1. Introduction
Acute myeloid leukemia (AML) is a heterogeneous disease characterized by genetic alterations and molecular abnormalities that drive the aberrant proliferation and differentiation of myeloid cells. Despite advances in target therapies, the prognosis for AML patients remains poor, necessitating the exploration of novel therapeutic strategies. The Clustered Regularly Interspaced Short Palindromic Repeats (CRISPR) gene editing technology, characterized by its programmability, simplified design process, and precision in targeting genes, offers numerous advantages such as ease of use, complete inactivation, high reproducibility and efficiency, and cost-effectiveness compared to RNA interference and other gene editing technologies such as ZFNs (zinc finger nucleases) and TALENs (transcription activator-like effector nucleases). The emergence of multiplexed genome editing CRISPR screening platform has opened new avenues for investigating the genomic landscape of AML and discovering new potential therapeutic targets.
2. CRISPR screens have revealed novel therapeutic targets for AML
CRISPR screen is a robust methodology for comprehensive biological exploration, allowing unbiased studies into gene function and yielding pivotal insights into diseases. The screen can be performed as in vitro, in vivo, and single-cell screens. In vitro screens are conducted in controlled laboratory settings, providing a controlled and reproducible environment for experiments. On the other hand, in vivo screens provide a more realistic representation of biological/pathological systems, capturing the complexity of interactions within living organisms. Notably, the emerging field of single-cell CRISPR screening involves precision gene manipulation at the individual cell level, facilitating an in-depth examination of genetic responses and variations within heterogeneous cell populations. Collectively, in vitro, in vivo, and single-cell CRISPR screens present versatile methodologies for genetic exploration, offering important insights that may contribute to the identification of novel therapeutic targets for AML ().
Figure 1. The illustration depicts the experimental procedures of in vitro, in vivo and single cell screens.
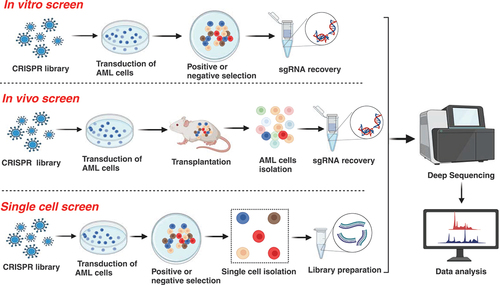
2.1. In vitro screens
The CRISPR system made its first breakthrough in 2013 by successfully editing genes in mammalian cells [Citation1,Citation2]. In the following year, the system was utilized for genome-scale screening, leading to the establishment of the CRISPR-Cas9 knockout (GeCKO) sgRNA library [Citation3,Citation4]. In the past several years, numerous CRISPR-based genomic screens were performed to identify functionally essential genes in AML. Tzelepis et al. developed an optimized CRISPR dropout screening platform to investigate genetic vulnerabilities in acute myeloid leukemia (AML). Through their experiments, they identified the histone acetyltransferase gene KAT2A (also known as GCN5) as a potential target for AML therapy. Inhibiting KAT2A was found to effectively suppress AML cell proliferation by inhibiting leukemogenic transcriptional programs and promoting cell differentiation, ultimately leading to apoptosis, while preserving normal cell function and viability [Citation5]. Moreover, Wang et al. developed cell surface antigen-guided genome-wide CRISPR screening to uncover genes that maintain the undifferentiated state, which is a defining characteristic of leukemia cells. They identified that one critical regulator of AML maintenance and differentiation is the RNA-binding protein ZFP36L2, which could interact with the 3’ untranslated region of key myeloid maturation genes, including the ZFP36 paralogs, to promote their mRNA degradation and suppress terminal myeloid cell differentiation. These results suggest that genetic inhibition of ZFP36L2 restores the mRNA stability of these targeted transcripts and ultimately triggers myeloid differentiation in leukemia cells [Citation6]. Furthermore, by taking advantage of CRISPR-based genomic screening, many other genes have also been confirmed as candidate therapeutic targets in AML, including the Ikaros Family Zinc Finger 1 (IKZF1), v-Crk Avian Sarcoma Virus CT10 Oncogene Homolog-Like (CRKL), and Phosphatidylinositol 3-kinase catalytic subunit type 3 (PIK3C3) [Citation7,Citation8].
CRISPR-based pooled genetic screens have also gained extensive use in the exploration of key factors responsible for drug resistance in AML. In a CRISPR-based loss-of-function screen, we identified SPRY3 and GSK3 as the leading hits. SPRY3 and GSK3 are key negative regulators of Ras/MAPK and Wnt signaling, respectively. Accordingly, we discovered that re-activations of these signaling pathways in AML are major mechanisms that confer resistance to a FLT3 inhibitor Quizartinib (AC220) [Citation9]. Similarly, Ling et al. conducted a genome-wide CRISPR knockout screen in human AML cell lines to unravel the underlying resistance mechanisms associated with the primary treatment for AML, doxorubicin, and cytarabine (Dox/AraC). It was confirmed that the gene responsible for AraC metabolism, cyclin-dependent kinase inhibitor 2A (CDKN2A), along with genes involved in regulating cell cycle arrest, checkpoint kinase 2 (CHEK2), and TP53, are all contributing to the resistance. Moreover, the study sheds light on a potentially promising therapeutic approach by highlighting the efficacy of venetoclax in overcoming resistance caused by impaired cell cycle arrest due to CDKN2A and CHEK2 dysregulation, offering a novel therapeutic strategy for AML patients facing such challenges [Citation10].
