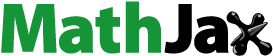
ABSTRACT
The majority of today’s fertilizers are based on mineral resources and fossil fuels. Among other factors, geopolitical sensitivities and rising fertilizer demand contribute to high and volatile prices. To reduce our dependency on non-renewable resources, valuable bio-based fertilizer components can be recovered through valorization of organic waste streams. However, from a farmer’s perspective, an optimization problem arises: the farmer wants to minimize costs while safeguarding crop nutrient uptake. Optimization research in the fertilizer domain focuses on scheduling fertilizer application or minimizing manure management costs. The model developed in this paper intends to minimize fertilizer costs for an individual vegetable farmer by considering the possibility of bio-based fertilizer prosumption through composting and anaerobic digestion as well as leaving crop residues on the field. In a Linear Programming model, these options are compared to purchasing mineral fertilizer, commercial compost or pig slurry. In addition, a distinction is made between different business models. Sensitivity analysis is carried out with respect to changing cost parameters. The results confirm that it is not optimal for a Flemish vegetable farmer to fulfil nutrient needs solely with bio-based fertilizer. Nevertheless, the study shows that it can be interesting to consider value chains involving regional cooperation.
1. Introduction
Today’s crop production is heavily dependent on mineral fertilizers, relying on natural gas through the Haber–Bosch process for nitrogen (N) production and mining for phosphorus (P) and potassium (K). Europe, in particular, is highly dependent on nutrient imports: nitrate-based fertilizers are imported mainly from Russia, Egypt and Algeria, while phosphate-based products are mainly sourced from Morocco and potassium chloride imports mainly come from Russia and Belarus (European Commission, Citation2019). At the same time, valorization of food waste can provide nutrient recovery opportunities. Approximately 129 Mt of food waste is generated annually along the European food supply chain, with contributions of 25% by the primary production sector, 24% by the processing and manufacturing sectors, and respectively 5% and 46% by retail and consumption (Caldeira et al., Citation2019). Considering the primary production sector, the nutrient losses of remaining crop residues represent an argument towards the collection and valorization of crop residues. In Flanders, food waste is mainly valorized as animal feed, anaerobically digested or returned to the soil (Monitoring Flanders, Citation2017).
In addition to the imbalance in the European nutrient cycle, fertilizers contribute to a wide range of negative environmental externalities. The production of mineral fertilizers relies on the extraction of non-renewable resources such as phosphate rock, potash and natural gas; the latter is not only used as a feedstock but also for process heat and energy (Yara, Citation2020). By relying on fossil fuels, mineral fertilizer production contributes to global warming (Snyder et al., Citation2009). Ammonia production is the most energy intensive step in nitrogen fertilizer production, but it is worth to note that the sector is making an effort to produce mineral fertilizer from renewable energy to reduce their CO2-impact (Yara, Citation2020). Additionally, inadequate application of inorganic nutrients can contribute to nutrient leaching. Nutrient pollution brings along issues such as eutrophication, causing algae to grow faster than ecosystems can handle: estimates suggest that the planetary boundary for biochemical flows (i.e. phosphorus and nitrogen) have already been exceeded (Steffen et al., Citation2015). Nevertheless, this problem is not limited to mineral fertilizers. A large proportion of nutrient pollution originates from nutrient inefficiency in the livestock sector (Buckwell & Nadeu, Citation2016). At the same time, by depleting the soil of its nutrients and organic matter, long term excessive use of mineral fertilizers makes the soil unproductive and stimulate soil deterioration (Khan et al., Citation2007). As soil organic matter plays an important role in bio-geochemical cycles, changes in soil organic matter content can impact nutrient availability in soils (Buckwell & Nadeu, Citation2016).
Bio-based fertilizer production, i.e. any valorization of biomass such as post-harvest residues or residues from livestock production and food processing into fertilizer ingredients, can bridge the gap between nutrient imports and nutrient losses by contributing to a resource-efficient economy (Chojnacka et al., Citation2020). This adds resilience to the food supply chain by accounting for events of supply disruption of phosphorus or natural gas (Buckwell & Nadeu, Citation2016). Additionally, vital organic matter can return to the soil (Buckwell & Nadeu, Citation2016). Several technologies can be used to convert residues into fertilizer ingredients. Well-established existing technologies are composting and anaerobic digestion, which can be combined with other techniques including mechanical separation and reversed osmosis (Vaneeckhaute et al., Citation2013), but future research might bring along other technologies such as biochar production through pyrolysis (Clare et al., Citation2014; Glaser et al., Citation2015) or microbial biomass production (Spanoghe et al., Citation2020).
However, in order to be applied in agriculture, bio-based fertilizers need to be competitive in comparison to mineral fertilizers. From a farmer’s point of view, the cost of fertilizer use should not increase and yields should not decrease. In other words, the cost per unit of nutrient absorbed by a farmer’s crops should be lower or similar for a bio-based fertilizer compared to a mineral fertilizer. If competitive pricing is not possible, for example due to differences in production cost, a farmer needs an additional incentive to switch from mineral fertilizers to bio-based fertilizers, such as a tax or subsidy put in place to correct the negative environmental externalities related to mineral fertilizers. In Flanders, policies currently rely on command-and-control regulation, including control of farmers and sanctions in case of policy violations (VLM, Citation2022b). From a farmer’s perspective, an optimization problem arises: a farmer wants to minimize the cost of fertilizer utilization while at the same time guarding sufficient nutrient uptake by his crops as well as soil health. Additionally, when opting for on-farm residue valorization, a farmer experiences a limited availability of organic residues that can be converted into fertilizers.
Some research has already been carried out on cost optimization of fertilizer usage. For example, Keplinger and Hauck (Citation2006) developed a linear programming (LP) model to minimize costs of manure application. Similarly, Rodias et al. (Citation2019) used a combined simulation and LP method to model organic fertilizer as liquid manure with the objective of minimizing costs. Bueno-Delgado et al. (Citation2016) developed an Android application for the optimization of fertilizer cost using a list of commercial fertilizers. A related model was designed by Gebrezgabher et al. (Citation2010) to maximize profits of an anaerobic digestion plant from electricity sales and digestate application. Jareonkitpoolpol et al. (Citation2018) use a LP-approach to determine the optimal blending of organic-chemical fertilizers. Nevertheless, optimization models for fertilizer prosumers, i.e. producers that act as both producers and consumers of bio-based fertilizers, are scarce. Clare et al. (Citation2014) calculate economic savings from fertilizer use by applying biochar in China. However, fertilizer prosumption can include other technologies such as anaerobic digestion, competing with both organic and inorganic fertilizers, and the results might be different in Europe.
The LP model developed in this paper addresses this gap by developing a fertilizer cost minimization approach intended to minimize costs for an individual farmer and explores how different business models affect the outcome of the model. A farmer can choose to buy fertilizer on the market or to produce bio-based fertilizer himself. In the latter case, they are assumed to be a fertilizer prosumer: they are both the provider of biomass and the end-user of the bio-based fertilizer. The farmer can produce different kinds of bio-based fertilizer such as compost or biogas digestate, but they also have the option to leave crop residues on the field. Additionally, in producing bio-based fertilizer, the farmer can take on different business models (BM). These business models will have different cost implications and can thus lead to different optimal solutions.
In the next section, the cost minimization model will be described. First, a general LP model will be elaborated upon. Then, the changes to the model considering the different business models will be presented. The LP model will be run with data for an average leek producing horticultural farm in Flanders. Leek is an interesting case to validate, not only because of its intensive nitrogen requirements, but also because it is the most produced vegetable in open air in Belgium (Fruit Logistica, Citation2021) and it is a feasible input for mono-digestion processes (De Dobbelaere et al., Citation2022). Using an LP-approach based on these data, the optimal amount of purchased mineral and/or organic fertilizer and produced bio-based fertilizer will be calculated for each business model. We start with a baseline scenario based on currently realistic parameters, and then move to other scenarios with varying cost and pricing parameters. Based on these calculations, it will be clear which business models and which fertilizers allow the lowest cost to an individual farmer under different scenarios. The case of leek cultivation in Flanders could provide interesting insights applicable to other horticultural crops and regions.
2. Methods
In this section, we describe the methodology used in this study. In 2.1, the cost minimization model is explained, including the business model differentiation. In section 2.2, the assumed values of the variables are elaborated upon based on available data.
