ABSTRACT
It is well known that the growth of legumes is greatly affected not only by fertilizer nitrogen but also by fixed nitrogen by symbiosis with rhizobia. We have reported that a polymorphism in SEN1, which is essential for nitrogen fixation in Lotus japonicus, could be used to enhance nitrogen fixation and plant growth. In this study, we examined whether the same strategy could be applied to Glycine max. Sequencing of orthologs of the LjSEN1 gene in 38 soybean cultivars revealed that the polymorphism occurred at Glyma.08g076300. We defined the standard sequence that most of the cultivars retained as Peking type SEN1, and the mutated sequence found in Enrei as Enrei type SEN1. To investigate the effect of genotype differences, we generated near-isogenic lines by crossing Enrei with Fukuyutaka, which has Peking type SEN1, and analyzed their characteristics. The results showed that Enrei type enhanced nitrogen fixation activity and promoted plant growth. Since soybean SEN1 has been reported to be a homolog of vacuolar iron transporter-like protein, we investigated the iron content in the nodules. In the result, the iron content in the nodules of Enrei type was significantly higher than that of Peking type. Iron is known to be present in the active center of nitrogenase, and the high iron content may have contributed to the increased nitrogen fixation activity. These results suggest that Enrei type SEN1 is a nitrogen fixation enhancing gene and that introduction of Enrei type SEN1 may reduce nitrogen fertilizer application and increase yield.
Introduction
Nitrogen is an important element in various plant growth processes such as protein synthesis, photosynthesis, and nutrient transport. Although, it is a nutrient that is easily lost in the soil, for example ammonia ions are converted to nitrogen gas or nitric oxide by nitrification and denitrification, so its application tends to be high (Seefeldt et al., Citation2009; Shi et al., Citation2013; Subbarao et al., Citation2009). Besides, the application of nitrogen fertilizers leads to problems such as soil acidification, water pollution, and increased greenhouse gas emissions (Gao et al., Citation2014; Zhang et al., Citation2023). In addition, fertilizer prices have skyrocketed by the Ukraine conflict from 2021. Hence, it has become extremely important to make it possible to grow crops with less nitrogen fertilizer worldwide.
Nitrogen-fixing bacteria such as rhizobia can fix atmospheric nitrogen in a form that plants can use, which is called biological nitrogen fixation (BNF). This BNF has many advantages, including safety, efficiency, and lack of secondary pollution (Bosse et al., Citation2021; Zhang et al., Citation2023). The symbiosis between legumes and rhizobia results in the formation of nodules on plant roots, and trade-fixed nitrogen and photosynthetic products (Long, Citation1989; Mylona et al., Citation1995; Schauser et al., Citation1999). Fixed nitrogen by rhizobia accounts for most of the nitrogen in seeds, and it has been reported that about 60–75% of the nitrogen in soybean, which is considered to have high nitrogen fixation capacity, is derived from the nitrogen fixed by rhizobia (Takahashi et al., Citation1992). In other words, it is important to maintain high activity of rhizobia to ensure high soybean production. Leguminous plants can grow on barren land compared to other crops because of the symbiotic nitrogen fixation. If nitrogen fixation can be enhanced, it is expected that they will be able to grow on even poorer land than before. Thus, in terms of crop production, enhanced symbiotic nitrogen fixation could achieve a reduction in nitrogen fertilizer (Aloo & Ojija, Citation2023).
It has been reported many studies that try to apply symbiotic nitrogen fixation to crop production. For example, studies for imparting nitrogen fixation ability to non-leguminous plants (Tsujimoto et al., Citation2018), and searching for plant growth-promoting rhizobacteria (PGPR) that enhance growth promotion by rhizobial symbiosis (Hasibuan et al., Citation2021; Win et al., Citation2018; Zhang et al., Citation2023). Tominaga et al. (Citation2012) also performed quantitative trait loci (QTL) analysis using RILs of Miyakojima MG20 and Gifu B129, the experimental lines of Lotus japonicus, to identify loci involved in symbiotic nitrogen fixation. The results revealed that there were five QTL with positive nitrogen fixation activity in MG20. In addition, Klein et al. (Citation2009) reported the existence of a QTL related to seed weight in the neighborhood one of them.
The SEN1 gene was found to be located in the vicinity of a QTL associated with seed weight. The SEN1 gene is essential for nitrogen fixation in nodules on L. japonicus (Hakoyama et al., Citation2012). The sen1 mutant forms nodules but does not exhibit any nitrogen fixation activity, resulting in remarkably poor growth under nitrogen-free conditions (Hakoyama et al., Citation2012; Kawaguchi et al., Citation2002; Suganuma et al., Citation2003). It is different from the characteristics of mutants without nitrogen fixation activity such as sst1, fen1, ign1, and Ljsym105, which are less active in nitrogen fixation than the wild type (Hakoyama et al., Citation2009; Hossain et al., Citation2006; Krusell et al., Citation2005; Kumagai et al., Citation2007). Therefore, the role of the SEN1 gene in nitrogen fixation is considered to be of extreme importance, and SEN1 may be one of the genes that provide the QTL mentioned above.
Nishida et al. (Citation2020) reported that a polymorphism in the LjSEN1 gene may be one of the causative genes of these QTLs, by analyzing the characteristics of near isogenic lines (NILs) and hairy-roots transformants. Furthermore, it was reported that MG20 type SEN1 promoted plant growth such as shoot length and root length, showed high nitrogen fixation, and increased seed weight. These results indicate that MG20 type SEN1 is a gene that enhances nitrogen fixation.
If MG20 type SEN1 enhances nitrogen fixation, then soybean (Glycine max), which has a polymorphism in the SEN1 ortholog (GmSEN1), may also have an enhanced nitrogen-fixation type SEN1. In this study, we showed the presence of polymorphisms in the GmSEN1 gene and investigated the effects of the different genotypes on nitrogen-fixation activity and plant growth.
Materials and methods
Plant material
Nucleotide sequence of GmSEN1s was determined using the 38 soybean cultivars shown in . Using Fukuyutaka having Peking type SEN1 as a background, a NILs of soybean with Enrei type SEN1 were created. Enrei type SEN1 lines were obtained by five times backcrossing with Fukuyutaka to F1 plant, obtained by crossing Enrei and Fukuyutaka. Five heterozygous lines were selected from BC5F2 obtained by selfing BC5F1, and by selfing these lines, a homozygous line of BC5F4 was finally isolated. BC5F4 was used in cultivation experiments.
Table 1. Cultivars used in this study.
