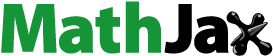
ABSTRACT
Spatiotemporal studies of the annual and seasonal climate variability and trend on an agroecological spatial scale for establishing a climate-resilient maize farming system have not yet been conducted in Ethiopia. The study was carried out in three major agroecological zones in northwest Ethiopia using climate data from 1987 to 2018. The coefficient of variation (CV), precipitation concertation index (PCI), and rainfall anomaly index (RAI) were used to analyze the variability of rainfall. The Mann-Kendall test and Sen’s slope estimator were also applied to estimate trends and slopes of changes in rainfall and temperature. High-significance warming trends in the maximum and minimum temperatures were shown in the highland and lowland agroecology zones, respectively. Rainfall has also demonstrated a maximum declining trend throughout the keremt season in the highland agroecology zone. However, rainfall distribution has become more unpredictable in the Bega and Belg seasons. Climate-resilient maize agronomic activities have been determined by analyzing the onset and cessation dates and the length of the growth period (LGP). The rainy season begins between May 8 and June 3 and finishes between October 26 and November 16. The length of the growth period (LGP) during the rainy season ranges from 94 to 229 days.
1. Introduction
Maize (Zea mays L.) is one of the most important cereal food crops worldwide, along with rice and wheat (Yarnell, Citation2008). It is a well-established and substantial crop for human food in several countries, especially in sub-Saharan Africa (SSA), Latin America, and a few countries in Asia, where it is consumed as human food and makes up about 20% of dietary calories (Shiferaw et al., Citation2011). Additionally, it is widely known that maize is the most crucial crop for ensuring food security in many countries, particularly in East Africa and throughout all of Ethiopia. In Ethiopia, maize is the second widely grown crop in terms of area coverage after teff (Eragrostis tef (Zuccagni) Trotter), and it is also the first productive among the main cereal crops. It can be grown in every agroecological zone in Ethiopia since it has a good capacity for coping with climatic stress in tropical and subtropical environments (Aman & Dawid, Citation2020). It is currently replacing the areas where wheat and teff are grown in the high-altitude agroecological zone, despite the fact that it is generally planted in the low-to medium-altitude agroecological zone (Gorfu & Ahmed, Citation2011). According to Taye et al. (Citation2019), the highland agroecology zone of our study location was suitable for the growth of maize.
Maize productivity is low compared to the global average, particularly in Ethiopia (Abate et al., Citation2015). The main causes of these problems include decreased soil fertility, inadequate input use, poor seed quality, pest and disease infestations, ineffective agronomic management techniques, and climate variability. Temperatures and rainfall variability have been found to have the greatest influence on maize yields in the northwest Ethiopian regions of Ethiopia out of all the above-mentioned variables. Recent research carried out at several sites provides strong evidence that variations in temperature and precipitation have an effect on the productivity of maize (Simane et al., Citation2016; Brown et al., Citation2017; Debela et al., Citation2015; Addisu et al., Citation2016; Gedefaw et al., Citation2018). Therefore, rainfall and temperature are two key climate variables that influence the vegetative growth and production of maize (Teshome et al., Citation2021). Thus, a number of studies on spatiotemporal climate variability and trend analysis were carried out in Ethiopia at the national level or for specific regions (Asfaw et al., Citation2016; Birara et al., Citation2018; Ademe et al., Citation2021; Asrat & Simane, Citation2018; Addis & Abirdew, Citation2021; Berhane et al., Citation2020).
However, detailed analysis of the seasonal and annual rainfall and temperature variability and trend, associated with rainfall onset and cessation dates, and LGP at the agroecological spatial scale for building climate-resilient maize farming systems has not yet been carried out in the study area. These characteristics have a significant impact on the agronomic tasks that must be performed in rain-fed agricultural systems, making them particularly crucial for constructing climate-resilient farming systems (Wakjira et al., Citation2021).
Resilience can be defined as an agricultural system's capacity to respond, absorb, or adapt to stress and change after a perturbation (Cabel & Oelofse, Citation2012). Therefore, a ‘resilient’ maize farming system would be able to produce maize even in the face of extreme temperatures and rainfall in a particular agroecological zone for maize production. The choice of a maize variety and determining planting and harvest dates based on the current weather are the main climate-resilient agronomic techniques explored in these studies. These practices involve the entire community, whose livelihood is strongly dependent on maize. Therefore, the spatiotemporal rainfall and temperature variability and trend analysis on an agroecology basis was an urgent need and highly relevant for restructuring maize farming systems in the maize-growing agroecology zones in our study area.
