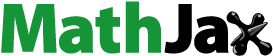
ABSTRACT
In the context of global warming and the increasing water shortage in dry areas, it is necessary to find a sustainable pathway for utilization of land resources (ULR) to solve these problems. For this purpose, using socioeconomic and environmental indicators from 2006 to 2018 and taking the Tarim Basin, China as an example, this study employed an integrated approach composed of the indicator evaluation system (IES) and the System Dynamics (SD) model to evaluate the possibility of sustainable ULR in the basin. During the simulation, three scenarios namely traditional development (TD), economic priority (EP) and harmonious development (HD) were designed, and different thresholds were set for each indicator of potential ULR in each sub-region to predict their future development status. The IES was established to derive the sustainable utilization degree (SUD) of ULR. The results show that the SUD in Tarim in 2030 is likely be 0.60 (TD), 0.62 (EP) and 0.68 (HD), indicating that only in HD scenario is it possible to find pathway to solve water scarcity problem and achieve sustainable ULR. This study provides a supportive idea for decision-making on sustainable ULR in Tarim and also an approach for similar sustainability assessment in other dry areas.
1. Introduction
Environmental resources, especially, land and water resources, are essential for human society as they support directly human life, food production and energy provision of a community, a region or a country (Holland et al., Citation2015; Zhang et al., Citation2018). As a natural endowment, land and water resources are fundamental and strategic for the region or the country (Amiri et al., Citation2014; Han et al., Citation2015; Rulli et al., Citation2013) and may determine the pattern of its agricultural and industrial development (Abdul et al., Citation2002; Cuceloglu et al., Citation2017; Jiang et al., Citation2018; Wang, Citation2018). Nevertheless, this may raise an important issue that how we can sustainably use land and water resources and make them to serve better our socioeconomic development while taking ecological protection into account (Chen et al., Citation2018).
Since the emergence of the theory of location (Weber, Citation1982), it has been regarded as the most primitive theoretical basis for land use planning. With the development of society, the sustainable utilization of land resources (ULR) has now received extensive attention as an important component of regional sustainable development (Lv, Citation2014). Taking grassland resources as the limiting factor, Janssen et al. (Citation2000) discussed the impact of different policies on grazing and grassland ecosystems and, on this basis, proposed an optimal management model for grazing grasslands in Australia; and Wu et al. (Citation2002), Wu (Citation2003), Wu and Zhang (Citation2003) and Wu et al. (Citation2013) established socioeconomic models of land use and land use change and discovered the positive and negative impacts of land management policies on croplands in Ningxia and rangeland in Ordos, China. To assess land use management in Santiago, Chile, Weiland et al. (Citation2011) developed an assessment index on land use change and urban expansion. In their interdisciplinary analysis of rapid land use change, Jose and Padmanabhan (Citation2016) revealed that sustainable land use is influenced by a combination of institutional and socio-demographic factors. They proposed that ecological and socioeconomic policies should be implemented to ensure sustainable land use. In assessing land use change and its drivers in Burkina Faso from 1983 to 2014, Bazame et al. (Citation2019) used multiple linear regression analysis and found that the failure of the policy system may have a significant impact on land resource change. By analysing the sustainable use potential of agricultural land in the Kazbegi region of the central Greater Caucasus, Theissen et al. (Citation2019) found that both the eco-environment and economic income have improved in the mountainous areas since the implementation of land use planning. From the perspectives of social, economic and ecological sustainability, Lan (Citation2020) constructed a sustainable land use evaluation system in Wuhan, China; combined with the sustainable land use evaluation, the results show that the level of sustainable land use in Wuhan is spatially heterogeneous. To reverse land degradation and promote sustainable intensification of smallholder agriculture, Nziguheba et al. (Citation2022) developed a framework of indicators to assess sustainable use while Mohamed et al. (Citation2014) used the Special Model for Sustainable Agriculture (SASM) to assess the impact of five factors (productivity, safety, conservation, economic viability and social acceptability) on the sustainable use of agricultural resources in the North Sinai region. To assess the most suitable scenario for soil and water use in Latvia, Sušnik et al. (Citation2021) simulated the area using a system dynamic (SD) model and made recommendations for future development.
As far as water resources are concerned, the ‘International Conference on Water and Environment – Development and Prospects for the Twenty-First Century’ (ICWE), held in Ireland in 1992, raised the issues of water resource systems and sustainability (Bruce, Citation1992; Flint, Citation2004). The principles of sustainability and equity were incorporated into the long-term planning and management of water resources by Gleick (Citation1996), which provides a new approach to the construction of sustainability indicators. Based on the review of international initiatives for sustainable development, Walmsley et al. (Citation2001) proposed a set of sustainability indicators for catchments in South Africa. Using the Water Act and consumer use permit requirements as constraints in the water resources assessment model, Ahmad and Prashar (Citation2010) assessed the potential impacts of different policies on water management to reduce water demand in cities of South Florida to meet the environmental flow demands. Taking the interaction between economic and social dimensions into consideration, Qi and Chang (Citation2011) applied the SD model to estimate and predict future water demand under the influence of changes in the macroeconomic environment in Florida. Considering the various changes in water resources in the future under different scenarios, Sun et al. (Citation2017) designed different development programmes with the SD model to simulate water resource situations and future changes from 2005 to 2020 in China and found that the harmonious development (HD) scheme can realize the sustainable development of society and the sustainable utilization of water resources. In combination of Matlab with the Water Evaluation and Planning System (WEAP), Tsanov et al. (Citation2020) found the best strategy to alleviate the pressure on water resources in the Vit River Basin, Bulgaria. In simulating water use in Texas using SD models from a view of the impact of climate change on water change, Rueda et al. (Citation2022) found that an increasingly warm and drier climate in the future threatens the sustainability and resilience of water systems. Garcia and Alamanos (Citation2022) simulated three common agricultural problems through optimization techniques and provided relevant adjustment countermeasures in different sites in Greece, Canada and Ireland.
In addition, some authors used land resources (or water resources) as constraints to analyse the related water resources (or land resources), and here are some examples. After a systematic study of the overall impact of land cover change on water resources in South Africa, Nkosi et al. (Citation2021) found that land management has a detrimental effect on the quality and quantity of water resources. Moltz et al. (Citation2020) proposed an approach for assessing water use efficiency that integrates sustainable water resource management with local land use planning decisions in the Potomac Watershed, USA. To accurately model future land use in the Gaborone Dam Basin in Botswana, Matlhodi et al. (Citation2021) proposed a combination of cellular automata with the Markov model that is able to reveal future land use change and impacts on water resources.