Determination of sequence
The leaves of 38 soybean cultivars were cut into 1 cm2 piece and DNA was extracted. To determine nucleotide sequence of four GmSEN1 gene, the 5’ UTR to 3’ UTR regions of GmSEN1 genes in 38 soybean cultivars were amplified by PCR and sequenced. The primers used for PCR was bellow; 5ʹ–ATGGCTACTAGTACACTCAG–3ʹ and 5ʹ–TTATTCTTTATCTAAAGCTT–3ʹ for Glyma.05G121600, 5ʹ–TCCCTCTGAAAACTCCAC–3ʹ and 5ʹ–CACAGCCTTACTTATCAT–3ʹ for Glyma.05G121500, 5ʹ–ATGGCGAATCGTTAACTATTTATCC–3ʹ and 5ʹ–AAATCTTTTTAACTTTTCTTATTGA–3ʹ for Glyma.08G076300, and 5ʹ–AGGGTTATTGTGGTTGTGGC–3ʹ and 5ʹ–TCATACTGAGTGCACACAGC −3ʹ for Glyma.08G076200.
Induction of hairy roots and nodulation experiment
Since there is no intron in SEN1 gene of G.max, the coding sequence (CDS) of SEN1 gene for Peking or Enrei were amplified by PCR with primers, 5ʹ–CACCATGGCTAATGCTACACCTAATGGT–3ʹ and 5ʹ–TTATTCTAAAGCGCTAGCACCCATT–3ʹ using genomic DNA as a template. Each amplified fragment was cloned into the pENTR/D-TOPO entry vector (Invitrogen, Carlsbad, CA, USA). The inserts of the entry clones were recloned into the pUB-GW-GFP vector (Maekawa et al., Citation2008), which was obtained from the National Bio Resource Project (NBRP) Lotus and Glycine (Japan), by LR reaction using the Gateway cloning system (Invitrogen). The resultant plasmid or empty vector (EV, negative control) was introduced into Agrobacterium rhizogenes LBA1334 by electroporation. Seeds of L. japonicus sen1 mutant were germinated for 2 days in the dark. Seedling hypocotyls was cut off, the cut surface was inoculated with A. rhizogenes, and shoots without roots were cocultured for 5 days in the dark. To induce hairy root formation, cocultured shoots were grown for 14 days (16 h light/8 h dark) on 1.5% B5 agar medium (Gamborg et al., Citation1968). Plants with green fluorescent protein-positive hairy roots were selected under a fluorescence microscope (SZX9, Olympus, Tokyo, Japan). Selected transformants were inoculated with Mesorhizobium loti MAFF303099 (1.0 × 107 cells), grown on vermiculite, and watered with N-free B&D medium (Broughton & Dilworth, Citation1971) for 28 days (16 h light/8 h dark).
Growth condition
The seeds treated under high humidity condition at 4°C in the dark for 1 week, were soaked in water overnight. Pots were filled with vermiculite and flooded, then seeds were sown.
The 1/5000a Wagener pots were filled with soil, which is mixtured same volume of lawn soil, river sand, and vermiculite, and watered 1 L of B&D liquid medium containing rhizobium, Bradyrhizobium diazoefficiens USDA110. B. diazoefficiens was cultured in YM liquid medium and added to B&D liquid medium at 1.0 × 107 cells/mL. For the comparison of growth with nitrogen, a 6 kg/10a nitrogen was used as 100% (8.57 mM KNO3), and the treatments were given B&D liquid medium containing KNO3 of 0, 50, or 100%.
Two seedlings per pot were transplanted and were grown for 28 days in a glasshouse. Fourteen days after transplanting, the plants watered 1 L of B&D liquid medium without KNO3; otherwise, the pots were placed in a tray containing water and allowed to absorb water from the bottom.
After 28 days of transplanting, shoot length, shoot weight, root weight, nodule number, nodule weight, and nitrogen-fixation activity were determined.
Acetylene-reduction assay for nitrogenase activity
Two plants inoculated with B. diazoefficiens were placed in a 70 mL test tube, which was then covered with a rubber serum cap and degassed for 30 s. Acetylene diluted 1:5 in air was injected into the tube, which was then incubated in a growth chamber at 25°C for 1 h. The amount of ethylene formed was determined by gas chromatography (Suzuki et al., Citation2008; Tominaga et al., Citation2009).
Real-time RT-PCR
Nodules were quickly frozen in liquid N2 and were stored at −80°C. Total RNA was prepared with an RNeasy plant mini kit (Qiagen, Basel, Switzerland). For real-time RT-PCR analysis, a one-step SYBR Primescript RT-PCR kit (Takara, Shiga, Japan) was used. Transcript levels were normalized against the GmActin transcript. The primers used for PCR was below; 5ʹ–ATCTTGACTGAGCGTGGTTA–3ʹ and 5ʹ–GCTGGTCCTGGCTGTCTCC–3ʹ for GmActin and 5ʹ–TTGCAAGCTACTTGGGCATC–3ʹ and 5ʹ–GGCTTTGGTGGTGTTTGGAA–3ʹ for GmSEN1.
X-ray fluorescence analysis
Nodules were sampled from plants at 28 days after inoculation and the fresh weight of root nodules was measured. Then nodules were dried at 65°C for a week. Next, the root nodules were flattened in a vise to reduce the thickness of the nodules as much as possible as a pretreatment for X-ray fluorescence analysis (Rayny EDX-800HS2, Shimadzu). Three measurements were taken on the same sample with each measurement taking 2 min. The average of the three measurements without nodule was used as the blank, and the blank value was subtracted from the value of each sample to obtain the X-ray fluorescence intensity of each sample.
Sequencing analysis for multiple alignment
Amino acid sequences of characterized Vacuolar Iron Transporter (VIT) family members were obtained from NCBI (https://www.ncbi.nlm.nih.gov) or Phytozome (https://phytozome-next.jgi.doe.gov). These included Eucalyptus grandis VIT1 (EgVIT1, XP_010066557.1), Arabidopsis thaliana VIT1 (AgVIT1, AT2G01770) and VTL1 (AtVTL1, AT1G21140), Oryza sativa VIT1 (OsVIT1, LOC_Os09g23300) and VIT2 (OsVIT2, LOC_Os04g38940), Triticum aestivum VIT1 (TaVIT1, Traes_2BL_54954138A.1) and VIT2 (TaVIT2, Traes_5DL_DA6449AC6.1), Medicago truncatula VTL4 (MgVTL4, Medtr4g094325) and VTL8 (MgVTL8, Medtr4g094335), Lotus japonicus SEN1 (LjSEN1, Lj4g0001587), and Glycine max SEN1 (GmSEN1a1 (GmVTL1a), Glyma05g121600, GmSEN1a2, Glyma05g121500, GmSEN1b1 (GmVTL1b), Glyma08g076300 and GmSEN1b2, Glyma08g076200). Multiple alignment was created with CLUSTALO (https://www.ebi.ac.uk/Tools/msa/clustalo/).
