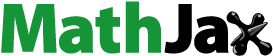
ABSTRACT
Off-target biodistribution of biologics bears important toxicological consequences. Antibody fragments intended for use as vectors of cytotoxic payloads (e.g. antibody-drug conjugates, radiotherapy) can accumulate at clearance organs like kidneys and liver, where they can cause dose-limiting toxicities. Renal and hepatic uptakes are known to be affected by protein electrostatics, which promote protein internalization through pinocytosis. Using minibodies as a model of an antibody fragment lacking FcRn recycling, we compared the biodistributions of leads with different degrees of accumulation at the kidney and liver. We identified a positive electrostatic patch highly conserved in a germline family very commonly used in the humanization of approved biologics. Neutralization of this patch led to a drastic reduction in the kidney uptake, leading to a biodistribution more favorable to the delivery of highly cytotoxic payloads. Next, we conducted a high throughput study of the electrostatic properties for all combinations of VH and VL germlines. This analysis shows how different VH/VL combinations exhibit varying tendencies to create electrostatic patches, resulting in Fv variants with different isoelectric points. Our work emphasizes the importance of carefully selecting germlines for humanization with optimal electrostatic properties in order to control the unspecific tissue uptake of low molecular weight biologics.
Introduction
The landscape of biologics has expanded to comprise constructs spanning a wide range of molecular weights and therapeutic modalities. The biodistribution and pharmacokinetics (PK) of these constructs can vary depending on a number of factors, primarily the molecular weight, recycling through the Fc neonatal receptor (FcRn), and surface-exposed charges.Citation1
Targeted proteins with molecular weight below the filtration threshold of the kidney glomeruli (approximately 70 kDa)Citation2 offer several advantages in terms of tumor penetration and rapid clearance, while retaining the target specificity and affinity of full-length IgGs (~150 kDa). In nuclear medicine, small biologics have been explored as vectors for diagnostic imaging and radiotherapy (e.g., single-chain Fvs (scFvs), Fabs, diabodies, minibodies, nanobodies, and DARPins), where the rapid blood clearance provides superior contrast ratio, and lower hematological exposure.Citation3 Antibody fragments (e.g., scFvs, Fabs, diabodies) have also been proposed as alternative scaffolds for antibody-drug conjugates, termed fragment drug conjugates (FDC), again aiming at exploiting the rapid systemic clearance to mitigate toxicity, and the accelerated tumor penetration compared to full-length IgGs.Citation4,Citation5
While immunoglobulins offer high binding specificity to tumor antigens, they also experience unspecific uptake at various tissues (especially clearance organs) as they internalize into cells through the process of pinocytosis.Citation6 Throughout the endothelial system, fluid phase endocytosis is the main driver of nonspecific internalization of IgGs and is mostly affected by electrostatic interactions with the negatively charged surface of cell membranes.Citation1 During this process, the invagination of the plasma membrane sequesters proteins into early endosomes, which can either recycle to the cell surface or migrate toward the juxtanuclear region where they mature into late endosomes (LE) and enter the lysosomal pathway.Citation7
For antibodies with a functional Fc domain, FcRn acts as a salvage mechanism, recycling the antibody back into circulation, de facto extending serum half-life while reducing off-target retention.Citation8 For antibody fragments that lack binding to FcRn, such salvaging mechanism is absent, and the internalized protein is retained into the LE, ultimately repurposed for lysosomal degradation with concomitant release of the toxic payload.
At clearance organs, protein electrostatics can also play more specific roles, with the glomerular basement membrane (GBM),Citation9 the proximal tubules brush border membrane,Citation10 and the liver sinusoid endothelium,Citation11 being primary sites of protein recycling. Small biologics show rapid serum clearance and can accumulate at the renal proximal tubules where they interact with the negatively charged glycocalyx coating the microvilli of the brush border membrane, as well as access a number of endocytic receptors.Citation12
Using in-silico electrostatic analysis on homology models, and in-vivo biodistributions in mice, we show that a conserved cluster of cationic residues found on a human germline very frequently used as a framework for humanization, can be responsible for unusually high kidney uptakes. This finding underscores that surface-exposed charged patches, while exerting a small influence on the PK and disposition of full-length antibodies, can significantly impact the biodistribution of small biologics.
Thus far, a systematic investigation of the human germlines elucidating how heavy chains and light chains pair to form antibodies with divergent electrostatic features has been lacking. To address this knowledge gap, we conducted an in-silico structure-based analysis of the electrostatic properties for all combinations of human VH and VL germlines and investigated their propensity to form clusters of charged residues. We observed distinct trends in which different framework families exhibited varying propensity to bring about proximities of equally charged residues. Specifically, a pronounced difference in the electrostatic contribution between kappa and lambda light chains was evident.
Our study underscores the significance of minimizing the electrostatic bias in antibody fragments during lead selection and humanization as a way of mitigating their background off-target uptake. It also highlights the importance of carefully selecting germline frameworks that minimize the clustering of charged residues.
