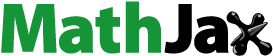
ABSTRACT
The infertility of sandy soil hampers the growth of many crops. Typically, chemical fertilizers are used to handle this issue, but they are expensive and have deleterious implications on the ecosystem. A two-year field investigation was carried out on cowpea cultivated in sandy soil to assess the effects of different watering levels (70, 80, 90, and 100% of the actual irrigation requirements), chemical fertilizers, organic fertilizers, and biofertilizer (Bradyrhizobium sp.), and their combinations on the morpho-physiological traits and yield of cowpea (Vigna unguiculata L. Walp, cv. Giza 18). Overall, the findings showed that cowpea osmoregulatory molecules (sugars, proteins, and proline) demonstrated greater accumulation, with the content depending on the watering level. The applied fertilizers demonstrated improved cowpea performance at the regular watering level, with chopped potato tops outperforming other fertilizer formulations. Furthermore, the combination of water stress treatments and fertilizer formulations was impactful in relieving the detrimental consequences of water stress as it enhanced plant growth performance, production efficiency, metabolic homeostasis, nutritional value, and mineral ions composition. Subsequently, our results demonstrated agro-wastes, particularly chopped potato tops, could be an effective substitute for chemical fertilizers in sandy soils to sustainably increase soil fertility as well as cowpea yield performance.
Introduction
Cowpea (Vigna unguiculata L. Walp) is a legume crop from the Fabaceae, that is widely cultivated across the tropics and subtropics of the world. It is an annual crop that can withstand water and salt stresses and is well suited to a variety of soil types and climatic conditions (Osipitan et al., Citation2021). It is a staple and versatile food legume across many African communities, where it is consumed as young leaves, immature pods, and dry seeds. Also, the entire plant can be used to feed livestock (Alemu et al., Citation2016). The economic value of cowpea is derived from its high nutritional value, as it contains high protein and fiber contents with low fats. Its seeds comprise 17 amino acids, some of which are essential like valine, lysine, leucine, and phenylalanine. Many of these amino acids are major constituents in livestock feeding and poultry growth. Additionally, cowpea leaves are incredibly valuable economically and nutritionally, offering a reasonable source of protein, vitamins (A, C, and B complex), and many essential nutrients, that could contribute to reducing human food insecurity and malnutrition (Gerrano et al., Citation2019). Cowpea cultivation enhances soil characteristics in the agricultural system by compensating for the nitrogen lost by cereals plantation. This is a result of its great ability to fix nitrogen through its symbiotic root nodules, which allows it to thrive even in nutrient-poor soils (Mndzebele et al., Citation2020).
In comparison to other crop species, cowpea was reported as a relatively drought-tolerant species, however its growth and yield can be deteriorated by water shortage (Nunes et al., Citation2019). The consequences of water stress on cowpea vary depending on the severity of the stress and the development stages of plants that the water stress occurs. Typically, the yield is substantially devastated when drought occurs during the reproductive and seed-filling stages (Nunes et al., Citation2022). Due to the difference in the dry matter between vegetative and reproductive organs, varied deficit irrigation timings and intensities throughout different growth phases may boost cowpea yield (Aboamera, Citation2010). Since cowpea is a row crop (planted in rows wide enough to be tilled or tended by agricultural machines), drip irrigation is the best method for growing it (Faloye & Alatise, Citation2015). This will ensure that the water is applied consistently, improving soil moisture homogeneity and water use efficiency, both of which will significantly boost productivity in periods of water shortage (Ortuani et al., Citation2019). Additionally, drip irrigation reduces energy requirements while also saving water and nutrients (Varughese et al., Citation2014). In the current time, a significant issue in the sector of agriculture pertains to the effective utilization of limited water resources for improved crop productivity. One potential technique for addressing this challenge involves the implementation of an integrated approach that incorporates deficit irrigation in conjunction with a drip irrigation system (Topak et al., Citation2016). Deficit irrigation, which refers to the practice of applying water below the level required for optimal crop growth, is a significant strategy employed to effectively reduce irrigation water consumption. This approach is frequently implemented in arid and semi-arid areas to enhance water use efficiency (WUE) (Shahrokhnia & Sepaskhah, Citation2016). Deficit irrigation enhances WUE by reducing irrigation episodes that have no influence on crop productivity. Nevertheless, it is worth noting that there are other advantages associated with this practice, such as the mitigation of nutrient leaching, the reduction of energy expenses in irrigation processes, the enhancement of the agricultural sector’s competitiveness, as well as the reduction of production costs (Falagán et al., Citation2015).
Chemical fertilizers are a major part of modern agriculture and are used to increase crop productivity. Nonetheless, the challenges associated with chemical fertilizers lie not in the fertilizers themselves but rather in their application methods. The ecosystem, the soil, and human health are all adversely affected by these fertilizers over time. Furthermore, frequent application of chemical fertilizers results in increased soil hardening, decreased soil organic matter, and a significant decline in major mineral nutrients. Additionally, it results in a weak microbial community and an increase in pathogenesis opportunities (Pahalvi et al., Citation2021). The agriculture system transition from chemical to organic mode must conserve the soil and ecosystems for a longer period of time and avoid or limit the negative impacts of chemical fertilizers (Verma et al., Citation2020). The term ‘organic fertilizer’ refers to a by-product from plant or animal origin that has sufficient nutrients to be used in crop fertigation (Fageria, Citation2012). The C/N ratio of the soil is balanced with the use of organic fertilizers, which also improves soil fertility and productivity. The nutrients in the deep layers of the soil become accessible to plants, particularly legume plants, because of increased biological activity in the soil (Sofi et al., Citation2018).
