Abstract
Context
Metformin (Met) has a protective effect against cardiac ischemia and reperfusion (I/R) injury.
Objective
This study uncovered the Met effect on ferroptosis in cardiac I/R.
Materials and methods
Sprague-Dawley rats underwent cardiac I/R treatment (ischaemia 30 min; reperfusion 24 h) (I/R group), and administered intravenously with Met (200 mg/kg) (I/R + Met group). Haematoxylin–eosin staining, Prussian blue staining, immunohistochemistry and transmission electron microscope were conducted on cardiac tissues. H9c2 cells underwent oxygen-glucose deprivation/reoxygenation (OGD/R group) and treated by Met (0.1 mM) (OGD/R + Met group). Adenosine monophosphate-activated protein kinase α (AMPKα) siRNA was transfected into OGD/R-induced H9c2 cells. Cell counting kit-8 (CCK-8) assay, dichloro-dihydro-fluorescein diacetate (DCFH-DA) and JC-1 staining were conducted on H9c2 cells. Ferroptosis-related indicators and gene expression were detected by enzyme-linked immunosorbent assay (ELISA), quantitative reverse transcription-polymerase chain reaction (qRT-PCR) and Western blot.
Results
In cardiac I/R rat, Met decreased heart and serum MDA, cardiac and serum non-heme iron, and serum CK-MB and LDH (inhibition rate: 50.0%, 48.8%, 47.6%, 29.5%, 30.6% and 34.7%, respectively), relieved cardiac tissue ferroptosis and mitochondria damage, increased fraction shortening and ejection fraction (157.5% and 146.2% on day 28, respectively), up-regulated AMPKα and down-regulated NOX4 in cardiac tissues. In OGD/R-induced H9c2 cells, Met (0.1 mM) increased cell viability (promotion rate: 170.0%), decreased non-heme iron and MDA (inhibition rate: 30.1% and 47.9%, respectively), relieved ferroptosis, up-regulated AMPKα and down-regulated NOX4. AMPKα silencing abrogated these effects of Met on the OGD/R-induced H9c2 cells.
Discussion and conclusions
Met shows effectiveness in relieving ferroptosis in cardiac I/R. In the future, Met may be an effective drug for relieving ferroptosis in cardiac I/R patients clinically.
Introduction
Cardiac ischemia and reperfusion (I/R) is a leading cause of mortality worldwide; over 15 million people die of cardiac I/R annually (Lahnwong et al. Citation2020). In clinical practice, the rapid restoration of blood flow to the heart after myocardial infarction (known as reperfusion process) is the most effective treatment method for acute myocardial infarction (Ge et al. Citation2021). However, the reperfusion process can trigger I/R injury such as induction of myocardial cell death and expansion of myocardial infarction size, which places patients at greater risk of adverse prognosis and even death (Lahnwong et al. Citation2020; Ge et al. Citation2021). Thus, mitigating the cardiac I/R injury is an important direction for the clinical treatment of acute myocardial infarction.
Ferroptosis is an iron- and reactive oxygen species (ROS)-dependent programmed cell death, which is distinct from other forms of cell death. Ferroptosis is a form of cell death primarily caused by the accumulation of ROS driven by lipid peroxidation (Shan et al. Citation2021; Stamenkovic et al. Citation2021). Ferroptosis has been revealed to exert an important role in multiple heart diseases, such as cardiac I/R, heart failure and cardiomyopathy (Wu et al. Citation2021). Iron deposition and ROS over-production are the main characteristics of cardiac I/R, which can trigger and exacerbate ferroptosis in cardiomyocytes and cardiac tissues. However, ferroptosis in turn promotes ROS accumulation to facilitate the death of cardiomyocytes (Ma et al. Citation2020). Thus, the obstruction of ferroptosis can allow the cardiomyocytes to be substantially protected (Zhao et al. Citation2021). At present, several drugs have been found to possess an inhibitory effect on ferroptosis in cardiac I/R. For instance, resveratrol can attenuate the oxidative stress and iron content in both cardiac I/R cell and animal models, which can protect against the cardiac I/R injury via ameliorating ferroptosis (Li T et al. Citation2022). Cyanidin-3-glucoside is widely contained in red or purple fruits and vegetables, possessing both antioxidant function and an anti-inflammatory effect. Cyanidin-3-glucoside is anticipated to be a potential drug for cardiac I/R injury, as it exhibits a mitigating effect on the cardiac I/R injury induced by ferroptosis (Shan et al. Citation2021). Ferulic acid has the antioxidant property, which is a key active ingredient extracted from Angelica sinensis (Oliv.) Diels (Umbelliferae). Ferulic acid can ameliorate cardiac I/R injury by inactivating ferroptosis (Liu et al. Citation2021).
Recently, metformin (Met) has been reported to possess a protective effect against the cardiac I/R injury (Zhang et al. Citation2020; Li W et al. Citation2021). However, the exact function and molecular mechanism of Met in mitigating cardiac I/R injury remain to be elucidated in detail. In our preliminary in vitro study, we established a cardiac I/R cell model by inducing H9c2 cell with oxygen-glucose deprivation/reoxygenation (OGD/R). It was noticed that adenosine monophosphate-activated protein kinase α (AMPKα) was down-regulated and nicotinamide adenine dinucleotide phosphate oxidase 4 (NOX4) was up-regulated in the OGD/R-treated H9c2 cells. Intriguingly, Met treatment of the OGD/R-treated H9c2 cells abrogated this effect. This study determines whether Met acts on cardiac I/R injury through regulating the AMPKα/NOX4 pathway.
Materials and methods
Animal Ethics Committee
Animal study in the present work has been ratified by the Animal Ethics Committee of Tianjin Chest Hospital (TJCH-2022-002), and implemented in line with the Guide for the Care and Use of Laboratory Animals.
Animals and grouping
Adult male Sprague-Dawley rats (n = 104, 220–250 g) were purchased from Vital River Laboratory Animal Technology (Beijing, China). All rats were kept in a 22 ± 2 °C room with free access to water and food. The rats were randomly divided into four groups (n = 26): control, I/R group, I/R + Met and I/R + Fer-1.
