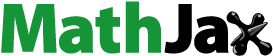
ABSTRACT
Many technological applications (apps) purport to help children learn academic material. Building on research in developmental and educational psychology, we developed and tested an app to teach biological and physical science content to preschool children. There were 21 children in the control condition (Mage = 50.30 months, SD = 8.61) and 21 children in the intervention condition (Mage = 53.21 months old, SD = 6.36). Children were given pre- assessments and post-assessments of their understanding of living things, inheritance, buoyancy, and balance. Half were assigned to play the app for 3 weeks or to a control condition that only completed the assessments. Based on ANCOVA and ANOVA models, children in the app condition increased in their understanding of living things and buoyancy on a near-transfer task from pre-test to post-test assessment, whereas the children in the control condition did not increase their understanding. The findings suggest that drill and tests apps focusing on science content that take account of folk theories of science can support children’s science learning.
Engaging with science prior to and at the start of formal schooling has benefits (Saçkes et al. Citation2011; Tao, Oliver, and Venville Citation2012). For example, facility with classifying entities into living or non-living things prior to formal school entry predicts children’s third grade science achievement (Saçkes Citation2013). Interacting with scientific content not only benefits science learning but also increases vocabulary generally (French Citation2004). Thus, science learned prior to school and outside of school influences children’s science achievement. Unfortunately, however, children often enter preschool with lower levels of science understanding compared to other domains, such as literacy (Greenfield et al. Citation2009). Once they enter school, children make smaller gains in science compared to other domains (Greenfield et al. Citation2009). Given the importance of scientific learning in preschool children, we tested whether a simple technological application (app) could support children’s scientific thinking about physical and biological science.
One method for increasing young children’s scientific knowledge is through interactive technology, such as tablets. Children are motivated by technology and thus, should be willing to engage with tablets rendering them an effective learning method (Donohue Citation2014). When designed effectively to fit developmental levels (e.g. Hirsh-Pasek et al. Citation2015), technology supports children’s learning (e.g. Bullock et al. Citation2017). Two reviews have demonstrated that touchscreens support young children’s learning (Griffiths et al. Citation2020; Xie et al. Citation2018). Only two studies included in these reviews focused on science learning. Kowk et al. (Citation2016) reported that 4- to 8-year-olds learned decontextualised facts (e.g., which animal is the fastest?) about animals equally well from touchscreens as from a researcher. Although children learned factual knowledge, it is difficult to assess whether this study examined memory per se rather than science. In the study focused on physics, Herodotou (Citation2018) found that 4- to 5-year-olds learned about projectile motion from playing the game, Angry Birds. Thus, there is evidence that technology can increase reasoning about physical science, but it is uncertain whether technology can increase reasoning about physical science by providing content knowledge only. Additionally, whether findings can be generalised to other aspects of science, such as biological science, or other aspects of physical science, such as buoyancy is unknown. Finally, few apps have tested children’s learning at home rather than school (Griffiths et al. Citation2020). Based on the developmental psychology literature, we developed a drill-and-test app to fill this gap by testing whether the app could increase children’s scientific thinking in biological and physical science.
There are a few perspectives on scientific thinking. Zimmerman and Klahr (Citation2018) argue that scientific thinking involves scientific content knowledge and process skills. Although content knowledge and procedural skills benefit each other (Zimmerman Citation2007), our app targets content knowledge to support children in developing their framework theories through increased knowledge (Gelman and Noles Citation2011). A core competence perspective advances that preschool-aged children have developed basic but cohesive folk theories (framework theories) to conceptualise the biological, physical, and psychological domains (Gelman Citation2003; Gelman and Noles Citation2011; Wellman and Gelman Citation1992). Thus, children develop knowledge about distinguishing the ontological categories of living versus non-living things and understanding of the characteristics of living things (biology) as well as the properties of physical objects (Gelman and Frazier Citation2012). Much modularised knowledge begins as implicit knowledge in the preschool years (Karmiloff-Smith Citation1995). Another approach to science learning argues that accurate observation, reasoning about causality, and explaining connections are the core skills employed in science understanding (Tolmie, Ghazali, and Morris Citation2016). Drawing children’s attention to similarities across different objects and entities can help them encode exemplars, which then further develop their theories (Tolmie, Ghazali, and Morris Citation2016).
Our app incorporated these perspectives. From the scientific thinking perspective (Zimmerman Citation2007), we provided content knowledge to children. Our app taught children knowledge within the domains of biology and physics following the framework theory model (Gelman and Frazier Citation2012). From the core skill perspective (Tolmie, Ghazali, and Morris Citation2016), our app provided children with feedback on their previous observations in the world. Thus, the app built upon all three perspectives of how children develop scientific thinking.
Theories of biology
Within the biological domain, children develop an ontological category of living things and recognise biological processes. By 3 years, children report that animals and plants grow. Not until 5, however, do children (like adults) report that inanimate objects do not increase in size over time (Rosengren, et al. Citation1991) and are above chance in distinguishing animate from inanimate objects in categorisation tasks (Wright, Poulin-Dubois, and Kelley Citation2015). A longitudinal study indicated that between 3 and 4 years, children become more like adults in distinguishing animals and plants from mobile and immobile artefacts. Children also begin to restrict the biological properties of growing, eating, and drinking to living things (Margett-Jordan, Falcon, and Witherington Citation2017). Children, however, remain confused about whether plants are alive until about aged 6 years (Tao Citation2016) with large increases in knowledge between preschool and primary school ages (Villanueva, Villarroel, and Antón Citation2021). Moreover, children continue to overgeneralise some biological properties to non-biological entities after 5 years with children attributing psychological properties (e.g. happiness) to a greater degree to robots than do adults (Jipson and Gelman Citation2007). Thus, although children may develop an ontological category of animate objects based on biological attributes by 4 years, there remain differences between adults and children in attribution of psychological categories and classification of life status to plants.