Ethiopia has three main agroecological zones, namely: the Highland Agroecology Zone (locally kwon as dega), the Midland Agroecology Zone (locally kwon as woina dega), and the Lowland Agroecology Zone (locally kwon as kola), with elevations of 2300–3200, 1500–2300, and 500–1500 masl, respectively (Gorfu & Ahmed, Citation2011). Agroecological zones have prominent physical features that can be divided into regions with similar altitude, climate, soil, and agricultural land uses. We chose agroecology as the appropriate geographical framework for our research because it is more important to the development of a climate-resilient maize farming system than administrative boundaries or river basins. Because of this, we used a novel crop-agroecological zonation approach that is unique to Sub-Saharan Africa in general and Ethiopia in particular to analyses climate variability and trend.
Ethiopia has three different seasons, called locally ‘bega’ (October to January), “belg” season (February to May), and “kiremt” (June to September). Specifically, the kirem, spring, and winter seasons are characterized by major rainy, small rainy, and dry conditions, respectively (Alemayehu & Bewket, Citation2016). The majority of agricultural activities were done in all of the maize-growing agroecology zones in northwest Ethiopia during the kiremt seasons. Delay in the onset of spring and kiremt season will cause livestock feed shortages, and early planted crops will be negatively affected (Asfaw et al., Citation2018; Eggen et al., Citation2019). These problems could need to be addressed by the development of a climate-resilient maize farming system through the temporal analysis of rainfall and temperature variability and trend. Therefore, the objective of the study was to analyzed rainfall and temperature variability and trends for building climate-resilient maize farming systems in major agroecology zones in northwest Ethiopia.
2. Materials and methods
2.1. Description of the study site
The study was carried out in the maize growing belt of the Mecha district in the northwest agroecology zones of Ethiopia (). These areas are located between 11.30°N latitude and 37.20°E longitude. The altitude ranges from 1000 to 3131 m.a.s.l. The average annual temperature and precipitation for the study area are 24.17°C and 1397.37 mm, respectively. The study region is divided into three zones based on Ethiopia's conventional agroecological classifications. Highland (low-productive agroecology zones), midland (high-productive agroecology zones), and lowland (medium-productive agroecology zones) agroecology zones (AEZ) (Gorfu & Ahmed, Citation2011), with elevations of 2300–3200, 1500–2300, and 500–1500 m.a.s.l., constituting 32%, 51%, and 17% of the total land of the study area, respectively. The primary source of income for the people living in the study region is mixed subsistence farming, which includes both agricultural agriculture and animal husbandry (Tessema & Simane, Citation2019).
2.2. Data type and source
The Enhancing National Climate Services (ENACTS) dataset was used to acquire daily precipitation and minimum and maximum temperature data for the research areas from 1987 to 2018. ENACTS is the first high-resolution gridded surface meteorological dataset (4 × 4 km) produced specifically for studies on surface climate processes in Ethiopia (Dinku et al., Citation2014). The Ethiopian National Meteorological Agency provided ENACTS data for 10 locations in each of the three maize agroecological zones in the Mecha District of northwest Ethiopia. Because of the following fundamental factors, ENACTS data have been utilized for climate studies recently: a. Due to the small number of stations, traditional measurements of climate characteristics did not accurately represent all the study sites (Alemayehu & Bewket, Citation2016). b. Because of the uneven distribution of the ground stations, there may be significant distances between them, sometimes exceeding 50 km. c. Due to recently installed stations, the majority of them did not offer long seasonal data records for trend research (Alemayehu & Bewket, Citation2016; Asfaw et al., Citation2018; Dinku et al., Citation2014); d. due to the fact that the station datasets have a significant amount of missing values (Asfaw et al., Citation2018).
2.3. Data analysis
2.3.1. Variability analysis
The coefficient of variation (CV), a relative measure of variability, displays the amount of a standard deviation relative to its mean. As a result, the coefficient of variation (CV) was used to determine the variability of annual and seasonal events:
(1)
(1) Where, CV is the coefficient of variation, σ is the standard deviation and µ is the mean precipitation of the recording period. Divided the degree of rainfall variability into the following categories: CV < 20%, indicates less variable, CV = 20% to 30%, indicates moderately variable and CV = 30%, indicates highly variable.
The Precipitation Concentration Index (PCI) is the main tool for identifying whether the region's rainfall patterns are regular or erratic. To assess the heterogeneity of intra-annual rainfall amount, the precipitation concentration index (PCI) was used (De Luis et al., Citation2011).
(2)
(2) Where Pi = rainfall amount of the ith month. The precipitation concentration index was also calculated on a seasonal scale for Kiremt rain season (June–Sep), Bega season (Oct–Jan) and Belg rain season (Feb–May), and using the following formula (De Luis et al., Citation2011).
(3)
(3)
PCI values were categorized as uniform (<10), moderate (11–15), irregular (16–20), and strongly irregular (>20) in monthly rainfall distributions (De Luis et al., Citation2011).
The rainfall anomaly index (RAI) is used as a single hydro-climatic metric to evaluate the wetness and dryness conditions related to climatic change. Therefore, the annual variability of rainfall was evaluated using the rainfall anomaly index (RAI) used by Tilahun (Citation2006), which is calculated as follows for positive anomalies:
(4)
(4) And for negative anomalies.