Cardiac I/R modelling and Met treatment of rats
A cardiac I/R rat model was constructed according to the following procedure: rats were deeply anesthetized by inhalation of 5% isoflurane and then maintained by inhalation of 2% isoflurane (Denorme et al. Citation2020). After intubation, the rodent respirator (Yuyan Scientific Instrument, Shanghai, China) was utilized for the ventilation of rats. The skin on the chest of rats was exposed and sterilized. The thoracic cavity was opened at the fourth intercostal space. We then applied a 6-0 silk suture to ligate the left anterior descending coronary artery of the rats for 30 min at about 2 mm distal to the original of the left anterior descending coronary artery. After 30 min ischemic treatment, the slipknot was released to perfuse the heart for 24 h (Shi and Hou Citation2021). The elevated ST segment on the electrocardiogram indicated a successful establishment of the cardiac I/R rat model. Rats without the ST segment elevation were excluded from this research.
Rats of the I/R group were only subjected to the cardiac I/R treatment. Rats of the control group only experienced the thoracotomy without the cardiac I/R procedure. For the I/R + Met group, after ischemic treatment, 200 mg/kg Met (dissolved in normal saline solution) was administered intravenously to the rats through the left femoral catheter, and then reperfusion treatment (Benjanuwattra et al. Citation2020). For the I/R + Fer-1 group, rats sequentially experienced the ischemic treatment, ferrostatin-1 (Fer-1, a common ferroptosis inhibitor, 4 mg/kg) treatment (administered intravenously) (Liao et al. Citation2021), and then reperfusion treatment.
After reperfusion, six rats of each group were randomly selected for collection of blood samples. Then, rats were euthanized to collect the whole heart. The hearts were all stored in liquid nitrogen.
The anaesthesia and euthanasia process of rats was implemented as follows: first, rats were deeply anaesthetized by inhalation of 5% isoflurane. When rats had no response to limb and head stimulation, they were considered to be deeply anaesthetized. Thereafter, rats were quickly killed by rapid cervical dislocation. After 10 s of rapid cervical dislocation, rats were judged to be dead if they stopped breathing and had no response to limb and head stimulation.
Cardiac function detection and survival analysis
After reperfusion, the remaining 20 rats of each group were kept for 28 days. At 4 h and on day 14 and day 28 post-reperfusion, rats were anesthetized by inhalation of 2% isoflurane and placed in a supine position on a thermal blanket at 37 °C. The heart rate, fraction shortening (FS%) and ejection fraction (EF%) were monitored by applying a Vevo3100 with an MX250 probe (FUJIFILM VisualSonics, Inc., Toronto, Canada) at an ultrasound frequency of 21 MHz. The 28-day survival of the 20 rats in each group was analysed. During this period, rats had free access to food and water.
Haematoxylin–eosin (HE) staining, Prussian blue staining and immunohistochemistry
The damage of rat cardiac tissues was evaluated by HE staining. The cardiac tissues were fixed for 24 h by immersion into 4% paraformaldehyde. After being embedded into paraffin, the cardiac tissues were cut into sections with a thickness of 4 μm. The dewaxing and rehydration of the cardiac tissue sections were implemented in sequence via treating with xylene and graded alcohol (100%, 95%, 90%, 80% and 70%). For HE staining, haematoxylin staining solution (Beyotime, Shanghai, China) and eosin staining solution (Beyotime, Shanghai, China) were sequentially utilized to stain the cardiac tissue sections in accordance with the directions. In terms of Prussian blue staining, the cardiac tissue sections were stained by Perls staining solution (Yanjin Biotechnology, Shanghai, China) and then eosin staining solution. Regarding immunohistochemistry, the cardiac tissue sections were first probed by rabbit anti-AMPKα (1:100, ab3759, Abcam, Shanghai, China) and rabbit anti-NOX4 (1:100, A-AP53328, AmyJet Scientific, Wuhan, China) overnight at 4 °C, and then reacted by horseradish peroxidase-conjugated goat anti-rabbit secondary antibody (1:200, E030120, Yanhui Biotechnology, Shanghai, China) for 2 h room temperature. The sections were then stained by diaminobenzidine (DAB, Beyotime, Shanghai, China) and counterstained by haematoxylin solution in accordance with the directions. After being dehydrated by graded alcohol (70%, 80%, 90%, 95% and 100%) and xylene, the cardiac tissue sections were sealed in neutral resin and observed under a microscope (Olympus, Tokyo, Japan).
Transmission electron microscope (TEM)
The cardiac tissues of rats were fixed by TEM-specific fixed solution, followed by being dehydrated with gradient acetone (50%, 70%, 80%, 90%, 95% and 100%). After being embedded into epoxy resin, the cardiac tissues were cut into sections with a thickness of 75 nm. The sections were spread onto the copper grid coating with carbon film, stained using uranium acetate for 10 min, and then treated with lead citrate containing sodium hydroxide for 10 min. Finally, the sections were placed under a TEM (Hitachi, Tokyo, Japan) to observe the damage of cardiac mitochondria.
Oxygen-glucose deprivation/reoxygenation treatment of H9c2 cells
H9c2 cell line was purchased from Lmai Bioengineering (Shanghai, China) and cultivated into Dulbecco’s modified Eagle medium (DMEM) containing 10% foetal bovine serum (FBS) at 37 °C, 5% CO2 and 95% air.
The induction of OGD/R was carried out as previously reported (Ma et al. Citation2020; Shan et al. Citation2021; Xu et al. Citation2021). Briefly, H9c2 cells were first subjected to the OGD induction by incubating at 94% N2, 5% CO2 and 1% O2 for 8 h in low-glucose DMEM. Then, H9c2 cells were cultivated at 95% air and 5% CO2 for 24 h in high-glucose DMEM containing 10% FBS for the reoxygenation.
Met and Fer-1 treatment of H9c2 cells
H9c2 cells were divided into four groups: control, OGD/R, OGD/R + Met and OGD/R + Fer-1 groups. For the control group, H9c2 cells were always grown under normal conditions, i.e., in high-glucose DMEM containing 10% FBS at 95% air and 5% CO2. H9c2 cells of the OGD/R group were only subjected to the OGD/R treatment. H9c2 cells of the OGD/R + Met group and the OGD/R + Fer-1 group were first subjected to the induction of OGD, and then cultured in high-glucose DMEM containing 10% FBS and 0.1 mM (Shi and Hou Citation2021) Met or 10 µM (Sun et al. Citation2021) Fer-1 at the start of the reoxygenation.