2.1. Cost minimization model
gives an overview of all fertilizers studied in this paper. We focus on a Flemish leek farmer. A distinction is made between urea as a mineral fertilizer and pig slurry, compost, crop residues and digestate as bio-based fertilizers. Another measure of distinction relates to the production level: for a horticultural farmer, crop residues, on-farm compost and digestate can involve fertilizer production as well as consumption. Considering all these fertilizers, a cost optimization model is formulated. The model minimizes total fertilization costs for an individual farmer while taking nutrient needs and residue availability into account. The specification of the objective function is as follows:
(1)
(1) Subject to constraints:
(2)
(2)
(3)
(3)
(4)
(4)
(5)
(5) where C is the cost of total-fertilizer application per hectare, which is minimized; mf is the application rate of purchased fertilizer f per hectare; pf is the price of fertilizer f; cb is the average cost to produce bio-based fertilizer b on the farm and mb is the application rate of fertilizer b used per hectare. Constraint 2 and 3 relate to the nitrogen and phosphorus matter of each type of fertilizer: Nf and Pf are respectively the nitrogen and phosphorus content of fertilizer f; Nb and Pb are the nitrogen and phosphorus content of prosumed bio-based fertilizer b and the minimal and maximal values Nmin, Nmax and Pmax define the required nutrient concentration range per hectare. Comparing nitrogen contents of fertilizers is not straightforward since nitrogen in bio-based fertilizers may be present in forms that are less available to plants compared to mineral fertilizers. Therefore, a nutrient availability coefficient ρ is considered. For nitrogen, this is the plant available nitrogen (PAN) coefficient. Constraint 4 relates to the Effective Organic Carbon (EOC) content of each fertilizer. EOC is the amount of carbon that can directly be digested by soil micro-organisms estimated as organic carbon present one year after input to the soil (Gobin et al., Citation2011). EOCf is the EOC content of fertilizer f; EOCb is the EOC content of prosumed fertilizer b and EOCmin is the EOC that is needed to compensate the yearly degradation of organic carbon. Since phosphorus (P) and potassium (K) are not limiting for leek fertilization, they are not considered in terms of minimal values. Constraint 5 relates to the availability of residues, with rb the volume conversion rate of organic residues to prosumed fertilizer b and T the amount of residues that a prosumer is able to collect and convert per hectare on a yearly basis.
Given constraints 2 and 4, an individual farmer will choose those fertilizers that offer the lowest price or cost per nutrient to satisfy his nutrient needs. Therefore, a bio-based fertilizer will only be attractive to an individual farmer if it offers a lower price for either PAN or EOC compared to a mineral fertilizer. If that is the case, the farmer will use the bio-based fertilizer, constrained by fertilization standards (RHS constraints 2 and 3) as well as a limited availability of residues (constraint 5), and purchase additional mineral fertilizer to satisfy his remaining nutrient needs.
Total costs of bio-based fertilizer production can be broken down into three main types of costs:
Installation costs I, including machinery, buildings and land. This is expressed as a yearly cost
with Y the lifetime of the installation;
Consumption-linked expenses, including:
o
as the cost of auxiliary material for each material y such as straw, with zy the amount of material needed to process one ton of residue tb and py the price for each unit of y;
o
as the variable cost of processing for each processing input e such as electricity, with ve the amount of that input needed to process one ton residues and pe the price for each unit of e. If applicable (e.g. electricity or heat), a distinction can be made between consumed input vec and produced input vep so that
;o
as post-harvest residue collection costs, if applicable;
o
as transport costs for biomass including cost per ton/km h and transported distance d.
Operation-linked expenses
for each yearly overhead cost such as maintenance and personnel.
As a summation of these cost parameters, the total cost (TC) of bio-based fertilizer production can be specified as follows:
(6)
(6)
The average cost per ton of bio-based fertilizer then equals:
(7)
(7)
The objective function (1) can be re-written as follows:
(8)
(8) The investment cost per ton of residue is a function of the maximum capacity of the plant. In other words, the higher the maximum amount of residue collected, the more the investor can pursue economies of scale by purchasing a larger unit at lower investment costs per ton of residue treated. The maximum amount of residue collected T is the sum of the available on-farm residues. Investment cost I can thus depend on nT with n the number of residue suppliers, assuming all participants are equal in terms of residue collection capacity.
2.1.1. Business model differentiation
Based on the sustainable circular business model typology by De Keyser and Mathijs (Citation2023) with applications to anaerobic digestion, adapted from the sustainable business model archetypes of Bocken et al. (Citation2014), we define three business models. We relate the social level of innovation to the ownership of the bio-based fertilizer technology: the owner can either be the end-user (i.e. a sufficiency BM), a group of end-users (i.e. a stewardship BM) or a third party such as a technology provider, waste processor or fertilizer producer (i.e. a functionality BM). From a farmer’s perspective, as the end-user, fertilizer application costs will differ depending on the business model of the technology owner.
In a stewardship BM, the owner of the technology is a group of end-users such as a farmers’ cooperative. From an individual farmer’s perspective, the share of bio-based fertilizer in relation to the total production will depend on the amount of residues they collect. This is very much in line with the concept of collective biogas investment presented by Zemo and Termansen (Citation2018), who investigate farmers’ willingness to participate in a collective biogas investment scheme where costs, benefits and responsibilities are shared proportionally between partners. Zemo and Termansen (Citation2018) conclude, based on a discrete choice experiment study of Danish farmers, that the optimal number of farmers in a partnership-based biogas plant is 14. The authors argue that farmers are willing to co-invest in a biogas plant for economic reasons, but behavioural motivations prevent them from forming partnerships with over 14 farmers (Zemo & Termansen, Citation2018). Therefore, we assume 14 co-investors in case of a stewardship BM. The residues will need to be transported to the conversion unit. The investment cost per ton of residues then relies on the sum of the maximum amount collected for all participants: I is based on nT, with n the number of co-investors. For a stewardship BM, the average costs are specified as in equation (7), and the objective function equals equation (8).
In a sufficiency BM, the owner of the technology is the end-user himself, so the distance to the residue-conversion unit can be assumed to be zero. For a sufficiency BM, the average bio-based fertilizer production cost becomes:
(9)
(9)
The objective function then becomes:
(10)
(10)
Lastly, in a functionality BM, the end-user has no ownership at all. In that case, they pay a fee s to a third party in exchange for residue conversion services. This third party can for example be a technology provider or an existing fertilizer producer. Following the full-cost pricing principle of Hall and Hitch (Citation1939), the third-party service provider charges a price s equal to the sum of the average total cost and a profit margin. The farmer will not need to invest in a residue conversion unit and will not have to pay raw material, processing or overhead costs for residue conversion. The service provider will operate one large conversion unit to provide services to many suppliers (n = 100). The average cost function is as follows:
(11)
(11)
The objective function then becomes:
(12)
(12)
These circular business models are only relevant in case of bio-based fertilizer prosumption with anaerobic digestion. In other words, the differentiation between sufficiency, stewardship and functionality business models only applies to the case of digestate prosumption.
2.1.2. Sensitivity analysis with different scenarios
The baseline LP-analysis is based on realistic costs and pricing parameters in 2020. In addition to a baseline estimation, eight different scenarios were modelled: an obligation to collect crop residues, increased electricity and fertilizer prices, regional hubs, investment support, increased digestate N and EOC content, alternative residue fertilizer value, farmyard manure availability and increased manure value.
In the first scenario, we assume that farmers are obligated to collect crop residues. As residue collection is a strategy to control nitrogen and phosphorus leaching, this obligation could be beneficial to reduce nutrient pollution. A second scenario includes increased electricity and fertilizer prices since this can boost the competitiveness of bio-based fertilizers. However, for this simulation, a rise in Chemical Engineering Plant Cost Index (CEPCI) and Consumer Price Index (CPI) is also considered, in accordance with price rises up until May 2022. In a third sensitivity analysis, we simulate the effects of regional hubs by assuming a lower distance between farms and anaerobic digestion units. Fourthly, we run the model with the incorporation of investment support to individual farmers. In a fifth scenario, we simulate the changes in the results with increased concentrations of nitrogen and effective organic carbon in compost and digestate. In a sixth scenario, in contrast to the baseline scenario, we assume that the farmer does consider the nutrients in crop residues when calculating maximum fertilizer rates to comply with regional regulations. In the seventh scenario, we assume that solid farmyard manure can also be applied as a fertilizer. Finally, a last scenario includes an increased manure value: while it is currently not the case given the manure surplus in Flanders, this scenario assumes that the leek farmer will pay the transport costs of pig slurry to the pig farmer.
2.1.3. Model limitations
The model presented here incorporates the most easily quantifiable cost factors of residue- and manure-based fertilizer production and application. Like similar models, some potentially important issues are ignored. First of all, the nutrient content of bio-based fertilizer depends on the exact inputs of the conversion process. As a consequence, this is not easily quantifiable. Therefore, it is necessary to define a range for the nitrogen plant availability coefficient and conduct a sensitivity analysis (scenario 5) to determine how the results change based on changes in nutrient content. Secondly, we assume equal collection by each farmer. However, in reality, this may differ according to the size and specificities of each farm. Farm heterogeneity is not considered. Assuming homogeneous farms allows for ease of calculation. Finally, we assume mono-cultivation and equal distribution of fertilizer across the land. For farmers growing multiple crops, the objective function and constraints can be adapted to include an integration across crops. Similarly, additional nutrient constraints can be formulated if other nutrients such as P and K are essential as well.
summarizes the methodology used in this paper. The next section describes the data used for the analysis. This includes farm specific data such as residue availability, nutrient needs and purchased fertilizer data, as well as prosumed bio-based fertilizer costs.