2.2. In vivo screens
While most genome-wide screenings have been primarily conducted in vitro, in vivo screening holds greater promise as it allows for the assessment of candidate gene essentiality within the microenvironment of a living organism, potentially influencing the physiological behavior of cancer cells. Mercier et al. conducted genome-wide CRISPR screens in murine models of AML for identifying genes, which are essential for interactions with the microenvironment in vivo. They confirmed that 72 genes including components of the major histocompatibility complex class I complex (CD47), complement receptor (Cr1l), and the b-4-galactosylation pathway are specifically essential for leukemic growth in vivo. More importantly, some genes like Fermt3, a master regulator of integrin signaling, have in vivo – specific dependency with high prognostic relevance [Citation11]. Likewise, the integration of a synergistic in vivo screening approach with CRISPR-competent PDX models elucidated the sodium/myo-inositol cotransporter (SLC5A3) as a metabolic vulnerability for AML, concurrently unveiling mitochondrial-associated RING finger 5 (MARCH5) as a pivotal guardian against apoptosis in AML [Citation12].
2.3. Single cell screens
Various hematological diseases, such as leukemia, lymphoma, and multiple myeloma, are characterized by significant genetic and phenotypic heterogeneities [Citation13]. This inherent diversity poses challenges for the conventional pooled CRISPR screenings. However, a recent breakthrough has addressed this limitation by integrating single-cell RNA sequencing (scRNA-seq) with CRISPR screening, employing innovative methods such as Perturb-Seq, CRISP-seq, and CROP-seq [Citation14]. Notably, Wessels et al. have introduced a novel approach known as Cas13 RNA Perturb-seq (CaRPool-seq), which utilized RNA-targeting CRISPR – Cas13d system to achieve effective combinational perturbations while facilitating multimodal single-cell profiling. Subsequently, CaRPool-seq was employed to perform multiplexed combinational perturbations of myeloid differentiation regulators in an AML model system. Through this process, the study unveiled extensive interactions between various chromatin regulators that can either enhance or suppress AML differentiation phenotypes [Citation15].
3. Conclusion
The multiplexed genome editing CRISPR screening platform represents a state-of-the-art and robust tool for extensively exploring complex genetic landscapes and discovering novel therapeutic targets for AML. Numerous research endeavors have focused on identifying AML-related genes governing cell fate and drug resistance through genome CRISPR screenings conducted in both in vitro and in vivo settings. Combining single-cell RNA-seq with genome CRISPR screening provides profound insights into cell-to-cell variation in AML and the microenvironment, facilitating high-resolution dissection of the disease’s pathogenic mechanisms and offering potential clinical applications. A summary of the representative in vitro and in vivo CRISPR screens conducted in AML to date is provided in . Importantly, the screen results are significantly influenced by the context of the screening process. If the identified hits in different screens, such as in vivo versus single-cell screens, do not overlap, it mostly reflects inherent differences between different screening approaches. For example, biological complexity is most pronounced in in vivo screens. Furthermore, in both in vitro and in vivo screens, the emphasis is on a population level, while single-cell screens prioritize individual cells, effectively addressing the inherent heterogeneity within the cell population.
Table 1. Representative in vitro and in vivo CRISPR screens pertaining to AML.
While genome editing CRISPR screening offers numerous advantages, it is also accompanied by inherent disadvantages and limitations. Unintended mutations, known as off-target effects, as well as instances of incomplete knockouts, can introduce complexities that hinder the accurate identification of new therapeutic targets. Furthermore, the intricacies of data analysis and the influence of cell type-specific effects present challenges in interpreting the results. Nevertheless, despite these challenges, CRISPR screening remains an indispensable and powerful tool for exploring intricate genetic landscapes and pinpointing potential therapeutic targets within the context of AML. The ongoing progress in technology and the continuous refinement of genome CRISPR screening hold immense promise in reshaping the landscape of precision medicine and deepening our understanding of AML pathogenesis.
4. Expert opinion
CRISPR screening emerges as a cutting-edge technology with significant potential to advance our understanding of AML. Through systematic exploration of the entire genome, CRISPR screens facilitate the identification of genetic factors propelling AML development, progression, and response to therapeutics. This groundbreaking approach empowers researchers to precisely manipulate genes at the population or individual cell level, presenting a powerful tool for a comprehensive investigation into gene functions in AML. The enthusiasm surrounding CRISPR screens in AML research is rooted in their capacity to discover novel therapeutic targets. This technology not only provides a deeper understanding of the molecular mechanisms underpinning AML but also opens avenues for discovering potential new targets for therapeutic intervention. Nevertheless, the potential for off-target effects in the context of CRISPR raises concerns about the unpredictable consequences of screening. This underscores the importance of continuous research and technological advancements aimed at minimizing off-target effects and enhancing the precision of CRISPR-based screening for AML research.