(5)
(5) Where; RAI = rainfall anomaly index, RF = the actual rainfall for a given year, MRF = mean annual rainfall over the full record of analysis, MH10 = the mean of the 10 highest values of annual rainfall on record, and ML10 = the mean of the 10 lowest values of annual rainfall on record. Years with positive and negative anomaly indicate years of high and low rainfall, respectively, compared to the mean climatology. Annual temperature anomaly was computed as the difference between a year’s average temperature and the long-term mean.
2.3.2. Trend analysis
Before performing the Mann-Kendall test, the autocorrelation issue was investigated for all rainfall and temperature time series data by computing the acf() function in the R software at a 5% significance level. Trends were analyzed using Sens’ slope estimator and modified Mann-Kendall (MK) trend tests (non-parametric trend tests). The MK trend test is the most suitable and favoured nonparametric test for identifying trends in time series climate data. This method is less influenced by missing values and uneven data distribution, and it is less sensitive to outliers because it considers the ranks of the observations rather than their actual numbers (Belay et al., Citation2019). S-Statistics: S-Statistics was applied to check for an increasing, decreasing, or no trend on the hydrometeorological data series in each of the selected weather station data in the MK test statistic, which is given as:
(6)
(6) Where n = number of data points, Xk and Xj = data values in time series k and j (j > k), and sgn(xj-xk) is defined as:
(7)
(7) The variance of S is computed as:
(8)
(8) where n is the number of data, m is the number of tied groups and tp is the number of data points in the ith group. Z-statistics test: The test statistics Z was used as a measure of significance of trend. If the value of Z is positive, it indicates increasing trends, while negative values of Z show decreasing trends. The value of S and VAR(S) are used to compute the test static Zs as follows.
(9)
(9) Z0 is a null hypothesis that signifies the trend is not significant and is recognized if Z statistics are statistically insignificant (Za/2< Z < Zα/2), where Zα/2 is the standardized normal deviation (Modarres & de Paulo Rodrigues da Silva, Citation2007). Hence, for the purpose of this study, 1, 5, and 10% significance levels were considered. Sen’s slope estimator and percentage change: the magnitude of the trend was estimated through Sen’s slope estimator in a non-parametric procedure. Both the slope (i.e. linear rate of change) and intercepts were computed according to Sen’s method. Likewise, the linear model can be calculated as follows:
(10)
(10) Where Q is the slope, B is constant, a set of linear slopes is calculated as follows:
(11)
(11)
Where Q is the slope, X denotes the variable, n is the number of data, and j, k are indices where j > k. The slope is estimated for each observation and the corresponding intercept is also the median of all intercepts. Median is computed from N observations of the slope to estimate the Sen’s Slope estimator:
(12)
(12) Where n is the number of time periods. The N values of Qi were ranked from smallest to largest and the median of slope or Sen’s estimator was computed as:
(13)
(13) Positive/negative values of Qi indicate an increasing/decreasing trend, respectively. Confidence intervals (Cα) about the time slopes were used to test significance of the trend and were computed as follows:
(14)
(14)
2.4. Analysis of onset, cessation date, and length of growing period (LGP)
In this study, we used the criteria to determine the onset, cessation date (end of the growing season or rainy season), and length of growing period (LGP) across the Mech district in northern Ethiopia from 1987 to 2018. All these variables were analyzed and estimated using Instat software version 3.36. Accordingly, the day that observed 20 mm of rainfall over three consecutive days without being followed by a dry spell longer than nine days within 30 days of planting day was considered the onset. whereas the stored soil water and its availability to the crop after the rainy season ended were referred to as the cession date (Stern et al., 2006). The Length of the growing period (LGP) is the average time it takes for rain to fall between the beginning and the cessation date. Then the LGP was determined between the onset and cessation dates of the growing season using meteorological data (1987–2018).
As a result, climate-resilient activities like the planting and harvest dates for the current climate condition were determined using the onset and session dates of rainfall, respectively. Whereas the LGP determines how crops and farming practices are distributed spatially in any given region. It is also critical to take the maturation period based on the rainfall regime into account when choosing the cultivars to be produced. Based on LGP analysis climate-resilient maize varieties suitable for each of the three main agroecological zones have been suggested. Hence, the onset, cessation date, and length of the growing period were analyzed using meteorological data (1987–2018) to recommend climate-resilient maize varieties suitable for each of the three main agroecological zones.