2.2. Data
2.2.1. Farm specific data
In this section, a leek farm is taken as an example. Together with Indonesia and Turkey, Belgium is among the largest leek producers worldwide (Faostat, Citation2022). In Flanders, Belgium’s northern region, leek is grown on 30% of the horticultural area, making it one of the most important vegetables in the region (Platteau et al., Citation2018). In the region of West-Flanders, with concentrated leek cultivation () and in accordance with D’Haene and Hofman (Citation2019), leek is mainly planted in loam and sandy loam fields. Belgium has a temperate marine climate with mild winters and summers, with precipitation throughout the year and with a slow increase over the years (World Bank Climate Change Knowledge Portal, Citation2022). Following the FADN-database, the average size of a Flemish specialist horticultural farm was 13 ha in 2020. The average leek yield on Flemish farms is between 34 and 55 ton/ha (Deuninck & Vervloet, Citation2016; D’Haene & Hofman, Citation2019), while leek residue yield in Flanders is about 25 ton fresh matter per hectare (De Dobbelaere et al., Citation2015b). We assume that all residues are collected after cleaning the leek harvest for the fresh market. An overview of the farm specific data used in the LP model is given in Appendix A ().
Figure 3. Leek plots in Flanders in 2021 (Departement Landbouw en Visserij, Citation2022).

For leek cultivation, nitrogen addition is very important, while phosphorus and potassium are not critical. Farmers often fertilize leek plots with mineral fertilizer and pig slurry (Brouckaert, Citation2022). Experimental research by D’Haene and Hofman (Citation2019) is used as the basis of this model regarding nitrogen requirements. In contrast to the perception of some farmers, low residual soil mineral nitrogen values can be achieved without yield reduction (D’Haene & Hofman, Citation2019). D’Haene and Hofman (Citation2019) find that the maximum leek yield is obtained at 150–200 kg/ha N, but since the mean minimal N-value in Flanders is 70 kg/ha N, application rates are in the order of 80–130 kg/ha N. This mean minimal N-value considers the mean nitrogen mineralization from soil organic matter and incorporation of cover crops or crop residues from earlier crops. Therefore, the minimal N values are higher during the summer months (D’Haene & Hofman, Citation2019). Constrained by Flemish regulations, nitrogen fertilization by animal manure or slurry cannot exceed 170 kg/ha Ntot, while standards for total plant available nitrogen range from 180–250 kg/ha N (VLM, Citation2022a). Phosphorus fertilization is also restricted by law: agricultural plots are divided into four classes. The fertilization standard differs for each crop and phosphorus fertilization class (VLM, Citation2022a). Assuming a moderately high phosphorus availability for horticultural soils (class III), we follow a fertilization standard of 55 kg/ha P2O5 or 24 kg/ha P (VLM, Citation2022a). However, if certified compost is used, only 50% of P2O5 is taken into account (VLM, Citation2022a). Most soils in Flanders are sandy loam soils. For these soils, the minimum yearly addition of effective organic carbon is 850 kg/ha EOC (VITO, Citation2015).
2.2.2. Prosumed bio-based fertilizer costs
In addition to buying fertilizer on the market or using pig slurry, a leek farmer can also choose to produce fertilizers for their own consumption. Based on leek residues, three prosumed fertilizers are distinguished: post-harvest crop residues, on-farm compost and digestate (). For these fertilizers, three types of costs are considered: installation costs, consumption-linked expenses and operation-linked expenses.
2.2.2.1. Installation costs
Composting requires a small investment in infrastructure. We assume facility construction costs for a small-scale on-farm composting plant for crop and pruning residues following Pergola et al. (Citation2018), adjusted to 2020 with the CEPCI.
Running an anaerobic digestion plant will require an investment as well. We refer to the pocket digester as described by De Dobbelaere et al. (Citation2015a), converting chicory residues into biogas. This digester required an initial investment cost of €900 000 (De Dobbelaere et al., Citation2015a). Every year, 4 190 ton of organic residues are digested. We further assume a lifetime of 15 years. Using the composite CEPCI to update these capital costs to 2020, we find an investment cost of €963 685.
However, the investment costs relate to the scale of the biogas digester. Since the available amount of residues differs for each business model, the scale of the optimal digester will also differ. To be able to make an unbiased comparison between all business models, we assume that ‘tailor made’ anaerobic digesters can be built, matching the amount of residues available. As a consequence, capacity utilization across all scales of digesters is maximized and equal. We use the ‘0.6 rule’ to describe the relationship between equipment costs and capacity increases. We can estimate the investment cost based on the pocket digester described by De Dobbelaere et al. (Citation2015a) as follows:
(13)
(13)
(14)
(14)
2.2.2.2. Consumption-linked expenses
Considering consumption-linked expenses, three types of costs are considered: raw material, processing costs and collection costs. For crop residues, none of these costs are relevant: the farmer only saves collection costs. In accordance with the reference for fresh market leek residues as described by Agneessens et al. (Citation2014), we assume that all residues are collected during the sorting and washing stage. They are redistributed on the fields on a later date (De Dobbelaere et al., Citation2015b).
Considering on-farm composting, leek residues are co-composted with more carbon-rich materials such as straw or pruning residues. To calculate raw material costs, we assume that the leek farmer can purchase bulking agents such as maize straw and wood chips from neighbouring farmers for €80/ton including transport. In reality, this cost will differ depending on the farmer’s and/or neighbour’s resources. Processing costs are based on the findings of Pergola et al. (Citation2018), adjusted for the CPI.
Finally, considering anaerobic digestion, both processing and collection costs are relevant. An overview is given in Appendix A (). The pocket digester case study by De Dobbelaere et al. (Citation2015a) indicates an annual net electricity production of 500 MWh, or 119 kW per ton of residues assuming a capacity of 4 190 ton, of which 29% is consumed by the digester itself. An electricity cost of €0.21/kWh is assumed (VREG, Citation2022). Transport costs are considered for a distance of 20 km. The transport cost for a tractor was €0.127/km per ton in 2018 (Van der Meulen et al., Citation2020). This includes fixed costs such as depreciation and insurance, variable costs such as fuel, staff costs and general operating costs. However, this number relates to the average cost per ton/km in terms of total tonnage. To estimate the average costs per ton/km in terms of effective capacity, we assume an average tare weight of 21 ton for a tractor-trailer combination with an empty load and an effective capacity of 29 ton (Van der Meulen et al., Citation2020). Within a range of 290 ton (i.e. 10 fully loaded trucks), the average cost is €0.26/km per ton of residues. Adjusted for the CPI, this is €0.27/km per ton of residues. The latter is multiplied by a factor 1.9 (see conversion rate in Appendix A, ) to include the weight reduction of ton residues to digestate: a full load for the outward journey with residues, and a load of 90% for the return journey with the digestate.
2.2.2.3. Operation-linked expenses
Finally, operation-linked expenses are considered. We assume that operational personnel expenses such as maintenance are only relevant in case of the operation of an anaerobic digestion unit. De Dobbelaere et al. (Citation2015a) find variable costs of €30 000 per year including administrative and maintenance costs for an installation with an annual net electricity production of 500 MWh. For a capacity of 4 190 ton/year and corrected with the CEPCI, this is €8/ton of organic residues.
2.2.3. Fertilizer characteristics
Two suitable fertilizers that are available on the Flemish market are urea and compost. In 2020, urea prices averaged €201/ton (IndexMundi, Citation2021). Urea is a form of industrially manufactured organic nitrogen. Most of the plant available nitrogen forms as a result of a reaction with water. When the urea application is planned before tillage or water application, nitrogen losses are prevented. The nitrogen content in urea (CH₄N₂O) makes up 46% of the total mass, i.e. 0.5 kg N per kg urea.
VLACO-certified compost can be purchased all over Flanders. For example, waste management organization Ecowerf sold VLACO-certified compost at €151 per batch of 10 ton in 2020, transport included (Ecowerf, Citation2021a). On average, VLACO fruit and vegetable waste compost contains 12 kg/ton N (VLACO, Citation2019). The plant available nitrogen or PAN-percentage is 15% and the effective organic carbon content of this type of compost is 123 kg/ton EOC (Vanrespaille et al., Citation2018; VLACO, Citation2019).
Animal slurries and manures can be a valuable source of nutrients for crop growth. Their nutrient content depends on many factors, including animal species: there is a significant variability in manure or slurry nutrient characteristics for livestock species such as pigs, poultry, beef and dairy livestock. Given the high representation of pig breeding in Flanders (Statistiek Vlaanderen, Citation2019), this paper focuses on pig slurry. Since pig slurry is retrieved from a neighbouring pig farm, no investment is needed for the vegetable farmer. Considering the manure surplus in Flanders, we assume that the pig farmer takes on the transport costs. Following Vanrespaille et al. (Citation2018), pig slurry contains 12 kg/ton EOC, 3.5 kg/ton P2O5 and 6.4 kg/ton total N with a PAN-coefficient of 60%. Schiavon et al. (Citation2009) estimate that every pig produces a minimum of 1.5 ton slurry per year. Cattle slurry contains 4.8 kg/ton N, 1.4 kg/ton P2O5 and 15 kg/ton EOC. However, solid manure can also be used as a fertilizer. Farmyard manure has a PAN-coefficient of 30% (Vanrespaille et al., Citation2018). Pig manure contains 7.5 kg/ton N, 9.0 kg/ton P2O5 and 57 kg/ton EOC, while this is respectively 7.1 kg/ton N, 2.9 kg/ton P2O2 and 46 kg/ton EOC for cattle manure (Vanrespaille et al., Citation2018).