NGS analysis
DNA used for whole-genome sequencing analysis was extracted from Enrei type (BC5F5). NGS analysis was performed on our behalf using eurofin (Grand Duchy of Luxembourg). Approximately 35 Gbp sequences were obtained. The reference sequence of Glycine max Wm82.a2.v1 was obtained from phytozome (https://phytozome.jgi.doe.gov). The sequences of Fukuyutaka (GmJMC025) and Enrei (GmJMC112) were obtained from the report of Kajiya-Kanegae et al. (Citation2021). The obtained short reads were mapped to the reference sequences using Bowtie2 (ver. 2.3.5.1), and single-nucleotide polymorphisms (SNPs) were detected using the Bcftools program (ver. 1.10.2). SNPs showing polymorphisms between Fukuyutaka and Enrei were identified. The genotype of BC5F5 was determined based on these SNPs sites, and a graphical genotype of BC5F5 was created using the R commands shown in Supplemental Table S1.
Results
Identification of GmSEN1
Since polymorphisms in LjSEN1 showed the difference of nitrogen fixation ability, it was thought that if polymorphisms exist in GmSEN1, there might be caused result in differences in nitrogen fixation activity. Therefore, we conducted a homology search for the LjSEN1 gene in G. max, and found high homology in two genes, Glyma.05G121600 (GmSEN1a1) and Glyma.08G076300 (GmSEN1b1). Because gene duplication had occurred in these genes during evolution, Glyma.05G121500 (GmSEN1a2) and Glyma.08G076200 (GmSEN1b2) were also included in the genes with high homology to LjSEN1 (Supplemental Fig. S1). All four GmSEN1 belong to the same clade as LjSEN1. Accordingly, these four genes were designated as GmSEN1.
Sequences of the four GmSEN1 genes were compared for 38 soybean cultivars, available at Saga University at that time (). The results showed that in GmSEN1a1, one nucleotide substitution was observed in Argomulyo, Akisengoku, and Indo253, but no amino acid substitution occurred. Similarly, in GmSEN1a2, a single base substitution was observed in Argomulyo, Akisengoku, Aodaizu, Hogyoku, Indo253, Peking, and PI416937, but no amino acid substitution occurred. In GmSEN1b1, there was one base substitution in Enrei and Tachinagaha, both of which were amino acid substitutions from glutamic acid (E) to valine (V). Finally, no differences in nucleotide sequences were detected in all 38 varieties of GmSEN1b2 ().
Table 2. Cultivars in which polymorphisms were detected in GmSEN1.
Based on these results, we next investigated whether the amino acid mutation in GmSEN1b1 affected nitrogen fixation activity and growth. In this study, the standard sequence of GmSEN1b1 is designated as Peking type SEN1 and the mutant sequence of this gene is designated as Enrei type SEN1.
Complementation experiment of Ljsen1–1 mutant by GmSEN1b1
To investigate whether GmSEN1b1, which was defined as one of the GmSEN1 genes in the homology analysis, acts in the same function as LjSEN1, we introduced GmSEN1b1 into the Ljsen1–1 mutant by hairy-roots transformation and investigated whether the phenotype was recovered. In Ljsen1–1 with EV, only a large number of white nodules were formed, while in transformed roots with Peking-type or Enrei-type GmSEN1, not only white nodules but also pink nodules, which are indicators of nitrogen fixation, were observed (). Weight of pink nodule was higher in the Peking type than in the Enrei type ().
Figure 1. Complementation of Ljsen1–1 mutant by GmSEN1 genes. Nodules phenotypes (a), nodule number (b), weight of pink nodule (c), ARA per plant (d), and ARA per pink nodule weight (e) at 28 days after inoculation. Arrows indicate pink nodules. EV; plants introduced with empty vector as negative control. n.d.; not detected. EV, Peking type and Enrei type; n = 20, 26, 21, respectively. Error bars represent standard error (SE). *p < 0.05 and **p < 0.01 by Student’s t-test.
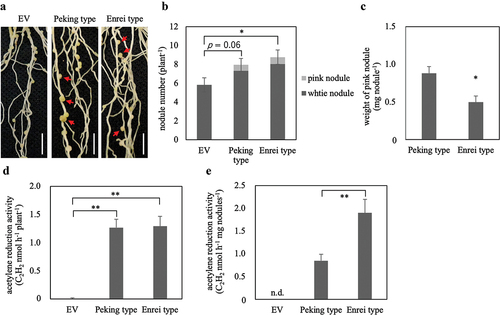
Although no difference was observed between the Peking type and Enrei type on acetylene reduction activity (ARA) per plant, ethylene production was clearly observed (). Furthermore, ARA per pink nodule weight was significantly higher in the Enrei type than in the Peking type (). These results strongly suggest that GmSEN1b1 may have the same function as LjSEN1.
Growth and nitrogen fixation activity in NILs
Using Fukuyutaka, which has the largest cultivated area in Japan, as a background, a NILs of soybean with Enrei type SEN1 were created. In this study, the Enrei type SEN1 lines was obtained by five-time backcrossing with Fukuyutaka to F1, obtained by crossing Enrei and Fukuyutaka, followed by self-pollination three times. The line with Peking type SEN1, obtained by segregation at this self-pollination, was used as a genetic control for comparison.
shows the growth of BC5F4 lines of Peking type and Enrei type grown in a glasshouse for 28 days after inoculation with B. diazoefficiens USDA110. The Enrei type was significantly higher than the Peking type in all the measured parameters except nodule number and ARA per nodule weight (). However, significant growth differences were also observed among NILs lineages (). This was thought to be due to the fact that different NILs caused different growth, and because the trials were conducted in a greenhouse, temperatures varied widely depending on the time of year, which in turn caused large fluctuations in growth. Therefore, the relative growth is shown in , where the growth of Fukuyutaka grown at the same time in each test is set as 100%.
Figure 2. Effects of different genotypes of GmSEN1 on soybean growth and symbiotic parameters. These were measured at 28 days after inoculation with B. diazoefficiens. (a) Fukuyutaka, NILs of Peking type and Enrei type plants. Bars = 5 cm. (b–g) each graph is expressed as a relative value when the value of Fukuyutaka is 100. Relative shoot length (b), relative shoot weight (c), relative root weight (d), relative nodule number (e), relative nodule weight (f), relative ARA per plant (g), and relative ARA per nodule weight (h). Fukuyutaka, Peking type and Enrei type; n = 20, 18, 20, respectively. Error bars represent standard error (SE). Means denoted by the same letter are statistically non-significant at p < 0.05 (tukey-kramer multiple comparison test). (i) Relative GmSEN1b1 gene expression (normalized to that of GmActin as an internal control). Peking type and Enrei type; n = 7. Error bars represent standard error (SE). No statistical difference was observed (student t-test).