Additionally, after the OGD treatment, DMEM containing different dose of Met (0.1, 0.2, 0.5 and 1 mM) was utilized to treat H9c2 cells at the start of the reoxygenation, in order to explore the function of Met on the AMPKα/NOX4 pathway in H9c2 cells.
H9c2 cell transfection
siRNA targeting AMPKα and negative control (NC) were purchased from GeneChem (Shanghai, China). H9c2 cells in serum-free DMEM experienced transfection with AMPKα siRNA and NC by using the Lipofectamine 3000 (Zeye Biotechnology, Shanghai, China). Additionally, H9c2 cells were treated by OGD/R and 0.1 mM Met. Then, cells were dispersed into serum-free DMEM to experience transfection of AMPKα siRNA by Lipofectamine 3000. The transfection procedure was executed in line with the directions of the Lipofectamine 3000. These H9c2 cells were set as the OGD/R + Met + AMPKα-siRNA group.
Cell counting kit-8 (CCK-8) assay
H9c2 cells underwent CCK-8 assay to research the cell viability. After the relevant treatment, H9c2 cells of each group were harvested and grown into 96-well plates for 24, 48 and 72 h culture in DMEM containing 10% FBS at 37 °C, 5% CO2 and 95% air. The seeding density of H9c2 cells was 1 × 104 cells/100 μL medium in each well. At specific time point, H9c2 cells were treated by 10 μL CCK-8 solution for 2 h at 37 °C. The optical density (OD) value of each well was detected by utilizing a multiwell microplate reader (Huisong Technology, Shenzhen, China). Five duplicate holes were set in each group.
Dichloro-dihydro-fluorescein diacetate (DCFH-DA) staining
The production of ROS in H9c2 cells was evaluated by the DCFH-DA staining. After the relevant treatment, H9c2 cells of each group were treated for 10 min by DCFH-DA (Biolab Technology, Beijing, China) at 37 °C. Then, phosphate-buffered saline (PBS) was used to wash away the residual DCFH-DA solution. The 4′,6-diamidino-2-phenylindole (DAPI) solution was added to stain the nuclei for 15 s. After removing the residual DAPI solution, H9c2 cells were observed under a fluorescence microscope (Olympus, Tokyo, Japan).
JC-1 staining
JC-1 staining is a widely used method to monitor the mitochondrial membrane potential. JC-1 fluoresces strongly red in the normal mitochondria and green in the damaged mitochondria. After the relevant treatment, H9c2 cells of each group were washed twice by PBS and then incubated by 500 μL JC-1 working solution (Beyotime, Shanghai, China) for 15 min at 37 °C, 5% CO2. The fluorescence staining of H9c2 cells was observed under a fluorescence microscope (Olympus, Tokyo, Japan).
Enzyme-linked immunosorbent assay (ELISA)
The cardiac tissues of rats were homogenized on ice and centrifuged to collect the supernatant. The blood samples of rats were centrifuged to harvest the supernatant. H9c2 cells of each group were harvested and lysed in cell lysate for 30 min on ice to collect the supernatant by centrifugation. The level of malondialdehyde (MDA), non-heme iron, creatine kinase-myocardial band (CK-MB) and lactate dehydrogenase (LDH) in these supernatant samples was monitored by utilizing the corresponding kits, including MDA kit (AmyJet Scientific, Wuhan, China), non-heme iron kit (Haring Biotechnology, Shanghai, China), CK-MB kit (Jianglai Biotechnology, Shanghai, China) and LDH kit (AmyJet Scientific, Wuhan, China). The detection procedure was implemented strictly in accordance with the directions of these kits.
Real-time quantitative reverse transcription-polymerase chain reaction (qRT-PCR)
TRIzol reagent (Yanjin Biotechnology, Shanghai, China) was utilized to extract total RNA in rat cardiac tissues and H9c2 cells. The reverse transcription reaction was implemented by using the Reverse Transcription Kit (Invitrogen, Carlsbad, CA) with the following parameters: 70 °C, 5 min; 42 °C, 60 min; 70 °C, 15 min. Subsequently, PCR was carried out by applying the SYBR Green qPCR Reagent Kit (Biomars, Beijing, China) on the Bio-Rad CFX Connect Real-Time PCR Detection System (Bio-Rad, Hercules, CA). The procedure of PCR was 94 °C, 30 s; 56 °C, 30s; 72 °C, 30s, with 38 cycles. The primers were: prostagl and in-endoperoxide synthase 2 (PTGS2), forward 5′-GAGCACCATTCTCCTTGAAA-3′ and reverse 5′-ATTGAGGCAGTGTTGATGATT-3′. AMPKα, forward 5′-CGGAGCCTTGATGTGGTAGG-3′ and reverse 5′-TTCATCCAGCCTTCCATTCTT-3′. Actin, forward 5′-CATGTACGTTGCTATCCAGGC-3′ and reverse 5′-CTCCTTAATGTCACGCACGAT-3′. The relative expression of PTGS2 mRNA was calculated by the 2−ΔΔCT method and normalized to actin.
Western blot
Total proteins in rat cardiac tissues and H9c2 cells were extracted by RIPA (Beyotime, Shanghai, China). The lysate was centrifuged and the concentration of total proteins in each supernatant sample was determined by BCA kit (Zeye Biotechnology, Shanghai, China) in line with the directions. The total protein samples underwent sodium dodecyl sulphate-polyacrylamide gel electrophoresis for separation. After being transferred onto polyvinylidene fluoride (PVDF) membranes, the proteins were subjected to the blockage by 5% skimmed milk for 2 h at room temperature. After that, primary antibody (1:1000) treatment of the proteins was performed for 12 h at 4 °C, and then secondary antibody (1:2000) treatment for 2 h at room temperature. Antibodies were all purchased from AmyJet Scientific (Wuhan, China) and the information of each antibody was listed below: rabbit anti-PTGS2 primary antibody (PAB9950), rabbit anti-AMPKα primary antibody (3113-100), rabbit anti-NOX4 primary antibody (PAB12095), rabbit anti-actin primary antibody (AS13-2640) and horseradish peroxidase-conjugated goat anti-rabbit secondary antibody (E030120). The protein blots were developed according to the instructions of ECL kit (Absin, Shanghai, China). The quantification of proteins was carried out by detecting the grey value of the blots via utilizing the Image J software (NIH, Bethesda, MD). Actin was the control to determine the relative expression of proteins.