Based on the SD model, Zhang (Citation2018b) and Zhang (Citation2018a) recently analysed the water resources and urbanization in Xinjiang and found that the expanding population and economic development will expand the demand for water resources in the region, especially, in the Tarim Basin, where the total amount of water resources is limited and the problem of water scarcity will become more serious in the future. In order to reveal the changes in water consumption on a long-term scale in the future, Fan et al. (Citation2020) simulated water consumption in Xinjiang from 2020 to 2050 under different scenarios and found that reducing irrigation water use is the key to overcoming water scarcity. However, due to the influence of geographical conditions, climate and local policies, the Tarim Basin has encountered problems such as irrational allocation of water and land resources with unbalanced supply and demand due to accelerated industrialization, oil exploitation, urbanization and irrigated land extension since the1990s. These problems constitute an important factor limiting the sustainable socioeconomic development in the Tarim Basin. It is, hence, of great importance to conduct an integrated modelling, taking all the elements of land and water resources in the basin into account to find solutions and pathways of sustainable development.
From the above brief review, we can find that the existing studies were focused on either land use and land resource modelling or simulation of water resources, and none of them dealt with sustainable use of both water and land resources. Moreover, the existing research focused on Xinjiang neither analysed water resources at the sub-region level, nor tackled both water and land resources as an integration, nor took the inequality of development among the sub-regions into account. This poses a difficulty to apply their results to guide the utilization of land and water resources at the local or sub-region level in Tarim. Furthermore, the existing approaches for assessing the sustainability issue are mainly knowledge-based empirical analysis (Janssen et al., Citation2000; Theissen et al., Citation2019; Weiland et al., Citation2011), evaluation using a number of indicators (Gleick, Citation1996; Lan, Citation2020; Walmsley et al., Citation2001) and system analysis including SD (Mohamed et al., Citation2014; Nkosi et al., Citation2021; Rueda et al., Citation2022; Sušnik et al., Citation2021). In contrast, few studies have been focused on an integrated systematic approach, e.g. a combination of two or three of the above ones for a systematic, comprehensive and objective analysis of sustainable ULR. These defects constitute the research gap of this paper, that is, to employ an integrated systematic approach using sub-region level data to conduct a comprehensive simulation on both land and water resources, taking the Tarim Basin in Xinjiang, China, as an example. The objectives of this study are to find the sustainability thresholds for harnessing land and water resources at the sub-region level in the study area through simulation of supply–demand relationship and socioeconomic development. The modelling results of this study are expected to provide a reference for decision-making on sustainable utilization of water and land resources (SUWLR) in Tarim, and the proposed approach will be extended to other arid and hyper-arid regions in the world for similar simulation purposes.
2. Materials and methods
2.1. Study area
The Tarim Basin, located in Xinjiang, is the largest inland basin of China (Chen, Citation1999; Jiang et al., Citation2022; Li et al., Citation2003). The basin consists of five sub-regions: Bayin Goleng Mongol Autonomous Prefecture (Bazhou), Kizilsu Kirgiz Autonomous Prefecture (Kezhou), Aksu, Kashgar and Hotan Prefectures.
The basin with an altitude of 800–1300 m lies between the Tianshan Mountains in the north, and Kunlun and Altun Mountains in the south (Jiang et al., Citation2022). The terrain is high in the west and low in the east. These mountains define a rhombus-shaped tectonic basin, the Tarim Basin, covering 73% of the total area of Xinjiang, approximately 1.2 × 106 km2 (Li et al., Citation2003). The landform of the basin looks like a ring shape where the margin is the gravel Gobi connected with the mountains, and the central parts are a vast desert. The marginal belts are actually pluvial fans from mountains and alluvial plains and oases along the Tarim River and its tributaries ().
The study area is situated in a warm temperate climate zone but with high aridity and frequent wind blowing, causing dust and sandstorms in spring and winter, constituting the main natural disasters in the basin. The average annual temperature is 9–11°C, slightly higher in the south than in the north. It is colder in the east than in the west in winter. Precipitation in the basin is sparse, and the average annual rainfall is about 106 mm, which cannot form runoff by itself, but the annual rainfall in the surrounding mountainous areas reaches 200–400 mm, which can converge into rivers flowing into the basin. Aksu River, Hotan River and Yarkand River are the main tributaries of the Tarim River, the longest inland river in China, supplying water resources for maintaining the ecological health of the rangelands and forests and keeping croplands in oases productive along the river in the basin (Jiang et al., Citation2022; Li et al., Citation2017).
2.2. Data and preprocessing
Land use data at 300-m spatial resolution from 2006 to 2018 were obtained from the European Space Agency (ESA: http://maps.elie.ucl.ac.be/CCI/viewer/index.php). Within the GIS interface (ArcMap 10.2), reprojection, clipping and resampling of these data were conducted, and the original 27 types of land use and land cover were merged into six major classes, namely, croplands, rangelands, forests, waters, urban and unused lands.
The socioeconomic data came from the Xinjiang Statistical Yearbooks and Xinjiang Water Resources Bulletins from 2006 to 2018 were the only available data we could find. These data include population, primary, secondary and tertiary industry products, outputs of the agriculture, forestry, animal husbandry and fishery, demand for food and meat production, total water consumption, in particular, consumption in industry, agricultural irrigation, life and ecological protection, e.g. afforestation.
Daily temperature data from 38 weather stations for the period 2006–2018 were obtained from the Data Centre of Resources and Environmental Sciences, Chinese Academy of Sciences.
Field data were acquired in August 2000 and May 2021 during the two field surveys conducted by our team in the Tarim Basin, and the main observation included land use and cover pattern, distribution of water and land resources, hydraulic engineering, urbanization and economic development.
The above data were used to construct the indicator evaluation system (IES) and to determine the parameters of the SD model.
2.3. Methods
As planned, instead of using a single evaluation approach, the research was to be conducted in an integrated systematic analysis composed of both indicator system (IS) and SD approaches, where the first is to establish an IS for evaluation of SUWLR covering three subsystems: ecological environment, resources and socioeconomic aspects, and the second is to build the SD model on the grounds of the IS. Furthermore, because of the significant gap between the development status and resource utilization of various states in the basin, the study was to be conducted at the sub-region level for analysis within three different scenarios, i.e. the traditional development (TD), economic priority (EP) and HD. Thus, the simulation results would allow us to obtain a comprehensive understanding of resource utilization in the basin and its sub-regions. Combined with the weight of each indicator in the course of the evaluation, we calculated the indicator scores and finally obtained the sustainable utilization degree (SUD) of the resources in the basin.