3. Results and discussion
3.1. Rainfall and temperature variability and trend
3.1.1. Rainfall variability
Based on the coefficient of variation (CV) values in , the annual and seasonal rainfall were not significantly influenced by space and time. Less spatial and temporal rainfall variability was observed at the Kiremt seasonal and annual time scales across the study area. On the contrary, high rainfall variability was recorded at the bega and belg seasons in all maize productive agroecology zones (). Similar findings were obtained by Bayable et al. (Citation2021) who confirmed that seasonal rainfall has greater inter-annual variability in the dry season (bega and belg) than in other seasons in the west Harerge Zone, eastern Ethiopia. Additionally, found that the seasonal variance in rainfall was large in the belg and bega, moderate in the Kiremt and low throughout the year. According to spatial analysis, the highland tends to show a slightly higher coefficient of variation than the lowland and midland maize growing agroecology zones in the seasonal and annual time scales, with the exception of belg seasonal rainfall (). This may be due to the fact that the highland is found at a high elevation as compared to the lowland and midland agroecology zones. Similar findings demonstrated that the highest value of rainfall variability was found in the agroecological zone at higher elevations in our study area (Taye et al., Citation2019). Contrary to our findings, Belay et al. (Citation2019) confirmed high rainfall variability in the lowland of the Western Margins of the Ethiopian Highlands between March and April (belg season).
Table 1. Annual and seasonal rainfall (mm), coefficient of variation (CV) and precipitation concentration index (PCI) (1987–2018).
The PCI analysis of annual rainfall showed an irregular year-to-year rainfall distribution in the study area. PCI analysis for seasonal rainfall revealed a highly irregular rainfall distribution in the study area (). The findings are directly comparable to those of Dawit et al. (Citation2019), who discovered significant irregularities in precipitation distribution during the kiremt seasons in the Guna Tana watershed, Upper Blue Nile Basin, Ethiopia. The bega and belg seasons, in particular, showed greater monthly variability than the kiremt season and annual scale (). Thus, a more erratic rainfall distribution has been shown in the bega and belg seasons in all maize crop production areas (Ademe et al., Citation2021)
3.1.2. Rainfall trends
The result of trend-test analysis for thirty stations in the study areas (1987–2018) is shown in . Except for the Belg season at lowland agroecology zones, the trend of rainfall decreased but was not statistically significant at α = 0.05 significance level in most of the maize productive agroecology zones during annual and seasonal time scales (1987–2018) (). The results of our analysis were confirmed by Belay et al. (Citation2021), who found that the Kiremt (summer), Bega (dry), and Belg (spring) seasonal rainfall showed a decreasing trend in Southern Ethiopia. During the annual and kiremt seasons, rainfall decreased by 63 and 41 mm per annum in highland and lowland agroecology zones, respectively. Therefore, relatively higher decreasing trends of rainfall were observed in the highland agroecology zones than in the lowland and midland agroecology zones during the annual time scales. Similar to this result, the declining trend for annual and kiremt rainfall was found at the Woleka sub-basin, northcentral Ethiopia (Asfaw et al., Citation2018). Recent research by Etana et al. (Citation2020) also showed that the amount of precipitation has reduced significantly more in the highland areas than in the lowland areas in three agroecological settings in Central Ethiopia. Therefore, appropriate maize cultivars will probably be needed for this agroecology zone in the future.
Table 2. Trends of rainfall in the northwest Ethiopia agroecology zone (1987–2018).
3.1.3. Rainfall anomaly index
The findings of the spatiotemporal analysis of the rainfall anomaly index (RAI) for the annual and seasonal time scales in the northwest Ethiopian maize agroecological zones are shown in . The highest positive and negative rainfall anomalies were observed in the 1990s and 2010s, respectively (). While a large proportion of negative anomalies were shown in annual (54.8%) and Bega season rainfall (61.3%), positive anomalies were exhibited in kiremt (41.9%) and belg season (48.4%). Most of the years with negative rainfall anomalies in the study area were caused by El Nio events. Similar findings were observed by Ademe et al. (Citation2020), who found that most rainfall anomalies between 1981 and 2016 corresponded with El Nio and La Nia events in an agricultural area of the Ethiopian Highlands. Therefore, the warm and cold ENSO occurrences (El Nio and La Nia) as well as the seasonal shift of the inter-tropical convergence zone (ITCZ) can also be considered contributing factors to this variability (Bayable et al., Citation2021). The results of the precipitation anomaly index effectively reflected the seasonal patterns of precipitation for Kiremt and the Bega rains, which feature erratic and unpredictable rainfall patterns that have an effect on both crop and livestock production.
3.1.4. Temperature variability
A spatiotemporal analysis of the average annual and seasonal minimum and maximum temperatures is shown in . The majority of temperature anomalies were negative from 1987 to 2000, but they changed to positive from 2001 to 2018. Hence, in comparison to the 1990s, the 2000s were also the warmest decade in terms of maximum and minimum temperatures. This result is in line with Tirfi and Oyekale (Citation2022), who showed anomalies in maximum and minimum temperatures were negatively low in 1981–1997 but positive in 2003–2018 in the Teff growing belts of Ethiopia. The highest positive and negative maximum temperature anomalies were shown in the highland-Belg (61.29%) ((g)) and lowland-Bega (54.83%) ((i)), respectively, over the last 32 years (1987–2018). This indicated that relatively warm and cool conditions were observed during the Belg and Bega seasons at highland and lowland agroecology zones from 1987 to 2018. In general, increasing trends for annual and seasonal maximum temperature anomalies were recorded for all maize growing agroecology zones and seasons in the study area ().