Statistical analysis
All experiments were implemented independently at least three times. Data were processed into the form of means ± standard deviation, and analysed by the GraphPad Prism 6.0 software (La Jolla, CA). Difference analysis between two groups was performed by two-tailed paired Student’s t-test, and that in at least three groups was carried out by one-way analysis of variance (with Tukey’s post hoc test). The threshold for a statistically significant difference was set as p < 0.05.
Results
Met ameliorated ferroptosis induced by cardiac I/R
The level of PTGS2 (a marker of ferroptosis) in the cardiac tissues of rats was detected to evaluate ferroptosis. Much higher PTGS2 mRNA and protein levels were occurred in the cardiac tissues of rats in the I/R group when relative to the control, the I/R + Met and the I/R + Fer-1 groups (p < 0.001). When matched to the I/R group, Met treatment (the I/R + Met group) inhibited PTGS2 mRNA expression by about 51.7% and PTGS2 protein expression by about 52.8% (). MDA level in the cardiac tissues and serum of rats was further monitored. As a result, a significantly higher MDA level was observed in both cardiac tissues and serum of rats in the I/R group, when compared to the control, the I/R + Met and the I/R + Fer-1 groups (p < 0.001). In contrast to the I/R group, Met (the I/R + Met group) reduced cardiac MDA level and serum MDA level at about 50.0% and 48.8%, respectively (). Similarly, a much higher non-heme iron level was shown in rat cardiac tissues and serum of the I/R group in comparison to the control, the I/R + Met and the I/R + Fer-1 groups (p < 0.05, p < 0.01 and p < 0.001). Met (the I/R + Met group) suppressed cardiac non-heme iron level and serum non-heme iron level at about 47.6% and 29.5%, respectively, in comparison to the I/R group ().
Figure 1. Met ameliorated ferroptosis induced by cardiac I/R. (A, B) qRT-PCR and Western blot were implemented respectively to evaluate the expression of PTGS2 mRNA and protein in the cardiac tissues of rats. (C, D) MDA level in the cardiac tissues and serum of rats was monitored via ELISA. (E, F) Non-heme iron level in the cardiac tissues and serum of rats was monitored by ELISA. (G) Prussian blue staining was executed on the cardiac tissues to monitor the iron ion deposition. (H) The mitochondrial disturbances in the cardiac tissues of rats were observed by TEM. The arrows represented the position of the mitochondria. *p < 0.05, **p < 0.01 and ***p < 0.001 vs. I/R group.
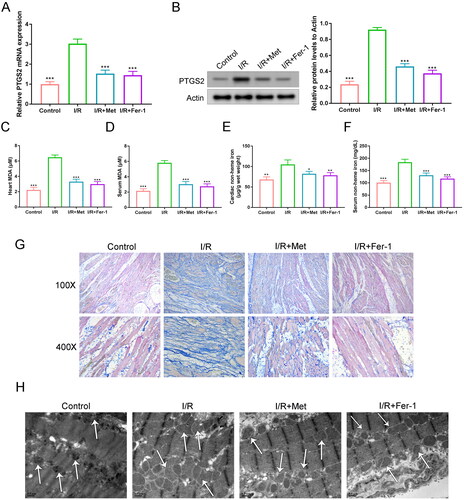
Prussian blue staining was conducted on rat cardiac tissues to evaluate the deposition of iron ion. Relative to the control, the I/R + Met and the I/R + Fer-1 groups, a much intensified Prussian blue staining was exhibited in rat cardiac tissues of the I/R group (). The mitochondrial disturbances in rat cardiac tissues were observed by TEM. The cardiac mitochondria were severely distorted in rat cardiac tissues of the I/R group, when compared to the control, the I/R + Met and the I/R + Fer-1 groups (). Collectively, Met ameliorated ferroptosis induced by cardiac I/R.
Met inhibited ferroptosis of the OGD/R-induced H9c2 cells
H9c2 cells were induced by OGD/R to establish the I/R cell model. CCK-8 assay showed the attenuated viability of H9c2 cells in the OGD/R group, relative to the control, the OGD/R + Met and the OGD/R + Fer-1 groups (p < 0.01 and p < 0.001). At 72 h, the promotion rate of Met (the OGD/R + Met group) on H9c2 cell viability was about 170.0%, relative to the OGD/R group (). Besides, the levels of PTGS2 mRNA and protein were much increased in H9c2 cells of the OGD/R group when compared to the control, the OGD/R + Met and the OGD/R + Fer-1 groups (p < 0.001). In contrast to the OGD/R group, the suppression rates of PTGS2 mRNA and protein were respectively about 52.1% and 48.8% in the OGD/R + Met group ().
Figure 2. Met inhibited ferroptosis in the OGD/R-induced H9c2 cells. (A) CCK-8 assay was executed to monitor the viability of H9c2 cells. (B, C) The expression of PTGS2 mRNA and protein in H9c2 cells was explored by qRT-PCR and Western blot. (D) DCFH-DA staining of H9c2 cells was utilized for the detection of the intracellular lipid ROS level. (E) JC-1 staining was performed to evaluate the mitochondrial damage in H9c2 cells. (F, G) The levels of non-heme iron and MDA in H9c2 cells was monitored by ELISA. **p < 0.01 and ***p < 0.001 vs. OGD/R group.
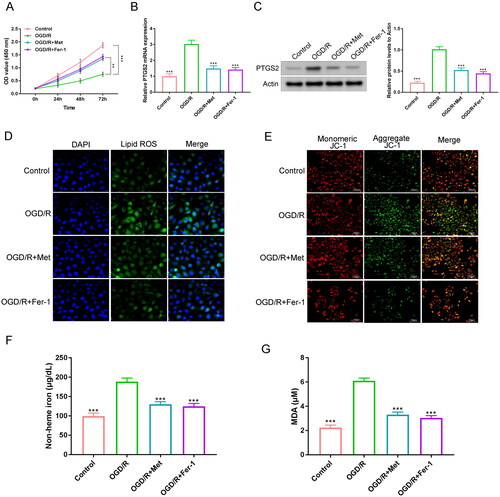
This research implemented DCFH-DA staining to assess the intracellular ROS level in H9c2 cells. Lipid ROS presented as green fluorescence. H9c2 cells of the OGD/R group exhibited much intensified green fluorescence staining than the control, the OGD/R + Met and the OGD/R + Fer-1 groups (). Additionally, JC-1 staining was used to detect the mitochondrial damage in H9c2 cells. By JC-1 staining, healthy mitochondria exhibited red fluorescence, while damaged mitochondria showed green fluorescence. As shown in , much intensified green fluorescence staining was discovered in H9c2 cells of the OGD/R group, in comparison to the control, the OGD/R + Met and the OGD/R + Fer-1 groups.