Leaving crop residues on the field is disregarded as a nitrogen fertilizing strategy since fresh crop residues are only available in restricted periods during the year and decay fast. This decay does not allow for storage and usage of the fertilizer at a later time (Viaene et al., Citation2017). Despite a small proportion of the nitrogen in crop residues being available for follow-up crops such as spring barley (De Haan et al., Citation2019), the nitrogen available for leek fertilization is assumed to be zero (). Nevertheless, nitrogen residuals of crop residues are considered by subtracting the mean minimal N-value of 70 kg/ha from the nitrogen application recommendation (D’Haene & Hofman, Citation2019). Considering the P-standard of 55 kg/ha P2O5 or 24 kg/ha P (VLM, Citation2022a), crop residues are not considered as a source of phosphorous, despite a total phosphorous content of 0.4 g/kg fresh matter as found by Viaene et al. (Citation2017). Finally, the decay of crop residues contributes to the soil EOC as this refers to the organic carbon that remains in the soil for at least a year. Viaene et al. (Citation2017) find that 7.2% of the fresh weight of leek residues is organic matter. Following Pribyl (Citation2010), 50% of this is organic carbon. Multiplying this with a humification coefficient of 23% (Sleutel, Citation2005), we find an EOC of 8.2 kg/ton.
Since leek residues can be considered a burden, bringing along significant nitrogen losses in the soil and complaints of odour when spread onto the field, a farmer can choose to convert the residues. After composting leek residues with 57% carbon rich bulking agents for 19 weeks, Viaene et al. (Citation2017) find a nitrogen content of 7.9 g N per kg dry matter, corresponding to 3.2 g N per kg fresh matter with a dry matter content of 40%. Similarly, the authors find a phosphorus content of 0.6 g P per kg fresh matter. Additionally, composted leek shows an organic matter content of 14.3% of the fresh weight (Viaene et al., Citation2017). Assuming that 50% of organic matter is organic carbon (Pribyl, Citation2010) and the humification coefficient for bio-ton residues compost in Flanders is 90% (Veeken et al., Citation2017), we estimate the EOC-content to be 64 g/kg EOC. A PAN-coefficient of 15% is assumed (Vanrespaille et al., Citation2018). Following VLACO (Citation2009b), we assume a conversion factor of 0.4: one ton organic residues converts into 0.4 ton compost. Since leek residues are co-composted with 57% bulking agents, the conversion factor for leek residues to compost is 0.9.
De Dobbelaere et al. (Citation2022) researched the technological potential of mono- digestion of leek residues to investigate if anaerobic digestion of leek residues as a mono-stream could occur in a stable way. Experiments resulted in a nitrogen content of 2.5 g N per kg De Dobbelaere et al. (Citation2022). The phosphorus content was not measured, but we assume 0.4 g P per kg fresh matter in accordance with a leek residue N/P ratio of 7 (Viaene et al., Citation2017). Based on Thomsen et al. (Citation2013), we assume that long-term soil sequestration is similar for digestate application compared to returning crop residues to the field, resulting in an equal EOC adjusted for the volume reduction. In accordance with Vanrespaille et al. (Citation2018), we assume a PAN-coefficient of 60%. Following VLACO (Citation2009a), we assume a conversion of 1 ton of residues to 900 kg of digestate or a conversion factor of 0.9. This does not involve a separation of solid and liquid fractions: in Flanders, most digestate is not separated or treated before application as a fertilizer (VLACO, Citation2009a).
3. Results
In this section, the results of the linear programming optimization will be discussed. First, for each scenario, the digestate costs is calculated following anaerobic digestion business models explained in section 2.1.1. Differences in business models and scenarios affect the average cost of bio-based fertilizer production. shows the average costs of digestate production. It appears that a sufficiency BM provides the highest average costs. This indicates that the savings in transport costs are lower than the increase in investment costs compared to a stewardship and functionality BM.
Table 1. Average costs of digestate in different business models and scenario’s (€/ton).
3.1. Baseline scenario
In the baseline scenario, the stewardship BM provides average digestate production costs of €16 for an individual farmer (). In this business model, it is assumed that 14 farmers co-invest in an anaerobic digestion plant and distribute the benefits - including energy production benefits - equally. To an individual farmer, this provides lower investment costs compared to a sufficiency BM where the farmer is the sole investor. For a sufficiency BM in which the farmer carries the investment costs alone, average costs are €25/ton digestate. Finally, for a functionality BM, the average costs are based on a fee that is paid to a service provider as well as some additional costs made by the farmer. A customer base of 100 homogenous farms is assumed. We further assume that the owner makes a profit by producing electricity and using it, for example, in a biorefinery structure. As such, the owner collects the residues and provides the fertilizer back to the farmer for a fee that includes a profit margin reduced by the amount of electricity cost savings. For a profit margin of 20%, the total costs to the farmer are lower than in a stewardship model. With an electricity cost of €0.21/kWh, the average costs amount to €35/ton digestate and the profit from electricity generation amounts to €28/ton. As such, the fee charged to the farmer is €15/ton.
It is notable that costs of on-farm composting are higher than costs of anaerobic digestion. For composting, raw material such as maize straw represents a large part of the average cost. If all carbon-rich bulking agents would be available for free, composting would be cheaper to the farmer than anaerobic digestion. Anaerobic digestion represents additional cost savings since the CHP-unit converts produced biogas to electricity. We assume that all produced energy is self-consumed. However, the user will be limited by the amount of residues available for conversion. Additionally, digestate may contribute to the nutrient needs in a lesser extent. For example, since no carbon-rich maize straw is added to the mono-digestion process, digestate offers a lower EOC-content. These considerations are important in deciding which fertilizers to purchase or produce.
The LP-model is run for a functionality BM, given that this BM provides the lowest digestate costs. The results obtained are presented in . The final values indicate the optimal application rates of each fertilizer. The reduced cost indicates the amount the objective coefficients (i.e. the average prices or costs) would have to improve before the optimal solution changes such that the corresponding variable takes on a positive final value. The allowable in- and decrease indicate the amounts by which the price or cost can in- or decrease without changing the optimal application rates.
Table 2. Baseline cost minimization sensitivity results – functionality.
It appears that, per hectare, it is optimal to purchase 60 kg urea and 4 ton certified compost, use 12 ton pig slurry and leave all crop residues on the field. This totals to a yearly cost of €72/ha. Considering plant-based bio-based fertilizers, it is optimal to leave crop residues on the field: the allowable cost increase for crop residues is €13. It can be calculated that digestate production will need to provide cost savings in order to be applied in the optimal solution: the reduced cost of the objective coefficient is €15. As a consequence, the distinction between sufficiency, stewardship and functionality BMs is not relevant. Similarly, the reduced cost for on-farm compost is €119.
The shadow prices indicate to what extent the total cost per hectare can be in- or decreased if the constraint changes with one unit. It is apparent that the shadow price of available crop residues is negative: as one more ton/ha of crop residues becomes available, total costs decrease with €1.2. This can be attributed to the increase in soil organic carbon. The same holds for the shadow price of phosphorous: as one more kg/ha P is allowed, certified compost will be replaced by pig slurry, which lowers the costs. For the lower bounds on N and EOC, the shadow prices are positive: as one more kg/ha of N and EOC is needed, the costs will increase with respectively €0.4 and €0.1. The upper bound nitrogen constraint as well as the animal nitrogen constraint are not binding.
3.2. Scenario 1: obligation to collect crop residues
In this scenario, we assume that the farmer is obliged to collect his crop residues and to have them collected by an intermunicipal waste management company. As such, it is assumed that the collection of crop residues brings along organic waste collection costs of €180/ton (Ecowerf, Citation2021b).
In this case, it is profitable to prosume digestate in all business models. In a functionality BM, the intermunicipal waste management company can collect the residues and carry out the anaerobic digestion process for a fee. They will then shift their business model from one in which profits are made from gateway fees to one in which electricity cost savings determine the profits. Finally, it is notable that the reduced cost of compost is €104. For example, in case the farmer can get all carbon-rich bulking residues for free, composting would be more profitable than anaerobic digestion.