Crop leftovers are a valuable source of organic fertilizers, which helps in improving the characteristics of the soil. Subsequently, by enhancing soil aggregation, promoting soil hydraulic conductivity, and lowering mechanical resistance and bulk density, organic fertilizers can improve the physical characteristics of the soil (Bhattacharyya et al., Citation2007). However, because organic fertilizers have a relatively poor nutrient composition and a slow rate of nutrient release, they are insufficient to satisfy crop needs easily. Therefore, applying organic fertilizers by themselves is not sufficient to support the efficient agricultural productivity required in the usual farming environment (Sun et al., Citation2015). In addition to enhancing soil fertility through many cropping systems, the combined application of plant residues and inorganic fertilizers could significantly reduce the negative nutrient balance (Sarkar et al., Citation2020). As a result, the plant benefits from higher nitrogen content from chemical fertilizers and enhanced carbon supply from organic fertilizers. Nevertheless, the current body of research lacks sufficient data on the correlation between irrigation management and fertilizer management and their impact on cowpea productivity. To the authors’ knowledge, no studies have been conducted thus far to evaluate the efficacy of various fertilizer formulations in mitigating the adverse effects of drought stress on cowpea plants. We are interested in investigating whether interactive management strategies can effectively mitigate the negative impacts of drought on cowpea plants. Specifically, we seek to understand the specific adaptation mechanisms employed by these management strategies to reduce drought-induced deteriorations. We pursue to determine whether these mechanisms involve maintaining adequate soil moisture levels during drought conditions, providing drought-resistant phytochemicals to the plants, stimulating a specific defense mechanism, or implement other related mechanisms. The findings of this study will provide valuable insights into the complex interactions between irrigation management and fertilizer management and their combined influence on cowpea production. The results can inform farmers, agronomists, and policymakers on the importance of integrated management practices to optimize crop yields and promote sustainable agricultural systems. Therefore, the purpose of this study is to determine how well cowpea performs under water stress conditions in newly reclaimed sandy soil using a surface drip irrigation system, as well as how it responds to different fertilizer formulations such as chemical, bio, and organic fertilization methods in terms of growth, yield, and biochemical response. As there are concerns about the impact of climate change on agricultural production in the future, this manuscript is expected to contribute to this issue.
Materials and methods
Study site and soil properties
The effectiveness of soil amendment with potato tops as an organic fertilizer and Bradyrhizobium sp. (strain 169) as a biofertilizer, along with chemical fertilizer, under various irrigation regimes using the surface drip irrigation system on the growth, yield and biochemical attributes of cowpea plant was investigated in a field experiment conducted in a sandy soil during the summer season of two successive years (May of 2021 and 2022) at Qalabshu Agricultural Research Station, Gamasa District, El-Dakahlia Governorate, Egypt (31.40N, 31.37E). Weather data of the experimental site are provided in Table S1.
Prior experiment setup, samples from the study site soil were acquired at random from two subsequent depths (0–15 and 15–30 cm) below the soil surface to evaluate some of the mechanical, physical and chemical characteristics of the soil (Alvarenga et al., Citation2008) (). Potato variety sponta, which is employed as the organic fertilizer in this experiment, was the preceding crop that is grown in the study soil. According to the procedures established by El-Serafy and El-Ghamry (Citation2006), chemical characteristics of vegetative potato tops were analyzed and displayed in . For determining the chemical characteristics of the used irrigation water, 500 ml pre-cleaned polyethylene bottles were used to collect water against the current’s flow. The collected irrigation water was analyzed based on the (APHA, Citation2001) method ().
Table 1. Physical and chemical characteristics of the study soil during the growing seasons (2021 and 2022).
Table 2. Chemical composition of vegetative potato tops.
Table 3. Chemical analysis of irrigation water.
Soil preparation and experiment setup
The soil of the study site was prepared as follows: a mounted rotary plough with 24 L-shaped blades that are positioned on the overall machine width was used to perform minimum tillage at a depth of 0.15 m. The tractor’s power take-off (PTO) was turned on at a speed of 540 rpm to achieve the rotary speed of 145 rpm.
Prior to the ploughing process, the study site was designed as a series of plots with a total area of 12 m2. Three different types of treatments were applied to the plots: control plots free of any amendments, potato tops (all the aerial parts left over after potato tubers collection) as an organic fertilizer, and NPK as a chemical fertilizer. Potato tops were introduced into the soil with the rate of 23 ton ha−1 (as organic fertilizer) in two different forms: whole, uncut potato tops, or potato tops that had been chopped into particles of 3 mm size using a mounted forage chopper. The chemical fertilizer in the form of NPK [200 kg NH₄NO₃ ha−1, 150 kg ha−1 KNO3 and 100 kg ha−1 Ca(H2PO4)2] was added after the seedling establishment, according to the recommended dose for cowpea.
The seeds of cowpea (Vigna unguiculata cv. Giza 18) were separated into two portions; one portion had no any treatment at all, and the other portion received an inoculation with Bradyrhizobium sp. (strain 169) as a biofertilizer with a rate of 0.96 kg ha−1 (obtained from the Production Unit for Biofertilizers at the Agricultural Research Center, Giza, Egypt, with a loading rate of 10−7 to 10−8 cells/g on vermiculite substrate). Cowpea seeds and the inoculum were combined for seed inoculation, and Arabic gum was administered to promote effective adherence. To prevent bacterial exposure to the sun, the inoculated seeds were air-dried in the shade for 30 min before being directly placed in the soil.
Cowpea seeds were sown in the plots at a rate of 23.8 kg ha−1. The seeds were planted 3 cm deep in the soil, with 60 cm between rows and 15 cm between hills. The seeds were sown on two different planting dates (NaN Invalid Date NaNand NaN Invalid Date NaNfor the first and the second season, respectively). The plots were categorized as untreated plots, plots with complete potato tops, plots with chopped potato tops, plots with chemical fertilizer, and plots with biofertilizer.
Using the formula given by Dukes et al. (Citation2012), the total irrigation water (TIW) was determined (m3 h−1) as follows:
Where, LR is the leaching requirements (m3 ha−1); CR is the crop water requirements (m3 ha−1); η is irrigation system efficiency (%) and A is the irrigated area (ha).
Where K1 and K2 represent the emitter uniformity and the drip irrigation efficiency coefficients, respectively.
Following seed sowing, the plots were irrigated at 70, 80, 90, and 100% of the actual irrigation needs for cowpea (2633.3 m3 ha−1). A surface drip irrigation system was used to implement irrigation treatments. The installed irrigation network included a centrifugal pump of 45 m3 h−1 discharge, pressure regulator, pressure gauges, flow meter, and control valves. The lateral lines of the network were 60 cm line spacing with GR-type emitters with a nominal discharge of 4 L h−1 at 150 kPa operating pressure.
Treatments and experimental design
The experiment layout was prepared as a split-plot design (SPD) with three replications per treatment. The main plots presented water regimes based on deficit irrigation treatments that encompassed levels of 70, 80, 90, and 100% of the actual cowpea irrigation requirements. The different fertilization techniques were demonstrated as the sub-plots. In addition to the control sub-plot, which received no fertilization, it contained fully conformed and chopped potato tops, as well as chemical and biological fertilizers. All other agricultural practices were performed in accordance with the recommendations of the Egyptian Ministry of Agriculture and Land Reclamation (Malr, Citation2018). Plants were harvested at 50 days old, and the plant height as well as dry biomass productivity were calculated. The plant height was determined by measuring the length in cm from the soil surface with a measuring tape. The obtained samples were then dried in a hot-air-forced oven at 60°C until a constant weight was reached, and the dry biomass (kg ha−1) was estimated. Then after, plants were allowed to grow till maturity under the specified experimental conditions, harvested in the first week of October, and the yield parameters were then evaluated. For this, the two middle rows of each experimental plot were harvested, and the yield parameters were then recorded. The measurements for number of pods per plant, number of seeds per pod, and weight of 100 seeds (g) were taken from an average of 50 mature pods from 10 randomly chosen plants. Subsequently, the seed yield (kg ha−1) was determined.