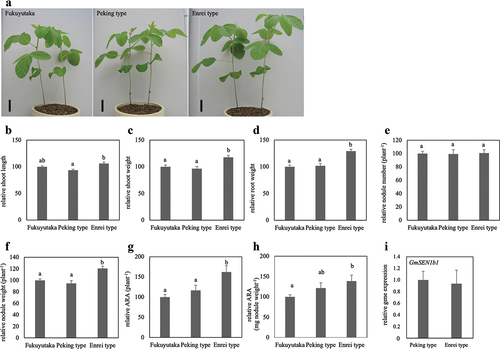
Table 3. Growth of BC5F4 generation progeny at 28 days after inoculation with B. diazoefficiens.
Typical plants of BC5F4 Peking type, Enrei type, and Fukuyutaka grown at the same time after 28 days after inoculation with B. diazoefficiens are shown in , and the growth results of each line were shown in . Shoot length of Enrei type plants were significantly longer than that of Peking type plants (), and Enrei type plants were significantly heavier in shoot and root weight than either Fukuyutaka or Peking type plants (). Although there was no difference in the number of nodules per plant (), Enrei type plants had significantly heavier nodule weight per plant than those of Fukuyutaka and Peking types (). Furthermore, the ARA per plant of the Enrei type was significantly higher than that of the Fukuyutaka and Peking type (). On the other hands, the ARA per nodule weight of Enrei type was significantly higher than that of Fukuyutaka, but not different from that of Peking type ().
The difference in growth between the Enrei type and Peking type could be attributed to differences in gene expression levels. Therefore, the gene expression level of GmSEN1b1 was investigated, and no difference was detected between the Peking type and Enrei type ().
These results indicate that the SEN1 gene of the Enrei type demonstrates higher nitrogen fixation activity than the Peking type, as a result the Enrei type promotes growth.
Multiple amino acid sequence alignment of VIT family and relative iron content of nodules in NILs
Glyma.05G121600 (GmSEN1a1) and Glyma.08G076300 (GmSEN1b1) are homologs of Vacuolar iron Transporter-Like protein (VTL), and it has been reported that these two could complement the yeast iron transporter CCC1 deficient mutant (Brear et al., Citation2020; Liu et al., Citation2020; Ram et al., Citation2021). shows the alignment of VTL and VIT, which has been reported to be involved in iron transport (Che et al., Citation2021; Connorton et al., Citation2017; Kato et al., Citation2019; Walton et al., Citation2020). GmSEN1b1 (VTL1b) shows the amino acid sequence in the Peking type, and the yellow box shows the substitution sites in the Enrei type. Metal Binding Domain (MBD) of VIT is not conserved in VTL, but amino acids reported to be important for iron transport in GmSEN1a1 (GmVTL1a) were highly conserved as shown in the red boxes (, Brear et al., Citation2020). Amino acids involved in ion transport from the MBD to the Transmembrane Domain (TMD), as indicated by the green box, and involved in ion binding, as indicated by the blue box, were highly conserved in VIT, but were less conserved in VTL. In addition, all four GmSEN1 genes were very similar in sequence ().
Figure 3. Multiple amino acid sequence alignment of VIT family and relative iron content of nodules. (a) multiple alignment were created using ClustalO (https://www.ebi.ac.uk/Tools/msa/clustalo/) with amino acid sequences of EgVIT1, AtVIT1, AtVTL1, OsVIT1, OsVIT2, TaVIT1, TaVIT2, MtVTL4, MtVTL8, LjSEN1, GmSEN1a1 (GmVTL1a), GmSEN1a2, GmSEN1b1 (GmVTL1b) and GmSEN1b2. ‘*’ indicates an amino acid conserved in all sequences; ‘:’ indicates conservation between groups of amino acids with strongly similar properties; ‘.’ indicates conservation between groups of amino acids with weakly similar properties. The secondary structure of VIT family indicates above the sequence. Red boxes indicate amino acid reported on Brear et al. (Citation2020) to be required for iron transport in yeast. Green boxes are important for ion translocation from the MBD to the TMD. Blue boxes are involved in ion binding in the MBD (Kato et al., Citation2019). Yellow box indicates the mutation position in Enrei type SEN1. (b) The relative iron content of nodule formed in roots of Peking type and Enrei type. Peking type and Enrei type; n = 30. Bars represent standard error (SE). **p < 0.01 by Student’s t-test.
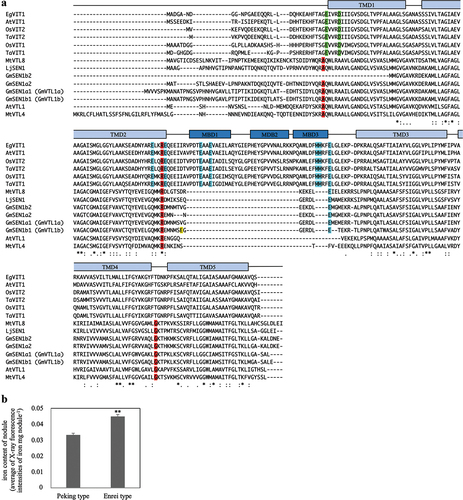
Iron was known to be essential for the active center of nitrogenase, a nitrogen fixation enzyme. To test the possibility that amino acid polymorphisms in GmSEN1b1 are involved in the transport of iron into the nodules, relative iron content in the nodules was measured by X-ray fluorescence using Peking type or Enrei type. The fluorescence intensity per nodule weight of Enrei type was significantly higher than that of Peking type (). These results suggest that Enrei type SEN1 is more effective in increasing the iron content in the nodules than Peking type SEN1, which may have resulted in increased nitrogen fixation activity.
Growth and nitrogen fixation activity in NILs on medium containing various concentrations of nitrogen
shows the relative growth of 28 days after inoculation with B. diazoefficiens under 0, 50, or 100% nitrogen treatments; nitrogen treatment of 100% indicates at 6 kg/10a nitrogen level (8.57 mM KNO3). Because the NIL showed significantly different growth depending on the tested season, the relative growth is shown in , where the growth of Peking type under the 0% nitrogen condition set as 100%. In the 0% nitrogen treatment, the same trend as in was obtained, with higher nitrogen fixation activity per plant and significantly higher shoot weight, root weight, and nodule weight in the Enrei type compared to the Peking type (). However, no significant difference was observed in shoot length, nodule number and ARA per nodule weight, although the Enrei type tended to be higher than the Peking type ().
