, OR 95% CI = 0.978 [0.891–1.073]), or magnesium (P = 0.218, OR 95% CI = 0.792 [0.546–1.148]) and RA. Sensitivity analysis verified the reliability of the results. Therefore, there is no evidence to support a causal relationship between iron, copper, zinc, and magnesium intake at the genetic level and the development of RA.
Introduction
Rheumatoid arthritis (RA) is a complex autoimmune and systemic inflammatory disease characterized by synovial inflammation, and is the most prevalent form of inflammatory arthritis. RA affects both synovial cells and chondrocytes in the joints, leading to synovial inflammation, cartilage injury, and bone erosion. RA is characterized by the formation of locally infiltrating synovial tissue that changes as the disease progresses. This condition is predominantly characterized by chronic, symmetrical, multisynovial arthritis and extra-articular lesions, often affecting small joints, such as those found in the hands, wrists, and feet, with recurring and symmetrical symptoms (Sparks Citation2019). Common clinical symptoms include skeletal muscle pain and joint swelling and stiffness, which significantly impairs patients’ function and reduces their quality of life (Kumar et al. Citation2016). The incidence of RA is approximately 0.5–1%, with a male-to-female ratio of 2.5/1. Although the disease can occur at any age, it usually affects individuals between the ages of 40–70 years, and its incidence increases with age. Approximately 40% of patients with RA become disabled after 10 years (Lee and Weinblatt Citation2001). The etiology of RA is not completely understood; however, genetic factors account for 60% of the risk. Other important risk factors include sex and smoking status. RA can progress to advanced stages, leading to joint destruction and eventual disability, with a significant societal impact. Currently, there is no definitive and effective radical treatment strategy for RA, and clinical management primarily aims to alleviate the symptoms. Further extensive and in-depth research is required to promote the prevention, clinical diagnosis, and treatment of RA.
Micronutrients are crucial for maintaining homeostasis in the body and are intricately linked to numerous diseases, including RA. Iron, a vital component of hemoglobin and myoglobin, facilitates oxygen transport, storage, and cellular utilization. Additionally, iron plays a pivotal role in cytochrome release within the mitochondria, mediating electron transfer in the electron transport chain (McDermid and Lonnerdal Citation2012). Iron homeostasis is intricately regulated by several serum proteins, cellular receptors, hormones, and other factors (Hentze et al. Citation2010). It is also associated with various diseases, immune regulation, and inflammatory response (Hentze et al. Citation2004; Ganz and Nemeth Citation2012). Copper is a causative agent of several diseases, and copper-lowering therapies have promoted the advancement of medical research in the areas of anti-angiogenesis, anti-fibrotic, and anti-inflammatory therapies (Brewer Citation2003). Adequate copper intake is essential for the normal utilization of dietary iron, because copper-dependent processes, such as intestinal iron absorption, iron release from storage, and iron incorporation into hemoglobin are essential for proper iron metabolism (Collins and Klevay Citation2011). Zinc is a vital micronutrient for nucleic acid metabolism, cell replication, tissue repair, and growth, and its deficiency is associated with a wide range of pathological conditions (Murakami and Hirano Citation2008). Zinc plays a pivotal role in physiological processes, including bone homeostasis, as most of the body’s zinc is stored in the bones. Zinc is not only a component of bone but also an important cofactor for many proteins involved in microstructural stability and bone remodeling (Huang et al. Citation2020). Magnesium plays a crucial role in the physiological functions of the human body by affecting oxygen uptake, energy production, and electrolyte balance (Geiger and Wanner Citation2012). An adequate dietary magnesium intake is closely associated with the maintenance of normal bone homeostasis (Rondanelli et al. Citation2021). These essential micronutrients are closely associated with RA and may affect diseases such as RA by causing programmed cell death, such as ferroptosis and cuproptosis (Zhao et al. Citation2022; T.; Zhao et al. Citation2022). Further research is needed to understand the specific role of these micronutrients in RA pathogenesis and to advance the progress of RA research.
The mechanism of RA pathogenesis remains unknown; however, genetic susceptibility and environmental factors plays an important role in RA. Although the risk factors for RA have not been fully established, various studies have focused on the role of micronutrients in RA. Several micronutrients are associated with RA. The most common micronutrients are iron, copper, zinc, and magnesium, and numerous studies have investigated the roles of these four micronutrients in RA. A study involving 102 patients with RA and 66 healthy controls found that patients with RA had significantly elevated blood copper levels, whereas iron and zinc levels were reduced. In addition, circulating blood copper levels in patients were positively correlated with DAS28-CRP and inflammatory markers, while circulating blood iron levels are negatively correlated with DAS28-CRP and inflammatory markers (Wang et al. Citation2023). A study on a Pakistani population also showed that patients with RA had elevated serum copper concentrations and significantly lower serum iron and zinc levels than healthy controls. Micronutrient-related studies have shown negative correlations between copper and zinc levels in patients with RA, but not in the control groups (Ullah et al. Citation2016). RA aggravates iron redistribution, reduces iron levels in the blood, increases iron levels in the synovium, and exacerbates local inflammation. The decrease in serum zinc levels in patients with RA may be due to increased zinc input into the cells in response to pro-inflammatory cytokine exposure. Therefore, zinc deficiency increases the release of proinflammatory mediators and reactive oxygen species (ROS) through epigenetic mechanisms, further promoting inflammation (Shao et al. Citation2023). Another study involving 110 patients with RA and 100 healthy controls also identified high serum copper and low zinc levels in patients with RA. Serum copper concentration positively correlated with disease activity based on the disease activity score. Low serum zinc and high serum copper concentrations may be associated with the presence of RA or a consequence of RA (Sahebari et al. Citation2016). A systematic review and meta-analysis also consistently concluded that serum zinc levels in patients with RA were significantly lower than those in healthy controls, while serum copper levels were significantly higher, suggesting that zinc and copper may play a role in the pathogenesis of RA (Ma et al. Citation2019). Magnesium reduces levels of tumor necrosis factor (TNF)-α, interleukin (IL)-1β, IL-6, and other inflammatory mediators, and prevents the activation of nuclear factor κB (NF-κB), suggesting that magnesium primarily inhibits innate immune responses. TNF-α, IL-1β, IL-6 and NF-κB play important roles in the pathogenesis and joint injury of RA. Patients with RA have reduced serum magnesium levels, which has short-term anti-inflammatory properties, and a short-term diet low in magnesium can reduce the severity of RA and reduce gene expression in the synovial tissue (Brenner et al. Citation2017). In addition, magnesium is relevant to bone health because it affects osteoblast and osteoclast functions and calcium homeostasis by regulating the concentrations of parathyroid hormones and calcitriol (Nieves Citation2014). Although a variety of micronutrients have been associated with RA, current research has focused mainly on the role of four micronutrients – iron, copper, zinc, and magnesium – in RA pathogenesis. This illustrates the importance of these micronutrients in the pathogenesis of RA. Previous studies have found that serum copper levels are positively correlated with inflammatory markers of RA, whereas serum iron levels are negatively correlated (Wang et al. Citation2023). Serum copper levels were positively correlated with RA disease activity, suggesting that an increase in serum copper levels may be a risk factor for RA (Sahebari et al. Citation2016). No positive or negative correlations were found between serum iron, zinc, or magnesium levels and disease activity in patients with RA. Therefore, it is important to investigate the causal relationship between these four micronutrients and RA at the genetic level.