More details about weather conditions during the growing season over the two years are provided in Table S1.
Chemical analysis
To assess the cowpea’s capacity to endure water stress conditions across various fertigation techniques, the resulting plants were examined for osmomodulatory molecules, including total soluble sugars, total proteins, and proline. Additionally, the nutritional value of the plants was evaluated through the analysis of crude fibers and ash contents. After being harvested, the aerial parts (straw) of cowpea plants were allowed to dry naturally for five days before being dried in a vacuum oven at 60°C for three days. The dried straw was pulverized utilizing an electrical mixture, passed through a 2 mm sieve, and stored in paper bags for further analysis.
Total soluble sugars from cowpea leaf powders were obtained by immersing them in 80% ethanol at 25°C overnight, intermittently agitating, and centrifuging the mixture at 1200 g for 20 min. Total soluble sugars in the supernatant were measured spectrophotometrically employing the method of Dubois et al. (Citation1965).
The total protein content of dry cowpea leaves was determined according to the method of Bradford (Citation1976). Using the Kjeldahl digestion unit, the dried powders were digested using nitric acid and H2O2. The digested mixture was neutralized with 4 N NaOH, and bovine serum albumin was employed as a reference protein to calculate the total protein content.
Using the approach outlined by Bates et al. (Citation1973), the proline content of cowpea leaf powders was estimated. Leaf powders were extracted with aqueous sulfosalicylic acid (3%), combined with the ninhydrin reagent, heated for 10 min at 100°C, cooled, and the absorbance was recorded at 520 nm. The proline content of cowpea leaves was assessed using a proline-created standard curve.
For the determination of crude fibers content (as a measure of the nutritional value), cowpea leaf powders were defatted with petroleum ether, heated with 1.25% H2SO4 under reflux for 30 min., and then filtered. The residue was repeatedly rinsed with distilled water before being heated in 1.25% NaOH for another 30 min under reflux. The residue was repeatedly rinsed with distilled water, dried at 100°C to a consistent weight, and the crude fibers were calculated (AOAC, Citation2010).
To determine the amount of ash (to ensure cowpea aerial parts safety as fodder), the dry leaf powders were weighed in a pre-weighed crucible, put in a muffle furnace, and completely ignited at 550°C for 8–12 h. The crucible was then weighed again after cooling in a desiccator with CaCl2 pellets at ambient temperature, and the ash percentage was determined (AOAC, Citation2010).
Mineral analysis
Nutrient availability depends on soil inorganic and organic components, plant vigor, soil conditions, and environmental factors. A porcelain crucible containing a 1.0 g sample of powdered leaf tissues was heated overnight at 500°C. The ash was then dissolved in 5 mL of HCI, and it was then heated for 30 min. at 50°C. The extract was then mixed with 35 mL of deionized water and filtered through Whatman #1 filter paper. An inductively coupled plasma (ICP) emission spectrophotometer (Polyscan 61E, Thermo Jarrell-Ash Corp., Franklin, MA, USA) was used to determine total nitrogen (N), phosphorus (P), and potassium (K) contents in leaf extracts (Hoenig et al., Citation1998).
Data analysis
The experiment year was treated as a random factor, so all experimental findings were averaged and assessed over two years. ANOVA in the general linear model was performed to quantify and appraise the results utilizing Minitab 19.11 (GLM) software. Constant factors involved four irrigation rates and five fertilizer formulations, whereas dependent variables included all of the assessed traits. Tukey’s HSD test was used as a posthoc test in the pairwise mode to determine whether there were any significant variations in the interaction implications. p value less than 5% was considered to be statistically significant.
Results and discussion
Two-way ANOVA of the investigated variables
The output of the two-way ANOVA of irrigation levels, fertilizer formulations, and their combinations on the agronomic parameters of cowpea in a two-field experiment performed in 2021 and 2022 are represented in . The results demonstrated that irrigation levels exhibited a very highly significant (p < 0.001) effect on all of the examined attributes, except for the nitrogen content which was non-significant (p > 0.5). However, all of the investigated attributes were very highly significantly impacted by the kind of applied fertilizer except for the significant (p < 0.1) effect on the nitrogen content. However, there were no statistically significant variations in the plant height, dry biomass, the weight of 100 seeds, ash content, or nitrogen concentration for the interaction between the irrigation levels and the fertilizers type. While the interactions between these treatments produced a highly significant impact on the number of seeds per pod and crude fibers content, the other variables responded to these interactions in a very highly significant manner.
Table 4. Two-way ANOVA analysis of the studied variables.
Yield attributes
(and Table S2) provide an overview on the impact of various irrigation rates (70–100%), fertilizer types (chemical, bio, and organic), and their interactions on the yield characteristics of cowpea grown in sandy soil. In comparison to the fully irrigated control, the lowest irrigation level (70%) led to reductions of 11.9, 66.0, 38.8, and 17.4% in number of pods per plant, number of seeds per pod, the weight of 100 seeds, and the total seed yield, respectively.
Table 5. Yield attributes of cowpea as affected by various irrigation levels, fertilizer types, and their interactions.
Additionally, there were notable differences between cowpea yield parameters according to the type of fertilizer formulation used. Under non-drought conditions, the cowpea yield increased with each fertilizer formulation that was applied compared to the non-fertigated control. The order of the fertilizers’ impact on cowpea yield was as follows: chopped potato tops, whole potato tops, the biofertilizer (Bradyrhizobium sp.), and then the chemical fertilizer (NPK). The decrement in cowpea yield attributes caused by insufficient watering dosages was effectively mitigated by the interactions of the different fertilizers and watering dosages, with chopped potato tops outperforming the other formulations at all watering levels.
The potato agro-wastes contain organic matter, organic carbon, nitrogen, phosphorus, and potassium, which may have been released to the soil to improve the supply of nutrients to roots for plant uptake, as well as enhanced soil physical properties and water holding capacity, which enhanced cowpea growth and yield under water stress. This may account for the improved yield attributes after the application of potato tops. Furthermore, Tartoura (Citation2010) demonstrated that the utilization of agro-wastes boost the soil water holding capability and increase its field capacity. As a result, this can lead to improved water uptake by plants under conditions of water scarcity, subsequently resulting in enhanced yield characteristics during water stress scenarios. Furthermore, many previous studies have reported the acquired enhancement in seed yield brought on by Bradyrhizobium inoculation in various legume species (Argaw, Citation2017; de Almeida Leite et al., Citation2022; Mittra et al., Citation2005). This increase in yield attributes may result from the decrease in bulk density and the provision of sufficient nutrients by the inoculating bacteria (Mittra et al., Citation2005).