ELISA was utilized to evaluate the levels of non-heme iron and MDA in H9c2 cells. Significantly increased non-heme iron and MDA levels were shown in H9c2 cells of the OGD/R group when matched to the control, the OGD/R + Met and the OGD/R + Fer-1 groups (p < 0.001). Matched to the OGD/R group, an inhibition rate of about 30.1% for non-heme iron and about 47.9% for MDA level was shown in the OGD/R + Met group (). Thereby, Met inhibited ferroptosis in the OGD/R-induced H9c2 cells.
Met improved survival and cardiac function of rats with I/R
The 28-day survival rate of I/R rats was analysed. Much lower 28-day survival was found in rats of the I/R group, when matched to the control, the I/R + Met and the I/R + Fer-1 groups (p < 0.05 and p < 0.001) (). Rats of the I/R group showed higher serum CK-MB and LDH levels than the control, the I/R + Met and the I/R + Fer-1 groups (p < 0.01 and p < 0.001). When compared to the I/R group, the inhibition rates of serum CK-MB and LDH were respectively about 30.6% and 34.7% in the I/R + Met group (). HE staining exhibited more severe cardiac tissue damage in rats of the I/R group, when matched to the control, the I/R + Met and the I/R + Fer-1 groups ().
Figure 3. Met improved survival and cardiac function of rats with I/R. (A) The 28-day survival rate of rats was analysed. (B, C) The serum CK-MB and LDH levels of rats were measured by ELISA. (D) HE staining of the cardiac tissues was performed to research the damage of the cardiac tissues. (E–G) The FS%, EF% and heart rate of rats were observed at 4 h and on day 14 and day 28 after reperfusion. *p < 0.05, **p < 0.01 and ***p < 0.001 vs. I/R group.
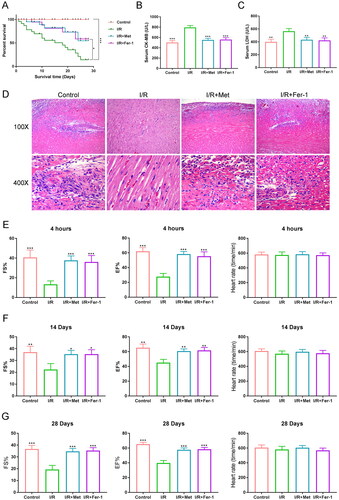
The cardiac function of rats was detected at 4 h and on day 14 and day 28 after post-reperfusion. As displayed in , at the three time points, rats of the I/R group had lower FS% and EF% than the control, the I/R + Met and the I/R + Fer-1 groups (p < 0.05, p < 0.01 or p < 0.001). Contrast to the I/R group, the FS% of the I/R + Met group was increased by 385.3%, 151.0% and 157.5% at 4 h, on day 14 and day 28, respectively. The EF% of the I/R + Met group was increased by 213.9%, 135.6% and 146.2% at 4 h, on day 14 and day 28, comparatively. However, the heart rate was similar in rats of the four groups. Therefore, Met improved survival and cardiac function of rats with I/R.
Met ameliorated ferroptosis in the OGD/R-induced H9c2 cells by reducing NOX4 via promoting AMPKα
This work detected the expression of AMPKα and NOX4 proteins in H9c2 cells. H9c2 cells of the OGD/R group expressed lower AMPKα protein and higher NOX4 protein than the control, the OGD/R + Met and the OGD/R + Fer-1 groups (p < 0.01 and p < 0.001). Compared to the OGD/R group, H9c2 cells of the OGD/R + Met group had a promotion rate of 390.0% on AMPKα protein and an inhibition rate of 39.5% on NOX4 protein (). AMPKα siRNA was transfected into H9c2 cells. The transfection efficiency is shown in . H9c2 cells of the AMPKα-siRNA group expressed lower AMPKα mRNA and protein when compared to the si-NC group (p < 0.001), indicating a successful transfection of H9c2 cells. Lower AMPKα protein and higher NOX4 protein were found in H9c2 cells of the OGD/R group when relative to the control and the OGD/R + Met groups (p < 0.001). Intriguingly, matched to the OGD/R + Met group, H9c2 cells of the OGD/R + Met + AMPKα-siRNA group expressed lower AMPKα protein and higher NOX4 protein (p < 0.05 and p < 0.001). Relative to the OGD/R + Met group, H9c2 cells of the OGD/R + Met + AMPKα-siRNA group exhibited a suppression rate of 82.1% on AMPKα protein and a promotion rate of 136.4% on NOX4 protein (). Thus, AMPKα siRNA was successfully transfected into H9c2 cells.
Figure 4. Met ameliorated ferroptosis in the OGD/R-induced H9c2 cells by reducing NOX4 via promoting AMPKα. (A) The expression of AMPKα and NOX4 proteins in H9c2 cells was detected by Western blots. (B) The transfection efficiency of H9c2 cells was evaluated by qRT-PCR and Western blots. (C) Western blot of AMPKα and NOX4 proteins in H9c2 cells of each group. (D) The viability of H9c2 cells was researched by CCK-8 assay. (E) JC-1 staining was performed to evaluate the mitochondrial damage in H9c2 cells. (F, G) Non-heme iron and MDA levels in H9c2 cells was monitored by ELISA. **p < 0.01 and ***p < 0.001 vs. OGD/R group or si-NC group. #p < 0.05 and ###p < 0.001 vs. OGD/R + Met group.