2.3.1. Evaluation based on IES
(1) The establishment of a sound IS will contribute to the assessment of the SUWLR (Yan et al., Citation2011), and it must conform to four principles: (1) on a scientific basis, the connotation of the indicator ought to be clear, which can reflect the connotation of SUWLR; (2) operational, i.e. the selection of the IS must be as simple and transparent as possible, and the quantification of the corresponding indicators and data acquisition should be easy, accurate and reliable, and the existing data and norms should be used as many as possible; (3) systematic, meaning that resource utilization is an integrated socioeconomic behaviour, and thus, evaluation indicators selected from a systematic point of view should be able to reflect all aspects of resource use and (4) dynamic, as sustainability is a development process metric, and the selected evaluation indicators may change with time but not static (Yin, Citation2001). In order to select indicators more accurately, we carried out a 2-week field survey in the Tarim Basin and inspected the local socioeconomy, water and land use. In combination with the data we obtained, we selected 16 indicators to reflect the utilization status of water and land in the basin including nine positive indicators, of which the more extensive the indicator value, the better and seven negative indicators (the smaller the indicator value, the better, see ). These indicators were classified into socioeconomic, resources and ecological environment subsystems ().
Table 1. Definition of the indicators.
Table 2. Final weights of the indicators in each subsystem.
The evaluation process based on multiple indicators consists of three parts: establishment of an IES, weight determination of indicators, and selection of an evaluation method. Among them, the weight determination of the evaluation indicators is an integral part of the evaluation process. In this paper, the entropy approach and analytic hierarchy process (AHP) were harnessed for calculating the weights of indicators.
2.3.1.1. Entropy approach
The weight is mainly determined according to the content delivered to each indicator by the user (Dai et al., Citation2016). If the value of an indicator has a significant difference, and the entropy value is small or, rather, a strong dispersion, we know that the indicator provides much helpful information, and its weight will also be substantial (Zou et al., Citation2005); otherwise, the weight of the indicator shall be small (Ren et al., Citation2021). The full calculation process of the entropy approach is shown as follows (Amiri et al., Citation2014; Han et al., Citation2015).
Before calculating the comprehensive index, the indicators as listed in should be standardized, that is, by converting the absolute value of the indicator into a relative value to solve the problem of homogeneity of different indicator values.
Positive indicator processing:
(1)
(1) Negative indicator processing:
(2)
(2) where Xij* represents the standardized value of the jth indicator of the ith sample, and max Xj and min Xj are the maximum and minimum values of the original data of the jth item.
The following procedure is to calculate the entropy value ej of each indicator with formula (3):
(3)
(3) where k > 0, ej ≥ 0,
, normalization,
Indicator weight calculation:
(4)
(4) where dj = 1-ej and Wj is the weight of the observed indicator.
2.3.1.2. AHP
AHP or simply named hierarchical analysis is a subjective and authoritative integrated decision-making approach proposed by T. L. Saaty in the 1970s. In order to facilitate the analysis, the water resources for sustainable development are classified and assigned weights for each indicator based on the previous work. The AHP consists of three main parts: the first one is to build the filiation models between the target layer, intermediate layer and bottom layer; the second is to determine the corresponding judgement matrix for easy calculation and the last one is to test the weight and randomness consistency of each factor.
The judgment matrix is normalized by column to find the weights:
(5)
(5)
(6)
(6) Here, the maximum characteristic root λmax of the judgment matrix can be calculated by
(7)
(7) where AWi denotes the ith element of vector AW.
Consistency check:
(8)
(8)
(9)
(9) where CI is the consistency index, and the smaller the value, the lower the degree of inconsistency; λmax is the maximum eigenvalue of the judgment matrix, and n is the order; RI is the random consistency index (when the index is 16, RI = 1.5943), and CR means the consistency ratio and when CR < 0.1, it denotes that the judgment matrix is consistent. The indicators listed in all passed the consistency test.
The final weights were determined using the average of the entropy values and the hierarchical analysis ().
2.3.2. Calculation of SUD
Earlier studies were largely focused on qualitative analysis (Yang et al., Citation2010), and this study makes an effort to conduct a quantitative analysis of SUD. After standardizing each evaluation indicator, the values of all indicators were weighted and summed to obtain the SUD of land and water resources in the Tarim Basin with formula (10):
(10)
(10) where t is year, SUD is the sustainable utilization degree in year t, w is the final weight of the evaluation indicators of each subsystem, x is the standardized value of the evaluation indicators, and i is the number of indicators. In combination with the results of the previous research, we classified SUD into five levels for the Tarim Basin ().
Table 3. Sustainable utilization degree (SUD).
2.3.3. SD model
The SD model starts from the system’s internal composition and uses the first-order differential equation to reflect the causal relationship among the variables in the system. It can simulate the time-varying behaviour of the nonlinear real-world complex systems and perform dynamic surveillance while taking into account the interactions between various variables within the system (Winz et al., Citation2009). In addition, it is able to handle chronic and cyclical problems for efficient analysis of complex systems (Davies & Simonovic, Citation2011). In this study, we used regression analysis within SPSS (Statistical Product Service Solutions), a package for statistics and modelling, through the collection of government-reported socioeconomic data and land use data to obtain the relationships between different land use types and their drivers. Ordinary first-order dynamic equations express the relationships among the variables. The system boundary of the model is the Tarim Basin including five sub-regions, e.g. Bazhou, Kezhou, Aksu, Kashgar and Hotan Prefectures, and the time span is from 2006 to 2030, in which 2006–2018 is the base period for which socioeconomic data were available and 2019–2030 for simulation with 1 year as a time step. The model is divided into four modules: resource utilization, population, economy and climate ().
3. Results and discussion
3.1. Land use changes and water consumption in the Tarim Basin
Our analysis revealed that from 2006 to 2018, the utilization rate of land resources increased from 30% to 31.3%, and croplands increased from 53,629 km2 to 58,183 km2 in the basin. As the government has been paying more attention to ecological protection in recent decades, the forests in Tarim have increased from 38,767 km2 to 41,428 km2, most of which is converted from unused lands thanks to afforestation activities, while rangeland, water-bodies and urban areas have not experienced a significant change (). The irrigated areas have gained an increase from 14,893 km2 to 26,568 km2 and were mostly concentrated in Kashgar and Aksu from 6222 km2 to 10,037 km2 and from 4078 km2 to 9100 km2, respectively (). Extensive irrigation has already caused a water demand exceeding the total amount of available water resources and, thus, turned these two sub-regions into a water-deficient state. In 2018, the water deficiency in Kashgar and Aksu reached 2.9 × 109 m3 and 5.2 × 109 m3, respectively (). The irrigated areas in Bazhou, Kezhou and Hotan are 4200, 2810 and 2421 km2, respectively, and the pressure on water supply and demand in these three prefectures was thus relatively low (). As seen in , per capita water consumption generally showed a downward trend with the development of socioeconomy except for Kezhou, and the water consumption per 10,000 yuan GDP in each sub-region also showed a similar trend.