3.3. Scenario 2: increased electricity and mineral fertilizer prices
A second scenario involves a better competitive position of bio-based fertilizers compared to mineral fertilizers due to an electricity as well as mineral fertilizer price rise. Electricity cost savings make sure that digestate costs are reduced significantly. However, when electricity prices rise, the CEPCI, transport costs and mineral fertilizer prices will also rise. We take on the example of the prices in May 2022. This includes a mineral fertilizer price of €669 and an electricity price of €0.42/kWh. Additionally, it also includes an increase in the CPI and CEPCI.Footnote1 For a sufficiency model, average digestate costs increase to €38, while they decrease to €8 and €5 respectively for stewardship and functionality business models. As such, the fertilizer application rates do not change. However, total costs increase up to €105/ha.
3.4. Scenario 3: regional hubs
A third scenario involves the consideration of regional hubs. Closing regional loops is often emphasized as a key success factor of the circular bioeconomy. McCormick and Kautto (Citation2013) argues that biorefineries, designed as decentralized solutions based on local conditions, are vital for the development of the bioeconomy. Farmers could diversify their businesses and ‘short-cut’ global supply chains by locally exchanging alternative inputs and outputs (Therond et al., Citation2017). For the estimations of scenario 3, a transport distance of 5 km is assumed for digestate in stewardship and functionality BMs. Despite digestate costs lowering to respectively €8 and €4, the cost is still not low enough for digestate to be an interesting fertilizer. However, it has to be noted that a combination of scenario 2 and 3, i.e. regional hubs with increased electricity and fertilizer prices, changes the results. In that case, a functionality business model for digestate is profitable, while a stewardship business model is not. The optimal application rates are then equal to the application rates in S1: digestate application is maximized and complemented with certified compost, pig slurry and urea.
3.5. Scenario 4: investment support
In Flanders, agricultural and industrial companies can qualify for different measures of investment support. For small-scale anaerobic digestion plants, small businesses can receive VEA investment support (Vlaams Energie- en Klimaatagentschap, Citation2022). Additionally, agricultural businesses also qualify for 30–40% VLIF investment support for some components of the pocket digester (Departement Landbouw & Visserij, Citation2022a). The pocket digester itself is not eligible for subsidies, but farmers with a unique idea can apply for VLIF-innovation support of 40% on the investment costs (Departement Landbouw & Visserij, Citation2022b). Industrial companies or large-scale agricultural businesses do not qualify for either VEA or VLIF support. As such, we only calculate the investment support for a sufficiency BM.
A farmer with 13 ha of leek, producing 25 ton residues per hectare, can purchase a ‘tailor-made’ anaerobic digestion unit with a lifetime of 15 years for a total investment cost of €207 844 following equation (30). Assuming a 40% investment support on the total investment costs (i.e. VLIF investment support as well as innovation support), this comes down to €124 706. Total costs per ton of digestate produced are then €17. Even if the investment support would be provided to large-scale agricultural businesses or industrial companies, the stewardship and functionality BMs are not profitable to an individual farmer.
3.6. Scenario 5: increased N and EOC content
The exact nutrient content of the biogas digestate cannot be determined. The nitrogen content, proportion of plant available nitrogen and the effective organic carbon content will differ according to the process inputs. Considering a range of plant available nitrogen between 50 and 70%, the digestate application rates do not change. This indicates that a small deviation in PAN-levels does not cause much difference in the overall results. Even with significant increases in the nitrogen or EOC-content, the results do not change.
3.7. Scenario 6: alternative residue fertilizer value
In the baseline scenario, the farmer does not take the crop residues into account when considering the phosphorus standards. In this scenario, we assume that while the crop residues are not useful to reach the minimum amount of fertilizer needed for leek cultivation, they are taken into account when considering the maximum nitrogen and phosphorous standards.
In this case, the farmer will still use all crop residues and some certified compost, but less pig slurry and more urea. In contrast to the upper nitrogen limit, the phosphorous limit was binding in the baseline scenario. Therefore, an increase of phosphorous fertilization by applying crop residues will change the application rates of other fertilizers. As such, pig slurry is very constraining in terms of the phosphorous content. The shadow price of additional residues remains negative, indicating that the EOC content remains useful despite the additions to the nutrient standards. Total costs of fertilization are €92/ha.
3.8. Scenario 7: farmyard manure availability
In the baseline scenario, we assumed the availability of pig slurry as an animal fertilizer given the high representation of pig breeding in Flanders (Statistiek Vlaanderen, Citation2019). In the seventh scenario, we assume that solid farmyard manure can also be applied as a fertilizer. We calculate this scenario based on the availability of pig slurry, pig manure, cattle slurry and cattle manure. The average costs of all animal-based fertilizers are zero given the manure surplus in Flanders. Pig or cattle farmers will thus take all disposal costs on them. Consistent with Flemish regulation, phosphorus originating from farmyard manure and compost only counts for 50% of its phosphorus content (VLM, Citation2022a).
The option of pig manure, cattle slurry and cattle manure does indeed affect the fertilizer decision: for each hectare of leek cultivation, roughly 5 ton of pig manure, 24 ton of cattle slurry and 0.5 ton of cattle manure should now be applied every year. This causes fertilizer costs to decrease to zero. While the phosphorus constraint is met, the total animal nitrogen fertilization amounts to 152 ton/ha, which is still lower than the maximal animal nitrogen standard of 170 ton/ha. As such, the animal nitrogen constraint is still not binding.
3.9. Scenario 8: increased manure value
Currently, a manure surplus in Flanders causes the value of manure to be very low. However, as manure gets more and more valuable, this equilibrium may shift. As such, the vegetable farmers looking for manure as a fertilizer, may take the transport costs on them. We assume a distance of 20 kilometer between these farms. To include the empty loaded drive back, we multiply the transport costs by a factor 1.6.Footnote2
In this scenario, it is too expensive to use pig slurry as a fertilizer. All fertilizer requirements are met by combining urea with certified compost and crop residues. Nevertheless, this changes if the pig slurry costs lower by €6/ton. This is the case if the distance between both farms decreases to less than 5.9 km.
4. Discussion
Prior studies have noted the importance of profit maximization and cost minimization related to fertilizer usage (Bueno-Delgado et al., Citation2016; Clare et al., Citation2014; Keplinger & Hauck, Citation2006; Rodias et al., Citation2019). However, optimization studies for bio-based fertilizer prosumption are scarce. The present study was designed to identify an LP model and to prove its applicability for leek production in Flanders. Another objective was to assess the effects of different business model configurations and future scenarios on the fertilizer trade-off.
Despite low costs, it is not profitable for a farmer to prosume digestate in any of the business models in most of the scenarios. Only in case of an obligation to collect crop residues (S1), digestate becomes an attractive option to convert leek residues. In that case, all business models are profitable. From a farmer’s perspective, the functionality BM is most profitable. In this BM, a service provider collects the leek residues and provides the farmer with the digestate as a fertilizer for a small fee. The service provider profits from the electricity production related to the biogas production.
However, in all other scenarios, it appears that digestate nor on-farm compost are attractive enough compared to leaving crop residues on the field. In the baseline scenario, it is optimal to complement the on-field residues with pig slurry and mineral fertilizer in order to fulfil the nitrogen needs and with certified compost to satisfy the organic carbon requirements. While the application of mineral fertilizer, pig slurry and crop residues corresponds to reality (Brouckaert, Citation2022; De Dobbelaere et al., Citation2015b), the application of certified compost does not. Nevertheless, the relative attractiveness of different fertilizers does change with changes in manure availability: as more farmyard manure is available, it is possible to fulfil all nutrient needs with animal manure. In contrast, with increased manure value and thus cost, it is optimal to increase the use of mineral fertilizer and compost instead of pig slurry.
Considering the application rates in all scenarios, represents the PAN- and EOC-contributions of each fertilizer. It is clear that urea is interesting in terms of PAN, while it does not contribute to EOC. Animal manure and slurry provide nitrogen while at the same time raising carbon stocks. As such, as is the case in other regions as well (Ochieng et al., Citation2022), there is evidence for trade-offs between mineral fertilizers and animal manures. Compost and crop residues are mainly interesting because of their contribution to EOC. This seems to be a critical factor in the competitiveness of digestate, since digestate competes with on-farm compost and crop residues in terms of residue utilization. Returning crop residues to the soil represents a cheap and efficient way of providing all residue organic matter to the soil.
Figure 4. PAN- and EOC-contributions of fertilizers as well as regional and on-farm circularity in different scenarios (colour) .

Since all bio-based fertilizers are produced in the region, all scenarios allow regional EOC-circularity to reach 100%, of which on-farm or prosumed EOC-circularity can contribute up to a 24%. Considering PAN-circularity, however, full regional circularity is only achieved in case of increased availability of farmyard manure. Moreover, with an alternative value of crop residues or an increased manure value, regional circularity decreases to 38% and 12% respectively compared to a 68% circularity rate in the baseline scenario. Nevertheless, in case of an obligation to collect crop residues, all crop residues will be anaerobically digested and regional circularity reaches 83% of which on-farm circularity contributes 42%.