Another component of children’s biological framework is recognising biological processes, such as inheritance. In contrast to their ontological category of living things, children’s understanding of inheritance may be less well developed in preschool (but see Springer and Keil Citation1989). Not until 7 years are children likely to differentiate patterns of inheritance in which children report that physical features are inherited by birth parents and psychological features are learned by adoptive parents (Solomon et al. Citation1996; Williams and Smith Citation2010). Moreover, white 5- to 6-year-old children are as likely to judge that a child may be equally to change their race as their emotional expressions, which suggests that they lack an understanding of the stability of inheritance (Roberts and Gelman Citation2016). Although preschoolers are similar to adults in attributing height to birth parents, they are less likely than adults to attribute weight as inherited from biological parents (Raman Citation2018). Similarly, children aged 7–13 are more likely to attribute intelligence and athleticism to choice or environment than genes, whereas adults are more likely to attribute these characteristics to genes than choice or environment (Meyer et al. Citation2020). In sum, children have a better developed category of ontological kinds than of biological processes, such as inheritance.
Theories of physics
The other domain the app targets is physics in which a framework theory involves understanding physical events, such as object dynamics, which rely on the properties of matter (Wellman and Gelman Citation1992). Our focus is on buoyancy and balance, both of which are central to children’s theories in physics. To determine an object’s density, the material properties of an object must be separated from its identity. To establish an object’s buoyancy, weight must be combined with volume in relation to the object while also representing the properties of the liquid. Because knowledge of matter underlies an understanding of buoyancy, many researchers have studied children’s understanding of buoyancy (e.g. Hsin and Wu Citation2011; Kallery Citation2015; Kohn Citation1993; Van Schaik et al. Citation2020). When examining children’s understanding of buoyancy, research has found that 3-year-old children are at chance at predicting whether objects will float or sink in water, whereas children older than 3 years and adults perform above chance (Kohn Citation1993). Kloos, Fisher, and Van Ordern (Citation2010) found that 4- to 5-year-olds perform better in identifying whether an object is buoyant when presented with objects in pairs when density was confounded with mass, but not when density was unconfounded with mass or volume. When using everyday materials, 5-year-old children are correct in identifying whether an object is buoyant at above chance levels (71%) and do not differ from 7-year-old children in their estimates (Rappolt-Schlichtmann et al. Citation2007). However, when asked to justify the buoyancy of an object, 5-year-olds are unable to encode one attribute (e.g. weight, material kind, etc.) per object (Rappolt-Schlichtmann et al. Citation2007), which may explain their difficulty. By age 7 years, children tend to encode at least one attribute of an object to explain buoyancy. Thus, although children may not be able to explain why objects are buoyant, they demonstrate an implicit understanding of whether objects are buoyant in water by 5 years.
Another way that attributes of objects, such as weight, may influence physical properties is through balance. Determination of balance on a fulcrum relies on weight and distance. When distance was held constant, children 4 years and older could determine what would happen to a scale when weights were added, but not 3 years (Wang, Williamson, and Meltzoff Citation2018). In contrast, children are less able to coordinate the effects of distance on a balance beam. Only with feedback, half of 5-year-olds, but not 4-year-olds could predict the effects of distance on a beam (Siegler and Chen Citation1998; Citation2002). More recent research also suggests that 5-year-olds can learn how to balance objects through instruction from an adult or book reading (Larsen, Venkadasalam, and Ganea Citation2020). Thus, understanding the effects of distance is more difficult than weight and children are below chance in their predictions at age 5 years. Nevertheless, both buoyancy and balance are central to developing theories of physics in children.
Supporting learning
There are many ways the current app was developed to support learning in biology and physics. First, children were taught guiding principles in each domain (e.g., which things are living) to help them develop scientific theories. The app provided verbal feedback because a large meta-analysis reported that direct teaching and guided discovery with feedback support learning more than pure discovery (Alfieri et al. Citation2011). Moreover, young preschoolers benefit from verbal feedback instead of non-verbal or textual one (Callagan and Reich Citation2018). Provision of immediate feedback reduces the cognitive load of the task, a precondition for successful learning (Sweller Citation2009), especially in revising scientific theories (Tolmie, Ghazali, and Morris Citation2016).
When designed to build on children’s previous knowledge (e.g. Hirsh-Pasek et al. Citation2015), technology supports children’s learning (e.g., Bullock et al. Citation2017). For this reason, we would expect our programme to support children to increase their understanding more about the topics of living things and buoyancy than inheritance and balance because 5-year-old children show facility with predictions in the former types of science (Margett-Jordan, Falcon, and Witherington Citation2017) more than the latter making learning more likely (Gelman and Frazier Citation2012). When children have a pre-existing scheme, it is more likely that they can learn information (Gelman and Frazier Citation2012).
Reviews on mathematical apps (Outhwaite et al. Citation2022) and reading development (Griffiths et al. Citation2020) have shown that drill and practice apps are common and successful for raising mathematical abilities and reading abilities, but that levelling of content is an important feature of successful apps (Outhwaite et al. Citation2022). Building on these reviews, we integrated levels into the app by asking children progressively more difficult concepts about each topic (e.g., inheritance) and including two topics in each domain that varied in difficulty (e.g., living things versus inheritance). Finally, we incorporated rewards by providing children a star for correct answers. Thus, we linked rewards to learning, rather than completion which has shown to improve children’s outcomes (Callagan and Reich Citation2018).
Finally, young children explore and learn with mobile devices in ways that are natural to them (touch, trial, and error). It is not only interactive apps that improve learning, but also drill and practice apps can support learning (Papadakis and Kalogniannakis Citation2017). Our app relied on drill and practice to help children learn. Drill and practice is easy to implement in an app and children can use it independently. In addition, drill and practice apps focus on the acquisition of learning content and have been shown to be very effective to improve mathematical knowledge (Outhwaite et al. Citation2022) and literacy (Kim et al. Citation2021).