Figure 3. Land use changes from 2006 to 2018: (a) croplands, (b) forests, (c) irrigated area and (d) rangelands.

Figure 4. (a) Supply and demand of water resources in each sub-region in 2018 and (b) total water consumption and total water resources in the basin from 2006 to 2018.

Table 4. Per capita water consumption (PCWC) and water consumption per 10,000 yuan GDP (WCPG) in each sub-region.
In terms of the water consumption of the primary, secondary and tertiary industries, there was a big difference among the sub-regions but the water consumption of the primary industry was dominated by agriculture-related activities, in particular, irrigation. Furthermore, the water consumption of the primary industry in the other four sub-regions all showed varying degrees of increase except for Kashgar from 2006 to 2018 (), which resulted in a large increase in the total water consumption of the basin. Overall, water stress in the basin increased from 57% to 73% in the period of 2006–2018, which enhanced water scarcity. The unbalance of water resources' supply and demand is a severe challenge in the Tarim Basin.
Table 5. Water consumption in primary, secondary and tertiary industries in each sub-region.
shows the domestic and eco-environmental water consumption of each sub-region. The total amount of domestic water consumption in 2018 increased significantly compared with that in 2006. Kashgar had the largest total amount of residential water consumption, 1.980 × 109 m3, due to its large population. However, domestic water consumption of each sub-region accounted for a relatively small proportion. The total area of eco-environmental water consumption had an evident decline compared with that of 2006 ().
Table 6. Domestic and eco-environmental water consumption in each sub-region.
The socioeconomic development is also not ideal, and the urbanization rate has only increased from 26% to 28% and there are considerable differences in urbanization rates among the different sub-regions. The urbanization rate of Bazhou in 2018 was 54%, reaching the average level in Xinjiang, whereas that in Aksu, Kezhou, Kashgar and Hotan was 33%, 23%, 22% and 22%, respectively, which were lower than the average.
It is seen that the basin is currently facing three problems: (1) with the expanding use of land resources, especially, expansion of the irrigated area and industry development, the total water resources are unlikely to meet the demand for water resources, and the mismatch between water and land resources is becoming more and more serious; (2) the socioeconomic development is slow, and the economic conditions and urbanization rates vary significantly among sub-regions or prefectures and (3) as a hyper-arid area, rainfall is very limited. Therefore, in the simulation of this study, we designed three scenarios, i.e. TD, EP and HD to predict the utilization of land and water resources with reference to the objectives of the ‘14th Five-Year Plan’ of Xinjiang (Zhang, Citation2018a).
3.2. Reliability of simulation
To ensure the pertinence of the simulation, it is necessary to debug the model and verify its reliability using historical data. In general, we will consider the model reliable when the relative deviations between the simulated and the real values are less than 10% (Forrester, Citation1971).
The historical data used for verification of the SD model in this study were those from 2015 to 2018. Due to the large amount of data, we took population, GDP, croplands and forests as examples for this purpose. Verification of the simulated results against the real values revealed the low difference/error, 2.13%, or, rather, high reliability of our models ().
Table 7. Reliability of simulation.
3.3. Simulation of SUWLR within different scenarios
3.3.1. Scenario 1: TD
The TD scenario simulates the existing development situation without artificial interference under reference conditions. The irrigation water quota, per capita domestic water consumption, per capita livestock, food demand, ecological water consumption, total water resources and other factors remain unchanged in the water module. Population growth rate, urbanization growth rate, primary, secondary and tertiary industry growth rates and irrigation growth rate are the averages of the past 4 years (). The climate variables are referred to in the Assessment Report of the International Panel on Climate Change (IPCC, Citation2013), that is, the average annual increase in temperature and rainfall is 0.075°C and 6.4 mm in the Tarim Basin.
Table 8. Thresholds of decision variables within Scenario 1 for different prefectures.
Within the TD scenario, the SUD is likely to be 0.6 in 2030 (), just reaching a good state. Regarding land resources, their utilization rate will increase and reach 33.5%, among which croplands, forests and irrigated areas will augment to 64,054, 42,272 and 28,547 km2, respectively, in 2030. As for water resources, the consumption will become more and more serious, mainly due to irrigation. The irrigation water in Bazhou, Aksu, Kezhou, Kashgar and Hotan is likely to reach 3.44, 7.98, 0.60, 8.30 and 2.08 × 109 m3, respectively, in 2030, taking possession of 57% of the water consumption in the entire basin. The overall water stress level will reach 0.84. In this case, the total water consumption of Kashgar and Aksu will not be guaranteed, and the water shortage in the two sub-regions will amount to 11.9 × 109 m3 and 2.7 × 109 m3, respectively. The contradiction between the supply and demand of water resources will further be enlarged in the future; and in the field of socioeconomy, the urbanization rate will be increased from 28% in 2006 to 34% in 2030, where it may reach 67% in Bazhou, the fastest growing prefecture in the Tarim Basin. However, the urbanization rate of Kezhou and Kashgar appears to be low, only 25%. The overall economic development may be in a slow state. The outputs of the primary, secondary and tertiary industries will be increased by 2 times, 2.5 times and 3 times, respectively, in comparison with those of 2018. In this TD scenario, the utilization rate of land resources will increase with agricultural and economic development, and correspondingly, the water stress level will also increase, especially, in the two sub-regions Kashgar and Aksu. The water shortage problem is likely to become more serious than before. Therefore, the development under TD may seriously violate the local policy related to land management.
3.3.2. Scenario 2: EP
Economic development will bring a series of impacts on population, water consumption and land use changes (Coale & Hoover, Citation2015), which indirectly affect the SUWLR. Given this, we designed the second scenario, i.e. the EP scenario, where rapid economic development would require the consumption of land and water resources but also the involvement of talents and labour force. The population growth rate will be forced to increase as much as possible to promote economic development, and it can be 1.25 times that of Scenario 1 and the growth rate of the primary, secondary and tertiary industries may reach 2 times that of Scenario 1. Meanwhile, the urbanization rate and the growth rate of the irrigated land tend to be 1.2 times and 1.25 times those of Scenario 1, respectively, to meet the economic development. Climate change, at least at the local level, will be accelerated due to the rapid development in industries, in which the increases in the annual mean temperature and rainfall are 0.1°C and 7.2 mm, respectively, given the same other parameters as in the TD scenario.