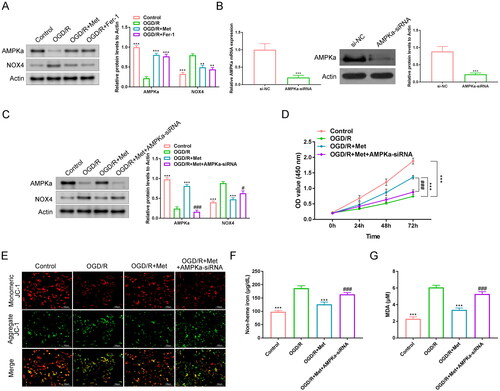
Subsequently, the viability of H9c2 cells was investigated by the CCK-8 assay. Lower viability was shown in H9c2 cells of the OGD/R group in comparison to the control and the OGD/R + Met groups (p < 0.001). Interestingly, the H9c2 cell viability of the OGD/R + Met + AMPKα-siRNA group was greatly reduced when compared to the OGD/R + Met group (p < 0.001). Matched to the OGD/R + Met group, the inhibition rate of H9c2 cell viability in the OGD/R + Met + AMPKα-siRNA group was about 34.6% (). JC-1 staining exhibited greatly intensified green fluorescence staining in H9c2 cells of the OGD/R group relative to the control and the OGD/R + Met groups. Compared to the OGD/R + Met group, the green fluorescence staining was obviously intensified in H9c2 cells of the OGD/R + Met + AMPKα-siRNA group (). Simultaneously, distinctly higher non-heme iron and MDA levels were observed in H9c2 cells of the OGD/R group, in contrast to the control and the OGD/R + Met groups (p < 0.001). However, relative to the OGD/R + Met group, H9c2 cells of the OGD/R + Met + AMPKα-siRNA group had a much increase in non-heme iron and MDA levels (p < 0.001). In comparison to the OGD/R + Met group, the promotion rates of non-heme iron and MDA levels were respectively 132.5% and 155.9% in the OGD/R + Met + AMPKα-siRNA group (). All of these results implied that Met ameliorated ferroptosis in the OGD/R-induced H9c2 cells by reducing NOX4 expression via promoting AMPKα.
Met promoted AMPKα to suppress NOX4 in cardiac I/R
The expression of AMPKα and NOX4 proteins in the cardiac tissues of rats was investigated by immunohistochemistry and Western blot. Matched to the control group, lower AMPKα protein and higher NOX4 protein occurred in rat cardiac tissues of the I/R group (p < 0.001). However, higher AMPKα protein and lower NOX4 protein were found in rat cardiac tissues of the I/R + Met group, when relative to the I/R group (p < 0.001). Relative to the I/R group, Met (the I/R + Met group) increased AMPKα protein by 344.4%, and decreased NOX4 protein by 65.1% (). Thus, Met elevated AMPKα expression and reduced NOX4 expression in the cardiac tissues of I/R rats.
Figure 5. Met promoted AMPKα to suppress NOX4 in cardiac I/R. (A, B) Immunohistochemistry and Western blot were utilized to research the expression of AMPKα and NOX4 proteins in the cardiac tissues of rats. ***p < 0.001 vs. I/R group. (C) Western blot was implemented to verify the expression of AMPKα and NOX4 proteins in H9c2 cells. **p < 0.01 and ***p < 0.001 vs. OGD/R group.
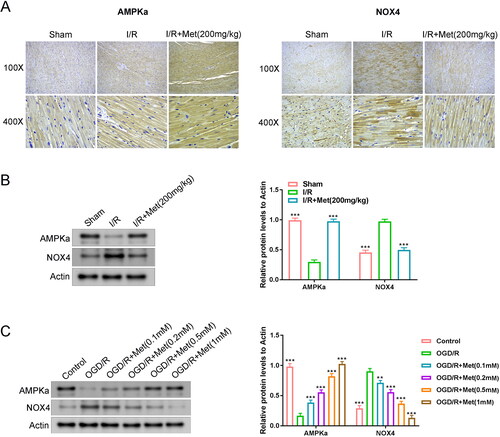
This study applied different concentrations of Met to treat the OGD/R-induced H9c2 cells. The results are listed in . Matched to the control group, lower AMPKα protein and higher NOX4 protein occurred in H9c2 cells of the OGD/R group. However, Met treatment increased the expression of AMPKα protein and reduced the expression of NOX4 protein in the OGD/R-induced H9c2 cells (p < 0.01 and p < 0.001). Hence, Met promoted AMPKα to suppress NOX4 expression in cardiac I/R.
Discussion
The current work investigated the effect of Met on cardiac I/R injury. It was implied that Met ameliorated ferroptosis in cardiac I/R rat by suppressing NOX4 via promoting AMPKα. Previous studies have suggested that Met could activate Sirtuin-1 (SIRT1) to down-regulate p53/miR-34a to ameliorate the cardiac I/R-induced apoptosis of cardiomyocytes (Li W et al. Citation2021). Simultaneously, in 90 cardiac infarction cases with ST-segment elevation, the regular use of Met before infarction has been suggested to reduce serum miR-34a, serum creatine kinase MBmass (CKMB) activity, and improve the ST-segment recovery of patients (Li W et al. Citation2021). Moreover, Met has been revealed to exert a protective effect on cardiac I/R, as it depresses the apoptosis of cardiomyocytes and mitigates the mitochondrial damage in the cardiac tissues of rat with cardiac I/R. The mechanism may be that Met intensifies the expression of six-transmembrane epithelial antigen of prostate 4 (STEAP4) in the cardiomyocytes (Luo et al. Citation2019). Currently, several reports have demonstrated the inhibitory effect of Met on ferroptosis in some human diseases, especially in malignant tumours (Hsu et al. Citation2021; Yang et al. Citation2021). However, whether Met alleviates cardiac I/R injury through suppressing ferroptosis has never been determined.