Hypotheses
In sum, research indicates that children can learn about projectile motion and decontextualised information about animals from apps (Herodotou Citation2018; Kowk et al. Citation2016). This knowledge is needed because there is a less of a focus on teaching science in the preschool years compared to other domains (Greenfield et al. Citation2009). Our study tested whether playing an app that used drill-and-test for three weeks could improve children’s science learning about four science topics from children’s pre-test to post-test assessment. A large body of research suggests that guided discovery with feedback can support children’s learning across a variety of domain (Alfieri et al. Citation2011). We hypothesised that children’s science learning would increase from the playing the app because children the app provided guided discovery with feedback. We expected that children assigned to play the app would outperform children assigned to the control condition.
A core competence perspective (Gelman and Frazier Citation2012) suggests that children’s knowledge of living things and buoyancy (Siegler and Chen Citation1998; Solomon et al. Citation1996) is greater than their knowledge of inheritance and balance in the preschool years (Kloos, Fisher, and Van Ordern Citation2010; Margett-Jordan, Falcon, and Witherington Citation2017). In addition, based on previous literature of when children understand different aspects of biological and physical science, we predicted that preschoolers would show an improved understanding of living things and buoyancy but that for balance and inheritance, we would observe smaller benefits of the training (if any). To test these hypotheses, we compared children’s pre-test to post-test assessment to a control group of children who did not play the games as well as looking at changes in pre-test to post-test assessment in children who played the games.
Method
Participants
Children in the control condition had a mean age of 50.30 months (SD = 8.61, range = 38–67 months; 11 girls, n = 21) and children in the intervention condition were a mean of 53.21 months old (SD = 6.36; range = 43–71 months; 8 girls, n = 21), which was not significantly different, F(1, 40) = 1.57, p = .22 (Bayes factor = .26). There were 21 children in the control condition and 24 in the intervention condition. However, three children refused to complete the full pre-assessment and were dropped leaving 21 children in each condition. All other children completed the full batteries of both pre-and post-assessment. Children were mostly recruited from state and private nurseries, word of mouth, and websites. Children were mostly from London, Sussex, and Surrey, except for three English children recruited online. Parents completed an informed consent form before children provided assent. Children were randomly assigned to condition. When children were assigned to the intervention condition, we contacted parents to interview children at home and to show them how to play the games. For children assigned to the control condition, children could be tested in their homes or the nurseries. No child tested had a formal diagnosis of a neurodevelopmental disorder as reported by parents. Families were mostly middle-class and had a university degree.
Measures
Children’s science learning measures: pre- and post-assessment
To assess knowledge, children completed a sorting task with 24 picture cards for each of the four science areas (i.e. balance, buoyancy, living things, and inheritance) and asked to sort based on whether the depicted image is conceptually correct or incorrect. There were three levels of difficulty for each area based on different principles. For example, the first level of buoyancy assessed children’s understanding that size does not influence buoyancy by showing children two pictures simultaneously. Each picture displays a large and a small wooden ring. In one picture, both wooden rings float (Correct). Another picture displayed one floating and one sinking (Incorrect). The child needed to place the cards into a correct and incorrect pile. Children received 1 point for each card correctly sorted from the 24 cards. We developed two versions with slightly different items. We counterbalanced which version served as a pre-test or post-test. No items in the tablet game were identical to the items in the assessment.
We created this measure because there are no standardised measures of young children’s science learning across biological and physical sciences from a core competence perspective. We previously piloted the assessment with 32 children who did not participate in the present study. Children had a mean age of 43.21 month (SD = 5.17; 3–5 years). All children sorted some cards correctly (minimum = 6; maximum = 20 out of 24 for each content area).
Raven’s coloured progressive matrices
Raven’s Coloured Progressive Matrices (RCPM; Raven and RavenCitation1990) was used to assess overall cognitive abilities. This test includes 36 items divided into three sub-tests of 12 items each. In each item the participant is presented with a coloured pattern of which one part is missing and the participant is asked to select the missing part out of six options. There is no time limit and participants were encouraged to complete all 36 items. Testing took place on a one-to-one basis in a quiet room at the participant’s home or school.
App
The app was developed in 2014 based on the existing apps provided by BrainyBaby. The app was presented on an electronic device and included four content games. The app started with a landing page where children first met Millie, an animated character who introduces children to each of the content games (Creatures = Living, Families = Inheritance, Sink or Float = buoyancy, Seesaw = balance). As an introduction, Millie asked the child a question (e.g. ‘Which one eats?’). Children saw four pictures on the screen (e.g. ball of yarn, caterpillar, etc) and was asked the correct answer. For the test question, no matter what the child indicated, the correct answer was indicated. Each science area has a test question. Following this question, children started playing the game. For each question, children indicate the correct answer out of the four displayed. There was an easy, medium, and difficult level for each game and three rounds of five questions for each level (15 questions per level and 45 questions per game). Based on the core competence perspective (Gelman Citation2003), the games followed children’s increases in learning with three levels of questions differentiated by their level of difficulty. displays these levels.
Table 1. Levels of games.
Children had to score at least 4 out of 5 questions correctly to play a new round and progress up a level. For each question, children were shown four possible answers to choose from. At the start of each content game (e.g. Creatures) there was an example for which children were told the correct answer before they could select an answer. All instructions were provided verbally and modelled given that children of this age are pre-literate (Callagan and Reich Citation2018).
For questions following the warm-up for each game, the game provided immediate feedback (correct: ‘You have won a gold star’ or incorrect: ‘That was a hard one’). Children received a gold star when they answered a question correct. See for examples of questions asked about each game. This programme may be found at http://brainy-kids.co.uk/preschool-science-games/#/landing.
Table 2. Example questions.
Procedure
Kingston University granted the study ‘Children’s Science Learning from Tablets’ ethical approval (approval code: 141528). Data can be accessible from the authors upon request. Parents returned consent forms through nurseries or to the researcher directly. After parental consent and child assent, children were either tested in their nurseries (n = 10) or homes (n = 32). Children first completed the RCMP (Raven and Raven Citation1990) so that we could rule out the confound of intervention or control group having higher overall fluid intelligence scores. Next, they were administered the science learning measures. Six children interviewed in their homes were interviewed online using video-conferencing software because of COVID-19. Parents remained with the child during the online interviews. Three weeks after the first assessment, children were interviewed using the alternative form of the science learning measures. After the pre-assessment, children and parents in the intervention group were shown how to play the games. If children completed the pre-assessment at nursery or using video-conferencing software, we organised a meeting over Skype to explain to the parent and child how to play the games. The games were always played in the home environment. In between the pre-and post-assessments, the children in the intervention group were asked to play the games for 20 min a day for at least five days a week for three weeks. We phoned or emailed families in the intervention group after one week to remind them to play the games. Children in the control group only completed the pre-and post-assessments three weeks apart.