Declaration of interests
The authors have no relevant affiliations or financial involvement with any organization or entity with a financial interest in or financial conflict with the subject matter or materials discussed in the manuscript. This includes employment, consultancies, honoraria, stock ownership or options, expert testimony, grants or patents received or pending, or royalties.
Article highlights
AML therapies remain challenging despite advances. The simplicity, efficiency, and cost-effectiveness of CRISPR gene editing surpass other methods, creating exciting avenues for AML research.
Genome-wide CRISPR screens elucidate pivotal genes implicated in AML pathogenesis and drug resistance.
In vivo CRISPR screens unveil indispensable genes governing AML growth within the microenvironment.
Integrating CRISPR with single-cell RNA sequencing exposes nuanced cell-to-cell heterogeneity in AML, facilitating the discovery of novel targeted therapeutic interventions.
Reviewer disclosures
A reviewer on this manuscript has disclosed they have a patent pending on a fusion detection assay based on CRISPR enrichment. Peer reviewers on this manuscript have no other relevant financial relationships or otherwise to disclose.
Additional information
Funding
References
- Cong L, Ran FA, Cox D, et al. Multiplex genome engineering using CRISPR/Cas systems. Science. 2013;339(6121):819–823. doi: 10.1126/science.1231143
- Mali P, Yang LH, Esvelt KM, et al. RNA-guided human genome engineering via Cas9. Science. 2013;339(6121):823–826. doi: 10.1126/science.1232033
- Shalem O, Sanjana NE, Hartenian E, et al. Genome-scale CRISPR-Cas9 knockout screening in human cells. Science. 2014;343(6166):84–87. doi: 10.1126/science.1247005
- Sanjana NE, Shalem O, Zhang F. Improved vectors and genome-wide libraries for CRISPR screening. Nat Methods. 2014;11(8):783–784. doi: 10.1038/nmeth.3047
- Tzelepis K, Koike-Yusa H, De Braekeleer E, et al. A CRISPR dropout screen identifies genetic vulnerabilities and therapeutic targets in acute myeloid leukemia. Cell Rep. 2016;17(4):1193–1205. doi: 10.1016/j.celrep.2016.09.079
- Wang E, Zhou H, Nadorp B, et al. Surface antigen-guided CRISPR screens identify regulators of myeloid leukemia differentiation. Cell Stem Cell. 2021;28(4):718–731. doi: 10.1016/j.stem.2020.12.005
- Aubrey BJ, Cutler JA, Bourgeois W, et al. IKAROS and MENIN coordinate therapeutically actionable leukaemogenic gene expression in MLL-r acute myeloid leukaemia. Nat Cancer. 2022;3(5):595–613. doi: 10.1038/s43018-022-00366-1
- Takacs G, Zhou Y, King DJ, et al. CRISPR dropout screens identify DHODH, PIK3C3, and crkl as potential therapeutic targets in acute myeloid leukemia. Blood. 2019;134(Supplement_1):1452. doi: 10.1182/blood-2019-128180
- Hou PP, Wu C, Wang YC, et al. A genome-wide CRISPR screen identifies genes critical for resistance to FLT3 inhibitor AC220. Cancer Res. 2017;77(16):4402–4413. doi: 10.1158/0008-5472.CAN-16-1627
- Ling VY, Straube J, Godfrey W, et al. Targeting cell cycle and apoptosis to overcome chemotherapy resistance in acute myeloid leukemia. Leukemia. 2023;37(1):143–153. doi: 10.1038/s41375-022-01755-2
- Mercier FE, Shi JT, Sykes DB, et al. In vivo genome-wide CRISPR screening in murine acute myeloid leukemia uncovers microenvironmental dependencies. Blood Adv. 2022;6(17):5072–5084. doi: 10.1182/bloodadvances.2022007250
- Lin S, Larrue C, Scheidegger NK, et al. An in vivo CRISPR screening platform for prioritizing therapeutic targets in AML. Cancer Discov. 2022;12(2):432–449. doi: 10.1158/2159-8290.CD-20-1851
- Zhu Y, Huang YH, Tan Y, et al. Single-cell RNA sequencing in hematological diseases. Proteomics. 2020;20(13):e1900228. doi: 10.1002/pmic.201900228
- Duan B, Zhou C, Zhu CY, et al. Model-based understanding of single-cell CRISPR screening. Nat Commun. 2019;10(1):2233. doi: 10.1038/s41467-019-10216-x
- Wessels HH, Méndez-Mancilla A, Hao YH. Efficient combinatorial targeting of RNA transcripts in single cells with Cas13 RNA Perturb-seq. Nat Methods. 2023;20(1):86–94. doi: 10.1038/s41592-022-01705-x