Figure 4. Spatial (horizontal) and temporal (vertical) minimum temperature anomalies in the north west Ethiopia (1987–2018). Source: own study. (a) Highlad-Annual (b) Midland-Annual (c) Lowland-Anual (d) Highlad-Kiremt (e) Midland- Kiremt (f) Lowland- Kiremt (g) Highland-Belg (h) Midland-Bleg (i) Lowland-Bleg (j) Highland-Bega (k) Midland –Bega (l) Lowland-Bega.

The highest positive and negative annual minimum temperature anomalies were measured in lowland (51.62%) ((A)) and highland agroecology zones (70.97%) ((c)), respectively, whereas the highest positive and negative seasonal minimum temperature anomalies were exhibited in midland-kiremt (61.29%) ((e)) and highland-kiremt (67.75%) ((d)), respectively, in the study period (1987–2018). In general, positive and negative anomalies account for 43% and 57% of the total observations, respectively, throughout the study period and maize productive agroecology zone (). This indicated that the general trends of the annual and seasonal anomalies in the minimum temperature had similar patterns to those of the maximum temperature.
3.1.5. Temperature trend
(a and b) show trends in minimum and maximum temperatures based on the Mann-Kendall test for three maize agroecology zones. In all research regions over the past 32 years (1987–2018) statistically significant increasing trends for annual and seasonal maximum and minimum temperatures were evaluated based on S or Z values (). The highland agroecology zone in particular showed the largest warming trend in maximum temperature throughout the annual and kiremt seasons, while the lowland agroecology zone showed the lowest warming trend in maximum temperature across all seasons ((a)). In contrast to our findings, Etana et al. (Citation2020) found a considerable warming trend in the maximum temperature during an annual time period in the lowland agroecology of Central Ethiopia.
Table 3. Temperature trends in northwest Ethiopia agroecology zone maize production area (1987–2018).
In all time scales from the 1987 to 2018 years, with the exception of the Belg season, significant (a = 0.001) warming trend in minimum temperatures were detected from lowland agroecology zones ((b)). While the lowest warming trend was on display from the midland agroecology zone all over the study area and period. The result of this study agrees with previous studies that indicated the annual maximum and minimum temperatures are currently trending upward in different stations in Ethiopia (Ademe et al., Citation2021; Habte et al., Citation2021).
Table 4. Statistical description of rainfall features in the study areas.
3.2. Analysis of the onset, cessation date and length of growing period
After examining temperature and rainfall variations and trends, we carried out the analyses of onset date, cessation date, and length of growing period (LGP) with the aim of developing climate-resilient maize agricultural systems. Kiremt is the main rainy season, when 85% to 95% of the country's food crops are produced (Gorfu & Ahmed, Citation2011). All agroecological zones in the research area use kiremt as their primary growing season, hence we focused on rainfall event analysis during this time.
Accordingly, May 8 (the second dekad of May) and June 3 (the first dekad of June) are the lower and upper quartiles of the date of onset of rainfall, respectively (). While the midpoint of the onset of rainfall was May 17 (the third dekad of May). Our findings totally agree with those of Bedane et al. (Citation2022), who report that the onset date started on May 22 (the third dekad of May) with a potential accumulation of more than 20 mm in the central Ethiopian highlands; thus, the planting date of maize crops could range from the second week of May up to the first week of June. The third week of May can be used as an optimal planting date in and around the study area. Therefore, the second week of May, the third week of May, and the first weeks of June would be suitable planting dates for long, medium, and short maturing maize varieties during the kiremt season in the highland, midland, and lowland agroecology zones of the Mecha areas in northwest Ethiopia, respectively.
The lower and upper quartiles are October 26 (the fourth dekad of October) and November 16 (the third dekad of November), respectively, and the median cessation date is November 6 (the first dekad of November) with a CV of 15.3% and SD of 4.3 days. Accordingly, on average, the rainy season ends in the first dekad of November in study area (). Harvesting, transporting, storing, and marketing of maize crop could thus be more easily accomplished in the study area after the first dekad of November. In this case, the third week of October, first week of November and third week of November could be an ideal harvesting week for short, medium and long maturing maize varieties in lowland, midland and long agroecology zones of Mecha northwest Ethiopia, respectively.
The LGP in the research region varies from 94 to 229 days during the kiremt rainy season, with a mean of 176 days, a CV of 8.2%, and an SD of 29.3 days, respectively. Therefore, according to agroecology, farmers in the northwest of Ethiopia must select ripening maize types for the 94–229-LGP.