Analysis plan
First, we examined control variables. To examine differences between the two groups on age, overall cognitive abilities, and the science learning measure version completed first, we conducted between-subjects ANOVA models with condition as the between-subjects factor. Second, to test our hypotheses, we conducted separate ANCOVA models for each of the science areas with pre-assessment scores on the science learning measures as covariates and post-assessment scores as DVs. Whether children had played the games on the app (intervention) or not (control) served as an IV. Next, we conducted repeated-measures ANOVAs separately by condition to see whether children increased in their learning from pre- to post-assessment scores. Pre- and post-assessment scores served as repeated factors. We used the percentage correct on the science learning measures. Homogeneity of slopes was met for all the ANCOVA models.
Results
Control variables
Twenty-one children in the control and 20 children in the intervention condition completed the RCPM task (one child in the intervention condition did not complete the RCPM). There were no statistically significant differences between children assigned to the control (M = 13.20, SD = 4.65) and intervention conditions (M = 15.17, SD = 4.12), F(1, 39) = 2.07, p = .16 (Bayes factor = .33) for RCPM raw scores. Score on RCPM correlated with age in months, r (39) = .50, p = .001. Pearson correlations indicated that scores on RCPM correlated with pre-assessment, r (39) = .35, p = .03 and post-assessment, r (39) = .51, p = .01. There were no differences in pre-assessment on any of the measures (inheritance, F(1, 40) = 1.41, p = .24; living, F(1, 40) = .05, p = .82, buoyancy, F(1, 40) = .50, p = .49; balance, F(1, 39) = .03, p = .87).
For the science learning measures, ANOVA models did not indicate effects based on whether children took the A or B version or interaction effects with condition on any of the four outcome variables (all Fs < 1). displays the percentage correct on pre- and post- assessment on the Science Learning Measures.
Table 3. Pre- and post-test percentage correct.
Effects of the app on science learning measures
To examine the hypotheses, we report the ANCOVA models. For living things, the child’s pre-assessment score predicted the post-assessment score, F(1, 39) = 4.27, p = .046, = .10. Above and beyond pre-assessment scores, children in the intervention condition scored higher than did children in the control condition on the post-assessment, F(1, 39) = 7.97, p = .007,
= .17. There was no change in children’s assessments in the control condition, F(1, 20) = .01, p = .94. In contrast, children’s scores increased from the pre- to the post-assessment in the intervention condition, F(1, 20) = 7.69, p = .01,
= .28.
For inheritance, the child’s pre-assessment score predicted the post-assessment score, F(1, 39) = 12.82, p = .001, = .25. Above and beyond pre-assessment scores, children in the intervention condition did not score higher than did children in the control condition on the post-assessment, F(1, 39) = .61, p = .44. In neither the intervention, F(1, 20) = 3.00, p = .10, nor the control condition, F(1, 20) = 2.32, p = .14, did children increase in their scores from pre- to the post-assessment.
For buoyancy, the child’s pre-assessment score predicted the post-assessment score, F(1, 39) = 12.04, p = .001, = .24. Above and beyond pre-assessment scores, children in the intervention condition scored higher than did children in the control condition on the post-assessment, F(1, 39) = 10.72, p = .002,
= .22. There was no change in the children’s assessments in the control condition, F(1, 20) = .87, p = .36. In contrast, children’s scores increased from pre-assessment to post-assessment in the intervention condition, F(1, 20) = 5.83, p = .03,
= .23.
For balance, the child’s pre-assessment score did not predict their post-assessment score, F(1, 39) = .92, p = .34. Above and beyond pre-assessment scores, children in the intervention condition scored higher than did children in the control condition on the post-assessment, F(1, 39) = 4.90, p = .03, = .11. Because the pre-assessment scores did not predict post-assessment scores, we re-ran the ANOVA to check that over-controlling for the pre-assessment scores did not increase the post-assessment to statistical significance. A between-subjects ANOVA with post-assessment scores as a DV and condition as an IV replicated the statistically significant difference in condition, F(1, 39) = 5.02, p = .03,
= .11. There was no change from pre- to post-assessment in the control condition, F(1, 20) = .10, p = .75, and only a marginally significant effect in the intervention condition, F(1, 20) = 3.59, p = .07,
= .15.
Discussion
The current study examined whether providing 3- to 5-year-olds with content knowledge via a technological app would increase their knowledge of biology and physical science content. In addition, we examined whether providing content knowledge alone would benefit specific sub-domains of biological and physical science. The app was successful in improving children’s knowledge of living things and buoyancy using a near-transfer task. We also found some suggestion that our app could help children learn about balance. In contrast, our drill-and-practice app did not help children learn about inheritance.
We included a control group to demonstrate that although children increase in their knowledge about these domains, children’ science knowledge does not increase in a short time frame without instruction. Supporting this view, the control group of children did not increase in their knowledge from pre-assessment to post-assessment in any science domain. Our study is a first step in testing the effectiveness of the app (see Green et al. Citation2019, for a description of best methods in intervention research). Children might have learned the material as well in an in-person session. Our findings suggest that children can learn content knowledge about buoyancy and living things (and possibly balance) from an app that uses drill and practice. Scientific content knowledge guides children in developing their theories of biology and physics (Gelman and Frazier Citation2012).