Mendelian randomization (MR) is an analytical method that uses single nucleotide polymorphisms (SNPs) as instrumental variables (IVs) to investigate the causal relationship between exposure and outcomes. MR analysis has been shown to have significant potential in the study of causality between diseases and traits (Zhuo et al. Citation2021). By mitigating confounding factors and reverse causality, MR studies can bolster causal inferences between exposure and outcome (Zhang Citation2022). Previous studies employed MR analysis to investigate the causal effects of iron and copper on lipid metabolic diseases and the relationship between zinc, copper, and ovarian cancer risk (Zhou et al. Citation2020; Lin and Yang Citation2021). The objective of this study was to employ MR analysis to examine genetic causality between iron, copper, zinc, magnesium, and RA.
Materials and methods
Data source
This study utilized summary data from genome-wide association studies (GWAS) on exposure to iron, copper, zinc, and magnesium obtained from the IEU OpenGWAS database (https://gwas.mrcieu.ac.uk/). The iron GWAS summary data included 23,986 samples and 2,096,457 SNPs, which were subjected to genotypic implantation and quality control procedures. Genotyping was performed, and the association between the imported SNPs and each iron phenotype was evaluated using an additive model of allelic effects. Phenotypic standardized residuals were adjusted for sex after controlling for age, principal component scores, and other study-specific covariates (Benyamin et al. Citation2014). The copper and zinc GWAS summary data included 2,603 samples and 2,543,646 and 2,543,610 SNPs, respectively. Imputation was performed using the MACH Markov Chain Haplotyping software with CEU individuals from Phase 2 of the HapMap project as a reference set. The most significant SNPs at each site were included as covariates to detect independent effects at significant sites, and the results were combined for meta-analysis (Evans et al. Citation2013). The magnesium GWAS summary data included 64,979 samples and 9,851,867 SNPs. In addition, outcome data on RA were obtained from the Finnish consortium (https://www.finngen.fi/), which included 153,457 samples and 16,380,169 SNPs. All cases were defined using the M13 code of the International Classification of Diseases-Tenth Revision (ICD-10). Genotyping was performed using Illumina (Illumina Inc, San Diego) and Affymetrix chip arrays (Thermo Fisher Scientific, Santa Clara, CA, USA), and detailed information on the participants, genotyping, imputation, and quality control can be found on the FinnGen website. All participants in both the exposure and outcome datasets were of European descent and included both males and females. As the data analyzed in this study have been published publicly, ethical approval and informed consent were not required. The data used in this study are presented in Table S1.
IVs selection
The quality of an MR analysis relies on the satisfaction of three fundamental assumptions: (1) a strong association between the IVs and exposure; (2) no association between the IVs and the outcome or confounding factors; and (3) the IVs only affect the outcome through exposure and not via other routes. To ensure the robustness of the MR analysis results, a series of stringent quality control measures were applied for the IV selection. First, the SNPs used as IVs were required to be strongly associated with exposure (P < 5 × 10−6 and F statistic > 10) (Ni et al. Citation2021), where the F statistic was calculated using the following formula: F = R2(N-K-1)/K(1-R2) (Dan et al. Citation2021). Second, to address the issue of strong linkage disequilibrium (LD) among selected SNPs, SNPs with LD were removed and only those without LD (defined by r2 <0.001 and clump distance > 10,000 kb) were retained as IVs (Chen et al. Citation2022). Thirdly, SNPs associated with the outcome (P < 5 × 10−6) were excluded. Fourth, confounding factors potentially influencing the MR analysis, such as smoking, obesity, and sex, were removed from the PhenoScanner database (http://www.phenoscanner.medschl.cam.ac.uk/phenoscanner) (Meisinger et al. Citation2020; Petrovska et al. Citation2021). Palindromic SNPs with intermediate allele frequencies were excluded (Gill et al. Citation2021).
Statistical analysis
This study employed the “TwoSampleMR” and “MendelianRandomization” packages in R (version 4.1.2) to conduct a two-sample MR analysis examining the potential causal association between the exposure and outcome of interest. Various MR analysis techniques were employed to assess this relationship, including the random-effects inverse variance weighted (IVW) method as the primary analysis method and the MR-Egger, weighted median, simple mode, and weighted mode as complementary methods. The IVW method is a classical MR statistical method that assumes that all the included SNPs are valid IVs. Wald ratios were calculated for each SNP to evaluate its impact on the results, and the inverse variance of SNPs was used as the weight of the meta-analysis to assess the causal relationship between exposure and outcomes (Cai et al. Citation2021). The MR-Egger method can provide relatively robust estimates even when IVs are weak instruments (Qu et al. Citation2021). The weighted median method can generate robust results when more than 50% of the weight is derived from invalid IVs (Bowden et al. Citation2016). This simple model is a model-based estimation method that provides robustness against pleiotropy (Li et al. Citation2022). When estimates from the most similar individuals are based on valid IVs, the weighted model can obtain reliable causal estimates (Cao et al. Citation2022). Nonetheless, compared with the IVW method, the power of the MR-Egger, weighted median, simple mode, and weighted mode was lower. Consequently, the findings of MR analysis between exposure and outcome primarily referred to the IVW method (Cao et al. Citation2022).