4. Conclusions
This study employed the spatiotemporal analysis of rainfall and temperature variability and trends for establishing a climate-resilient maize farming system in the major agroecology zones of Northwest Ethiopia. Our research focused on three major agroecological zones associated with three distinct seasons in the Mecha area of northwestern Ethiopia. After examining temperature and rainfall variations and trends, we carried out analyses of onset date, cessation date, and length of growing period (LGP) with the aim of developing climate-resilient maize agricultural systems.
In the seasonal and annual time scales (1987–2018), the highland agroecology zone tends to exhibit higher variation and declining trends in rainfall than the midland and lowland agroecology zones. In annual rainfall, a high percentage of negative anomalies were visible (54.8%). Most of these negative rainfall anomalies in the study region coincided with El Niño events. The majority of temperature anomalies were negative from 1987 to 2000, but they changed to positive from 2001 to 2018. Hence, in comparison to the 1990s, the 2000s were also the warmest decade in terms of maximum and minimum temperatures in the study area. Specifically, the highland agroecology zone displayed the highest rising trend in maximum temperature over the course of the annual and kiremt seasons. Whereas the lowland agroecology zones showed a highly significant (a = 0.001) warming trend in all time periods from 1987 to 2018, with the exception of the Belgian season. In general, over the past 32 years (1987–2018), statistically significant rising trends for annual and seasonal maximum and minimum temperatures were observed from all research locations.
The second week of May marked the beginning of rainfall, which continued until the first week of June. The third week of May marked the midpoint of the onset of rainfall. Thus, the planting date of maize crops could range from the second week of May up to the first week of June. The third week of May can be used as an optimal planting date in and around the study area. Therefore, the second week of May, the third week of May, and the first weeks of June would be suitable planting dates for long, medium, and short maturing maize varieties during the kiremt season in the highland, midland, and lowland agroecology zones of the Mecha areas in northwest Ethiopia, respectively. The fourth week of October, the first week of November, and the third week of November were the early, median, and late cessation dates, respectively. In this case, the third week of October, the first week of November, and the third week of November could be ideal harvesting weeks for short, medium, and long maturing maize varieties in lowland, midland, and long agroecology zones of Mecha, northwest Ethiopia, respectively. The LGP in the research region varies from 94 to 229 days. Therefore, according to agroecology, farmers in the northwest of Ethiopia must select ripening maize types for the 94–229-LGP.
In conclusion, all of the northwest Ethiopian agroecology zones have shown persistent warming trends and increasingly irregular and concentrated rainfall patterns. The following climate-resilient maize farming activities should be implemented in the research region and other agroecological zones: the second week of May, the third week of May, and the first weeks of June would be ideal planting dates for long, medium, and short maturing maize types during the kiremt season, respectively. Whereas the third week of October, the first week of November, and the third week of November could be ideal harvesting weeks for short, medium, and long maturing maize varieties depending on agroecology, respectively. In general, maize varieties should ripen between 94 and 229 LGP.
Authors’ contributions
Abebe Zeleke: Responsible for conceptualization, designed research method, data collection, analysis, and writing-original draft; Kindie Tesfaye: Conceptualization, methodology, investigation, validation, writing-review & editing; Tilahun Tadesse: Conceptualization, supervision, writing-review & editing; Teferi Alem: Conceptualization, supervision, writing-review & editing; Dereje Ademe: Conceptualization, supervision, writing-review & editing; Enyew Adgo: Conceptualization, supervision, writing-review & editing.
Supplemental Material
Download MS Word (23.2 KB)Disclosure statement
No potential conflict of interest was reported by the author(s).
Additional information
Funding
References
- Abate, T., Shiferaw, B., Menkir, A., Wegary, D., Kebede, Y., Tesfaye, K., Kassie, M., Bogale, G., Tadesse, B., & Keno, T. (2015). Factors that transformed maize productivity in Ethiopia. Food Security, 7(5), 965–981. https://doi.org/10.1007/s12571-015-0488-z
- Addis, Y., & Abirdew, S. (2021). Smallholder farmers’ perception of climate change and adaptation strategy choices in Central Ethiopia. International Journal of Climate Change Strategies and Management, 13(4/5), 463–482. https://doi.org/10.1108/IJCCSM-09-2020-0096
- Addisu, S., Fissha, G., Gediff, B., & Asmelash, Y. (2016). Perception and adaptation models of climate change by the rural people of lake Tana sub-basin, Ethiopia. Environmental Systems Research, 5(1), 1–10. https://doi.org/10.1186/s40068-016-0059-0
- Ademe, D., Zaitchik, B. F., Tesfaye, K., Simane, B., Alemayehu, G., & Adgo, E. (2020). Climate trends and variability at adaptation scale: Patterns and perceptions in an agricultural region of the Ethiopian highlands. Weather and Climate Extremes, 29. https://doi.org/10.1016/j.wace.2020.100263
- Ademe, D., Zaitchik, B. F., Tesfaye, K., Simane, B., Alemayehu, G., & Adgo, E. (2021). Analysis of agriculturally relevant rainfall characteristics in a tropical highland region: An agroecosystem perspective. Agricultural and Forest Meteorology, 311(May), 108697. https://doi.org/10.1016/j.agrformet.2021.108697
- Alemayehu, A., & Bewket, W. (2016). Vulnerability of smallholder farmers’ to climate change and variability in the central highlands of Ethiopia Arragaw Alemayehu and Woldeamlak Bewket introduction. Ethiopian Journal of the Social Sciences and Humanities, 12, 1–24.