The simulation results show that rapid economic development will lead to an increasing demand for land resources and their utilization rate in the basin. Croplands, forests and irrigated areas will reach 72,381, 45,410 and 29,276 km2, respectively, in 2030. At the same time, economic development may provoke a rapid increase in urbanization rate, reaching 42% in 2030 and, in particular, 87% in Bazhou, whereas it may be only 30% in Kashgar and Aksu. Extension in land use, especially, in irrigated areas, will definitely cause a rise in water demand, and the total consumption of water resources in 2030 can even amount to 41.1 × 109 m3, of which 22.9 × 109 m3 will be dedicated to irrigation. Hence, the contradiction of water supply and demand will become more prominent, and the overall water stress level may attain 0.97. The SUD of water and land resources in 2030 is likely to be 0.62 (), gaining an increase compared with that of the TD scenario. If this development pattern continues, Tarim will move into a state of extreme water shortage in the future. The water and land resources will be highly mismatched, and the limited water resources will not be able to meet the needs of human life, economic development and agricultural irrigation. According to the local policies, it is necessary to ensure a healthy environment while economic development takes place and to realize a win–win situation between economic development and ecological protection. Therefore, we think that the EP scenario disrupts the balance of resources and will not be capable of meeting the requirement of sustainable development.
3.3.3. Scenario 3: HD
To sort out a series of contradictory problems caused by rapid economic development and consumption of resources, we have designed the third scenario, i.e. the HD scenario. Under this scenario, ensuring both the use of land resources and economic development in a harmonious way requires first of all an improvement in water use efficiency. In the process of simulation under this scenario, the growth rate of irrigated land and water consumption in the secondary and tertiary industries were adjusted to 0.8 times that in the traditional TD scenario, and the per capita domestic water consumption in urban and rural areas to 70L/person-day and 50L/person-day in 2030. The environmental conditions in Xinjiang are similar to those in Israel, and thus, we may refer to their experience (Wang et al., Citation2018; Zhang, Citation2018a). In terms of economic development, the growth rate of the three major industries has increased by 1.8 times compared with that in Scenario 1. As there is an emphasis on ecological and environmental protection, the increase in annual mean temperature and rainfall are likely to be 0.05°C and 6.4 mm, respectively, given the same conditions for other variables as in Scenario 1.
The simulation results of this scenario demonstrate that the water demand in Tarim is likely to be 21.7 × 109 m3 in 2030, which is 43% lower than the TD scenario, and the water stress level is also reduced to 0.54, implying that the total water resources in Tarim can meet the demand. This shall be conducive to overcoming the issue of the current water shortage in the basin. However, spatial variability is observed, that is, there will be a water shortage of 3.3 × 109 m3 in Aksu while the water availability can meet the demand in Kashgar. We believe that these two prefectures can achieve SUWLR by maintaining this development scenario in the future. Croplands, forests and irrigated areas will increase to 70,477, 44,819 and 26,916 km2, respectively in 2030. As we have lowered the quota of irrigation water, there will be no mismatch issue between water and land resources. With respect to social and economic development, the overall urbanization rate will reach 38% in 2030, where the maximum tends to be 77% in Bazhou and the minimum 27% in both Kashgar and Aksu. It is noted that there will be still a difference in the urbanization rate among the different sub-regions.
The increments of the primary, secondary and tertiary industries will amount to three, five and six times, respectively, in comparison with the outputs of 2018. Since we have reduced the water consumption per unit output of the secondary and tertiary industries, the rapid progress of the industry will not increase the supply pressure of water resources. The SUD in the Tarim Basin will reach 0.68 in 2030 (), 8% higher than that of Scenario 1. Hence, we consider it appropriate to improve water use efficiency through human intervention and rational policy implementation. An ecologically healthy economic development while reducing population pressure shall be one of the pathways to keep the socio-environmental system in balance and ensure SUWLR in Tarim. Therefore, the HD scenario seems to be the best one for socioeconomic development in the study area.
3.4. Perspective on sustainability
The Tarim Basin has unique geographical conditions with sufficient land resources but at a low utilization rate. From the above simulation results (), we understand that the SUWLR is unlikely to be achieved in both TD and EP scenarios because of the following reasons:
(1) | In the case of the TD scenario, the consumption of water resources due to the increase in irrigation area and urbanization rate will have a significant increase and so will the overall pressure level of water resources. Nevertheless, the overall socioeconomic development is relatively slow, and the SUD is only 0.6. Based on the comprehensive prediction results and evaluation thresholds, it is noted that the sustainable utilization of resources cannot be realized in this scenario. |
Samie et al. (Citation2017) showed similar results in the dynamic scenario simulation of land use change in Punjab, Pakistan. They predicted land use changes in Punjab from 2010 to 2030 and found that under the regular operation, i.e. business as usual (BAU) scenario, except for cultivated land and built-up area, other land use types will have a decrease in 2030, and the overall land utilization rate will also decrease. In addition, they all have a common problem: an increase in arable land but a lack of sufficient water resources at the same time will lead to an increasingly severe mismatch of water and land resources in the future. This is quite similar to our results in Scenario 1.
(2) | The rapid socioeconomic development in the EP scenario will take place at the cost of consumption of land and water resources, in which the pressure level of water resources might reach 0.97 and the sustainability of water and land use would be 0.62. Although the result seems better than that of the TD scenario, the water resources have been in an excessive consumption state. Thus, it is unlikely to achieve a SUWLR. |
Dawadi and Ahmad (Citation2013) surveyed the effects of population increase and climate change on water resources in the Las Vegas Valley (LVV). They evaluated changes in water resources under different population growth and demand management and found that, based on expected population growth, without corresponding management policies, LVV residents will not have enough water. However, by formulating corresponding water-saving policies, the water demand will be reduced by 30% in 2035, which can, thus, solve the problem of water scarcity.
(3) | The HD scenario considers economic development, land use expansion and water resources conservation in a harmonious way. For water-scarce regions, improvement in irrigation technology and reduction in irrigation water consumption have become increasingly important to SUWLR (McDonald et al., Citation2014). In particular, certain successful experiences and technologies developed by other countries or communities in dry areas, e.g. Israel, can be referred to because the country has dramatically alleviated the severe shortage of water resources in agricultural production through its rational water resource utilization and management system supported with the advanced and efficient agrarian water conservancy and irrigation technology. For this reason, a series of water conservation measures should be taken in Tarim as well. As mentioned earlier, the cultivated land, forests and irrigated areas together with urban areas are likely to increase in 2030, and the land utilization rate is going to be between the TD and EP scenarios. Due to the adoption of water-saving measures (Zhang, Citation2018a), the total consumption of water resources in 2030 will be 21.7 × 109 m3, and the sustainability of water and land use will be 0.68. The comprehensive prediction and evaluation show that it is likely to realize the sustainable utilization of resources in this scenario. |
Strengthening the construction of water conservancy facilities is also very important for the SUWLR. Kotir et al. (Citation2016) established an SD simulation model for sustainable water resources management and agricultural development for the Volta River Basin in Ghana and found that the development in the water infrastructure scenario seems to offer the most significant benefit to local people by increasing crop yields and net farm income and the development of water infrastructure. This can be referred to as well in sustainable development planning and management in Tarim.