A battery of tests was conducted to assess the robustness of the MR analysis results. First, Cochran’s Q statistic was used for MR-IVW and Rucker’s Q statistic was used for MR-Egger to detect heterogeneity, where a P > 0.05 was considered indicative no heterogeneity (Hemani et al. Citation2018). Second, the intercept test of the MR Egger and the MR pleiotropy residual sum and outlier (MR-PRESSO) were utilized to assess horizontal pleiotropy, where a P > 0.05 was considered indicative of no horizontal pleiotropy (Yang et al. Citation2023). Third, MR-PRESSO was used to identify outliers in the MR analysis (Meng et al. Citation2022). Fourth, the “Leave-one-out” analysis was performed to investigate whether a single SNP influenced the genetic causality assessment between the exposure and outcome (Huang et al. Citation2021). Fifth, the MR robust adjusted profile score (MR-RAPS) method was applied to evaluate whether the MR analysis of exposures and outcome conformed to the normal distribution, where a P > 0.05 indicated conformity to the normal distribution (Zhang Citation2022). Finally, maximum likelihood, penalized weighted median, and fixed effects (IVW) were used as validation methods to confirm the reliability of the genetic causal assessment between exposures and outcomes (Yang et al. Citation2023).
Results
IVs selection
We identified SNPs that exhibited a strong association with exposure to iron, copper, zinc, and magnesium, and subsequently removed any LD. Specifically, we identified 11 iron-associated SNPs, six copper-associated SNPs, eight zinc-associated SNPs, and 19 magnesium-associated SNPs, all of which demonstrated synergistic effects with the outcome. All SNPs were determined to be independent of both outcome and potential confounding factors. Subsequently, we used 11 iron-associated SNPs as IVs to perform a genetic causality assessment between iron intake and RA, one of these SNPs (rs7209063) was identified as a palindrome (Table S2). Similarly, we used six copper-associated SNPs as IVs to perform a genetic causality assessment between copper and RA without identifying any palindromic SNPs (Table S3). We also used eight zinc-associated SNPs as IVs to perform genetic causality assessments between zinc and RA and identified one palindromic SNP (rs10931753) (Table S4). We further used 19 magnesium-associated SNPs as IVs to perform genetic causality assessments between magnesium and RA without identifying any palindromic SNPs (Table D5).
MR analysis
The random-effects IVW analysis demonstrated that iron (P = 0.429, odds ratio [OR] 95% confidence interval [CI] = 0.919 [0.746–1.133]), copper (P = 0.313, OR 95% CI = 0.973 [0.921–1.027]), zinc (P = 0.633, OR 95% CI = 0.978 [0.891–1.073]), and magnesium (P = 0.218, OR 95% CI = 0.792 [0.546–1.148]) do not possess any genetic causal relationship with RA. Furthermore, the results obtained from the MR-Egger, weighted median, simple mode, and weighted mode analyses support the conclusion that iron, copper, zinc, and magnesium are not genetically causative of RA (P > 0.05) ().
Figure 1. MR analysis results of exposures (iron, copper, zinc, and magnesium) and outcome (rheumatoid arthritis). Five methods: random-effects IVW, MR Egger, weighted median, simple mode, and weighted mode.
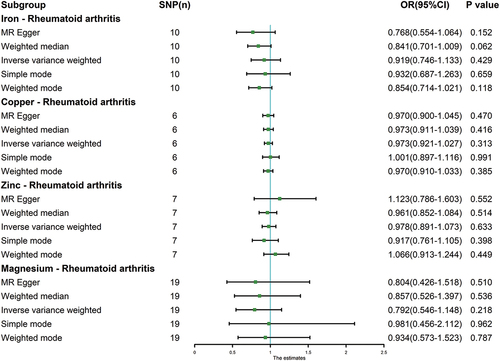
Figure 2. Scatter plot. a: iron and rheumatoid arthritis; b: copper and rheumatoid arthritis; c: zinc and rheumatoid arthritis; d: magnesium and rheumatoid arthritis.
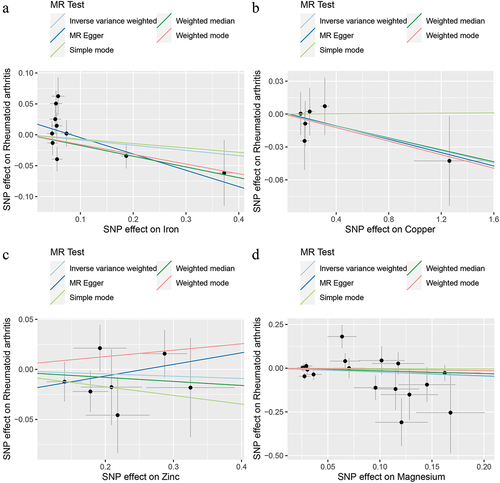
Genetic causality assessment between iron and RA, as determined using Cochran’s Q statistic of MR-IVW, demonstrated significant heterogeneity (P < 0.05). However, the Rucker’s Q statistic of the MR-Egger indicated no heterogeneity (P > 0.05). Conversely, for copper, zinc, and magnesium, the Cochran’s Q statistic for MR-IVW and Rucker’s Q statistic for MR-Egger indicated no heterogeneity (P > 0.05). Furthermore, both the intercept tests of the MR Egger and MR-PRESSO revealed no horizontal pleiotropy in the genetic causality assessment between iron, copper, zinc, magnesium, and RA (P > 0.05). MR-PRESSO also indicated no outliers in this relationship (). Additionally, the “Leave one out” analysis demonstrated that a single SNP did not significantly affect the genetic causality assessment between iron, copper, zinc, magnesium, and RA (). MR-RAPS analysis demonstrated that the genetic causality between these variables was normally distributed (P > 0.05) ( , ). However, no P-value was given for the MR-RAPS analysis of copper and RA, as the number of IVs was only six, and MR-RAPS could only provide a P-value for seven or more IVs. Finally, the results of the maximum likelihood, penalized weighted median, and IVW (fixed effects) analyses also supported the conclusion that iron, copper, zinc, and magnesium had no causal genetic relationship with RA (P > 0.05; ).