Soil amendments like compost, rhizobacteria and organic agro-wastes are common examples of innovative approaches that have been investigated to boost agricultural yield and soil fertility. Numerous earlier studies have demonstrated that introducing organic amendments to soil can enhance its texture, boost its fertility, maintain the health of the soil across time, enhance microbial activity and, most importantly, promote crop yields (Khatoon et al., Citation2020; Liang et al., Citation2021).
According to the investigation of Singh et al. (Citation2019), bacterial strains employed as biofertilizers have the potential to boost the crop species’ nutritional levels, nitrogen fixation, antioxidant potential, phenolic contents, and photoassimilation rates (Singh et al., Citation2019). Bradyrhizobium inoculation is a typical approach for mitigating water stress and boosting yield components of legume species. The increased crop yield of Bradyrhizobium-inoculated and water-stressed plants could be due to the improved nitrogen assimilation, and acquisition of different nutrients from the soil (Htwe et al., Citation2019). As a result, the capacity of microorganisms and agro-wastes to release nutrients and provide phytohormones beneficial for boosting cowpea yield. Subsequently, supplementing the soil with agro-wastes (like potato tops) or inoculating it with beneficial microorganisms (like Bradyrhizobium) could be viewed as an efficient eco-friendly remedy to the issue of water stress in nutrient-poor sandy soils.
Osmomodulators level
provides an overview of the concentrations of the osmoregulatory molecules (soluble sugars, total proteins, and proline) in the shoot system of cowpea plants grown in the studied sandy soil and exposed to different irrigation levels, different fertilizer formulations, and their combined interactions. The concentration of osmomodulatory compounds was profoundly affected when cowpea was exposed to varying levels of water deficit. The amount of water supplied had a reverse association with the concentration of the investigated osmolytes. As opposed to the well-watered control, the lowest watering level (70%) led to increases in the amounts of soluble sugars, total protein, and proline of 46.5, 48.6, and 71.8%, respectively. Likewise, soluble sugars showed a declining avenue based on the type of the used fertilizer at the full watering situation, with the greatest reduction (28.6%) caused by the application of chopped potato tops, compared to the unfertilized control. Compared to the fertilizer-free treatment under well-watered conditions, total protein concentrations were higher with chemical and organic fertilizers, while they were lower with the biofertilizer. Complete potato tops fertilizer was the leading fertilizer in enhancing total protein content in cowpea. In terms of proline concentration, chemical fertilizer increased it while bio and organic fertilizers decreased it, with chopped potato tops producing the most pronounced decrease (30.5%) at 70% irrigation treatment.
Figure 1. Osmomodulators level of cowpea as affected by various irrigation levels, fertilizer types, and their interactions. Different letters donate statistically significant differences following Tukey test (p < 5%).
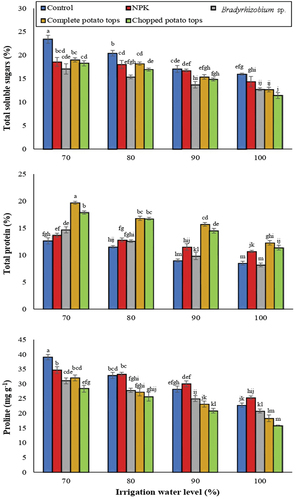
Osmomodulators levels in cowpea were impacted by the integrative interactions of various fertilizer formulations at varying watering levels. In comparison to fertilizer-free treatments at the same watering levels, total soluble sugars and total proline concentrations in cowpea showed a prominent decline with the applied fertilizer formulations at varying watering levels. The biofertilizer (Bradyrhizobium) caused the greatest reduction in soluble sugars and chopped potato tops caused the greatest reduction in proline content, as compared to the other fertilizer formulations. However, the use of different fertilizer formulations has led to an increase in total protein, with complete potato tops often producing the foremost rise in comparison to other fertilizer forms.
Some earlier studies, in accordance with us, reported that adding agro-wastes and root nodulating bacteria to soil boosted plant growth and water stress tolerance (Chiappero et al., Citation2019; Razavipour et al., Citation2018). Accordingly, the use of fertilizers exhibited beneficial effects across all irrigation levels. This suggests that the impact of fertilizers was not specifically related to enhancing drought resistance, but rather to promoting basal growth and increasing yields, irrespective of the soil water conditions. This improvement may be triggered by various mechanisms, including the improvement in nutritional, hormonal, molecular, and biochemical status brought on by enhanced mineral and water uptake. Plant growth-promoting bacteria have been reported to control water stress by synthesizing exopolysaccharides, volatile compounds, phytohormone, and exoenzymes, which has been found to increase tolerance to water stress (Vurukonda et al., Citation2016). Additionally, they enhanced osmolytes and antioxidant enzymes, controlling stress-responsive genes and causing alterations in the root system (Khan et al., Citation2019; Vurukonda et al., Citation2016). As a result, plants don’t require an enormous osmolyte accumulation to offset the osmotic stress produced by a water shortage.
Agro-wastes also has been reported to promote organic compounds biosynthesis, enhancing nutrient use efficiency, and consequently improving plant growth and physiological performance. As a result, plants grown in soils amended with agro-wastes may be less susceptible to water stress (Armada et al., Citation2014). Subsequently, due to the organic and biological amendments, compatible osmolytes may be redirected into alternative metabolic pathways that produce more macromolecules to promote plant growth or they may be easily degraded when the normal water status returns (Singh et al., Citation2015). Overall, Bradyrhizobim and potato tops treatments of the cowpea plant resulted in decreased soluble sugars and proline contents, as well as an increase in total protein, which contributed to alleviating water stress. Hence, the plant did not demonstrate the implications of water stress as a result of the adjustments offered.
Nutritional value
The experimental treatments, including watering rates, fertilizer formulas, and their integrated combinations, had a substantial impact on the nutritional value of cowpea as measured by the ash and crude fibers percentages (). While the maximum ash content (4.20%) was obtained at the fully irrigated control treatment, the lowest ash content (3.55%) was attained at the two lowest irrigation levels (70 and 80%). However, the ash content of cowpea was not significantly affected by the various fertilizer formulations, with the least amount of ash (3.86%) being achieved when chemical and complete potato tops were employed as fertilizers. Concerning the integrative interactions between water levels and fertilizer formulations, the ash content demonstrated a reasonable decline by the interaction of the two factors, with the exception of the combination of complete potato tops as fertilizer with the various watering levels, as it increased ash content compared to the fertilizer-free treatments.
Figure 2. Nutritional value of cowpea as affected by various irrigation levels, fertilizer types, and their interactions. Different letters donate statistically significant differences following Tukey test (p < 5%).