These findings suggest that, with current policies and prices, it is not financially viable for a leek farmer to produce bio-based compost or digestate based on on-farm residues. However, leaving crop residues on the field is a viable option, despite low fertilizing contributions because of nitrogen leaching. As a consequence, application of mineral fertilizer, pig slurry or certified compost is essential to fulfil nitrogen and organic carbon needs. The main limitations towards full circularity do not only relate to the availability of residues, but also to the relatively high costs of composting and anaerobic digestion compared to leaving crop residues on the field. Anaerobic digestion is only profitable if policies would prohibit the application of crop residues post-harvest. A note of caution is due here relating to externality pricing. If environmental externalities such as global warming and nitrogen pollution are priced correctly, some fertilizers will be relatively less attractive. This can for example include incentives towards carbon farming.
Finally, the study points out interesting opportunities of regional value chain cooperation. For example, Flemish intermunicipal companies can play a role in regional bio-based fertilizer prosumption. Not only are they currently selling VLACO certified compost (Ecowerf, Citation2021a), but they can also play a role as a service provider in a functionality BM. In that case, their business model would shift to one in which profits are made from electricity production instead of gate fees. However, this is only profitable for the farmer in case of an obligation to collect crop residues. Other regional value chain actors such as cattle and pig farmers can be involved in a bio-based fertilizer exchange network while simultaneously reducing the Flemish manure surplus.
The model in this study is a cost minimization model with a fixed nitrogen requirement to ensure current yield quality and quantity. An extended model with variable yield outcome can also be constructed based on a nitrogen-yield response function or production function. However, constructing a linear nitrogen-yield response function would be an oversimplification of reality, since yields depend on many other factors and high N-supply can lead to poor product quality and diseases, affecting taste and profits (D’Haene & Hofman, Citation2019). Nevertheless, one can reason that the farmer will apply more fertilizer as long as the marginal profit of nitrogen supply by the fertilizer exceeds the cost. Given the specifications in this study, this will result in an increase in mineral fertilizer use until the maximum nitrogen constraint imposed by governmental standards is met (i.e. a maximum of 270 kg/ha). The application rates of other fertilizers will not change given the phosphorus and residue availability constraints.
The ability to extrapolate these results is subject to certain limitations. Firstly, some factors were not addressed in the study. For instance, in a functionality BM, costs could be even lower than assumed in this study because of expertise, knowledge or existing resources. Moreover, disposal costs of the biogas plants at the end of their lifetime were not considered in either of the business models. Additionally, time preferences are not considered. The investment cost was calculated per ton of residues converted over a lifetime of 15 years. However, farmers’ attitude towards future events may result in particularly high discount rates (Duquette et al., Citation2012). Secondly, the assumption of ‘tailor-made’ anaerobic digesters is currently not a realistic assumption. However, it did allow for unbiased comparison across business models. Even if this assumption involves an underestimation of the investment costs, the results remain: on-farm leek digestion is not profitable. Thirdly, while this study may be representative for a leek farmer in Flanders, the results may not be applicable to other regions. Finally, it is important to bear in mind that this study focuses on leek cultivation. Given the specific nutrient needs and crop residue content, it is hard to generalize the findings to the cultivation of other crops. Crop rotation with other crops was not considered. This could, however, impact the findings. To this purpose, gathering additional data and modelling of crop rotations will provide an added value. Crop models such as NDICEA (van der Burgt & Timmermans, Citation2009; Van den Burgt & Hanegraaf, Citation2021), APSIM (Holzworth et al., Citation2014) or EU-Rotate_N (Rahn et al., Citation2010) account for the interaction between soil and climate to model nitrogen dynamics in cropping systems. These models allow simulation of different fertilization and crop management strategies on crop rotations for vegetable and arable crops.
5. Conclusion
This study develops a LP model intended to minimize fertilization costs for an individual farmer by considering the possibility of bio-based fertilizer production from organic residues. The second aim of the study was to investigate the effects of different business model configurations as well as the effects of plausible future scenarios on the average and total costs.
The study has shown that, given the baseline parameters, it is unlikely for a Flemish leek farmer to fulfil his nutrient needs solely with bio-based fertilizer. In addition to leaving crop residues on the field, purchasing certified compost and using pig slurry from a neighbouring pig farmer, an individual leek farmer should also purchase mineral fertilizer: a mix of fertilizers is the optimal solution in the cost-minimizing approach. Additionally, given the trade-off between investment cost and transport cost based on the data used in this paper, a sufficiency BM involving no shared or third-party investment, provided higher average costs for anaerobic digestion. Nevertheless, the stewardship and functionality BMs did not provide costs low enough for anaerobic digestion to be interesting. A second major finding is thus that innovative business models involving partnerships or cooperation with others do not necessarily ensure that bio-based fertilizer prosumption becomes an attractive option.
Sensitivity analyses point out that digestate prosumption is only profitable if cost residues are not allowed to be left on the field. Secondly, higher electricity and mineral fertilizer prices do not affect the fertilizer application rates when they are countered by an increase in CEPCI. Additionally, the existence of regional hubs does not influence the application of bio-based fertilizer: costs are still too high, even if the conversion plant is located close by. With investment support up to 40% for small installations, sufficiency business models provide lower costs compared to stewardship or functionality BMs, but not low enough for digestate to be interesting. Increased digestate N and EOC content do not affect the baseline results. However, application rates change slightly when the P- and N-content of crop residues are taken into account considering the fertilization standards. Additionally, if pig and cattle manure and slurry are available for free, a leek farmer can satisfy all nutrient needs with animal-based fertilizers while leaving his residues on the fields. However, when manure becomes more valuable and the leek farmer will have to pay for the transport costs, this may not be the case anymore. In that case, it depends on the distance between farms.
Overall, this study challenges the idea that bio-based fertilizers are sufficient to bridge the gap between nutrient imports and nutrient losses in Europe from a farmer’s perspective. However, it points out the importance of value chain cooperation between vegetable farmers and animal farmers, as well as the importance of regionally available certified compost. Additionally, the findings have implications for understanding the trade-off between several fertilizer characteristics such as cost, nutrient content and input availability.
While caution is needed in the extrapolation of the results, this study offers insights that can be applied to farming systems with similar crop cultivations and costs. Further studies can apply the model to other crops, incorporating specific regional conditions and time preferences. To arrive at a good understanding of a farmer’s cost minimization problem, additional studies will be needed that incorporate fertilization needs of different crops while distinguishing crop rotated plots and considering the crop residues and bio-based fertilizer mix composition. Future technologies to produce alternative fertilizer blends such as biochar or insect frass production could be considered and incorporated in the model. The model developed in this study can be used as a baseline to compare the competitiveness of each fertilizer compound and feasibility of business models. Future studies on these topics are therefore recommended.
Disclosure statement
The authors declare that they have no known competing financial or non-financial interests that could have appeared to influence the work reported in this paper.
Additional information
Funding
Notes
1 Latest available CEPCI-data from May 2022.
2 Based on the phosphorus constraint, the amount of pig slurry transported will not exceed 16 ton. To transport 16 ton of pig slurry over a distance of 20 km, total costs are estimated to be €96, while costs of the empty ride back are €55. This results in a ratio of 1.6. The higher the amount of pig slurry transported, the lower this ratio.
References
- Agneessens, L., Vandecasteele, B., Van de Sande, T., Goovaerts, E., Crappé, S., Elsen, A., Willekens, W., & De Neve, S. (2014). Onderzoek naar het beheer van oogstresten bij vollegrondsgroenten en mogelijkheden van gewassen en teeltrotaties met het oog op de waterkwaliteitsdoelstellingen van het Actieprogramma 2011–2014 (MAP4): Hoofdrapport’, studie uitgevoerd in opdracht van de Vlaamse Landmaatschappij (VLM). http://www.vlm.be/nl//SiteCollectionDocuments/Mestbank/Studies/Studie%20oogstresten%20groenten/Project_Oogstresten_Hoofdrapport.pdf.
- Bocken, N. M. P., Short, S. W., Rana, P., & Evans, S. (2014). A literature and practice review to develop sustainable business model archetypes. Journal of Cleaner Production, 65, 42–56. https://doi.org/10.1016/j.jclepro.2013.11.039
- Brouckaert, A. (2022). Bemesten op maat van het perceel via bedrijfsadvies bemesting. Inagro. https://inagro.be/nieuws/bemesten-op-maat-van-het-perceel-bedrijfsadvies-bemesting.
- Buckwell, A., & Nadeu, E. (2016). Nutrient Recovery and Reuse (NRR) in European agriculture. A review of the issues, opportunities, and actions. RISE Foundation, Brussels.