Figure 3. Leave one out analysis. a: iron and rheumatoid arthritis; b: copper and rheumatoid arthritis; c: zinc and rheumatoid arthritis; d: magnesium and rheumatoid arthritis.
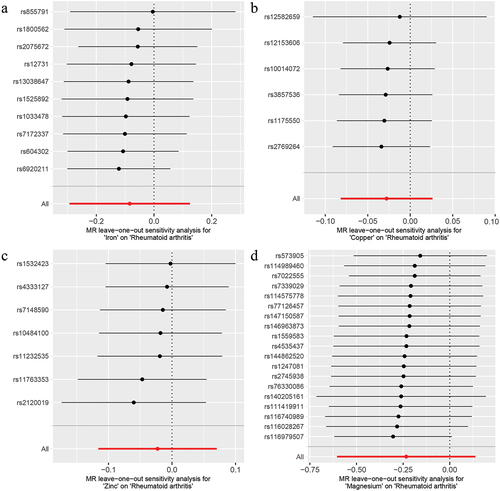
Figure 4. The normal distribution plot of MR analysis. a: iron and rheumatoid arthritis; b: copper and rheumatoid arthritis; c: zinc and rheumatoid arthritis; d: magnesium and rheumatoid arthritis.
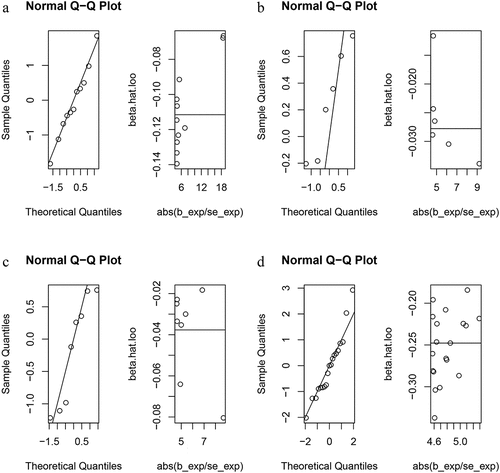
Figure 5. MR analysis results of exposures (iron, copper, zinc, and magnesium) and outcome (rheumatoid arthritis). Three methods: maximum likelihood, penalised weighted median, and IVW (fixed effects).
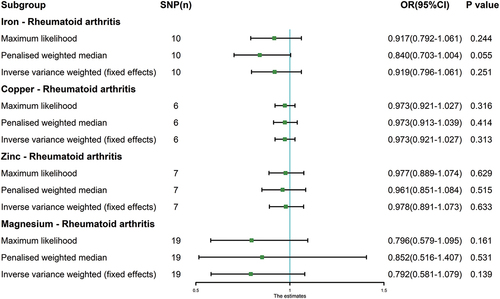
Table 1. Sensitivity analysis of the MR analysis results of exposure and outcome.
Discussion
This study used MR to investigate the causal relationships between iron, copper, zinc, magnesium, and RA. The MR analysis showed that there was no causal relationship between these nutrients and RA at the genetic level, suggesting that any correlation observed in previous studies may be due to factors other than genetics. MR is a relatively new approach based on Mendel’s laws of inheritance, which uses genetic susceptibility to investigate causal relationships between different traits. This method has the advantage of avoiding the bias that can occur in observational studies and is considered a reliable way to assess causality between risk factors and clinical disorders (Meng et al. Citation2022). Additionally, MR can overcome the common limitations of traditional studies, such as confounding factors and reverse causality (Huang et al. Citation2021). Therefore, the results of this study are relatively reliable because they were obtained using MR, which is a robust approach for investigating causal relationships.
Iron deficiency can occur in patients with normal hemoglobin levels, in addition to anemia, due to factors such as insufficient dietary iron intake, gastrointestinal iron absorption disorders, and iron loss from bleeding. This can result in absolute iron deficiency, wherein the body lacks adequate iron reserves. Insufficient iron availability during cellular processes can lead to functional iron deficiency, which is often associated with inflammation (Tański et al. Citation2021). Anemia is a common complication in patients with RA and its occurrence in such patients may be linked to both inflammation and iron deficiency. Iron plays a direct role in the inflammatory response by promoting the production of proinflammatory cytokines by T cells. In patients with RA, serum iron levels have been found to be significantly lower and negatively correlated with disease activity, while iron deposits have been observed in the synovial fluid (Khalaf et al. Citation2019; Wang et al. Citation2023). Additionally, high levels of iron in the synovial membrane and synovial fluid of RA patients, along with chronic inflammatory responses, can catalyze the formation of hydroxyl radicals, leading to irreversible cartilage damage (Ahmadzadeh et al. Citation1989). Local deposition of iron caused by the overexpression of hepcidin, a major regulator of iron metabolism and homeostasis, can also contribute to inflammatory anemia in patients with RA (Sato et al. Citation2020). Moreover, iron plays a key regulatory role in ferroptosis, a newly discovered form of cell-regulated death implicated in RA and other autoimmune and inflammatory diseases. Ferroptosis activators, such as RSL3, can exacerbate synovitis, whereas the ferroptosis inducer, erastin, has been found to reduce ferritin heavy chain 1(FHC1) expression in the synovium and fibroblast-like synoviocytes (FLS) in patients with RA, suggesting that iron metabolism is involved in the ferroptosis of RA-FLS (Luo and Zhang Citation2021; Ling et al. Citation2022; Long et al. Citation2022).