Figure 4. Relative growth of NILs at 28 days after inoculation with B. diazoefficiens with KNO3. Each graph is expressed as a relative value when the value of Peking type of 0% is 100. Relative shoot length (a), relative shoot weight (b), relative root weight (c), relative nodule number (d), relative nodule weight (e), relative ARA per plant (f), and relative ARA per nodule weight (g). 0% of Peking type and Enrei type; n = 10, 10, 50% of Peking type and Enrei type; n = 10, 10, 100% of Peking type and Enrei type; n = 9, 9, respectively. Error bars represent standard error (SE). *p < 0.05 and **p < 0.01 by Student’s t-test.
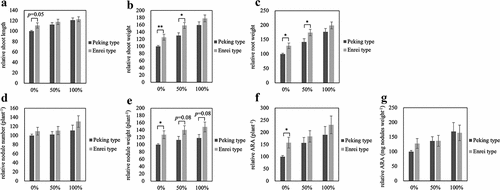
In the 50% nitrogen treatment, shoot and root weights of Enrei type were significantly higher than those of Peking type (), and a trend of higher nodule weight was observed in Enrei type than in Peking type (p = 0.08, ). Other parameters also showed no significant differences, but the Enrei type tended to be higher than the Peking type () except the ARA per nodule weight (). Although there was no significant difference in nodule weight, the Enrei type tended to be higher than the Peking type in the 100% nitrogen treatment (p = 0.08, ). Moreover, the Enrei type tended to be higher in all other parameters than the Peking type (). These results showed that Enrei type SEN1 enhanced nitrogen fixation activity and plant growth in the absence of nitrogen. In addition, it was suggested that Enrei type SEN1 promote plant growth in the presence of nitrogen as well as in the absence of nitrogen.
Furthermore, the Enrei type in the 50% nitrogen treatment showed similar shoot and root weights and nitrogen fixation activity as the Peking type in the 100% nitrogen treatment (). These results suggest that soybean can be grown with half the amount of nitrogen fertilizer applied by introduce Enrei type SEN1.
Genome analysis of NIL
Genome analysis was performed using extracted DNA from the next generation of the Enrei type of BC5F4–5, which showed particularly high growth in the NIL cultivation experiments (). The genome analysis was performed by matching the genome with the whole genome of Williams W82 to detect SNPs, investigating whether each SNP was from Fukuyutaka or Enrei, and creating a graphical genotype from the positions of the SNPs ().
Figure 5. Diagrammatic representation of graphical genotype of Enrei type NIL plant (BC5F5). Yellow boxes indicate chromosome region derived from Fukuyutaka, and red boxes indicate chromosome region derived from Enrei. Black bars indicate SNPs detected between Enrei type NIL and Williams W82.
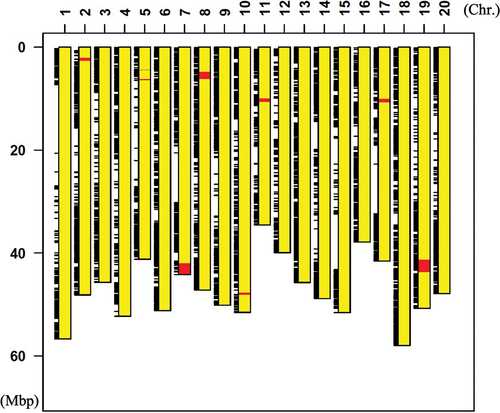
The results showed that we obtained BC5F5 in which approximately 98.6% of the genome was from Fukuyutaka and the remaining 1.4% was from Enrei (). As expected, the neighbourhood of SEN1 on chromosome 8 was Enrei type. In addition, the genotypes of 1.4% Enrei-originating chromosomal regions on chromosomes 7, 8, 11, 17 and 19 were analyzed by high-resolution melting (HRM) assay (Yamagata et al., Citation2018) in Peking type and Enrei type plants of NILs (BC5F6). Both Peking type and Enrei type plants showed the Fukuyutaka homozygous allele in the chromosome 17 region. Other regions (chromosomes 7, 11, and 19) showed segregated genotypes in both Peking type and Enrei type plants. The chromosome 8 region containing the GmSEN1b1 gene showed different genotypes between Peking type and Enrei type plants (Supplementary Table S2). These genotype data strongly support that the difference in nitrogen fixation phenotype between NILs is caused by the difference in GmSEN1b1 alleles.
Discussion
Legumes are known to be able to grow on poor soils compared with other crops, which is thought to be due to symbiotic nitrogen fixation by rhizobia (Ohyama et al., Citation2009; Salvagiotti et al., Citation2008). However, in the cultivation of legume crops such as soybean, nitrogen fertilization is commonly applied because symbiotic nitrogen fixation alone is insufficient to satisfy the nitrogen requirements for plant growth and crop production (Zhang et al., Citation2013). These facts indicate that if symbiotic nitrogen fixation can be enhanced, it may be possible to achieve the same level of growth with reduced nitrogen fertilizer, and/or to increase yield with the same amount of nitrogen fertilizer as before. In other words, improved nitrogen fixation ability is one of the major points of future soybean breeding, and the identification of the loci that affect the enhancement of nitrogen fixation ability is an important issue. However, there are no known examples of increasing nitrogen fixation ability in soybean.