We know that using live, in-person interventions have similarly shown that children learn about these topics in museum and classroom activities. For example, Tenenbaum, Rappolt-Schlichtmann, and Zanger (Citation2004) found that activities involving attention to the contents of objects increased kindergarten children’s understanding of sinking and floating. More recently, classroom activities support 4- to 6-year-olds to understand that the material of an object is central to buoyancy and that size, weight, and shape are irrelevant (Kallery Citation2015). Similarly, after a half hour intervention many 5-year-olds were able to reference the material kind of objects (Hsin and Wu Citation2011) Through an individual conversation in which children’s attention was drawn to plants’ similarities with animals, 5- to 6-year-olds increased their understanding of living things and classified plants as living things (Zogza and Papamichael Citation2000). Although previous interventions conducted in person on the topics of living things and buoyancy are effective in increasing children’s knowledge, they are time consuming and expensive to implement. Moreover, the app was able to improve learning through simple trial and error. Our findings provide support that children’s knowledge of living things and buoyancy can be increased through children playing an app, which is less expensive than visiting a museum.
Our app may have been successful because it was informed by literature on how children learn content, both in terms of how to teach young children successfully as well as the specific content taught in the app. Based on a meta-analysis, children were provided verbal feedback because timely feedback supports learning more than does discovery learning (Alfieri et al. Citation2011). Although our app only provided motivational feedback about whether or not the child’s answer was correct and not why their answer was correct or incorrect (e.g. explanatory feedback), the verbal feedback included in the app allowed the preschoolers to successfully develop their knowledge through trial and error (Blair Citation2013). The app also included a reward system that promoted children’s learning, rather than completion of the game. In addition, the app featured different levels for children to progress through. Previous evaluation of mathematical apps in preschoolers has shown that learning outcomes were maximised when the maths apps included levelling as well as feedback (Outhwaite et al. Citation2022). Finally, the app taught children principles in each domain (e.g. which materials float and sink) so that they could begin to draw regularities. Accurate observation is hypothesised to be central to scientific learning (Tolmie, Ghazali, and Morris Citation2016). Drawing children’s attention to content knowledge can help them accurately encode information. However, information about causality might have further increased children’s development of theories given causality’s hypothesised relations to scientific learning (Tolmie, Ghazali, and Morris Citation2016). Thus, an app that taught children why the content was correct may have been even more beneficial. As the app included many best practices to help preschoolers learn through apps (Callagan and Reich Citation2018), it is currently not possible to evaluate what specifically makes learning through the app successful. However, it is likely that it is the combination of these features that makes the app developmentally appropriate (Meyer et al. Citation2021; Walker Citation2010).
Teaching preschoolers about balance through the app games was less successful than teaching about buoyancy. The balancing games focused on the distance of the objects from the balancing point. When learning about balance, 4- and 5-year-old children learn to understand that weight makes a difference when given feedback in trials involving difference in weight. In contrast, few 4-year-olds and only 45% of 5-year-olds learned about the role of distance after receiving feedback in which distance differed on a balance scale (Siegler and Chen Citation1998). Children younger than 5 may need more than simple motivational feedback to learn about distance. Explanations guide children’s learning of counterintuitive topics (Kelemen Citation2019). As such, future interventions should include explanatory feedback that clarifies why distance influences the balance of a beam to guide learning (Alfieri et al. Citation2011).
Children may also need explanations as well as contextual background about mechanisms to construct their theories of inheritance, which may explain why our intervention was not effective in increasing children’s understanding of inheritance. However, a simple explanation of intrauterine development and birth did not lead to increases in 4- or 7-year-old children’s understanding about inheritance (Williams and Afleck Citation1999). In contrast, after ten lessons about birth and genetic material, 5-year-olds were able to increase their understanding of inheritance (Ergazaki et al. Citation2015). Our intervention did not focus on the mechanisms underlying inheritance, which young children may need to construct their theories. Future research should examine whether providing richer information (e.g. explanations) after incorrect responses could support increased learning (Callagan and Reich Citation2018). After such information is provided, children could be asked to guess again, which would increase the degree to which they play an active role in the game (Hirsh-Pasek et al. Citation2015).
Alternatively, balance and inheritance may be concepts that are too advanced for children of these ages to learn. Although children can learn via training, there are developmental progressions in their learning (Gelman and Frazier Citation2012). Some knowledge in children’s naïve theories is acquired at later stages than other knowledge. Perhaps, these concepts are too difficult until children are older and have developed richer theories of biology and physics in which they can incorporate the new information.
Limitations
There are limitations to the present study. One limitation is that we did not study how parents support children’s learning about science in the home environment. Parents’ explanations support children in developing their conceptual understanding of science. Everyday conversations with parents help children negotiate their understanding of science topics (Hohenstein and Tenenbaum Citation2023; Tenenbaum and Hohenstein Citation2016). These conversations vary with the educational and cultural backgrounds of families (Tenenbaum and Callanan Citation2008). Parents may use different types of talk when scaffolding children using iPads whilst playing reader apps (Neumann Citation2018). Future research should examine whether parents also scaffold science differently when playing apps with scientific content.
Another limitation is that we did not examine how children’s interactions with technology changed over the course of the study. Certainly it would be valuable to study other outcomes instead of simply focusing on cognitive learning gains. Future research with open-ended apps should examine how children’s relationships with technology change over time as an additional measure of learning.
Impact on theory and practice
The current study has shown that children playing games on an app that provides content knowledge can increase children’s understanding of living things and buoyancy after only three weeks. These findings increase our theoretical understanding of how preschoolers develop their framework theories and show that not all science knowledge can be easily increased through providing conceptual facts. For example, particular aspects of children’s biological theories (distinguishing living things) were easier for children to learn than other aspects (inheritance), which provides further evidence for the order in which children can appropriate knowledge about these topics. Moreover, previous research using apps had only examined knowledge about the core competence of motion (Herodotou Citation2018). Like our study, Herodotou also did not teach children information in a live session compared to playing a computer game. Thus, the current study adds to the evidence that core competences can be provided through children playing games on apps.