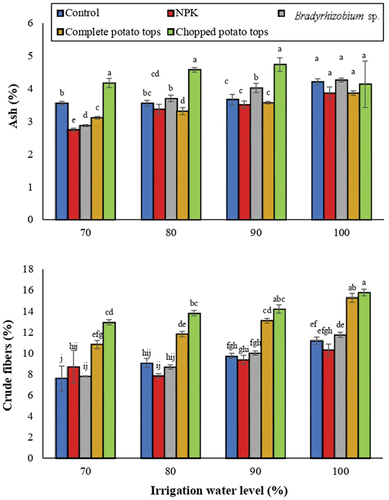
Correspondingly, as the level of water stress increased, crude fibers content gradually decreased in cowpea, with 70% yielding the least amount (7.75%). With the exception of chemical fertilizer, all fertilizer formulations improved the crude fibers content, with the chopped potato tops providing the highest fiber content (15.77%) at the fully irrigated condition. Regarding the interactions of watering levels and fertilizer formulations, the application of chemical fertilizer (NPK) led to a reduction in crude fibers level, whereas the use of bio and organic fertilizers typically resulted in an increase in crude fibers level, in most cases, when compared to fertilizer-free and water-stressed treatments. The highest level of crude fibers was found when the chopped potato tops were employed at various watering dosages.
The integration of the absorbed nutrients into the structure of various macromolecules as well as their involvement in a variety of metabolic activities can be identified as the explanation for the decreased ash content after cowpea fertigation with chemical, bio, and complete potato tops. However, the increased soil fertility and improved soil properties, and the hydraulic status of the rhizosphere as a result of the small size and large surface area of the chopped potato tops could be used to explain the increased ash content attained by the application of chopped potato tops. The improved mineral content, redox homeostasis, photosynthetic performance, and nitrogen assimilation, which led to more macromolecules being synthesized to increase plant vigor, could be the explanation for the increased crude fiber content in water-stressed cowpea plants following the application of various fertilizer forms in this study.
Vegetative attributes
The data presented in (and Table S3) depicts how different irrigation levels, fertilizer types, and their interactions affect the vegetative attributes of cowpea plants in the studied sandy soil. The results demonstrated that the decrease in water level had a comparatively adverse effect on plant height and dry biomass. Accordingly, at the lowest irrigation level (70%) the plant height and the dry biomass were decreased by 8.4, and 15.0%, respectively, as compared to the fully watered treatment. Regarding fertilizer types, plant height was increased with all fertilizer types that were administered compared to the control treatment. However, when compared to the other fertilizer formulations, chopped potato tops stood out as having the greatest impact on boosting vegetative characteristics of cowpea plants at the full irrigation (100%) level. Cowpea biomass also significantly responded to the types of fertilizers applied, with the maximum dry biomass (2888.6 kg ha−1) being attained when the chopped potato tops were used as an organic fertilizer under fully watered conditions. The integrative combinations of fertilizers and water treatments have improved the vegetative attributes of cowpea, with chopped potato tops outperforming other fertilizers at all irrigation levels. Nonetheless, the yield of cowpea dry biomass was higher with the complete potato tops and the biofertilizer (Bradyrhizobium sp.) than with the chemical fertilizer.
Table 6. Vegetative attributes of cowpea as affected by various irrigation levels, fertilizer types and their interactions.
The tolerance of cowpea to water stress was increased by varying degrees by all the applied fertilizer formulations, with the superiority of the organic one (potato tops). Bradyrhizobium inoculation has been reported to increase the nutrient supply of drought-stressed plants, favoring their osmotic homeostasis and promoting cell turgor (Kaushal & Wani, Citation2016), in addition to triggering many metabolic pathways to maintain plant performance to withstand drought conditions (Barbosa et al., Citation2018).
The increased biomass production as a result of the incorporation of agro-wastes into the soil appears to be correlated with the improved plant ability to absorb water, maintain nutritional homeostasis, and activate the photosynthetic machinery, particularly under water stress conditions. Tartoura (Citation2010) demonstrated that the beneficial effects of agro-wastes on soil could mainly be related to the enhancement of water holding capacity and boosting the field capacity of the soil, delivering more water to the stressed plants. The biological amendments (bacteria and fungi) may enhance the plant’s capacity to cope with the stress brought on by water scarcity. These modifications assist stressed plants by starting hormonal signaling, as abscisic acid (ABA) and jasmonate, controlling transpiration rate, and preserving hydraulic balance (Chaumont & Tyerman, Citation2014). As a result, microbial and agro-wastes amendments may be used as eco-friendly substitutes for chemical fertilizers to varying degrees under diverse agricultural circumstances. This could minimize the environmental impact of the chemical fertilizers used and increase sustainability.
Mineral nutrients content
According to the data reported in , the fertilizer formulation used, the amount of irrigation water applied, and their combined interactions had a substantial impact on the mineral content of cowpea in the studied sandy soil. The degree of water stress has a considerable negative impact on the levels of the three nutrients that were being studied: nitrogen (N), phosphorus (P), and potassium (K). In comparison to the fully watered treatment, the N, P, and K concentrations decreased by 14.0, 46.3, and 32.6%, respectively, at the lowest watering level (70%). Meanwhile, the employed fertilizer formulations significantly raised the concentrations of N, P, and K at the fully irrigated conditions in comparison to the non-fertigated control, with chopped potato tops yielding the greatest level of the three investigated elements. Owing to the interactive combination of watering levels and fertilizer treatments, the mineral content of cowpea showed a pronounced increment with the highest content achieved by the chopped potato tops, except for the N content of the irrigation level of 80%, when compared to the other fertilizer formulations under all the studied irrigation levels. In comparison to the bio and chemical fertilizer formulation, potato tops offered a substantial content of the major nutrients required for plant metabolic activities. So, the organic fertilizer represented by chopped potato tops may offer a feasible treatment for sandy soils under water stress that are minerally deficient.
Figure 3. Mineral nutrient content of cowpea as affected by various irrigation levels, fertilizer types, and their interactions. Different letters donate statistically significant differences following Tukey test (p < 5%).