QTL analysis in L. japonicus has reported the existence of a loci showing high nitrogen fixation activity in Miyakojima MG20 (Tominaga et al., Citation2012). Furthermore, Nishida et al. (Citation2020) suggested that SEN1 may be one of the causative genes and reported that MG20 type SEN1 is a nitrogen fixation enhancing gene. These facts suggest that if polymorphisms exist in the orthologous genes of G. max, it may be possible to find enhanced nitrogen fixation gene(s) in G. max, as in the case of L. japonicus. Therefore, we investigated the effects of different genotypes on nitrogen fixation activity and plant growth in the hopes of producing soybean with enhanced nitrogen fixation. An orthologue of LjSEN1 in G. max was identified, and the presence of amino acid polymorphisms in GmSEN1 was clarified (). Furthermore, GmSEN1b1 complemented the Ljsen1–1 mutant, suggesting that GmSEN1b1 has a similar function to LjSEN1 (). In addition, GmSEN1 is highly homologous to the VIT in Eucalyptus, Arabidopsis, rice and wheat (). It was reported that VIT retains five TMDs and three MBDs (Cao, Citation2019; Sorribes-Dauden et al., Citation2020). However, GmSEN1 lacks MBDs and has less conserved amino acids involved in ion transport and binding (). In addition, one of the GmSEN1, GmSEN1a1 has been reported to encode GmVTL1a, which promotes iron transport to bacteroids (Brear et al., Citation2020; Liu et al., Citation2020; Ram et al., Citation2021), and has high homology with MtVTL4 and MtVTL8 have also been reported to be iron transporters localized to the symbiont membrane (Walton et al., Citation2020). Although the MBD is deleted in these VTL homologs, the amino acids reported to be involved in iron binding are highly conserved (Brear et al., Citation2020). Moreover, it is not clear whether the substitution of amino acid in the Enrei type, which is located between the TMD and the deleted MBD, is involved in iron binding; however, the difference in iron content in the nodules between the Peking type and Enrei type suggests that this polymorphism may have altered the efficiency of iron transport and consequently affected nitrogen fixation activity (). Transcripts per million (TPM) results at Soybean Expression Atlas (https://venanciogroup.uenf.br/cgi-bin/gmax_atlas/index.cgi) showed that the four GmSEN1 genes were expressed only in nodules, and the expression levels in GmSEN1a1, GmSEN1a2, GmSEN1b1, and GmSEN1b2 were 10.78, 2.29, 8.99, and 6.48, respectively. In addition, GmSEN1a1 (GmVTL1a) is more highly expressed than GmSEN1b1 (GmVTL1b), and is also thought to be highly active in iron transport (Liu et al., Citation2020). However, no polymorphism was detected in GmSEN1a1, so this study focused on GmSEN1b1. MG20 type SEN1 of L. japonicus was reported to be the genotype that enhanced nitrogen fixation, but the mutation was in the region upstream of the first TMD (Nishida et al., Citation2020). Therefore, it is possible that both polymorphisms of LjSEN1 and GmSEN1b1 enhance nitrogen fixation, but the mechanism of enhancement may not be the same.
When GmSEN1b1 was the Enrei type, nitrogen fixation was enhanced and plant growth was accelerated (). The growth promotion by the different genotypes of SEN1 was more pronounced when the plants were grown under nitrogen-free conditions and less so when nitrogen was present (). Furthermore, the Enrei type in the 50% nitrogen treatment showed similar nitrogen fixation activity and plant growth as the Peking type in the 100% nitrogen treatment (). Soybean yield is known to be affected not only by nitrogen fertilizer supply but also by nitrogen supply through symbiotic nitrogen fixation by rhizobia (Bohlool et al., Citation1992; Salvagiotti et al., Citation2008). In addition, it has been reported that plant growth at maturity, especially shoot weight, tends to correlate with soybean yield (M. Takahashi et al., Citation2003). In conclusion, the use of Enrei type soybean, which showed similar nitrogen fixation activity and plant growth in the 50% nitrogen treatment as the Peking type in the 100% nitrogen treatment, indicates the possibility of maintaining yield even when nitrogen fertilizer application in conventional agriculture is reduced by half. Moreover, Nishida et al. (Citation2020) reported an increase in seed weight in enhanced nitrogen-fixation L. japonicus using MG20 type SEN1. In other words, an increase in yield due to an increase in seed weight can be expected in Enrei type soybean with enhanced nitrogen fixation in this study.
The ARA per nodule weight tended to be higher in the Enrei type than in the Peking type at nitrogen-free condition (), but no difference was observed between the Enrei type and the Peking type in the presence of nitrogen (). On the other hand, in , the Enrei type had lower nodule weight and higher ARA per nodule weight than the Peking type. The effect of amino acid polymorphisms of GmSEN1b1 on nodule weight is currently unknown and will be the subject of future work.
No difference in gene expression of GmSEN1b1 was observed between Peking type and Enrei type (). Moreover, since no nucleotide substitutions were identified between Enrei and Fukuyutaka in the sequence of about 1,800 bp from the translation start point of GmSEN1b1, it is unlikely that there is a large difference in expression between Enrei and Fukuyutaka. Therefore, it is thought that the differences in growth and ARA obtained between Peking type and Enrei type are not due to differences in gene expression. However, because the four GmSEN1 genes are very similar (), it is possible that the other three genes may also be detected, and further detailed analysis by RNA-seq is needed in the future.
It was predicted by calculation that 98.4% of the chromosomes of the resulting plants would be derived from the parental lines by repeating the backcrossing five times, and the Enrei type soybean produced in this study was almost in accordance with the theoretical value, as shown in , where approximately 98.6% of the chromosomes were derived from Fukuyutaka. This fact suggests that Enrei type soybean has almost the same characteristics as Fukuyutaka. Moreover, the results of the HRM assay (Supplemental Table S2) strongly support that the effect of enhanced nitrogen fixation is caused by the region of chromosome 8 containing the Enrei type SEN1 allele.
Soybean has relatively slow initial growth, but vigorous growth of this initial growth may ensure photosynthesis and increase yield. Therefore, in this study, we compared the growth of plants 28 days after inoculation with rhizobia. Future research should be conducted to determine if it is possible to increase yield by using Enrei type soybean with enhanced nitrogen fixation, and to what extent nitrogen fertilization can be reduced to obtain the same level of yield as now.
Supplemental Material
Download MS Word (20.8 KB)Supplemental Material
Download MS Word (21.1 KB)Supplemental Material
Download TIFF Image (21 MB)Disclosure statement
No potential conflict of interest was reported by the author(s).