This study used Fer-1 (an inhibitor of ferroptosis) and Met to treat cardiac I/R rats and OGD/R-induced H9c2 cells. It was revealed that Met possessed a similar effect on the cardiac I/R injury as Fer-1. Both Met treatment and Fer-1 treatment could suppress the expression of PTGS2 in the cardiac tissues of cardiac I/R rats and the OGD/R-induced H9c2 cells. PTGS2 is an important marker of ferroptosis, possessing a promotion effect on ferroptosis (Li N et al. Citation2020). Further, in this article, Met treatment and Fer-1 treatment reduced the levels of MDA, non-heme iron, CK-MB and LDH in the cardiac tissues of cardiac I/R rats and the OGD/R-induced H9c2 cells, repressed the concentration of iron ion in the cardiac tissues of cardiac I/R rats, and restrained the accumulation of ROS in the OGD/R-induced H9c2 cells. As we know, the main cause of ferroptosis is the deposition of iron and the accumulation of ROS driven by the lipid peroxidation (Ma et al. Citation2020; Shan et al. Citation2021; Stamenkovic et al. Citation2021). Thus, Met could attenuate the content of iron ion, lipid peroxidation, and the accumulation of ROS, thereby ameliorating the cardiac I/R injury. Myocardial tissue damage and mitochondrial damage are critical contributors of cardiac I/R injury (Wang and Zhou Citation2020). In this work, Met treatment and Fer-1 treatment mitigated the mitochondrial and myocardial tissue damage in the cardiac I/R rats and the OGD/R-induced H9c2 cells, and improved the heart function and survival of cardiac I/R rats. Additionally, this study detected the heart injury of rat immediately after I/R, the cardiac function of rats at 4 h and on days 14 and 28 after reperfusion, and the 28-day survival of rats. For in vitro experiments, the acute injury was selected. It has been reported that the reperfusion process can lead to severe acute cardiac injury adverse events, such as the enhanced myocardial cell death, the enlarged infarct areas and the aggravated cardiac dysfunction. The irreversible damage to the heart resulting from these acute adverse events is the main cause of eventual patient death (Lahnwong et al. Citation2020; Ge et al. Citation2021). Thus, this paper selected acute injury for in vitro experiments.
As previously reported, Met could restrict the expression of NOX4 by up-regulating AMPK, thereby attenuating the oxidative damage and injury after cardiac I/R (Shi and Hou Citation2021). Moreover, Met exhibited a protective effect on cardiac I/R by suppressing the activation of the NLRP3 inflammasome via activating the AMPK pathway (Zhang et al. Citation2020). Similarly, this study uncovered that Met and Fer-1 treatment could promote the expression of AMPKα and reduce the expression of NOX4 in the cardiac tissues of cardiac I/R rats and the OGD/R-induced H9c2 cells. Intriguingly, AMPKα silencing abrogated the suppression of Met and Fer-1 on ferroptosis and the NOX4 expression in the OGD/R-induced H9c2 cells. Therefore, Met might ameliorate ferroptosis in cardiac I/R by reducing NOX4 via promoting AMPKα. AMPK is a kind of stress-activated kinase, possessing a protective effect on cardiac I/R, such as improving the mitochondrial function and restraining the inflammatory response induced by cardiac I/R (Chen et al. Citation2018). Moreover, AMPK exerts a protective effect on the I/R injury of multiple organs, including heart, kidney, lung, brain and liver, through suppressing oxidative stress, inflammatory response, endoplasmic reticulum stress and mitochondrial function. It is thus considered to be a promising treatment target for I/R injury (Ding et al. Citation2020). Recently, activated AMPK has been implied to repress ferroptosis (Lee et al. Citation2020). NOX4 is a key generator of ROS, and the inhibition of NOX4 can ameliorate the heart function of mice with cardiac I/R (Szekeres et al. Citation2021). Regarding ferroptosis, NOX4 has been found to induce ferroptosis in astrocytes by impairing the mitochondrial metabolism and promoting the lipid peroxidation (Park et al. Citation2021). This paper proved that Met ameliorated ferroptosis in cardiac I/R by inhibiting NOX4 via enhancing AMPKα expression. The study by Shi and Hou (Citation2021) suggested that Met could relieve the oxidative stress injury and apoptosis of cardiomyocytes after cardiac I/R by inhibiting NOX4 via activating AMPK. However, they did not study the influence of Met on ferroptosis in cardiac I/R. This work uncovered a new mechanism by which Met ameliorated cardiac I/R injury by attenuating ferroptosis through suppressing NOX4 via activating AMPKα. This study provided a more precise molecular basis for Met to be utilized in the clinical treatment of cardiac I/R injury.
This study has limitations. The peak time of ferroptosis after cardiac I/R should be researched. Besides, in the fifth part of the result section, it was noticed that, the higher dose of Met (from 0.1mM to 1 mM), the more pronounced the promotion of AMPKα expression and the inhibition of NOX4 expression in the OGD/R-induced H9c2 cells. Thus, it is better to further investigate whether this effect of Met is in a dose-dependent manner. However, these experiments cannot be performed at present due to the limitations of the laboratory. This issue will be the focus of our research.
This work explored the effect of Met on the cardiac I/R injury. The results implied that Met possessed a protective effect on the cardiac I/R injury. It could ameliorate ferroptosis in cardiac I/R by reducing NOX4 via promoting AMPKα. Thus, Met may be a promising drug in the clinical treatment of cardiac I/R injury.
Disclosure statement
No potential conflict of interest was reported by the author(s).
Data availability statement
The authors confirm that the data supporting the findings of this study are available within the article.
Additional information
Funding
References
- Benjanuwattra J, Apaijai N, Chunchai T, Kerdphoo S, Jaiwongkam T, Arunsak B, Wongsuchai S, Chattipakorn N, Chattipakorn SC. 2020. Metformin preferentially provides neuroprotection following cardiac ischemia/reperfusion in non-diabetic rats. Biochim Biophys Acta Mol Basis Dis. 1866(10):165893.
- Chen X, Li X, Zhang W, He J, Xu B, Lei B, Wang Z, Cates C, Rousselle T, Li J. 2018. Activation of AMPK inhibits inflammatory response during hypoxia and reoxygenation through modulating JNK-mediated NF-κB pathway. Metabolism. 83:256–270.
- Denorme F, Manne BK, Portier I, Eustes AS, Kosaka Y, Kile BT, Rondina MT, Campbell RA. 2020. Platelet necrosis mediates ischemic stroke outcome in mice. Blood. 135(6):429–440.
- Ding R, Wu W, Sun Z, Li Z. 2020. AMP-activated protein kinase: an attractive therapeutic target for ischemia–reperfusion injury. Eur J Pharmacol. 888:173484.
- Ge X, Meng Q, Wei L, Liu J, Li M, Liang X, Lin F, Zhang Y, Li Y, Liu Z, et al. 2021. Myocardial ischemia–reperfusion induced cardiac extracellular vesicles harbour proinflammatory features and aggravate heart injury. Extracell Vesicles. 10:e12072.