- Aman, M., & Dawid, J. (2020). Morphological response of Maize (Zea mays) to nitrogen fertilizer application rate at SNNP, Ethiopia. International Journal of Research Studies in Agricultural Sciences, 6(8), 10–14. https://doi.org/10.20431/2454-6224.0608002
- Asfaw, A., Simane, B., Hassen, A., & Bantider, A. (2018). Variability and time series trend analysis of rainfall and temperature in northcentral Ethiopia: A case study in Woleka sub-basin. Weather and Climate Extremes, 19(December 2017), 29–41. https://doi.org/10.1016/j.wace.2017.12.002
- Asfaw, S., McCarthy, N., Lipper, L., Arslan, A., & Cattaneo, A. (2016). What determines farmers’ adaptive capacity? Empirical evidence from Malawi. Food Security, 8(3), 643–664. https://doi.org/10.1007/s12571-016-0571-0
- Asrat, P., & Simane, B. (2018). Farmers’ perception of climate change and adaptation strategies in the Dabus. Ecological Processes, 7(7), 1–13.
- Bayable, G., Amare, G., Alemu, G., & Gashaw, T. (2021). Spatiotemporal variability and trends of rainfall and its association with Pacific Ocean Sea surface temperature in West Harerge Zone, Eastern Ethiopia. Environmental Systems Research, 10(1). https://doi.org/10.1186/s40068-020-00216-y
- Bedane, H. R., Beketie, K. T., Fantahun, E. E., & Feyisa, G. L. (2022). The impact of rainfall variability and crop production on vertisols in the central highlands of Ethiopia. Environmental Systems Research, 11. https://doi.org/10.1186/s40068-022-00275-3
- Belay, A., Demissie, T., Recha, J. W., Oludhe, C., Osano, P. M., Olaka, L. A., Solomon, D., & Berhane, Z. (2021). Analysis of climate variability and trends in Southern Ethiopia. Climate, 9(6), 96. https://doi.org/10.3390/cli9060096
- Belay, A. S., Fenta, A. A., Yenehun, A., Nigate, F., Tilahun, S. A., Moges, M. M., Dessie, M., Adgo, E., Nyssen, J., Chen, M., Van Griensven, A., & Walraevens, K. (2019). Evaluation and application of multi-source satellite rainfall product CHIRPS to assess spatio-temporal rainfall variability on data-sparse western margins of Ethiopian highlands. Remote Sensing, 11(22), 1–22. https://doi.org/10.3390/rs11222688
- Berhane, A., Hadgu, G., Worku, W., & Abrha, B. (2020). Trends in extreme temperature and rainfall indices in the semi-arid areas of Western Tigray, Ethiopia. Environmental Systems Research, 9(1), https://doi.org/10.1186/s40068-020-00165-6
- Birara, H., Pandey, R. P., & Mishra, S. K. (2018). Trend and variability analysis of rainfall and temperature in the tana basin region, Ethiopia. Journal of Water and Climate Change, 9(3), 555–569. https://doi.org/10.2166/wcc.2018.080
- Brown, M. E., Funk, C., Pedreros, D., Korecha, D., Lemma, M., Rowland, J., Williams, E., & Verdin, J. (2017). A climate trend analysis of Ethiopia: Examining subseasonal climate impacts on crops and pasture conditions. Climatic Change, 142(1–2), 169–182. https://doi.org/10.1007/s10584-017-1948-6
- Cabel, J. F., & Oelofse, M. (2012). An indicator framework for assessing agroecosystem resilience. Ecology and Society, 17(1). https://doi.org/10.5751/ES-04666-170118
- Dawit, M., Halefom, A., Teshome, A., Sisay, E., Shewayirga, B., & Dananto, M. (2019). Changes and variability of precipitation and temperature in the Guna Tana watershed, Upper Blue Nile Basin, Ethiopia. Modeling Earth Systems and Environment, 5(4), 1395–1404. https://doi.org/10.1007/s40808-019-00598-8
- Debela, N., Mohammed, C., Bridle, K., Corkrey, R., & McNeil, D. (2015). Perception of climate change and its impact by smallholders in pastoral/agropastoral systems of Borana, South Ethiopia. SpringerPlus, 4(1). https://doi.org/10.1186/s40064-015-1012-9
- De Luis, M., González-Hidalgo, J. C., Brunetti, M., & Longares, L. A. (2011). Precipitation concentration changes in Spain 1946–2005. Natural Hazards and Earth System Science, 11(5), 1259–1265. https://doi.org/10.5194/nhess-11-1259-2011
- Dinku, T., Block, P., Sharoff, J., Hailemariam, K., Osgood, D., del Corral, J., Cousin, R., & Thomson, M. C. (2014). Bridging critical gaps in climate services and applications in Africa. Earth Perspectives, 1(1), 15. https://doi.org/10.1186/2194-6434-1-15
- Eggen, M., Ozdogan, M., Zaitchik, B., Ademe, D., Foltz, J., & Simane, B. (2019). Vulnerability of sorghum production to extreme, sub-seasonal weather under climate change. Environmental Research Letters, 14(4), 045005. https://doi.org/10.1088/1748-9326/aafe19
- Etana, D., Snelder, D. J. R. M., & Van Wesenbeeck, C. F. A. (2020). Trends of Climate Change and Variability in Three Agro-Ecological Settings in Central Ethiopia : Contrasts of Meteorological Data and Farmers’ Perceptions.