Supplementary material
Supplemental data for this article can be accessed online at https://doi.org/10.1080/1343943X.2024.2326643
Additional information
Funding
References
- Aloo, F., & Ojija, B. N. (2023). The role of Rhizobia toward food production, food and soil security through microbial agro-input utilization in developing countries. Case Studies in Chemical and Environmental Engineering, 8, 100404. https://doi.org/10.1016/j.cscee.2023.100404
- Bohlool, B. B., Ladha, J. K., Garrity, D. P., & George, T. (1992). Biological nitrogen fixation for sustainable agriculture: A perspective. Plant and Soil, 141(1–2), 1–11. https://doi.org/10.1007/BF00011307
- Bosse, M. A., da Silva, M. B., de Oliveira, N. G. R. M., de Araujo, M. A., Rodrigues, C., de Azevedo, J. P., & Dos Reis, A. R. (2021). Physiological impact of flavonoids on nodulation and ureide metabolism in legume plants. Plant Physiology and Biochemistry, 166, 512–521. https://doi.org/10.1016/j.plaphy.2021.06.007
- Brear, E. M., Bedon, F., Gavrin, A., Kryvoruchko, I. S., Torres‐Jerez, I., Udvardi, M. K., Smith, P. M. (2020). GmVTL1a is an iron transporter on the symbiosome membrane of soybean with an important role in nitrogen fixation. New Phytologist, 228(2), 667–681. https://doi.org/10.1111/nph.16734
- Broughton, W. J., & Dilworth, M. (1971). Control of leghaemoglobin synthesis in snake beans. Biochemical Journal, 125(4), 1075–1080. https://doi.org/10.1042/bj1251075
- Cao, J. (2019). Molecular evolution of the vacuolar iron transporter (VIT) family genes in 14 plant species. Genes (Basel), 10(2), 144. https://doi.org/10.3390/genes10020144
- Che, J., Yamaji, N., & Ma, J. F. (2021). Role of a vacuolar iron transporter OsVIT2 in the distribution of iron to rice grains. New Phytologist, 230(3), 1049–1062. https://doi.org/10.1111/nph.17219
- Connorton, J. M., Jones, E. R., Rodríguez-Ramiro, I., Fairweather-Tait, S., Uauy, C., & Balk, J. (2017). Wheat vacuolar iron transporter TaVIT2 transports fe and mn and is effective for biofortification. Plant Physiology, 174(4), 2434–2444. https://doi.org/10.1104/pp.17.00672
- Gamborg, O. L., Miller, R., & Ojima, K. (1968). Nutrient requirements of suspension cultures of soybean root cells. Experimental Cell Research, 50(1), 151–158. https://doi.org/10.1016/0014-4827(68)90403-5
- Gao, M., Qiu, J., Li, C., Wang, L., Li, H., & Gao, C. (2014). Modeling nitrogen loading from a watershed consisting of cropland and livestock farms in China using manure-DNDC. Agriculture. Agriculture, Ecosystems & Environment, 185, 88–98. https://doi.org/10.1016/j.agee.2013.10.023
- Hakoyama, T., Niimi, K., Watanabe, H., Tabata, R., Matsubara, J., Sato, S., Suganuma, N. (2009). Host plant genome overcomes the lack of a bacterial gene for symbiotic nitrogen fixation. Nature, 462(7272), 514–517. https://doi.org/10.1038/nature08594
- Hakoyama, T., Niimi, K., Yamamoto, T., Isobe, S., Sato, S., Nakamura, Y., Suganuma, N. (2012). The integral membrane protein SEN1 is required for symbiotic nitrogen fixation in lotus japonicus nodules. Plant and Cell Physiology, 53(1), 225–236. https://doi.org/10.1093/pcp/pcr167
- Hasibuan, R. F. M., Miyatake, M., Sugiura, H., Agake, S. I., Yokoyama, T., Bellingrath-Kimura, S. D., Ohkama-Ohtsu, N. (2021). Application of biofertilizer containing bacillus pumillus TUAT1 on soybean without inhibiting infection by Bradyrhizobium diazoefficiens USDA110. Soil Science and Plant Nutrition, 67(5), 535–539. https://doi.org/10.1080/00380768.2021.1959837
- Hossain, M. S., Umehara, Y., & Kouchi, H. (2006). A novel fix symbiotic mutant of Lotus japonicus, Ljsym105, shows impaired development and premature deterioration of nodule infected cells and symbiosomes. Molecular Plant-Microbe Interactions, 19(7), 780–788. https://doi.org/10.1094/MPMI-19-0780
- Kajiya-Kanegae, H., Nagasaki, H., Kaga, A., Hirano, K., Ogiso-Tanaka, E., Matsuoka, M., Iwata, H. (2021). Whole-genome sequence diversity and association analysis of 198 soybean accessions in mini-core collections. DNA Research, 28(1), dsaa032. https://doi.org/10.1093/dnares/dsaa032
- Kato, T., Kumazaki, K., Wada, M., Taniguchi, R., Nakane, T., Yamashita, K., Nureki, O. (2019). Crystal structure of plant vacuolar iron transporter VIT1. Nature Plants, 5(3), 308–315. https://doi.org/10.1038/s41477-019-0367-2
- Kawaguchi, M., Imaizumi-Anraku, H., Koiwa, H., Niwa, S., Ikuta, A., Syono, K., & Akao, S. (2002). Root, root hair, and symbiotic mutants of the model legume Lotus japonicus. Molecular Plant-Microbe Interactions, 15(1), 17–26. https://doi.org/10.1094/MPMI.2002.15.1.17
- Klein, M. A., Grusak, M. A., & Francki, M. (2009). Identification of nutrient and physical seed trait QTL in the model legume Lotus japonicus. Genome, 52(8), 677–691. https://doi.org/10.1139/G09-039
- Krusell, L., Krause, K., Ott, T., Desbrosses, G., Kramer, U., Sato, S., Udvardi, M. K. (2005). The sulfate transporter SST1 is crucial for symbiotic nitrogen fixation in lotus japonicus root nodules. The Plant Cell, 17(5), 1625–1636. https://doi.org/10.1105/tpc.104.030106
- Kumagai, H., Hakoyama, T., Umehara, Y., Sato, S., Kaneko, T., Tabata, S., & Kouchi, H. (2007). A novel ankyrin-repeat membrane protein, IGN1, is required for persistence of nitrogen-fixing symbiosis in root nodules of Lotus japonicus. Plant Physiology, 143(3), 1293–1305. https://doi.org/10.1104/pp.106.095356
- Liu, S., Liao, L. L., Nie, M. M., Peng, W. T., Zhang, M. S., Lei, J. N., Chen, Z. C. (2020). A VIT‐like transporter facilitates iron transport into nodule symbiosomes for nitrogen fixation in soybean. New Phytologist, 226(5), 1413–1428. https://doi.org/10.1111/nph.16506
- Long, S. R. (1989). Rhizobium-legume nodulation: Life together in the underground. Cell, 56(2), 203–214. https://doi.org/10.1016/0092-8674(89)90893-3
- Maekawa, T., Kusakabe, M., Shimoda, Y., Sato, S., Tabata, S., Murooka, Y., & Hayashi, M. (2008). Polyubiquitin promoter-based binary vectors for overexpression and gene silencing in lotus japonicus. Molecular Plant-Microbe Interactions, 21(4), 375–382. https://doi.org/10.1094/MPMI-21-4-0375
- Mylona, P., Pawlowski, K., & Bisseling, T. (1995). Symbiotic nitrogen fixation. The Plant Cell, 7(7), 869. https://doi.org/10.2307/3870043
- Nishida, Y., Hiraoka, R., Kawano, S., Suganuma, N., Sato, S., Watanabe, S., Suzuki, A. (2020). SEN1 gene from Lotus japonicus MG20 improves nitrogen fixation and plant growth. Soil Science and Plant Nutrition, 66(6), 864–869. https://doi.org/10.1080/00380768.2020.1834829
- Ohyama, T., Ohtake, N., Sueyoshi, K., Tewari, K., Takahashi, Y., Ito, S., Nishiwaki, T., Nagumo, Y., Ishii, S., & Sato, T. (2009). Nitrogen fixation and metabolism in soybean plants. Nova Science Publishes, IncNitrogen Fixation Research Progres.