Copper, an essential trace element, is closely associated with the immune system through its presence in human binding proteins, including ceruloplasmin and superoxide dismutase. These proteins play vital roles in maintaining immune system homeostasis and antioxidant activity (Chakraborty et al. Citation2015). Copper produced by liver cells stimulates the pro-inflammatory factor IL; thus, inflammation can increase the copper concentration. RA patients have higher copper levels, and serum copper levels are significantly positively correlated with morning stiffness (Chakraborty et al. Citation2015). Therefore, serum copper levels are considered potential biomarkers of disease activity in patients with RA. The copper-chelating agent, penicillamine, has a therapeutic effect on RA by inhibiting the growth of various cell types by chelating copper ions (Harada et al. Citation2002). Copper-mediated cuproptosis has also been implicated in the development of RA. The breakdown of mitochondrial respiration leads to cuproptosis, mainly due to the direct binding of excess copper to the fatty acylated component of the tricarboxylic acid (TCA) cycle, resulting in the aggregation of lipid-acylated-related proteins, loss of iron-sulfur cluster proteins, and ultimately cell death due to intracellular protein toxic stress (Tsvetkov et al. Citation2022). The molecular and immunological characteristics of cuproptosis in RA have been studied extensively (Zhao et al. Citation2022; Zhou et al. Citation2023). Similarly, zinc is closely related to immunity and plays a vital role in the immune system by influencing innate and adaptive immune cells. Endogenous zinc levels can affect the number and function of immune cells, including macrophages, T cells, and B cells, which are essential for the immune response against RA (Wang et al. Citation2023). Activation of these immune cells leads to the release of pro-inflammatory cytokines, including IL-1, IL-6, and TNF, causing inflammation in RA and further joint injury (Gao et al. Citation2018). Low levels of zinc have been found in patients with RA, which may be related to high copper concentrations in these patients. Copper and zinc have an antagonistic relationship: excess zinc may decrease copper absorption, whereas high doses of copper may decrease zinc absorption (Onal et al. Citation2011; Sahebari et al. Citation2016). However, some studies have found no difference in serum or plasma copper content in patients with RA compared to control groups or even lower levels, whereas serum or plasma zinc levels remained normal or higher (Mierzecki et al. Citation2011; Li et al. Citation2014). Therefore, the effects of copper and zinc levels on RA remains unclear.
Magnesium is an essential micronutrient that serves as a major intracellular ion and cofactor in many enzymatic reactions involved in post-receptor signaling. It plays key roles in energy metabolism, fatty acid synthesis, and glucose utilization (Barbagallo et al. Citation2003). In chronic inflammatory diseases, including RA, oral magnesium has been shown to improve insulin sensitivity in non-diabetic patients with low magnesium levels. Magnesium also plays a crucial regulatory role in the activation of NF-κB, production of pro-inflammatory cytokines, and systemic inflammation, and is closely related to the pathogenesis of RA (Sugimoto et al. Citation2012; Brenner et al. Citation2017). Furthermore, magnesium levels are frequently reduced in patients with RA, and magnesium supplementation has been shown to reduce fasting blood sugar (FBS), insulin, and Homeostasis Model Assessment of Insulin Resistance (HOMA-IR) in these patients. This suggests that magnesium supplementation may be an alternative method for prevent type 2 diabetes in patients with RA (Norouzi et al. Citation2022). Studies have also demonstrated that decreased magnesium levels, dyslipidemia, and elevated uric acid levels may be risk factors for cardiovascular disease in patients with newly diagnosed RA (Chavan et al. Citation2015). A cross-sectional study in women in the United States showed a U-shaped relationship between dietary magnesium intake and RA, with moderate dietary magnesium intake having a potential protective effect in women with RA (Hu et al. Citation2020).
Previous studies have established a close correlation between essential nutrients and RA. However, this study negated the causal relationship between them at the genetic level, thereby presenting a novel perspective for investigating the correlation between essential nutrients and RA. However, some limitations of this study should be acknowledged. First, the sample was restricted to individuals of European ancestry. Given the close association between genetic research and ethnicity, these findings must be approached with caution when extrapolating to other populations. Second, certain diseases can cause changes in micronutrient levels in the body, which may have affected the results of this study. However, MR analysis is a direct causal assessment between exposure and outcome, independent of other factors; therefore, certain diseases that theoretically cause changes in micronutrient levels in the body should not have a large impact on the results of MR analysis. Third, at genome-wide significance levels of 5 × 10−8, we did not obtain sufficient SNPs for MR analysis. Therefore, we expanded the genome-wide significance level to 5 × 10−6. Many previous studies have also set the genome-wide significance level at 5 × 10−6, or even 1 × 10−5 (Ni et al. Citation2021). Therefore, it is reasonable for us to set the significance level to 5 × 10−6. However, this is still a limitation of this study that may make our level of genome-wide significance not particularly high, but which would not have affected our results in nature due to MR’s powerful causal assessment ability.
Conclusion
Based on the findings of this study, it can be concluded that there is no discernible causal association between the genetic levels of iron, copper, zinc, and magnesium and RA. However, it is possible that these elements exhibit correlations at levels other than genetics. Consequently, further research and analyses are necessary to fully elucidate the nature of the relationship between these factors.
List of abbreviations
RA | = | rheumatoid arthritis |
MR | = | Mendelian randomization |
SNPs | = | single nucleotide polymorphisms |
IVs | = | instrumental variables |
GWAS | = | genome-wide association studies |
LD | = | linkage disequilibrium |
IVW | = | inverse variance weighted |
MR-PRESSO | = | MR pleiotropy residual sum and outlier |
MR-RAPS | = | MR robust adjusted profile score |
OR | = | odds ratio |
CI | = | confidence interval |
FHC1 | = | ferritin heavy chain 1 |
FLS | = | fibroblast-like synoviocytes |
IL | = | interleukin |
TCA | = | tricarboxylic acid |
TNF | = | tumor necrosis factor |
NF-Κb | = | nuclear factor κB |
FBS | = | fasting blood sugar |
HOMA-IR | = | Homeostasis Model Assessment of Insulin Resistance. |
Authors’ contributions
The study was designed by Peng Xu and Mingyi Yang. Mingyi Yang, Yani Su, and Ke Xu conducted dataset analysis and interpreted the findings. Mingyi Yang, Xianjie Wan and Jiale Xie were responsible for data download. Lin Liu and Zhi Yang provided software support. The manuscript was written and edited by Mingyi Yang, with support from Peng Xu.