- Bueno-Delgado, M. V., Molina-Martínez, J. M., Correoso-Campillo, R., & Pavón-Mariño, P. (2016). Ecofert: An android application for the optimization of fertilizer cost in fertigation. Computers and Electronics in Agriculture, 121, 32–42. https://doi.org/10.1016/j.compag.2015.11.006
- Caldeira, C., De Laurentiis, V., Corrado, S., van Holsteijn, F., & Sala, S. (2019). Quantification of food ton residues per product group along the food supply chain in the European Union: A mass flow analysis. Resources, Conservation and Recycling, 149, 479–488. https://doi.org/10.1016/j.resconrec.2019.06.011
- Chojnacka, K., Moustakas, K., & Witek-Krowiak, A. (2020). Bio-based fertilizers: A practical approach towards circular economy. Bioresource Technology, 295. https://doi.org/10.1016/j.biortech.2019.122223
- Clare, A., Barnes, A., McDonagh, J., & Shackley, S. (2014). From rhetoric to reality: Farmer perspectives on the economic potential of biochar in China. International Journal of Agricultural Sustainability, 12(4), 440–458. https://doi.org/10.1080/14735903.2014.927711
- De Dobbelaere, A., De Keulenaere, B., De Mey, J., Lebuf, V., Meers, E., Ryckaert, B., Schollier, C., & Van Driessche, J. (2015a). Small-scale Anaerobic Digestion: Case studies in Western Europe. Inagro vzw, Rumbeke-Beitem (Belgium).
- De Dobbelaere, A., Vervisch, B., Ryckaert, B., Annicaert, B., Lebuf, V., & Van Driessche, J. (2015b). Development of agro-sidestreams for energy. http://pomwvl.be/sites/default/files/uploads/duurzaam_ondernemen/doc/ARBOR_Agroresidues_Case20Study20Report.pdf bioenergy.
- De Dobbelaere, A., Willems, B., Vandendriessche, S., Leenknegt, J., & Meers, E. (2022). Potential of leek residues for biogas production through mono-digestion. Unpublished manuscript.
- De Haan, J., Wesselink, M., & Verstegen, H. (2019). Leek production in conventional and organic arable-vegetable rotations in the Netherlands. Acta Horticulturae, 1253(1253), 351–358. https://doi.org/10.17660/actahortic.2019.1253.46
- De Keyser, E., & Mathijs, E. (2023). A typology of sustainable circular business models with applications in the bioeconomy. Frontiers in Sustainable Food Systems, 6. https://doi.org/10.3389/fsufs.2022.1028877
- Departement Landbouw en Visserij. (2022). Landbouwgebruikspercelen LV, 2021 [Dataset; Geopunt]. https://www.vlaanderen.be/DataCatalogRecord/d869db4a-73f4-470e-b5ca-a0f7cd3ab585.
- Departement Landbouw & Visserij. (2022a). VLIF-investeringssteun voor land- en tuinbouwers. https://lv.vlaanderen.be/nl/subsidies/vlif-steun/vlif-investeringssteun-voor-land-en-tuinbouwers.
- Departement Landbouw & Visserij. (2022b). Projectsteun voor innovaties in de landbouw. https://lv.vlaanderen.be/nl/subsidies/vlif-steun/projectsteun-voor-innovaties-de-landbouw-0.
- Deuninck, J., & Vervloet, D. (2016). Rendabiliteits- en kostprijsanalyse groenten in open lucht. https://www.vlaanderen.be/publicaties/rentabiliteits-en-kostprijsanalyse-groenten-in-openlucht-op-basis-van-het-landbouwmonitoringsnetwerk.
- D’Haene, K., & Hofman, G. (2019). Marketable yield quantity and quality of leek and residual soil mineral nitrogen in relation to nitrogen fertilization. Acta Horticulturae, 1253(1253), 109–116. https://doi.org/10.17660/actahortic.2019.1253.15
- Duquette, E., Higgins, N., & Horowitz, J. (2012). Farmer discount rates: Experimental evidence. American Journal of Agricultural Economics, 94(2), 451–456. https://doi.org/10.1093/ajae/aar067
- Ecowerf. (2021a). Compost afhalen in een recyclagepark. https://www.ecowerf.be/compost-afhalen-in-een-recyclagepark.
- Ecowerf. (2021b). Recyclagepark (containerpark) Landen. https://www.ecowerf.be/recyclagepark-landen#tarief_kmo_voor_particulieren.
- European Commission. (2019). Fertilizers in the EU: prices, trade and use (Nr. 15). https://ec.europa.eu/info/sites/info/files/food-farming-fisheries/farming/documents/market-brief-fertilisers_june2019_en.pdf.
- FAOSTAT. (2022). Leeks and other alliaceous vegetables [Dataset]. https://www.fao.org/faostat/en.
- Fruit Logistica. (2021). European Statistics Handbook. https://www.fruitlogistica.com/fruit-logistica/downloads-alle-sprachen/auf-einen-blick/european_statistics_handbook_2021.pdf.
- Gebrezgabher, S. A., Meuwissen, M. P. M., Prins, B. A. M., & Lansink, A. G. J. M. O. (2010). Economic analysis of anaerobic digestion—A case of Green power biogas plant in The Netherlands. NJAS - Wageningen Journal of Life Sciences, 57(2), 109–115. https://doi.org/10.1016/j.njas.2009.07.006
- Glaser, B., Wiedner, K., Seelig, S., Schmidt, H.-P., & Gerber, H. (2015). Biochar organic fertilizers from natural resources as substitute for mineral fertilizers. Agronomy for Sustainable Development, 35(2), 667–678. https://doi.org/10.1007/s13593-014-0251-4
- Gobin, A., Campling, P., Janssen, L., Desmet, N., van Delden, H., Hurkens, J., Lavelle, P., & Berman, S. (2011). Soil organic matter management across the EU best practices, constraints and trade-offs. Final Report for the European Commission’s DG Environment. https://ec.europa.eu/environment/soil/pdf/som/full_report.pdf.
- Hall, R. L., & Hitch, C. J. (1939). Price theory and business behavior. Oxford Economic Papers, 2(1), 12–45. https://doi.org/10.1093/oxepap/os-2.1.12
- Holzworth, D. P., Huth, N. I., DeVoil, P. G., Zurcher, E. J., Herrmann, N. I., McLean, G., Chenu, K., van Oosterom, E. J., Snow, V., Murphy, C., Moore, A. D., Brown, H., Whish, J. P. M., Verrall, S., Fainges, J., Bell, L. W., Peake, A. S., Poulton, P. L., Hochman, Z., … Keating, B. A. (2014). APSIM – evolution towards a new generation of agricultural systems simulation. Environmental Modelling and Software, 62, 327–350. https://doi.org/10.1016/j.envsoft.2014.07.009
- IndexMundi. (2021). Urea - Monthly Price (Euro per Metric Ton) - Commodity Prices - Price Charts, Data, and News - IndexMundi. www.indexmundi.com. https://www.indexmundi.com/commodities/?commodity = urea&months = 120¤cy = eur.
- Jareonkitpoolpol, A., Ongkunaruk, P., & Janssens, G. K. (2018). Determination of the optimal blending problem of organic-chemical fertilizer under uncertainty. Soil Use and Management, 34(4), 449–460. https://doi.org/10.1111/sum.12449
- Keplinger, K., & Hauck, L. (2006). The economics of manure utilization: Model and application. Journal of Agricultural and Resource Economics, 31((02|2)), 414–440. https://doi.org/10.22004/ag.econ.8611
- Khan, S. A., Mulvaney, R. L., Ellsworth, T. R., & Boast, C. W. (2007). The myth of nitrogen fertilization for soil carbon sequestration. Journal of Environmental Quality, 36(6), 1821–1832. https://doi.org/10.2134/jeq2007.0099
- McCormick, K., & Kautto, N. (2013). The bioeconomy in Europe: An overview. Sustainability, 5(6), 2589–2608. https://doi.org/10.3390/su5062589
- Monitoring Flanders. (2017). Food waste and food losses: Prevention and Valorisation. Flemish Food Supply Chain Platform for Food Loss. https://www.voedselverlies.be/en.
- Ochieng, J., Afari-Sefa, V., Muthoni, F., Kansiime, M., Hoeschle-Zeledon, I., Bekunda, M., & Thomas, D. (2022). Adoption of sustainable agricultural technologies for vegetable production in rural Tanzania: Trade-offs, complementarities and diffusion. International Journal of Agricultural Sustainability, 20(4), 478–496. https://doi.org/10.1080/14735903.2021.1943235
- Pergola, M., Persiani, A., Palese, A. M., Di Meo, V., Pastore, V., D’Adamo, C., & Celano, G. (2018). Composting: The way for a sustainable agriculture. Applied Soil Ecology, 123, 744–750. https://doi.org/10.1016/j.apsoil.2017.10.016
- Platteau, J., Lambrechts, G., Roels, K., & Van Bogaert, T. (2018). Landbouwrapport 2018. In Departement Landbouw en Visserij (Issue december). Departement Landbouw en Visserij. Vlaamse Overheid. https://rundveeloket.be/sites/default/files/inline-files/2018 Landbouwrapport.pdf.