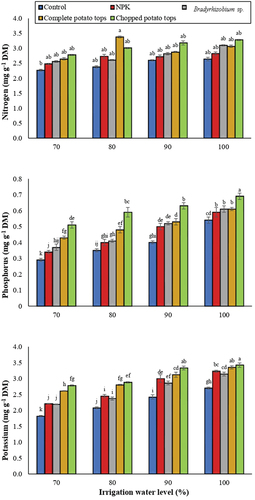
Since agro-wastes are the main source of organic matter in the soil, they are also the most affordable source that farmers may employ to increase the fertility of their soil. Additionally, bio-fertilizers have been cited as crucial elements of integrated nutrition management (Asadu et al., Citation2018; Lesueur et al., Citation2016). The presence of organic matter in the agro-wastes enhances the physical properties of the soil and provides the required energy to the soil decomposers. Therefore, the enhanced nutritional content of cowpea, particularly N and K, by the applied potato tops as an organic fertilizer may be attributable to the increased nutritional potential of the soil as a result of improved microbial degradation of the agro-wastes. Azimi et al. (Citation2018) suggest that the enhanced activity of potassium-solubilizing and nitrogen-fixing bacteria may be responsible for the increase in N and K contents caused by the treatment with agro-wastes. The increased activity of phosphate-solubilizing bacteria can be attributed to the increase in phosphorus concentration (Ting et al., Citation2014). Our findings describing the advantageous contribution of Bradyrhizobium on cowpea elemental composition are consistent with those of El-Sawah et al. (Citation2021), who demonstrated an enhancement in soil quality, soil enzymatic activity, and increased available nutrients (N, P, and K) by employing Bradyrhizobium as biofertilizer. Thus, the increased N content could be a direct result of the enhanced nitrogen-fixation efficiency of the plant caused by Bradyrhizobium (Barea et al., Citation2005). Furthermore, the high concentrations of P and K in the plant tissues may be due to Bradyrhizobium‘s ability to solubilize P (El-Sawah et al., Citation2021). By solubilizing and mineralizing K and P, releasing compounds that regulate plant growth, and decomposing organic matter in the soil, biofertilizers sustain a rich soil environment under drought situations (Sinha et al., Citation2010).
Conclusion
Application of potato agro-wastes as a soil amendment or Bradyrhizobium inoculation as a biofertilizer reduced the detrimental effects of water stress on cowpea, contributing to a substantially higher rate of plant growth, yield, metabolic performance, and nutrient uptake. By enhancing the physical characteristics of the soil, boosting nutrient uptake, and modulating the metabolic processes of the plant under well-watered conditions, the application of organic and biofertilizers increased biomass and seed yield. In comparison to chemical fertilizer, both fertilizer formulations increased plant yield and growth under water stress conditions. Furthermore, the performance of cowpea growth and yield was boosted by the combination of various fertilizer formulations and watering rates, with chopped potato tops outperforming all the formulations investigated. The overall findings demonstrated the agro-wastes’ positive impact on cowpea plants’ ability to withstand water stress. Our findings open new avenues for utilizing organic and biofertilizers to mitigate water stress in forage and crop plants growing in sandy soils deficient in nutrients. To fully understand how the investigated fertilizer formulations have improved plant tolerance to drought, more investigation is still required in the future. Furthermore, more studies are required to validate the potential for achieving more water savings (<70%) through the application of a mixture of chemical, organic, and biofertilizers, while simultaneously ensuring that cowpea yield remains unaffected.
Supplemental Material
Download MS Word (18.5 KB)Disclosure statement
No potential conflict of interest was reported by the author(s).
Supplemental data
Supplemental data for this article can be accessed online at https://doi.org/10.1080/1343943X.2024.2340833
Additional information
Funding
References
- Aboamera, M. A. (2010). Response of cowpea to water deficit under semi-portable sprinkler irrigation system. Misr Journal of Agricultural Engineering, 27(1), 170–190. https://doi.org/10.21608/mjae.2010.107154
- Alemu, M., Asfaw, Z., Woldu, Z., & Fenta, B. A. (2016). Cowpea (Vigna unguiculata (L.) Walp.) (Fabaceae) landrace diversity in Northern Ethiopia. International Journal of Biodiversity and Conservation, 8(11), 297–309. https://doi.org/10.5897/IJBC2016.0946
- Alvarenga, P., Gonçalves, A. P., Fernandes, R. M., de Varennes, A., Vallini, G., Duarte, E., & Cunha-Queda, A. C. (2008). Evaluation of composts and liming materials in the phytostabilization of a mine soil using perennial ryegrass. Science of the Total Environment, 406(1), 43–56. https://doi.org/10.1016/j.scitotenv.2008.07.061
- AOAC. (2010). Official methods of analysis of association of official analytical chemists (18th ed.). Washington, DC.
- APHA. (2001). Standard methods for the examination of water and wastewater. Apha.
- Argaw, A. (2017). Organic and inorganic fertilizer application enhances the effect of bradyrhizobium on nodulation and yield of peanut (arachis hypogea L.) in nutrient depleted and sandy soils of Ethiopia. International Journal of Recycling of Organic Waste in Agriculture, 6(3), 219–231. https://doi.org/10.1007/s40093-017-0169-3
- Armada, E., Portela, G., Roldán, A., & Azcón, R. (2014). Combined use of beneficial soil microorganism and agrowaste residue to cope with plant water limitation under semiarid conditions. Geoderma, 232-234, 640–648. https://doi.org/10.1016/j.geoderma.2014.06.025
- Asadu, C. O., Aneke, N. G., Egbuna, S. O., & Agulanna, A. C. (2018). Comparative studies on the impact of bio-fertilizer produced from agro-wastes using thermo-tolerant actinomycetes on the growth performance of maize (Zea mays) and okro (Abelmoschus esculentus). Environmental Technology and Innovation, 12, 55–71. https://doi.org/10.1016/j.eti.2018.07.005
- Azimi, S., Salehi, M. B., & Bahador, N. (2018). Isolation and identification of Streptomyces ramulosus from soil and determination of antimicrobial property of its pigment. Modern Medical Laboratory Journal, 1(1), 36–41. https://doi.org/10.30699/mmlj17-01-07
- Barbosa, D. D., Brito, S. L., Fernandes, P. D., Fernandes-Júnior, P. I., & Lima, L. M. (2018). Can bradyrhizobium strains inoculation reduce water deficit effects on peanuts? World Journal of Microbiology and Biotechnology, 34(7), 87. https://doi.org/10.1007/s11274-018-2474-z