Consent for publication
All authors agree to publish the manuscript in this journal.
Supplemental Material
Download MS Word (28.5 KB)Disclosure statement
No potential conflict of interest was reported by the authors.
Supplementary material
Supplemental data for this article can be accessed online at https://doi.org/10.1080/09603123.2023.2274377
Data availability statement
This study analyzed publicly available datasets that can be accessed via the FinnGen consortium (https://www.finngen.fi/) and the IEU OpenGWAS database (https://gwas.mrcieu.ac.uk/).
Additional information
Funding
References
- Ahmadzadeh N, Shingu M, Nobunaga M. 1989. Iron-binding proteins and free iron in synovial fluids of rheumatoid arthritis patients. Clin Rheumatol. 8(3):345–351. doi:10.1007/BF02030347.
- Barbagallo M, Dominguez LJ, Galioto A, Ferlisi A, Cani C, Malfa L, Pineo A, Busardo A, Paolisso G. 2003. Role of magnesium in insulin action, diabetes and cardio-metabolic syndrome X. Mol Aspects Med. 24(1–3):39–52. doi:10.1016/s0098-2997(02)00090-0.
- Benyamin B, Esko T, Ried JS, Radhakrishnan A, Vermeulen SH, Traglia M, Gogele M, Anderson D, Broer L, Podmore C, et al. 2014 Oct 29. Novel loci affecting iron homeostasis and their effects in individuals at risk for hemochromatosis. Nat Commun. 5:4926. doi:10.1038/ncomms5926.
- Bowden J, Davey Smith G, Haycock PC, Burgess S. 2016. Consistent estimation in Mendelian randomization with some invalid instruments using a weighted median Estimator. Genet Epidemiol. 40(4):304–314. May. doi:10.1002/gepi.21965.
- Brenner M, Laragione T, Gulko PS. Short-term low-magnesium diet reduces autoimmune arthritis severity and synovial tissue gene expression. Physiol Genomics. 2017 Apr 1 49(4):238–242. doi:10.1152/physiolgenomics.00003.2017.
- Brewer GJ. 2003. Copper in medicine. Curr Opin Chem Biol. 7(2):207–212. Apr. doi:10.1016/s1367-5931(03)00018-8.
- Cai Y, Zhang G, Liang J, Jing Z, Zhang R, Lv L, Dang X. 2021. Causal relationships between osteoarthritis and senile central nerve System dysfunction: a bidirectional two-sample Mendelian randomization study. Front Aging Neurosci. 13:793023. doi: 10.3389/fnagi.2021.793023.
- Cao Z, Wu Y, Li Q, Li Y, Wu J. 2022. A causal relationship between childhood obesity and risk of osteoarthritis: results from a two-sample Mendelian randomization analysis. Ann Med. 54(1):1636–1645. Dec. doi:10.1080/07853890.2022.2085883.
- Chakraborty M, Chutia H, Changkakati R. 2015. Serum copper as a marker of disease activity in rheumatoid arthritis. J Clin Diagn Res. Dec 9(12):BC09–11. doi:10.7860/JCDR/2015/14851.7001.
- Chavan VU, Ramavataram D, Patel PA, Rupani MP. 2015. Evaluation of serum magnesium, lipid profile and various biochemical parameters as risk factors of cardiovascular diseases in patients with rheumatoid arthritis. J Clin Diagn Res. Apr 9(4):BC01–05. doi:10.7860/JCDR/2015/12206.5740.
- Chen Y, Shen J, Wu Y, Ni M, Deng Y, Sun X, Wang X, Zhang T, Pan F, Tang Z. 2022. Tea consumption and risk of lower respiratory tract infections: a two‑sample Mendelian randomization study. Eur J Nutr. 62(1):1–9. doi:10.1007/s00394-022-02994-w.
- Collins JF, Klevay LM. 2011. Copper. Adv Nutr. Nov 2(6):520–522. doi:10.3945/an.111.001222.
- Dan YL, Wang P, Cheng Z, Wu Q, Wang XR, Wang DG, Pan HF. Circulating adiponectin levels and systemic lupus erythematosus: a two-sample Mendelian randomization study. Rheumatology (Oxford). 2021 Feb 1 60(2):940–946. doi:10.1093/rheumatology/keaa506.
- Evans DM, Zhu G, Dy V, Heath AC, Madden PA, Kemp JP, McMahon G, St Pourcain B, Timpson NJ, Golding J, et al. 2013 Oct 1. Genome-wide association study identifies loci affecting blood copper, selenium and zinc. Hum Mol Genet. 22(19):3998–4006. doi:10.1093/hmg/ddt239.
- Ganz T, Nemeth E. 2012. Hepcidin and iron homeostasis. Biochim Biophys Acta. 1823(9):1434–1443. Sep. doi:10.1016/j.bbamcr.2012.01.014.
- Gao H, Dai W, Zhao L, Min J, Wang F. 2018. The role of zinc and zinc homeostasis in macrophage function. J Immunol Res. 2018:1–11. doi: 10.1155/2018/6872621.
- Geiger H, Wanner C. 2012. Magnesium in disease. Clin Kidney J. 5(Suppl 1):i25–i38. Feb. doi:10.1093/ndtplus/sfr165.
- Gill D, Karhunen V, Malik R, Dichgans M, Sofat N. 2021. Cardiometabolic traits mediating the effect of education on osteoarthritis risk: a Mendelian randomization study. Osteoarthr Cartil. Mar 29(3):365–371. doi:10.1016/j.joca.2020.12.015.
- Harada S, Sugiyama E, Taki H, Shinoda K, Fujita T, Maruyama M, Kobayashi M. 2002 Dec. D-penicillamine cooperates with copper sulfate to enhance the surface expression of functional fas antigen in rheumatoid synovial fibroblasts via the generation of hydrogen peroxide. Clin Exp Rheumatol. 20(4):469–476.