4. Conclusions
With the purpose of achieving a reliable simulation of the SUD of land and water resources, an integrated hybrid approach composed of the IES and SD modelling was proposed and experimented with the Tarim Basin with its five sub-regions. The predicted development within Scenario 3 (HD) seems sustainable in the utilization of land and water resources, better than the other two. However, no matter which scenario is considered, Kashgar and Aksu are most likely to be in shortage of water resources even if it is in a better situation in the third HD scenario. The irrigated areas in Kashgar and Aksu are much larger than those in the other three sub-regions. As an agricultural economic zone cultivated with various crops, e.g. maize and cotton, the reduction of irrigation water is an assurance for the SUWLR in Tarim. Hence, technological innovation and water-saving irrigation practices may improve water use efficiency and increase the yield per unit area, and this appears essential and plausible. The thresholds produced and results obtained may provide relevant advice for local governments to make sustainable land use and land management policies in the region.
This study made a new effort in the simulation and realization of SUWLR in a dry region, Tarim. Due to the complexity and uncertainty of environmental resources, many issues deserve further exploration, e.g. mineral resources and geological settings. Actually, mineral ores including oil, gas and metal and non-metal ores are part of the environmental resources, and they shall be incorporated into the IES and then into SD modelling. For the time being, weather parameters involved in this study are rainfall and temperature, and in further work, evaporation and sunshine duration shall be integrated as well for complete modelling. At last, the integrated approach can be extended to other areas for similar sustainability modelling in dry areas in the world.
Disclosure statement
No potential conflict of interest was reported by the author(s).
Data availability statement
The datasets used and/or analysed during the current study are available from the corresponding author upon reasonable request.
Additional information
Funding
References
- Abdul, G., Manzoor, G., & Ghulam, M. (2002). Review agriculture in the Indus plains: Sustainability of land and water resources. International Journal of Agriculture and Biology, 4, 429–437.
- Ahmad, S., & Prashar, D. (2010). Evaluating municipal water conservation policies using a dynamic simulation model. Water Resources Management, 24(13), 3371–3395. https://doi.org/10.1007/s11269-010-9611-2
- Amiri, V., Rezaei, M., & Sohrabi, N. (2014). Groundwater quality assessment using entropy weighted water quality index (EWQI) in Lenjanat, Iran. Environmental Earth Sciences, 72(9), 3479–3490. https://doi.org/10.1007/s12665-014-3255-0
- Bazame, R., Tanrıvermis, H., & Kapusuz, Y. E. (2019). Land management and sustainable use of land resources in the case of Burkina Faso. Land Degradation & Development, 30(6), 608–621. https://doi.org/10.1002/ldr.3247
- Bruce, J. P. (1992). Meteorology and hydrology for sustainable development. Secretariat of the World Meteorological Organization.
- Chen, B., Han, M. Y., Peng, K., Zhou, S. L., Shao, L., Wu, X. F., Wei, W. D., Liu, S. Y., Li, Z., Li, J. S., & Chen, G. Q. (2018). Global land-water nexus: Agricultural land and freshwater use embodied in worldwide supply chains. Science of the Total Environment, 613, 931–943.
- Chen, Y. (1999). Resource environment and regional sustainable development in the Tarim Basin (in Chinese). Resources and Environment in Arid Area, 13(1), 11–15.
- Coale, A. J., & Hoover, E. M. (2015). Population growth and economic development. Princeton University Press.
- Cuceloglu, G., Abbaspour, K., & Ozturk, I. (2017). Assessing the water-resources potential of Istanbul by using a soil and water assessment tool (SWAT). Hydrological Model. Water, 9, 814.
- Dai, M., Wang, L., & Wei, X. (2016). Spatial differentiation of water resources carrying capacity in Guangxi based on entropy weight fuzzy comprehensive evaluation model. Soil Water Conserv. Res, 23, 193–199.
- Davies, E. G., & Simonovic, S. P. (2011). Global water resources modeling with an integrated model of the social-economic-environmental system. Advances in Water Resources, 34(6), 684–700. https://doi.org/10.1016/j.advwatres.2011.02.010
- Dawadi, S., & Ahmad, S. (2013). Evaluating the impact of demand-side management on water resources under changing climatic conditions and increasing population. Journal of Environmental Management, 114, 261–275. https://doi.org/10.1016/j.jenvman.2012.10.015
- Fan, M., Xu, J., Chen, Y., Li, D., & Tian, S. (2020). How to sustainably use water resources-A case study for decision support on the water utilization of Xinjiang, China. Water, 12(12), 3564. https://doi.org/10.3390/w12123564
- Flint, R. W. (2004). The sustainable development of water resources. Water Resources Update, 127(2004), 48–59.
- Forrester, J. (1971). World dynamics. Wright-Allen Press.
- Fu, K. (2009). Research on sustainable development based on the carrying capacity of water and land resources in Zhumadian City (PhD dissertation). Lanzhou University (in Chinese). https://kns.cnki.net/kcms2/article/abstract?v=b4E8SuETvlIuVui8Wq-utH7U5d43k4wityTpuyU5byWx1ov0enyk6nwS2FDfjtnwuV9I-9HHWiSetPW3twRltjCi_B8jvZmwYqvRZJtOBRKSeQy8Y6_JnQw1eJ4KQVY0JTEM5SB7rYQm4tQ8E2sAmw==&uniplatform=NZKPT&language=CHS
- Garcia, J. A., & Alamanos, A. (2022). Integrated modelling approaches for sustainable agri-economic growth and environmental improvement: Examples from Greece, Canada and Ireland. Land, 11(9), 1548. https://doi.org/10.3390/land11091548
- Gleick, P. H. (1996). Water in crisis: Paths to sustainable water use. Ecological Application, 8(3), 571–579. https://doi.org/10.1890/1051-0761(1998)008[0571:WICPTS]2.0.CO;2
- Guo, Y. (2016). Research on the optimal allocation of water and land resources for ecosystem services: A case study of Zhengzhou City (PhD dissertation). Zhengzhou University (in Chinese). https://kns.cnki.net/kcms2/article/abstract?v=b4E8SuETvlKAghmWdJCrEa-d7xhxOyXVZgDcFh__Gmu1aaRzwJ25bRfKKM94FkWZsBjdjplx8E3WB-exLvjDzkDswbIJYrhJnpTEDGjOoDHNMsDxo1SSV86iMrcYxcRZbhA7WZ90ueR1RJMQSKgjvw==&uniplatform=NZKPT&language=CHS
- Han, B., Liu, H., & Wang, R. (2015). Urban ecological security assessment for cities in the Beijing–Tianjin–Hebei metropolitan region based on fuzzy and entropy methods. Ecological Modelling, 318, 217–225. https://doi.org/10.1016/j.ecolmodel.2014.12.015
- Holland, R. A., Scott, K. A., Flörke, M., Brown, G., Ewers, R. M., Farmer, E., Kapos, V., Muggeridge, A., Scharlemann, J. P., Taylor, G., & Barrett, J. (2015). Global impacts of energy demand on the freshwater resources of nations. Proceedings of the National Academy of Sciences, 112(48), E6707–E6716.