Finally, whilst previous studies related to educational apps in general, have often focused on evaluating the use of apps in the school environment (Griffiths et al. Citation2020), the current study focuses on apps in the home environment. Seeing the importance of preschoolers’ science knowledge for further science achievement (Saçkes et al. Citation2011; Tao, Oliver, and Venville Citation2012), the use of apps can be an inexpensive and motivational way to improve science knowledge in children. Children are often willing to engage with tablets (Donohue Citation2014). As such, apps present a significant opportunity for out-of-school, informal learning when designed in educationally appropriate ways (Hirsh-Pasek et al. Citation2015).
In terms of implications for early childhood education, we would recommend that apps are used to support learning in the classroom. We would not recommend that apps supplant instruction. However, apps can reinforce learning in the classroom to help children make accurate observations, necessary for science learning (Tolmie, Ghazali, and Morris Citation2016). In such a way, technology can complement in-person learning.
Conclusion
In sum, the current study provides a better understanding that apps that provide content knowledge only can improve science knowledge as well as what their limitations are. A better understand of which type of app works (in terms of type of content and level of interactivity) for which type of science learning will help parents and teachers to choose apps more effectively. Future studies should also examine the impact on preschoolers’ theories. Knowledge about the effectiveness of apps as a learning tool is needed. During the COVID crisis, much in-person learning was curtailed or supplanted with online learning in the home. For many reasons, it is especially important that developmental and educational science inform apps to support and increase children’s science learning in the home environment.
Data availability statement
Anonymised data will be available from reasonable request from the authors.
Disclosure statement
No potential conflict of interest was reported by the author(s).
References
- Alfieri, Lewis, Patricia J. Brooks, Naomi J. Aldrich, and Harriet R. Tenenbaum. 2011. “Does Discovery-based Instruction Enhance Learning?” Journal of Educational Psychology 103: 1–18. https://doi.org/10.1037/a0021017.
- Blair, Kristen P. 2013. Learning in Critter Corral: Evaluating Three Kinds of Feedback in a Preschool Math app. In Proceedings of the 12th International Conference on Interaction Design and Children, 372–375. https://doi.org/10.1145/2485760.2485814.
- Bullock, Emma P., Jessica F. Shumway, Christina M. Watts, and Patricia S. Moyer-Packenham. 2017. “Affordance Access Matters: Preschool Children’s Learning Progressions While Interacting with Touch-screen Mathematics Apps.” Technology, Knowledge and Learning 22 (3): 485–511. https://doi.org/10.1007/s10758-017-9312-5.
- Callagan, Melissa N., and Stephanie M. Reich. 2018. “Are Educational Preschool Apps Designed to Teach? An Analysis of the app Market.” Learning, Media and Technology 43 (3): 280–293. https://doi.org/10.1080/17439884.2018.1498355.
- Donohue, Chip, ed. 2014. Technology and Digital Media in the Early Years: Tools for Teaching and Learning. New York and London: Routledge.
- Ergazaki, Marida, Eftychia Valanidou, Maria-Christina Kasimati, and Mara Kalantzi. 2015. “Introducing a Precursor Model of Inheritance to Young Children.” International Journal of Science Education 37 (18): 3118–3142. https://doi.org/10.1080/09500693.2015.1121551.
- French, Lucia. 2004. “Science as the Center of a Coherent, Integrated Early Childhood Curriculum.” Early Childhood Research Quarterly 19 (1): 138–149. https://doi.org/10.1016/j.ecresq.2004.01.004.
- Gelman, Susan A. 2003. The Essential Child: Origins of Essentialism in Everyday Thought. Oxford, UK: Oxford University Press..
- Gelman, Susan A., and Brandy Frazier. 2012. “Development of Thinking in Children.” In The Oxford Handbook of Thinking and Reasoning, edited by K. J. Holyoak, and R. G. Morrison, 513–528. Oxford, UK: Oxford University Press..
- Gelman, Susan A., and Nicholaus S. Noles. 2011. “Domains and Naïve Theories.” WIRES Cognitive Science 2 (5): 490–502. https://doi.org/10.1002/wcs.124.
- Green, Shawn C., Daphne Bavelier, Arthur F. Kramer, Sophia Vinogradov, Ulrich Ansorge, Karlene K. Ball, Ulrike Bingel, et al. 2019. “Improving Methodological Standards in Behavioral Interventions for Cognitive Enhancement.” Journal of Cognitive Enhancement 3 (1): 2–29. https://doi.org/10.1007/s41465-018-0115-y.
- Greenfield, Daryl B., Jamie Jirout, Ximena Dominguez, Ariela Greenberg, Michelle Maier, and Janna Fuccillo. 2009. “Science in the Preschool Classroom: A Programmatic Research Agenda to Improve Science Readiness.” Early Education and Development 20 (2): 238–264. https://doi.org/10.1080/10409280802595441.
- Griffiths, Shayl F., Mary B. Hagan, Perrine Heymann, Brynna H. Heflin, and Daniel M. Bagner. 2020. “Apps as Learning Tools: A Systematic Review.” Pediatrics 145 (1): e20191579. https://doi.org/10.1542/peds.2019-1579.
- Herodotou, Christothea. 2018. “Mobile Games and Science Learning: A Comparative Study of 4 and 5 Years old Playing the Game Angry Birds.” British Journal of Educational Technology 49 (1): 6–16. https://doi.org/10.1111/bjet.12546.
- Hirsh-Pasek, Kathy, Jennifer M. Zosh, Roberta M. Golinkoff, James H. Gray, Michael B. Robb, and Jordy Kaufman. 2015. “Putting Education in “Educational” Apps.” Psychological Science in the Public Interest 16 (1): 3–34. https://doi.org/10.1177/1529100615569721.
- Hohenstein, Jill M., and Harriet R. Tenenbaum. 2023. “Family Conversations About Species Change as Support for Children’s Developing Understandings of Evolution.” Science Education 107: 810–834. https://doi.org/10.1002/sce.21783.
- Hsin, Ching-Ting, and Hsin-Kai Wu. 2011. “Using Scaffolding Strategies to Promote Young Children’s Scientific Understandings of Floating and Sinking.” Journal of Science Education and Technology 20: 656–666. https://doi.org/10.1007/s10956-011-9310-7.