- Hemani G, Zheng J, Elsworth B, Wade KH, Haberland V, Baird D, Laurin C, Burgess S, Bowden J, Langdon R, et al. 2018. The MR-Base platform supports systematic causal inference across the human phenome. Elife. 7. May 30. doi:10.7554/eLife.34408.
- Hentze MW, Muckenthaler MU, Andrews NC. Balancing acts: molecular control of mammalian iron metabolism. Cell. 2004 Apr 30 117(3):285–297. doi:10.1016/s0092-8674(04)00343-5.
- Hentze MW, Muckenthaler MU, Galy B, Camaschella C. Two to tango: regulation of mammalian iron metabolism. Cell. 2010 Jul 9 142(1):24–38. doi:10.1016/j.cell.2010.06.028.
- Huang T, Yan G, Guan M. 2020. Zinc homeostasis in bone: zinc transporters and bone diseases. Int J Mol Sci. 21(4):1236. Feb 12. doi: 10.3390/ijms21041236.
- Huang G, Zhong Y, Li W, Liao W, Wu P. 2021. Causal relationship between parathyroid hormone and the risk of osteoarthritis: a Mendelian randomization study. Front Genet. 12:686939. doi:10.3389/fgene.2021.686939.
- Hu C, Zhu F, Liu L, Zhang M, Chen G. Relationship between dietary magnesium intake and rheumatoid arthritis in US women: a cross-sectional study. BMJ Open. 2020. Nov 9 10(11):e039640. doi:10.1136/bmjopen-2020-039640.
- Khalaf W, Al-Rubaie HA, Shihab S. Studying anemia of chronic disease and iron deficiency in patients with rheumatoid arthritis by iron status and circulating hepcidin. Hematol Rep. 2019 Feb 19 11(1):7708. doi:10.4081/hr.2019.7708.
- Kumar LD, Karthik R, Gayathri N, Sivasudha T. 2016. Advancement in contemporary diagnostic and therapeutic approaches for rheumatoid arthritis. Biomed Pharmacother. 79:52–61. Apr. doi:10.1016/j.biopha.2016.02.001.
- Lee DM, Weinblatt ME. 2001. Rheumatoid arthritis. Lancet. 358(9285):903–911. doi:10.1016/s0140-6736(01)06075-5.
- Li J, Liang Y, Mao H, Deng W, Zhang J. 2014. Effects of B-lymphocyte dysfunction on the serum copper, selenium and zinc levels of rheumatoid arthritis patients. Pak J Med Sci. 30(5):1064–1067. Sep. doi:10.12669/pjms.305.5214.
- Ling H, Li M, Yang C, Sun S, Zhang W, Zhao L, Xu N, Zhang J, Shen Y, Zhang X, et al. 2022. Glycine increased ferroptosis via SAM-mediated GPX4 promoter methylation in rheumatoid arthritis. Rheumatology (Oxford). 61(11):4521–4534. doi:10.1093/rheumatology/keac069.
- Li C, Niu M, Guo Z, Liu P, Zheng Y, Liu D, Yang S, Wang W, Li Y, Hou H. 2022. A mild causal relationship between tea consumption and obesity in general population: a two-sample Mendelian randomization study. Front Genet. 13:795049. doi:10.3389/fgene.2022.795049.
- Lin S, Yang H. 2021. Ovarian cancer risk according to circulating zinc and copper concentrations: a meta-analysis and Mendelian randomization study. Clin Nutr. Apr 40(4):2464–2468. doi:10.1016/j.clnu.2020.10.011.
- Long L, Guo H, Chen X, Liu Y, Wang R, Zheng X, Huang X, Zhou Q, Wang Y. 2022. Advancement in understanding the role of ferroptosis in rheumatoid arthritis. Front Physiol. 13:1036515. doi:10.3389/fphys.2022.1036515.
- Luo H, Zhang R. 2021. Icariin enhances cell survival in lipopolysaccharide-induced synoviocytes by suppressing ferroptosis via the xc-/GPX4 axis. Exp Ther Med. Jan 21(1):72. 10.3892/etm.2020.9504.
- Ma Y, Zhang X, Fan D, Xia Q, Wang M, Pan F. 2019. Common trace metals in rheumatoid arthritis: a systematic review and meta-analysis. J Trace Elements Med Biol. 56:81–89. doi:10.1016/j.jtemb.2019.07.007.
- McDermid JM, Lonnerdal B. 2012. Iron. Adv Nutr. 3(4):532–533. Jul 1. doi: 10.3945/an.112.002261.
- Meisinger C, Linseisen J, Leitzmann M, Baurecht H, Baumeister SE. 2020. Association of physical activity and sedentary behavior with type 2 diabetes and glycemic traits: a two-sample Mendelian randomization study. BMJ Open Diabetes Res Care. Dec 8(2):e001896. doi:10.1136/bmjdrc-2020-001896.
- Meng H, Jiang L, Song Z, Wang F. 2022. Causal associations of circulating lipids with osteoarthritis: a bidirectional Mendelian randomization study. Nutrients. 14(7):1327. Mar 22. doi: 10.3390/nu14071327.
- Mierzecki A, Strecker D, Radomska K. 2011. A pilot study on zinc levels in patients with rheumatoid arthritis. Biol Trace Elem Res. 143(2):854–862. Nov. doi:10.1007/s12011-010-8952-2.
- Murakami M, Hirano T. 2008. Intracellular zinc homeostasis and zinc signaling. Cancer Sci. 99(8):1515–1522. Aug. doi:10.1111/j.1349-7006.2008.00854.x.
- Nieves JW. 2014. Bone. Maximizing bone health–magnesium, BMD and fractures. Nat Rev Endocrinol. May 10(5):255–256. doi:10.1038/nrendo.2014.39.
- Ni JJ, Xu Q, Yan SS, Han BX, Zhang H, Wei XT, Feng GJ, Zhao M, Pei YF, Zhang L. 2021. Gut microbiota and psychiatric disorders: a two-sample Mendelian randomization study. Front Microbiol. 12:737197. doi: 10.3389/fmicb.2021.737197.