- Hsu S-K, Cheng K-C, Mgbeahuruike MO, Lin Y-H, Wu C-Y, Wang H-MD, Yen C-H, Chiu C-C, Sheu S-J. 2021. New insight into the effects of metformin on diabetic retinopathy, aging and cancer: nonapoptotic cell death, immunosuppression, and effects beyond the AMPK pathway. Int J Mol Sci. 22(17):9453.
- Lahnwong S, Palee S, Apaijai N, Sriwichaiin S, Kerdphoo S, Jaiwongkam T, Chattipakorn SC, Chattipakorn N. 2020. Acute dapagliflozin administration exerts cardioprotective effects in rats with cardiac ischemia/reperfusion injury. Cardiovasc Diabetol. 19(1):91.
- Lee H, Zandkarimi F, Zhang Y, Meena JK, Kim J, Zhuang L, Tyagi S, Ma L, Westbrook TF, Steinberg GR, et al. 2020. Energy-stress-mediated AMPK activation inhibits ferroptosis. Nat Cell Biol. 22(2):225–234.
- Li N, Wang W, Zhou H, Wu Q, Duan M, Liu C, Wu H, Deng W, Shen D, Tang Q. 2020. Ferritinophagy-mediated ferroptosis is involved in sepsis-induced cardiac injury. Free Radic Biol Med. 160:303–318.
- Li T, Tan Y, Ouyang S, He J, Liu L. 2022. Resveratrol protects against myocardial ischemia–reperfusion injury via attenuating ferroptosis. Gene. 808:145968.
- Li W, Jin S, Hao J, Shi Y, Li W, Jiang L. 2021. Metformin attenuates ischemia/reperfusion-induced apoptosis of cardiac cells by downregulation of p53/microRNA-34a via activation of SIRT1. Can J Physiol Pharmacol. 99(9):875–884.
- Liao S, Apaijai N, Luo Y, Wu J, Chunchai T, Singhanat K, Arunsak B, Benjanuwattra J, Chattipakorn N, Chattipakorn SC. 2021. Cell death inhibitors protect against brain damage caused by cardiac ischemia/reperfusion injury. Cell Death Discov. 7(1):312.
- Liu X, Qi K, Gong Y, Long X, Zhu S, Lu F, Lin K, Xu J. 2021. Ferulic acid alleviates myocardial ischemia reperfusion injury via upregulating AMPKα2 expression-mediated ferroptosis depression. J Cardiovasc Pharmacol. 79(4):489–500.
- Luo T, Zeng X, Yang W, Zhang Y. 2019. Treatment with metformin prevents myocardial ischemia–reperfusion injury via STEAP4 signaling pathway. Anatol J Cardiol. 21(5):261–271.
- Ma S, Sun L, Wu W, Wu J, Sun Z, Ren J. 2020. USP22 protects against myocardial ischemia–reperfusion injury via the SIRT1-p53/SLC7A11-dependent inhibition of ferroptosis-induced cardiomyocyte death. Front Physiol. 11:551318.
- Park MW, Cha HW, Kim J, Kim JH, Yang H, Yoon S, Boonpraman N, Yi SS, Yoo ID, Moon JS. 2021. NOX4 promotes ferroptosis of astrocytes by oxidative stress-induced lipid peroxidation via the impairment of mitochondrial metabolism in Alzheimer’s diseases. Redox Biol. 41:101947.
- Shan X, Lv Z-Y, Yin M-J, Chen J, Wang J, Wu Q-N. 2021. The protective effect of cyanidin-3-glucoside on myocardial ischemia–reperfusion injury through ferroptosis. Oxid Med Cell Longev. 2021:8880141.
- Shi Y, Hou SA. 2021. Protective effects of metformin against myocardial ischemia–reperfusion injury via AMPK‑dependent suppression of NOX4. Mol Med Rep. 24(4):712.
- Stamenkovic A, O'Hara KA, Nelson DC, Maddaford TG, Edel AL, Maddaford G, Dibrov E, Aghanoori M, Kirshenbaum LA, Fernyhough P, et al. 2021. Oxidized phosphatidylcholines trigger ferroptosis in cardiomyocytes during ischemia–reperfusion injury. Am J Physiol Heart Circ Physiol. 320(3):H1170–H1184.
- Sun L, Wang H, Yu S, Zhang L, Jiang J, Zhou Q. 2021. Herceptin induces ferroptosis and mitochondrial dysfunction in H9c2 cells. Int J Mol Med. 49(2):17.
- Szekeres FLM, Walum E, Wikström P, Arner A. 2021. A small molecule inhibitor of Nox2 and Nox4 improves contractile function after ischemia–reperfusion in the mouse heart. Sci Rep. 11(1):11970.
- Wang J, Zhou H. 2020. Mitochondrial quality control mechanisms as molecular targets in cardiac ischemia–reperfusion injury. Acta Pharm Sin B. 10(10):1866–1879.
- Wu X, Li Y, Zhang S, Zhou X. 2021. Ferroptosis as a novel therapeutic target for cardiovascular disease. Theranostics. 11(7):3052–3059.
- Xu S, Wu B, Zhong B, Lin L, Ding Y, Jin X, Huang Z, Lin M, Wu H, Xu D. 2021. Naringenin alleviates myocardial ischemia/reperfusion injury by regulating the nuclear factor-erythroid factor 2-related factor 2 (Nrf2)/system xc-/glutathione peroxidase 4 (GPX4) axis to inhibit ferroptosis. Bioengineered. 12(2):10924–10934.
- Yang J, Zhou Y, Xie S, Wang J, Li Z, Chen L, Mao M, Chen C, Huang A, Chen Y. 2021. Metformin induces ferroptosis by inhibiting UFMylation of SLC7A11 in breast cancer. Exp Clin Cancer Res. 40:206.
- Zhang J, Huang L, Shi X, Yang L, Hua F, Ma J, Zhu W, Liu X, Xuan R, Shen Y, et al. 2020. Metformin protects against myocardial ischemia–reperfusion injury and cell pyroptosis via AMPK/NLRP3 inflammasome pathway. Aging. 12(23):24270–24287.
- Zhao W-K, Zhou Y, Xu T-T, Wu Q. 2021. Ferroptosis: opportunities and challenges in myocardial ischemia–reperfusion injury. Oxid Med Cell Longev. 2021:9929687.