- Ram, H., Sardar, S., & Gandass, N. (2021). Vacuolar iron transporter (like) proteins: Regulators of cellular iron accumulation in plants. Physiologia Plantarum, 171(4), 823–832. https://doi.org/10.1111/ppl.13363
- Salvagiotti, F., Cassman, K. G., Specht, J. E., Walters, D. T., Weiss, A., & Dobermann, A. (2008). Nitrogen uptake, fixation and response to fertilizer N in soybeans: A review. Field Crops Research, 108(1), 1–13. https://doi.org/10.1016/j.fcr.2008.03.001
- Schauser, L., Roussis, A., Stiller, J., & Stougaard, J. (1999). A plant regulator controlling development of symbiotic root nodules. Nature, 402(6758), 191–195. https://doi.org/10.1038/46058
- Seefeldt, L. C., Hoffman, B. M., & Dean, D. R. (2009). Mechanism of Mo-dependent nitrogenase. Annual Review of Biochemistry, 78(1), 701–722. https://doi.org/10.1146/annurev.biochem.78.070907.103812
- Shi, Z., Zhang, Y., Zhou, J., Chen, M., & Wang, X. (2013). Biological removal of nitrate and ammonium under aerobic atmosphere by Paracoccus versutus LYM. Bioresource Technology, 148, 144–148. https://doi.org/10.1016/j.biortech.2013.08.052
- Sorribes-Dauden, R., Peris, D., Martínez-Pastor, M. T., & Puig, S. (2020). Structure and function of the vacuolar Ccc1/VIT1 family of iron transporters and its regulation in fungi. Computational and Structural Biotechnology Journal, 18, 3712–3722. https://doi.org/10.1016/j.csbj.2020.10.044
- Subbarao, G. V., Kishii, M., Nakahara, K., Ishikawa, T., Ban, T., Tsujimoto, H., Ito, O. (2009). Biological nitrification inhibition (BNI)—Is there potential for genetic interventions in the Triticeae? Breeding Science, 59(5), 529–545. https://doi.org/10.1270/jsbbs.59.529
- Suganuma, N., Nakamura, Y., Yamamoto, M., Ohta, T., Koiwa, H., Akao, S., & Kawaguchi, M. (2003). The lotus japonicus Sen1 gene controls rhizobial differentiation into nitrogen-fixing bacteroids in nodules. Molecular Genetics and Genomics, 269(3), 312–320. https://doi.org/10.1007/s00438-003-0840-4
- Suzuki, A., Yamashita, K., Ishihara, M., Nakahara, K. I., Abe, M., Kucho, K. I., Arima, S. (2008). Enhanced symbiotic nitrogen fixation by Lotus japonicus containing an antisense β-1, 3-glucanase gene. Plant Biotechnology, 25(4), 357–360. https://doi.org/10.5511/plantbiotechnology.25.357
- Takahashi, M., Arihara, J., Nakayama, N., & Kokubun, M. (2003). Characteristics of growth and yield formation in the improved genotype of supernodulating soybean (glycine max L. Merr.). Plant Production Science, 6(2), 112–118. https://doi.org/10.1626/pps.6.112
- Takahashi, Y., Chinushi, T., Nakano, T., & Ohyama, T. (1992). Evaluation of N2 fixation and N absorption activity by relative ureide method in field-grown soybean plants with deep placement of coated urea. Soil Science and Plant Nutrition, 38(4), 699–708. https://doi.org/10.1080/00380768.1992.10416700
- Tominaga, A., Gondo, T., Akashi, R., Zheng, S. H., Arima, S., & Suzuki, A. (2012). Quantitative trait locus analysis of symbiotic nitrogen fixation activity in the model legume Lotus japonicus. Journal of Plant Research, 125(3), 395–406. https://doi.org/10.1007/s10265-011-0459-1
- Tominaga, A., Nagata, M., Futsuki, K., Abe, H., Uchiumi, T., Abe, M., Suzuki, A. (2009). Enhanced nodulation and nitrogen fixation in the abscisic acid low-sensitive mutant enhanced nitrogen fixation1 of Lotus japonicus. Plant Physiology, 151(4), 1965–1976. https://doi.org/10.1104/pp.109.142638
- Tsujimoto, R., Kotani, H., Yokomizo, K., Yamakawa, H., Nonaka, A., & Fujita, Y. (2018). Functional expression of an oxygen-labile nitrogenase in an oxygenic photosynthetic organism. Scientific Reports, 8(1), 7380. https://doi.org/10.1038/s41598-018-25396-7
- Walton, J. H., Kontra‐Kováts, G., Green, R. T., Domonkos, Á., Horváth, B., Brear, E. M., Balk, J. (2020). The Medicago truncatula Vacuolar iron Transporter‐Like proteins VTL4 and VTL8 deliver iron to symbiotic bacteria at different stages of the infection process. New Phytologist, 228(2), 651–666. https://doi.org/10.1111/nph.16735
- Win, K. T., Oo, A. Z., Ohkama-Ohtsu, N., & Yokoyama, T. (2018). Bacillus pumilus strain TUAT-1 and nitrogen application in nursery phase promote growth of rice plants under field conditions. Agronomy, 8(10), 216. https://doi.org/10.3390/agronomy8100216
- Yamagata, Y., Yoshimura, A., Anai, T., & Watanabe, S. (2018). Selection criteria for SNP loci to maximize robustness of high-resolution melting analysis for plant breeding. Breeding Science, 68(4), 488–498. https://doi.org/10.1270/jsbbs.18048
- Zhang, X. N. A. U., Huang, G. N. A. U., Bian, X. N. A. U., & Zhao, Q. C. A. O. (2013). Effects of root interaction and nitrogen fertilization on the chlorophyll content, root activity, photosynthetic characteristics of intercropped soybean and microbial quantity in the rhizosphere. Soil and Environment, 59(2), 80–88. https://doi.org/10.17221/613/2012-PSE
- Zhang, Y., Xu, Q., Wang, G., & Shi, K. (2023). Mixed enterobacter and klebsiella bacteria enhance soybean biological nitrogen fixation ability when combined with rhizobia inoculation. Soil Biology and Biochemistry, 184, 109100. https://doi.org/10.1016/j.soilbio.2023.109100