- IPCC. (2013). Climate change 2013: The physical science basis. Contribution of working group I to the fifth assessment report of the intergovernmental panel on climate change. Cambridge University Press, 1535 p.
- Janssen, M. A., Walker, B. H., Langridge, J., & Abel, N. (2000). An adaptive agent model for analysing co-evolution of management and policies in a complex rangeland system. Ecological Modelling, 131(2-3), 249–268. https://doi.org/10.1016/S0304-3800(00)00256-8
- Jiang, J., Xiao, G., Wu, W., Ke, X., He, Y., Song, Y., Zhou, X., Zhang, M., Liu, Y., Fu, X., Li, J., Peng, S., Qin, Y., Zhou, C., Li, Y., Li, W., & Tu, Q. (2022). Is river-course change associated with the crustal movement? Geocarto International, 37(26), 11846–11866. https://doi.org/10.1080/10106049.2022.2060328
- Jiang, Q. (2011). Evaluation of land and water carrying capacity in the Sanjiang Plain and simulation of its sustainable use. (PhD dissertation). Northeast Agricultural University (in Chinese). https://kns.cnki.net/kcms2/article/abstract?v=b4E8SuETvlL9UT3Ocrk_SzjTaUXv5BAwlUcI3e78aUpfLYbmjkFoRN38yr69FmSx001NKR2VqsqrfYXd2eXH-7DZsN9sw3vtfDZ74-yRc3I-Ef1MO8TdsEtwPv8kyatCNB2fZyCwQm7xiVrfVwX6sQ==&uniplatform=NZKPT&language=CHS
- Jiang, Q., Zhao, Y., Wang, Z., Fu, Q., Wang, T., Zhou, Z., & Dong, Y. (2018). Simulating the evolution of the land and water resource system under different climates in Heilongjiang Province, China. Water, 10(7), 868. https://doi.org/10.3390/w10070868
- Jose, M., & Padmanabhan, M. (2016). Dynamics of agricultural land use change in Kerala: A policy and social-ecological perspective. International Journal of Agricultural Sustainability, 14(3), 307–324. https://doi.org/10.1080/14735903.2015.1107338
- Kotir, J. H., Smith, C., Brown, G., Marshall, N., & Johnstone, R. (2016). A system dynamics simulation model for sustainable water resources management and agricultural development in the Volta River Basin, Ghana. Science of the Total Environment, 573, 444–457. https://doi.org/10.1016/j.scitotenv.2016.08.081
- Lan, X. (2020). Research on sustainable land use in Wuhan City based on resource value accounting. (Master Thesis). China University of Geosciences, 2020 (in Chinese). https://link.cnki.net/doi/10.27492/d.cnki.gzdzu.2020.000056
- Li, L., Lambin, E. F., Wu, W., & Servais, M. (2003). Land-cover changes in Tarim Basin (1964-2000): Application of post-classification change detection technique. In X. Pan, W. Gao, M. H. Glantz, & Y. Honda (Eds.), Ecosystems dynamics, ecosystem-society interactions, and remote sensing applications for semi-arid and arid land (Vol. 4890, pp. 74–89). SPIE Proceedings.
- Li, Z., Yu, G. A., Brierley, G. J., Wang, Z., & Jia, Y. (2017). Migration and cutoff of meanders in the hyperarid environment of the middle Tarim River, northwestern China. Geomorphology, 276, 116–124. https://doi.org/10.1016/j.geomorph.2016.10.018
- Lv, J. (2014). Simulation study on the coupling coordination of water and soil resources utilization and ecological environment in karst mountainous areas in southeastern Yunnan (Kunming Master Thesis). University of Science and Technology, 2014 (in Chinese). https://kns.cnki.net/kcms2/article/abstract?v=b4E8SuETvlIENyqqU1Q6TSjJDJZ9UJBX8TmO-McgwSLJ9ZYDbGCCQOfY72C4WT1dlyIXOgmfP1jthwQtxheCHj01219PxleDg5qNEMD1aVESCxIUqCJNLsBXP3VmnQ2arYHhj08wrMdY4RxYx15oXQ==&uniplatform=NZKPT&language=CHS
- Matlhodi, B., Kenabatho, P. K., Parida, B. P., & Maphanyane, J. G. (2021). Analysis of the future land use land cover changes in the Gaborone dam catchment using CA-Markov model: Implications on water resources. Remote Sensing, 13(13), 2427. https://doi.org/10.3390/rs13132427
- McDonald, R. I., Weber, K., Padowski, J., Flörke, M., Schneider, C., Green, P. A., Gleeson, T., Eckman, S., Lehner, B., Balk, D., Boucher, T., Grill, G., & Montgomery, M. (2014). Water on an urban planet: Urbanization and the reach of urban water infrastructure. Global Environmental Change, 27, 96–105. https://doi.org/10.1016/j.gloenvcha.2014.04.022
- Mohamed, E. S., Saleh, A. M., & Belal, A. A. (2014). Sustainability indicators for agricultural land use based on GIS spatial modeling in North of Sinai-Egypt. The Egyptian Journal of Remote Sensing and Space Science, 17(1), 1–15. https://doi.org/10.1016/j.ejrs.2014.05.001
- Moltz, H. L., Wallace, C. W., Sharifi, E., & Bencala, K. (2020). Integrating sustainable water resource management and land use decision-making. Water, 12(8), 2282. https://doi.org/10.3390/w12082282
- Nkosi, M., Mathivha, F. I., & Odiyo, J. O. (2021). Impact of land management on water resources, a South African context. Sustainability, 13(2), 701. https://doi.org/10.3390/su13020701
- Nziguheba, G., Adewopo, J., Masso, C., Nabahungu, N. L., Six, J., Sseguya, H., Taulya, G., & Vanlauwe, B. (2022). Assessment of sustainable land use: Linking land management practices to sustainable land use indicators. International Journal of Agricultural Sustainability, 20(3), 265–288. https://doi.org/10.1080/14735903.2021.1926150
- Qi, C., & Chang, N. B. (2011). System dynamics modeling for municipal water demand estimation in an urban region under uncertain economic impacts. Journal of Environmental Management, 92(6), 1628–1641. https://doi.org/10.1016/j.jenvman.2011.01.020
- Ren, L., Gao, J., Song, S., Li, Z., & Ni, J. (2021). Evaluation of water resources carrying capacity in Guiyang City. Water, 13(16), 2155. https://doi.org/10.3390/w13162155