- Barea, J.-M., Pozo, M. J., Azcón, R., & Azcón-Aguilar, C. (2005). Microbial co-operation in the rhizosphere. Journal of Experimental Botany, 56(417), 1761–1778. https://doi.org/10.1093/jxb/eri197
- Bates, L. S., Waldren, R. P., & Teare, I. D. (1973). Rapid determination of free proline for water-stress studies. Plant and Soil, 39(1), 205–207. https://doi.org/10.1007/BF00018060
- Bhattacharyya, R., Chandra, S., Singh, R. D., Kundu, S., Srivastva, A. K., & Gupta, H. S. (2007). Long-term farmyard manure application effects on properties of a silty clay loam soil under irrigated wheat–soybean rotation. Soil and Tillage Research, 94(2), 386–396. https://doi.org/10.1016/j.still.2006.08.014
- Bradford, M. M. (1976). A rapid and sensitive method for the quantitation of microgram quantities of protein utilizing the principle of protein-dye binding. Analytical Biochemistry, 72(1–2), 248–254. https://doi.org/10.1016/0003-2697(76)90527-3
- Chaumont, F., & Tyerman, S. D. (2014). Aquaporins: Highly regulated channels controlling plant water relations. Plant Physiology, 164(4), 1600–1618. https://doi.org/10.1104/pp.113.233791
- Chiappero, J., Cappellari, L. D. R., Sosa Alderete, L. G., Palermo, T. B., & Banchio, E. (2019). Plant growth promoting rhizobacteria improve the antioxidant status in mentha piperita grown under drought stress leading to an enhancement of plant growth and total phenolic content. Industrial Crops and Products, 139, 111553. https://doi.org/10.1016/j.indcrop.2019.111553
- de Almeida Leite, R., Martins, L. C., dos Santos França Ferreira, L. V., Barbosa, E. S., Alves, B. J. R., Zilli, J. E., Paulo, A. A., & da Conceição Jesus, E. (2022). Co-inoculation of rhizobium and bradyrhizobium promotes growth and yield of common beans. Applied Soil Ecology, 172, e104356. https://doi.org/10.1016/j.apsoil.2021.104356
- Dubois, M., Gilles, K., Hamilton, J., Rebers, P., & Smith, F. (1965). Colorimetric method for determination of sugars and related substances. Analytical Chemistry, 28(3), 350–356. https://doi.org/10.1021/ac60111a017
- Dukes, M. D., Zotarelli, L., Liu, G. D., & Simonne, E. H. (2012). Principles and practices of irrigation management for vegetables. University of Florida IFAS Extension, 1–14. https://edis.ifas.ufl.edu/publication/CV107
- El-Sawah, A. M., El-Keblawy, A., Ali, D. F. I., Ibrahim, H. M., El-Sheikh, M. A., Sharma, A., Alhaj Hamoud, Y., Shaghaleh, H., Brestic, M., Skalicky, M., Xiong, Y., & Sheteiwy, M. S. (2021). Arbuscular mycorrhizal fungi and plant growth-promoting rhizobacteria enhance soil key enzymes, plant growth, seed yield, and qualitative attributes of guar. Agriculture, 11(3), e194. https://doi.org/10.3390/agriculture11030194
- El-Serafy, Z. M., & El-Ghamry, A. M. (2006). Methods of soil and water analysis (practices). In Z. M. El-Serafy & A. M. El-Ghamry (Eds.), Soils Department, Faculty of Agriculture (p. 253). Mansoura University.
- Fageria, N. K. (2012). Role of soil organic matter in maintaining sustainability of cropping systems. Communications in Soil Science and Plant Analysis, 43(16), 2063–2113. https://doi.org/10.1080/00103624.2012.697234
- Falagán, N., Artés, F., Artés-Hernández, F., Gómez, P. A., Pérez-Pastor, A., & Aguayo, E. (2015). Comparative study on postharvest performance of nectarines grown under regulated deficit irrigation. Postharvest Biology and Technology, 110, 24–32. https://doi.org/10.1016/j.postharvbio.2015.07.011
- Faloye, O. T., & Alatise, M. O. (2015). Effect of varying water applications on evapotranspiration and yield of cowpea under sprinkler irrigation system. International Journal of Agriculture and Crop Sciences, 8(3), 307–319. https://org/IJACS/2015/8-3/307-319
- Gerrano, A. S., Rensburg Van, W. S. J., Venter, S. L., Shargie, N. G., Amelework, B. A., Shimelis, H. A., & Labuschagne, M. T. (2019). Selection of cowpea genotypes based on grain mineral and total protein content. Acta Agriculturae Scandinavica, Section B Soil & Plant Science, 69(2), 155–166. https://doi.org/10.1080/09064710.2018.1520290
- Hoenig, M., Baeten, H., Vanhentenrijk, S., Vassileva, E., & Quevauviller, P. (1998). Critical discussion on the need for an efficient mineralization procedure for the analysis of plant material by atomic spectrometric methods. Analytica Chimica Acta, 358(1), 85–94. https://doi.org/10.1016/S0003-2670(97)00594-1
- Htwe, A. Z., Moh, S. M., Moe, K., & Yamakawa, T. (2019). Biofertilizer production for agronomic application and evaluation of its symbiotic effectiveness in soybeans. Agronomy, 9(4), 1–16. https://doi.org/10.3390/agronomy9040162
- Kaushal, M., & Wani, S. P. (2016). Plant-growth-promoting rhizobacteria: Drought stress alleviators to ameliorate crop production in drylands. Annals of Microbiology, 66(1), 35–42. https://doi.org/10.1007/s13213-015-1112-3
- Khan, N., Bano, A., Rahman, M. A., Guo, J., Kang, Z., & Babar, M. A. (2019). Comparative physiological and metabolic analysis reveals a complex mechanism involved in drought tolerance in chickpea (Cicer arietinum L.) induced by PGPR and PGRs. Scientific Reports, 9(1), e2097. https://doi.org/10.1038/s41598-019-38702-8
- Khatoon, Z., Huang, S., Rafique, M., Fakhar, A., Kamran, M. A., & Santoyo, G. (2020). Unlocking the potential of plant growth-promoting rhizobacteria on soil health and the sustainability of agricultural systems. Journal of Environmental Management, 273, e111118. https://doi.org/10.1016/j.jenvman.2020.111118
- Lesueur, D., Deaker, R., Herrmann, L., Bräu, L., & Jansa, J. (2016). The production and potential of biofertilizers to improve crop yields. In N. K. Arora, S. Mehnaz, & R. Balestrini (Eds.), Bioformulations: for sustainable agriculture (pp. 71–92). Springer India. https://doi.org/10.1007/978-81-322-2779-3_4.
- Liang, X., Chen, Q., Rana, M. S., Dong, Z., Liu, X., Hu, C., Tan, Q., Zhao, H., Sun, X., & Wu, S. (2021). Effects of soil amendments on soil fertility and fruit yield through alterations in soil carbon fractions. Journal of Soils and Sediments, 21(7), 2628–2638. https://doi.org/10.1007/s11368-021-02932-z
- Malr, E. (2018). The ministry of agriculture and land reclamation. Annual Bulletin of Indicators of Agricultural Statistics.