- Norouzi M, Rezvankhah B, Haeri MR, Heydari H, Tafaroji J, Shafigh N, Avval JO, Dahmardeh AR, Masoumzadeh N, Gharehbeglou M. 2022. Magnesium supplementation and insulin resistance in patients with rheumatoid arthritis. Eur J Transl Myol. 32(3): Jul 5. doi:10.4081/ejtm.2022.10622.
- Onal S, Naziroglu M, Colak M, Bulut V, Flores-Arce MF. 2011. Effects of different medical treatments on serum copper, selenium and zinc levels in patients with rheumatoid arthritis. Biol Trace Elem Res. 142(3):447–455. Sep. doi:10.1007/s12011-010-8826-7.
- Petrovska N, Prajzlerova K, Vencovsky J, Senolt L, Filkova M. 2021. The pre-clinical phase of rheumatoid arthritis: from risk factors to prevention of arthritis. Autoimmun Rev. 20(5):102797. May. doi:10.1016/j.autrev.2021.102797.
- Qu Z, Yang F, Hong J, Wang W, Li S, Jiang G, Yan S. Causal relationship of serum nutritional factors with osteoarthritis: a Mendelian randomization study. Rheumatology (Oxford). 2021 May 14 60(5):2383–2390. doi:10.1093/rheumatology/keaa622.
- Rondanelli M, Faliva MA, Tartara A, Gasparri C, Perna S, Infantino V, Riva A, Petrangolini G, Peroni G. 2021. An update on magnesium and bone health. Biometals. 34(4):715–736. Aug. doi:10.1007/s10534-021-00305-0.
- Sahebari M, Ayati R, Mirzaei H, Sahebkar A, Hejazi S, Saghafi M, Saadati N, Ferns GA, Ghayour-Mobarhan M. 2016. Serum Trace element concentrations in rheumatoid arthritis. Biol Trace Elem Res. 171(2):237–245. Jun. doi:10.1007/s12011-015-0501-6.
- Sato H, Takai C, Kazama JJ, Wakamatsu A, Hasegawa E, Kobayashi D, Kondo N, Nakatsue T, Abe A, Ito S, et al. 2020. Jun 18. Serum hepcidin level, iron metabolism and osteoporosis in patients with rheumatoid arthritis. Sci Rep. 10(1):9882. doi:10.1038/s41598-020-66945-3.
- Shao Y-R, Xu D-Y, Lin J. 2023. Nutrients and rheumatoid arthritis: from the perspective of neutrophils. Front Immunol. 14. doi:10.3389/fimmu.2023.1113607.
- Sparks JA. Rheumatoid arthritis. Ann Intern Med. 2019. Jan 1 170(1):ITC1–ITC16. doi:10.7326/AITC201901010.
- Sugimoto J, Romani AM, Valentin-Torres AM, Luciano AA, Ramirez Kitchen CM, Funderburg N, Mesiano S, Bernstein HB. Magnesium decreases inflammatory cytokine production: a novel innate immunomodulatory mechanism. J Immunol. 2012 Jun 15 188(12):6338–6346. doi:10.4049/jimmunol.1101765.
- Tański W, Chabowski M, Jankowska-Polańska B, Jankowska EA. 2021. Iron metabolism in patients with rheumatoid arthritis. Eur Rev Med Pharmacol Sci. 25(12):4325–4335. doi:10.26355/eurrev_202106_26140.
- Tsvetkov P, Coy S, Petrova B, Dreishpoon M, Verma A, Abdusamad M, Rossen J, Joesch-Cohen L, Humeidi R, Spangler RD, et al. 2022. Copper induces cell death by targeting lipoylated TCA cycle proteins. Sci. 375(6586):1254–1261. doi:10.1126/science.abf0529.
- Ullah Z, Ullah MI, Hussain S, Kaul H, Lone KP. 2016. Determination of serum Trace elements (Zn, Cu, and Fe) in Pakistani patients with rheumatoid arthritis. Biol Trace Elem Res. 175(1):10–16. doi:10.1007/s12011-016-0746-8.
- Wang H, Zhang R, Shen J, Jin Y, Chang C, Hong M, Guo S, He D. 2023. Circulating level of blood iron and copper associated with inflammation and disease activity of rheumatoid arthritis. Biol Trace Elem Res. 201(1):90–97. Jan. doi:10.1007/s12011-022-03148-z.
- Yang M, Wan X, Zheng H, Xu K, Xie J, Yu H, Wang J, Xu P. 2023. No Evidence of a genetic causal relationship between ankylosing spondylitis and gut microbiota: A two-sample Mendelian randomization study. Nutrients. 15(4):1057. Feb 20. doi: 10.3390/nu15041057.
- Yang M, Xu J, Zhang F, Luo P, Xu K, Feng R, Xu P. 2023. Large-scale genetic correlation analysis between spondyloarthritis and human blood metabolites. J Clin Med. 12(3):1201. Feb 2. doi: 10.3390/jcm12031201.
- Zhang J. 2022. Mendelian randomization study implies causal linkage between telomere length and juvenile idiopathic arthritis in a European population. J Inflamm Res. 15:977–986. doi:10.2147/JIR.S354619.
- Zhao J, Guo S, Schrodi SJ, He D. 2022. Cuproptosis and cuproptosis-related genes in rheumatoid arthritis: implication, prospects, and perspectives. Front Immunol. 13:930278. doi: 10.3389/fimmu.2022.930278.
- Zhou Y, Li X, Ng L, Zhao Q, Guo W, Hu J, Zhong J, Su W, Liu C, Su S. 2023. Identification of copper death-associated molecular clusters and immunological profiles in rheumatoid arthritis. Front Immunol. 14:1103509. doi:10.3389/fimmu.2023.1103509.
- Zhou J, Liu C, Francis M, Sun Y, Ryu MS, Grider A, Ye K. 2020. The causal effects of blood iron and copper on lipid metabolism diseases: evidence from phenome-wide Mendelian randomization study. Nutrients. 12(10):3174. Oct 17. doi: 10.3390/nu12103174.
- Zhuo C, Zhao J, Chen M, Lu Y. 2021. Physical activity and risks of cardiovascular diseases: a Mendelian randomization study. Front Cardiovasc Med. 8:722154. doi: 10.3389/fcvm.2021.722154.