- Pribyl, D. W. (2010). A critical review of the conventional SOC to SOM conversion factor. Geoderma, 156(3–4), 75–83. https://doi.org/10.1016/j.geoderma.2010.02.003
- Rahn, R. C., Zhang, K., Lillywhite, R., Ramos, C., Doltra, J., de Paz, J., Riley, H., Fink, M., Nendel, C., Thorup-Kristensen, K., Pedersen, A., Piro, F., Venezia, A., Firth, C., Schmutz, U., Rayns, F., & Strohmeyer, K. (2010). The development of the EU-rotate_N model and its use to test strategies for nitrogen use across Europe. Acta Horticulturae, 852(852), 73–76. https://doi.org/10.17660/actahortic.2010.852.7
- Rodias, E. C., Sopegno, A., Berruto, R., Bochtis, D. D., Cavallo, E., & Busato, P. (2019). A combined simulation and linear programming method for scheduling organic fertiliser application. Biosystems Engineering, 178, 233–243. https://doi.org/10.1016/j.biosystemseng.2018.11.002
- Schiavon, S., Dal Maso, M., Cattani, M., & Tagliapietra, F. (2009). A simplified approach to calculate slurry production of growing pigs at farm level. Italian Journal of Animal Science, 8(3), 431–455. https://doi.org/10.4081/ijas.2009.431
- Sleutel, S. (2005). Carbon sequestration in cropland soils: Recent evolution and potential of alternative management options. PhD thesis, Ghent University, Ghent, Belgium.
- Snyder, C. S., Bruulsema, T. W., Jensen, T. L., & Fixen, P. E. (2009). Review of greenhouse gas emissions from crop production systems and fertilizer management effects. Agriculture, Ecosystems & Environment, 133(3–4), 247–266. https://doi.org/10.1016/j.agee.2009.04.021
- Spanoghe, J., Grunert, O., Wambacq, E., Sakarika, M., Papini, G., Alloul, A., Spiller, M., Derycke, V., Stragier, L., Verstraete, H., Fauconnier, K., Verstraete, W., Haesaert, G., & Vlaeminck, S. E. (2020). Storage, fertilization and cost properties highlight the potential of dried microbial biomass as organic fertilizer. Microbial Biotechnology, 13(5), 1377–1389. https://doi.org/10.1111/1751-7915.13554
- Statistiek Vlaanderen. (2019). Veestapel. https://www.statistiekvlaanderen.be/nl/veestapel.
- Steffen, W., Richardson, K., Rockstrom, J., Cornell, S. E., Fetzer, I., Bennett, E. M., Biggs, R., Carpenter, S. R., de Vries, W., de Wit, C. A., Folke, C., Gerten, D., Heinke, J., Mace, G. M., Persson, L. M., Ramanathan, V., Reyers, B., & Sorlin, S. (2015). Planetary boundaries: Guiding human development on a changing planet. Science, 347(6223), 736–747. Article ID 1259855. https://doi.org/10.1126/science.1259855
- Therond, O., Duru, M., Roger-Estrade, J., & Richard, G. (2017). A new analytical framework of farming system and agriculture model diversities. Agronomy for Sustainable Development, 37(3), 1–24. https://doi.org/10.1007/s13593-017-0429-7
- Thomsen, I. K., Olesen, J. E., Møller, H. B., Sørensen, P., & Christensen, B. T. (2013). Carbon dynamics and retention in soil after anaerobic digestion of dairy cattle feed and faeces. Soil Biology and Biochemistry, 58, 82–87. https://doi.org/10.1016/j.soilbio.2012.11.006
- Van den Burgt, G. J., & Hanegraaf, M. C. (2021). Modelleren van stikstof en organische stof met NDICEA; Toepassing op datasets van de systeemproeven Bodemkwaliteit op zand en BASIS. Wageningen Research, Rapport WPR-880.
- Van Der Burgt, G., & Timmermans, B. (2009, June 24–26). The NDICEA model: A supporting tool for nitrogen management in arable farming (pp. 4–102). BIOACADEMY 2009 - Proceedings, organic farming: a response to economic and environmental challenges, Lednice na Moraveˇ, Czech Republic.
- Van der Meulen, S., Grijspaardt, T., Mars, W., van der Geest, W., Roest-Crollius, A., & Kiel, J. (2020). Cost Figures for Freight Transport – final report. Panteia. https://www.google.com/url?sa = t&rct = j&q = &esrc = s&source = web&cd = &ved = 2ahUKEwjQrsyOwbLvAhVMnqQKHaq2ClgQFjAAegQIBhAD&url = https%3A%2F%2Fwww.kimnet.nl%2Fbinaries%2Fkimnet%2Fdocumenten%2Fformulieren%2F2020%2F05%2F26%2Fcost-figures-for-freight-transport%2FCost%2Bfigures%2Bfor%2Bfreight%2Btransport%2B-%2Bfinal%2Breport.pdf&usg = AOvVaw3GwMv49W_0ydeLLOOCschD.
- Vaneeckhaute, C., Meers, E., Michels, E., Ghekiere, G., Accoe, F., & Tack, F. M. G. (2013). Closing the nutrient cycle by using bio-digestion ton residues derivatives as synthetic fertilizer substitutes: A field experiment. Biomass and Bioenergy, 55, 175–189. https://doi.org/10.1016/j.biombioe.2013.01.032
- Vanrespaille, H., Smets, S., Verbeke, M., Gorissen, A., Hex, L., Elsen, A., & Bries, J. (2018). Organische bemesting: Wat en hoe? Bodemkundige Dienst van België vzw. https://www.vcm-mestverwerking.be/nl/nieuws/48/publicatie-praktijkgids-organische-bemesting-wat-en-hoe.
- Veeken, A., Adani, F., Fangueiro, D., & Stoumann Jensen, L. (2017). The value of recycling organic matter to soils: Classification as organic fertiliser or organic soil improver. EIP-AGRI. https://ec.europa.eu/eip/agriculture/sites/default/files/fg19_minipaper_5_value_of_organic_matter_en.pdf.
- Viaene, J., Agneessens, L., Capito, C., Ameloot, N., Reubens, B., Willekens, K., Vandecasteele, B., & De Neve, S. (2017). Co-ensiling, co-composting and anaerobic co-digestion of vegetable crop residues: Product stability and effect on soil carbon and nitrogen dynamics. Scientia Horticulturae, 220, 214–225. https://doi.org/10.1016/j.scienta.2017.03.015
- VITO. (2015). Code van goede praktijk bodembescherming. https://esites.vito.be/sites/reflabos/2015/Online%20documenten/CVGP_versie_februari_2015.pdf.
- Vlaams Energie- en Klimaatagentschap. (2022). Steun micro-WKK. https://www.energiesparen.be/steun-micro-wkk.
- VLACO. (2009a). Ecologische en economische voordelen digestaat. https://www.vlaco.be/sites/default/files/generated/files/page/ecol-en-econ-waardering-digestaat-vlaco-vzw.pdf.
- VLACO. (2009b). Ecologische en economische voordelen gft- en groencompost. https://www.vlaco.be/sites/default/files/generated/files/page/ecologische-en-economische-waardering-compost-eindversie-1.pdf.
- VLACO. (2019). Gemiddelde samenstelling van Vlaco-compost | Vlaco. https://www.vlaco.be/compost-gebruiken/wat-is-compost/gemiddelde-samenstelling-van-vlaco-compost.
- VLM. (2022a). Normen en richtwaarden 2022. Vlaamse Landmaatschappij. https://www.vlm.be/nl/SiteCollectionDocuments/Publicaties/mestbank/Bemestingsnormen_2022.pdf.
- VLM. (2022b). Sancties | Vlaamse Landmaatschappij. Vlaamse Landmaatschappij. https://www.vlm.be/nl/themas/waterkwaliteit/Mestbank/Controle/sancties/Paginas/default.aspx.
- VREG. (2022). Prijzen Elektriciteit. https://dashboard.vreg.be/report/DMR_Prijzen_elektriciteit.html.
- World Bank Group. (2022). Belgium. World Bank Climate Change Knowledge Portal. https://climateknowledgeportal.worldbank.org/country/belgium/climate-data-historical.
- Yara. (2020). Yara Sustainability Report 2020. https://www.yara.com/siteassets/investors/057-reports-and-presentations/annual-reports/2020/yara-sustainability-report-2020-web.pdf/.
- Zemo, K. H., & Termansen, M. (2018). Farmers’ willingness to participate in collective biogas investment: A discrete choice experiment study. Resource and Energy Economics, 52, 87–101. https://doi.org/10.1016/j.reseneeco.2017.12.001
Appendix A
Table A1. Farm specific data (based on FADN, De Dobbelaere et al., Citation2015b; Deuninck & Vervloet, Citation2016; D’Haene & Hofman, Citation2019; VITO, Citation2015; VLM, Citation2022a).
Table A2. Anaerobic digestion production data (based on Agneessens et al., Citation2014; De Dobbelaere et al., Citation2015a; Van der Meulen et al., Citation2020; VREG, Citation2022).
Table A3. Fertilizer characteristics (based on De Dobbelaere et al., Citation2021a; Ecowerf, Citation2021a; IndexMundi, Citation2021; Pribyl, Citation2010; Sleutel, Citation2005; Thomsen et al., Citation2013; Vanrespaille et al., Citation2018; Veeken et al., Citation2017; Viaene et al., Citation2017; VLACO, Citation2009a, Citation2019).