- Mittra, B. N., Karmakar, S., Swain, D. K., & Ghosh, B. C. (2005). Fly ash—a potential source of soil amendment and a component of integrated plant nutrient supply system. Fuel, 84(11), 1447–1451. https://doi.org/10.1016/j.fuel.2004.10.019
- Mndzebele, B., Ncube, B., Nyathi, M., Kanu, S. A., Fessehazion, M., Mabhaudhi, T., Amoo, S., & Modi, A. T. (2020). Nitrogen fixation and nutritional yield of cowpea-amaranth intercrop. Agronomy, 10(4), e565. https://doi.org/10.3390/agronomy10040565
- Nunes, H. G. G. C., de Pinho Sousa, D., Moura, V. B., Ferreira, D. P., de Nóvoa Pinto, J. V., de Oliveira Vieira, I. C., da Silva Farias, V. D., de Oliveira, E. C., & de Souza, P. J. (2019). Performance of the aquacrop model in the climate risk analysis and yield prediction of cowpea (Vigna unguiculatta L. Walp). Australian Journal of Crop Science, 13(7), 1105–1112. https://doi.org/10.21475/ajcs.19.13.07.p1590
- Nunes, C., Moreira, R., Pais, I., Semedo, J., Simões, F., Veloso, M. M., & Scotti-Campos, P. (2022). Cowpea physiological responses to terminal drought-Comparison between four landraces and a commercial variety. Plants, 11(5), e593. https://doi.org/10.3390/plants11050593
- Ortuani, B., Facchi, A., Mayer, A., Bianchi, D., Bianchi, A., & Brancadoro, L. (2019). Assessing the effectiveness of variable-rate drip irrigation on water use efficiency in a vineyard in northern Italy. Water, 11(10), e–1964. https://doi.org/10.3390/w11101964
- Osipitan, O. A., Fields, J. S., Lo, S., & Cuvaca, I. (2021). Production systems and prospects of cowpea (Vigna unguiculata (L.) Walp.) in the United States. Agronomy, 11(11), e2312. https://doi.org/10.3390/agronomy11112312
- Pahalvi, H. N., Rafiya, L., Rashid, S., Nisar, B., & Kamili, A. N. (2021). Chemical fertilizers and their impact on soil health. In G. H. Dar, R. A. Bhat, M. A. Mehmood, & K. R. Hakeem (Eds.), Microbiota and biofertilizers, ecofriendly tools for reclamation of degraded soil environs (pp. 1–20). Springer International Publishing. https://doi.org/10.1007/978-3-030-61010-4_1.
- Razavipour, T., Moghaddam, S. S., Doaei, S., Noorhosseini, S. A., & Damalas, C. A. (2018). Azolla (Azolla filiculoides) compost improves grain yield of rice (Oryza sativa L.) under different irrigation regimes. Agricultural Water Management, 209, 1–10. https://doi.org/10.1016/j.agwat.2018.05.020
- Sarkar, S., Skalicky, M., Hossain, A., Brestic, M., Saha, S., Garai, S., Ray, K., & Brahmachari, K. (2020). Management of crop residues for improving input use efficiency and agricultural sustainability. Sustainability, 12(23), e9808. https://doi.org/10.3390/su12239808
- Shahrokhnia, M. H., & Sepaskhah, A. R. (2016). Effects of irrigation strategies, planting methods and nitrogen fertilization on yield, water and nitrogen efficiencies of safflower. Agricultural Water Management, 172, 18–30. https://doi.org/10.1016/j.agwat.2016.04.010
- Singh, M., Kumar, J., Singh, S., Singh, V. P., & Prasad, S. M. (2015). Roles of osmoprotectants in improving salinity and drought tolerance in plants: A review. Reviews in Environmental Science and Bio/technology, 14(3), 407–426. https://doi.org/10.1007/s11157-015-9372-8
- Singh, M., Singh, D., Gupta, A., Pandey, K. D., Singh, P. K., & Kumar, A. (2019). Plant growth promoting rhizobacteria: Application in biofertilizers and biocontrol of phytopathogens. In A. K. Singh, A. Kumar, & P. K. Singh (Eds.), PGPR Amelioration in Sustainable Agriculture (pp. 41–66). Woodhead Publishing. https://doi.org/10.1016/B978-0-12-815879-1.00003-3.
- Sinha, R. K., Valani, D., Chauhan, K., & Agarwal, S. (2010). Embarking on a second green revolution for sustainable agriculture by vermiculture biotechnology using earthworms: Reviving the dreams of Sir Charles Darwin. Journal of Agricultural Biotechnology and Sustainable Development, 2(7), 113–128.
- Sofi, P. A., Baba, Z. A., Hamid, B., & Meena, R. S. (2018). Harnessing soil rhizobacteria for improving drought resilience in legumes. In R. S. Meena, A. Das, G. S. Yadav, & R. Lal (Eds.), Legumes for soil health and sustainable management (pp. 235–275). Springer Singapore. https://doi.org/10.1007/978-981-13-0253-4_8.
- Sun, R., Guo, X., Wang, D., & Chu, H. (2015). Effects of long-term application of chemical and organic fertilizers on the abundance of microbial communities involved in the nitrogen cycle. Applied Soil Ecology, 95, 171–178. https://doi.org/10.1016/j.apsoil.2015.06.010
- Tartoura, K. A. H. (2010). Alleviation of oxidative-stress induced by drought through application of compost in wheat (Triticum aestivum L.) plants. American-Eurasian Journal of Agricultural & Environmental Sciences, 9(2), 208–216.
- Ting, A. S. Y., Hermanto, A., & Peh, K. L. (2014). Indigenous actinomycetes from empty fruit bunch compost of oil palm: Evaluation on enzymatic and antagonistic properties. Biocatalysis and Agricultural Biotechnology, 3(4), 310–315. https://doi.org/10.1016/j.bcab.2014.03.004
- Topak, R., Acar, B., Uyanöz, R., & Ceyhan, E. (2016). Performance of partial root-zone drip irrigation for sugar beet production in a semi-arid area. Agricultural Water Management, 176, 180–190. https://doi.org/10.1016/j.agwat.2016.06.004
- Varughese, A., Balan, M. T., Sajna, A., & Swetha, K. P. (2014). Cowpea inside polyhouse with varying irrigation and fertigation levels. International Journal of Engineering Research and Development, 10(8), 18–21. https://org/a5679b2d7f0fd989fbbcfdbf563e01353038b95
- Verma, B. C., Pramanik, P., & Bhaduri, D. (2020). Organic fertilizers for sustainable soil and environmental management. In R. S. Meena (Ed.), Nutrient dynamics for sustainable crop production (pp. 289–313). Springer Singapore. https://doi.org/10.1007/978-981-13-8660-2_10.
- Vurukonda, S. S. K. P., Vardharajula, S., Shrivastava, M., & SkZ, A. (2016). Enhancement of drought stress tolerance in crops by plant growth promoting rhizobacteria. Microbiological Research, 184, 13–24. https://doi.org/10.1016/j.micres.2015.12.003