- Rueda, V., Young, M. H., Faust, K., Rateb, A., & Leibowicz, B. D. (2022). System dynamics modeling in local water management: Assessing strategies for the city of Boerne, Texas. Water, 14(22), 3682. https://doi.org/10.3390/w14223682
- Rulli, M. C., Saviori, A., & D’Odorico, P. (2013). Global land and water grabbing. Proceedings of the National Academy of Sciences, 110(3), 892–897. https://doi.org/10.1073/pnas.1213163110
- Samie, A., Deng, X., Jia, S., & Chen, D. (2017). Scenario-based simulation on dynamics of land-use-land-cover change in Punjab Province, Pakistan. Sustainability, 9(8), 1285. https://doi.org/10.3390/su9081285
- Sun, Y., Liu, N., Shang, J., & Zhang, J. (2017). Sustainable utilization of water resources in China: A system dynamics model. Journal of Cleaner Production, 142, 613–625. https://doi.org/10.1016/j.jclepro.2016.07.110
- Sušnik, J., Masia, S., Indriksone, D., Brēmere, I., & Vamvakeridou-Lydroudia, L. (2021). System dynamics modelling to explore the impacts of policies on the water-energy-food-land-climate nexus in Latvia. Science of The Total Environment, 775, 145827. https://doi.org/10.1016/j.scitotenv.2021.145827
- Theissen, T., Aurbacher, J., Bedoshvili, D., Felix-Henningsen, P., Hanauer, T., Hüller, S., & Waldhardt, R. (2019). Environmental and socio-economic resources at the landscape level–potentials for sustainable land use in the Georgian Greater Caucasus. Journal of Environmental Management, 232, 310–320. https://doi.org/10.1016/j.jenvman.2018.11.024
- Tsanov, E., Ribarova, I., Dimova, G., Ninov, P., Kossida, M., & Makropoulos, C. (2020). Water stress mitigation in the Vit River Basin based on WEAP and MatLab simulation. Civil Engineering Journal, 6(11), 2058–2071. https://doi.org/10.28991/cej-2020-03091602
- Walmsley, J., Carden, M., Revenga, C., Sagona, F., & Smith, M. (2001). Indicators of sustainable development for catchment management in South Africa-review of indicators from around the world. Water Sa, 27(4), 539–550. https://doi.org/10.4314/wsa.v27i4.4968
- Wang, Y. (2018). Study on the influence of the change of water resources and its impact on ecological security in the arid: An example of Ebinur Lake Basin in Xinjiang (Master Thesis). Xinjiang University (in Chinese). https://kns.cnki.net/kcms2/article/abstract?v=b4E8SuETvlI-dI24me3w-d6wtRdSAsdbuaSbLSx6QB3JKOT6OCKiTT9a94Y70QcqT1Cb0-
- Wang, Z., Jiang, Q., Fu, Q., Jiang, X., & Mo, K. (2018). Eco-environmental effects of water resources development and utilization in the Sanjiang Plain, Northeast China. Water Supply, 18(3), 1051–1061. https://doi.org/10.2166/ws.2017.177
- Weber, A. (1982). On the location of industries. Progress in Human Geography, 6(1), 120–128. https://doi.org/10.1177/030913258200600109
- Weiland, U., Kindler, A., Banzhaf, E., Ebert, A., & Reyes-Paecke, S. (2011). Indicators for sustainable land use management in Santiago de Chile. Ecological Indicators, 11(5), 1074–1083. https://doi.org/10.1016/j.ecolind.2010.12.007
- Winz, I., Brierley, G., & Trowsdale, S. (2009). The use of system dynamics simulation in water resources management. Water Resources Management, 23(7), 1301–1323. https://doi.org/10.1007/s11269-008-9328-7
- Wu, W. (2003). Application de la geomatique au suivi de la dynamique environnementale en zones arides (PhD Dissertation). Université Panthéon-Sorbonne - Paris I, 2003. https://theses.hal.science/tel-00011113/document
- Wu, W., De Pauw, E., & Zucca, C. (2013). Using remote sensing to assess impacts of land management policies in the Ordos rangelands in China. International Journal of Digital Earth, 6(sup2), 81–102.
- Wu, W., Lambin, E. F., & Courel, M. F. (2002). Land use and cover change detection and modeling for North Ningxia, China. Proceedings of Map Asia, Bangkok, Thailand, Aug 7–9, 2002.
- Wu, W., & Zhang, W. (2003). Present land use and cover patterns and their development potential in North Ningxia. Journal of Geographical Sciences, 13(1), 54–62. https://doi.org/10.1007/BF02873147
- Yan, B. Y., Xing, J. S., Tan, H. R., Deng, S. P., & Tan, Y. N. (2011). Analysis on water environment capacity of the Poyang Lake. Procedia Environmental Sciences, 10, 2754–2759. https://doi.org/10.1016/j.proenv.2011.09.427
- Yang, G., Wang, Y. J., He, X. L., & Li, J. F. (2010). The study of three evaluation models of water resources carrying capacity in Manas River Basin. Advanced Materials Research, 113, 442–449. https://doi.org/10.4028/www.scientific.net/AMR.113-116.442
- Yin, J. (2001). Study on the connotation of sustainable land use and its evaluation index system (in Chinese). Journal of Hebei Agricultural University, 2001(01), 78–81.
- Zhang, M., Liu, Y., Wu, J., & Wang, T. (2018). Index system of urban resource and environment carrying capacity based on ecological civilization. Environmental Impact Assessment Review, 68, 90–97. https://doi.org/10.1016/j.eiar.2017.11.002
- Zhang, N. (2018a). High-efficient water-saving irrigation development and 13th Five-Year Plan in Xinjiang Uygur Autonomous Region (in Chinese). China Water Resources, 13, 36–38.
- Zhang, Z. (2018b). Research on coupled and coordinated development of urbanization and water resources in Xinjiang (PhD dissertation). Xinjiang University, 2018 (in Chinese). https://kns.cnki.net/kcms2/article/abstract?v=b4E8SuETvlL2x29J8QpjLLwmUolPgHwMnh94ZVRZyttoV5EYl2qbO8ETocUweOSjcvQOvfJt-5RiKpWQrT9eE8OBOdKVUQh3cGWQS2l4bVnK87m-pKEdQ7dZdNTMmk23fSog7NOz439t-pnsCbQGXA==&uniplatform=NZKPT&language=CHS
- Zou, Z., Sun, J., & Ren, G. (2005). Weighting of fuzzy evaluation factors by entropy method and its application in water quality evaluation. Acta Scientiae Circumstantiae, 25, 552–556.