- Gedefaw, M., Yan, D., Wang, H., Qin, T., Girma, A., Abiyu, A., & Batsuren, D. (2018). Innovative trend analysis of annual and seasonal rainfall variability in amhara regional state, Ethiopia. Atmosphere, 9(9), 326. https://doi.org/10.3390/atmos9090326
- Gorfu, D., & Ahmed, E. (2011). Crops and Agro-ecological Zones of Ethiopia. 47. http://www.google.dk/url?sa=t&rct=j&q=&esrc=s&source=web&cd=1&ved=0CDQQFjAA&url=http://prrp-ethiopia.org/index.php/component/phocadownload/category/1-public-documents?download=51:crops-and-agro-ecological-zones-total-dereje-gorfu&ei=X91jUbLDFYH27AbBtYGgCg
- Habte, A., Mamo, G., Worku, W., Ayalew, D., & Gayler, S. (2021). Spatial variability and temporal trends of climate change in southwest Ethiopia: Association with farmers’ perception and Their adaptation strategies. Advances in Meteorology, 2021, 1–13. https://doi.org/10.1155/2021/3863530
- Modarres, R., & de Paulo Rodrigues da Silva, V. (2007). Rainfall trends in arid and semi-arid regions of Iran. Journal of Arid Environments, 70(2), 344–355. https://doi.org/10.1016/j.jaridenv.2006.12.024
- Shiferaw, B., Prasanna, B. M., Hellin, J., & Bänziger, M. (2011). Crops that feed the world 6. Past successes and future challenges to the role played by maize in global food security. Food Security, 3(3), 307–327. https://doi.org/10.1007/s12571-011-0140-5
- Simane, B., Zaitchik, B. F., & Foltz, J. D. (2016). Agroecosystem specific climate vulnerability analysis: Application of the livelihood vulnerability index to a tropical highland region. Mitigation and Adaptation Strategies for Global Change, 21(1), 39–65. https://doi.org/10.1007/s11027-014-9568-1
- Taye, M., Simane, B., Zaitchik, B. F., Selassie, Y. G., & Setegn, S. (2019). Rainfall variability across the agro-climatic zones of a tropical highland: The case of the jema watershed, northwestern Ethiopia. Environments – MDPI, 6(11), 118. https://doi.org/10.3390/environments6110118
- Teshome, H., Tesfaye, K., Dechassa, N., Tana, T., & Huber, M. (2021). Smallholder farmers’ perceptions of climate change and adaptation practices for maize production in eastern Ethiopia. Sustainability (Switzerland), 13(17), 9622. https://doi.org/10.3390/su13179622
- Tessema, I., & Simane, B. (2019). Vulnerability analysis of smallholder farmers to climate variability and change: An agro-ecological system-based approach in the Fincha’a sub-basin of the upper blue Nile basin of Ethiopia. Ecological Processes, 8(1). https://doi.org/10.1186/s13717-019-0159-7
- Tilahun, K. (2006). Analysis of rainfall climate and evapo-transpiration in arid and semi-arid regions of Ethiopia using data over the last half a century. Journal of Arid Environments, 64(3), 474–487. https://doi.org/10.1016/j.jaridenv.2005.06.013
- Tirfi, A. G., & Oyekale, A. S. (2022). Analysis of trends and variability of climatic parameters in Teff growing belts of Ethiopia. Open Agriculture, 7(1), 541–553. https://doi.org/10.1515/opag-2022-0113
- Wakjira, M. T., Peleg, N., Anghileri, D., Molnar, D., Alamirew, T., Six, J., & Molnar, P. (2021). Rainfall seasonality and timing: Implications for cereal crop production in Ethiopia. Agricultural and Forest Meteorology, 310, 108633. https://doi.org/10.1016/j.agrformet.2021.108633
- Yarnell, A. (2008). Feeding Africa. Chemical and Engineering News, 86(4), 74. https://doi.org/10.1021/cen-v086n004.p074