- Jipson, Jennifer L., and Susan A. Gelman. 2007. “Robots and Rodents: Children’s Inferences About Living and Non-living Kinds.” Child Development 78 (6): 1675–1688. https://doi.org/10.1111/j.1467-8624.2007.01095.x.
- Kallery, Maria. 2015. “Science in Early Years Education: Introducing Floating and Sinking as a Property of Matter.” International Journal of Early Years Education 23 (1): 31–53. https://doi.org/10.1080/09669760.2014.999646.
- Karmiloff-Smith, Annette. 1995. “Annotation: The Extraordinary Cognitive Journey from Foetus through Infancy.” Journal of Child Psychology and Psychiatry 36 (8): 1293–1313. https://doi.org/10.1111/j.1469-7610.1995.tb01665.x.
- Kelemen, Deborah. 2019. “The Magic of Mechanism: Explanation-Based Instruction on Counterintuitive Concepts in Early Childhood.” Perspectives on Psychological Science 14 (4): 510–522. https://doi.org/10.1177/1745691619827011.
- Kim, James, J. Gilbert, Qun Yu, and Charles Gale. 2021. “Measures Matter: A Meta-Analysis of the Effects of Educational Apps on Preschool to Grade 3 Children’s Literacy and Math Skills.” AERA Open 7: 23328584211004183.
- Kloos, Heidi, Anna Fisher, and Guy C. Van Ordern. 2010. “Situated Naïve Physics: Task Constraints Decide What Children Know About Density.” Journal of Experimental Psychology: General 139 (4): 625. https://doi.org/10.1037/a0020977.
- Kohn, Amy S. 1993. “Preschoolers’ Reasoning About Density: Will it Float?” Child Development 64 (6): 1637–1650. https://doi.org/10.2307/1131460.
- Kowk, Kristine, Siba Ghrear, Vivian Li, Taeh Haddock, Patrick Coleman, and Susan A.J. Birch. 2016. “Children Can Learn New Facts Equally Well from Interactive Media Versus Face to Face Instruction.” Frontiers in Psychology 7: 1603. https://doi.org/10.3389/fpsyg.2016.01603.
- Larsen, Nicole E., Vaunam P. Venkadasalam, and Patrica A. Ganea. 2020. “Prompting Children’s Belief Revision About Balance through Primary and Secondary Sources of Evidence.” Frontiers in Psychology 11: 1503. https://doi.org/10.3389/fpsyg.2020.01503.
- Margett-Jordan, Tessa, Rachael G. Falcon, and David C. Witherington. 2017. “The Development of Preschoolers’ Living Kinds Concept: A Longitudinal Study.” Child Development 88 (4): 1350–1367. https://doi.org/10.1111/cdev.12709.
- Meyer, Meredith, Stephen. O. Roberts, Toby E. Jayaratne, and Susan. A. Gelman. 2020. “Children’s Beliefs About Causes of Human Characteristics: Genes, Environment, or Choice?” Journal of Experimental Psychology: General 149 (10): 1935–1949. https://doi.org/10.1037/xge0000751.
- Meyer, Meredith, Jennifer M. Zosh, Caroline McLaren, Michael Robb, Harlen McCafferty, Roberta M. Golinkoff, Kathy Hirsh-Pasek, and Jenny Radesky. 2021. “How Educational are “Educational” Apps for Young Children? App Store Content Analysis Using the Four Pillars of Learning Framework.” Journal of Children and Media 15 (4): 526–548. https://doi.org/10.1080/17482798.2021.1882516.
- Neumann, Michelle M. 2018. “Parent Scaffolding of Young Children’s Use of Touch Screen Tablets.” Early Child Development and Care 188 (12): 1654–1664. https://doi.org/10.1080/03004430.2016.1278215.
- Outhwaite, Laura, Rin Early, Christothea Herodotou, and Jo Van Herwegen. 2022. Can Maths Apps Add Value to Young Children's Learning? A Systematic Review and Content Analysis. London: Nuffield Foundation.
- Papadakis, Stamatios, and Michail Kalogniannakis. 2017. “Mobile Educational Applications for Children: What Educators and Parents Need to Know.” International Journal of Mobile Learning and Organisation 11: 256. https://doi.org/10.1504/IJMLO.2017.085338.
- Raman, Laksmi. 2018. “Do Children Think That Inheritance Determines Height and Weight?” Infant and Child Development 27 (1), Advance online publication. https://doi.org/10.1002/icd.2041.
- Rappolt-Schlichtmann, Gabriella, Harriet R. Tenenbaum, Margy Koepke, and Kurt W. Fischer. 2007. “Transient and Robust Knowledge: Contextual Support and the Dynamics of Children’s Reasoning About Density.” Mind, Brain, and Education 1: 98–108. https://doi.org/10.1111/j.1751-228X.2007.00010.x.
- Raven, John C., and John Hugh Raven. 1990. Manual for Raven’s Progressive Matrices and Vocabulary Scales: Research Supplement. Vol 3. Oxford: Oxford Psychologists Press.
- Roberts, Stephen O., and Susan A. Gelman. 2016. “Can White Children Grow Up to Be Black? Children’s Reasoning About the Stability of Emotion and Race.” Developmental Psychology 52 (6): 887–893. https://doi.org/10.1037/dev0000132.
- Rosengren, K.S., Gelman, S.A., Kalish, C.W. and McCormick, M., 1991. As time goes by: Children's early understanding of growth in animals. Child Development, 62 (6): 1302–1320.
- Saçkes, Mesut. 2013. “Children’s Competencies in Process Skills in Kindergarten and Their Impact on Academic Achievement in Third Grade.” Early Education & Development 24 (5): 704–720. https://doi.org/10.1080/10409289.2012.715571.
- Saçkes, Mesut, Kathy C. Trundle, Randy L. Bell, and Ann A. O’Connell. 2011. “The Influence of Early Science Experience in Kindergarten on Children’s Immediate and Later Science Achievement: Evidence from the Early Childhood Longitudinal Study.” Journal of Research in Science Teaching 48 (2): 217–235. https://doi.org/10.1002/tea.20395.
- Siegler, Robert S., and Zhe Chen. 1998. “Developmental Differences in Rule Learning: A Microgenetic Analysis.” Cognitive Psychology 36 (3): 273–310. https://doi.org/10.1006/cogp.1998.0686.
- Siegler, Robert S., and Zhe Chen. 2002. “Development of Rules and Strategies: Balancing the Old and the New.” Journal of Experimental Child Psychology 81 (4): 446–457. https://doi.org/10.1006/jecp.2002.2666.
- Solomon, Gregg E. A., Susan C. Johnson, Deborah Zaitchik, and Susan Carey. 1996. “Like Father, Like Son: Young Children’s Understanding of How and Why Offspring Resemble Their Parents.” Child Development 67 (1): 151–171. https://doi.org/10.2307/1131693.
- Springer, Ken, and Frank C. Keil. 1989. “On the Development of Biologically Specific Beliefs: The Case of Inheritance.” Child development 60: 637–648. https://doi.org/10.2307/1130729.
- Sweller, John. 2009. “Cognitive Bases of Human Creativity.” Educational Psychology Review 21 (1): 11–19. https://doi.org/10.1007/s10648-008-9091-6.
- Tao, Ying. 2016. “Young Chinese Children’s Justifications of Plants as Living Things.” Early Education and Development 27 (8): 1159–1174. https://doi.org/10.1080/10409289.2016.1210456.
- Tao, Ying, Mary Oliver, and Grady Venville. 2012. “Long-term Outcomes of Early Childhood Science Education: Insights from a Cross-National Comparative Case Study on Conceptual Understanding of Science.” International Journal of Science and Mathematics Education 10 (6): 1269–1302. https://doi.org/10.1007/s10763-012-9335-2.
- Tenenbaum, Harriet R., and Maureen A. Callanan. 2008. “Parents’ Science Talk to Their Children in Mexican-descent Families Residing in the USA.” International Journal of Behavioral Development 32: 1–12. https://doi.org/10.1177/0165025407084046.
- Tenenbaum, Harriet R., and Jill M. Hohenstein. 2016. “Parent-child Talk About the Origins of Living Things.” Journal of Experimental Child Psychology 150: 314–329. https://doi.org/10.1016/j.jecp.2016.06.007.
- Tenenbaum, Harriet R, Gabrielle Rappolt-Schlichtmann, and V V Zanger. 2004. “Children’s learning about water in a museum and in the classroom.” Early Childhood Research Quarterly 19 (1): 40–58.
- Tolmie, Andrew K., Zayba Ghazali, and Suzanne Morris. 2016. “Children’s Science Learning: A Core Skills Approach.” British Journal of Educational Psychology 86 (3): 481–497. https://doi.org/10.1111/bjep.12119.
- Van Schaik, J. E., T. Slim, R. K. Franse, and M. E. Raijmakers. 2020. “Hands-on Exploration of Cubes’ Floating and Sinking Benefits Children’s Subsequent Buoyancy Predictions.” Frontiers in Psychology 11: 1665. https://doi.org/10.3389/fpsyg.2020.01665.
- Villanueva, Xabier, Jose Domingo Villarroel, and Avaro Antón. 2021. “Young Children’s Drawings of Plant World: A Cohort Study Analysing Pictorial Content.” Journal of Biological Education 55 (3): 225–237. https://doi.org/10.1080/00219266.2019.1679656.
- Walker, Harry. 2010. “Evaluating the Effectiveness of Apps for Mobile Devices.” Journal of Special Education Technology 24 (4): 59–63.
- Wang, Zhidan, Rebecca A. Williamson, and Andrew N. Meltzoff. 2018. “Preschool Physics: Using the Invisible Property of Weight in Causal Reasoning Tasks.” Plos One, https://doi.org/10.1371/journal.pone.0192054.
- Wellman, Henry M., and Susan A. Gelman. 1992. “Cognitive Development: Foundational Theories of Core Domains.” Annual Review of Psychology 43 (1): 337–375. https://doi.org/10.1146/annurev.ps.43.020192.002005.
- Williams, Joanne M., and Gillian Afleck. 1999. “The Effects of an Age-Appropriate Intervention on Young Children's Understanding of Inheritance.” Educational Psychology 19 (3): 259–275. https://doi.org/10.1080/0144341990190302.
- Williams, Joanne M., and Lesley A. Smith. 2010. “Concepts of Kinship Relations and Inheritance in Childhood and Adolescence.” British Journal of Developmental Psychology 28 (3): 523–546. https://doi.org/10.1348/026151009X449568.
- Wright, Kristyn, Diane Poulin-Dubois, and Elizabeth Kelley. 2015. “The Animate-Inanimate Distinction in Preschool Children.” British Journal of Developmental Psychology 33 (1): 73–91. https://doi.org/10.1111/bjdp.12068.
- Zie, Heping, Ji Peng, Mengyuan Qin, Xuzhe Huang, Fei Tian, and Zongkui Zhou. 2018. “Can Touchscreen Devices Be Used to Facilitate Young Children’s Learning? A Meta-analysis of Touchscreen Learning Effect.” Frontiers in Psychology 9: 2580. https://doi.org/10.3389/fpsyg.2018.02580.
- Zimmerman, Corinne. 2007. “The Development of Scientific Thinking Skills in Elementary and Middle School.” Developmental Review 27 (2): 172–223. https://doi.org/10.1016/j.dr.2006.12.001.
- Zimmerman, Corinne, and David Klahr. 2018. “Stevens' Handbook of Experimental Psychology and Cognitive Neuroscience.” Steven’s Handbook of Experimental Psychology and Cognitive Neuroscience 4: 1–25. https://doi.org/10.1002/9781119170174.epcn407.
- Zogza, Vassiliki, and Yannis Papamichael. 2000. “The Development of the Concept of Alive by Preschoolers Through a Cognivive Conflict Teaching Intervention.” European Journal of Psychology of Education 15 (2): 191–205. https://doi.org/10.1007/BF03173174.