Results
Electrostatics engineering and biodistribution of a minibody with uncharged CDRs
Minibodies are 80 kDa homodimers consisting in two scFvs fused to a human IgG1 CH3 domain via a flexible linker.Citation13 Since they lack FcRn binding, they show rapid serum clearance with most of the protein cleared from circulation within 24 h. As expected, different minibodies can display remarkably different biodistributions, with some minibodies showing primarily renal clearance and others mostly clearing through the hepatobiliary route. Since all minibodies in our study share the same hinge/CH3 scaffold, differences in biodistribution can be attributed primarily to the scFv region.
Minibody Mb1.1 was humanized with germlines IGHV1–69*01 and IGKV1–39*01 for the VH and VL, respectively. In Mb1.1 the scFv does not show surface-exposed positively charged residues on the complementary-determining regions (CDRs) (). However, modeling Adaptive Poisson-Boltzmann Solver (APBS) potentials show a large positive patch on the LC-FR2 corresponding to residues LC-K47, K50 and K53 (Aho numbering) (). These residues form a closely packed group with the centers of mass of the side chains falling within 11 Å, potentially also involving the N-terminal amine of the VH and LC-K145 from the IGKJ4*01 J-segment. Other smaller positive patches are visible: on the heavy chain, K13 and K14, and HC-R45, R77, R97 form two small patches on HC-FR1 and HC-CDR2/FR3, respectively.
Figure 1. APBS isopotential surfaces and ex-vivo biodistribution for scFv Mb1. Electrostatic potential cutoff was set to 1 kT/e. Positive charges and negative charges are colored blue and red, respectively. Numbers in parentheses represent changes in positive and negative charges between constructs. (a) scFv Mb1.1 does not show surface-exposed charges on the CDRs. Dotted curves denote the CDRs boundaries over a space-filling model. HC-CDR1, CDR2 and CDR3 are depicted in dark orange, midtone orange and light orange, respectively. LC-CDR1, CDR2 and CDR3 are in dark violet, midtone violet, and light violet. (b) two 180° orientations for scFv Mb1.1 showing clusters of positive residues in blue on germline frameworks. (c and d) ex-vivo kidney and liver biodistributions of minibody Mb1 variants at 24 h p.i. Data are shown as mean ± SEM. One-way ANOVA followed by Bonferroni’s post hoc test. ****p < .0001, **p < .01, *p < .05 vs. Mb1.1; ###p < 0.001 vs. Mb1.4. e) neutralization of K50 on scFv Mb1.2 and K50/K53 in Mb1.3. F) introduction of negative charges on the HC-CDR2 contribute to the formation of a negative patch in scFv Mb1.4.

As shown in , Mb1.1 displays high kidney uptake 24 h post-injection (p.i.) with the activity density reaching 62.3 ± 3.7%ID/gr. Partial neutralization of the VL patch in Mb1.2 (LC-K50Q) (), led to a drastic drop in the kidney activity with a two-fold reduction at 24 h. Further neutralization of this patch in Mb1.3 (LC-K50Q, K53Q) did not provide further reduction in the kidney activity ().
To investigate if negative charges would further reduce the kidney uptake, we modified the scFv in Mb1.2 introducing a negative patch on the HC-CDR2 at positions 68, 72 and 73 (Mb1.4 in ). As shown, the presence of this negative cluster did not produce any further reduction in kidney activity at 24 h () but resulted in an increase in the liver uptake ().
Immunofluorescence microscopy on kidney sections from mice injected with Mb1.1 shows that at the 24 h timepoint the minibody staining was almost exclusively co-located with megalin, indicating retention at the proximal tubules (). This confirms that after 24 h the minibody has completely crossed the glomerular basement membrane. Also, no uptake in the distal tubules is observable. For comparison, a full-length antibody at 24 h p.i. shows localization within the glomerular capillaries, indicating minimal passage through the GBM ().
Figure 2. (a) immunofluorescence microscopy of mouse kidney at 24 h after i.v. injection with minibody Mb1.1. White arrows point at glomeruli. Colocalization with megalin indicates the minibody accumulates at the proximal tubules. Gray: uromodulin (distal tubules); red: minibody; green: megalin (proximal tubules); blue: DAPI. (b) as a comparison, a full-length antibody control at 24 h post i.v. injection shows signal confined within the glomerular capillaries, owing to the slow blood clearance of full-length IgG, and indicating that the immunoglobulin has not experienced meaningful passage through the glomerular basement membrane. Green: podoplanin (glomeruli); yellow: megalin (proximal tubules); red: full-length antibody; blue: DAPI.

Electrostatics engineering and biodistribution of a minibody with charges on the CDRs
In a more complex case, we investigated a minibody with scFv bearing charges on the CDRs. Minibody Mb2.1 was humanized against the same germlines of Mb1.1 (IGHV1–69*01/IGKV1–39*01) and shows the same positive patch at the LC-FR2 (LC-K47, K50, K53) (), and a comparably high kidney activity at 24 h (). This patch neighbors an additional positively charged region over the CDRs arising from residues HC-K73/R111 on the HC-CDR2 and CDR3 and K110 on the LC-CDR3 (). Two negatively charged clusters, LC-E29/E111, and D67 on the HC-CDR2, that neighbor D83/E84 on HC-FR3 are visible. For this construct, neutralizing the patch on the LC framework (LC-K50Q, K53Q) in Mb2.2 only caused a partial reduction of kidney activity at 24 h ().
Figure 3. APBS isopotential surfaces and ex-vivo biodistribution for minibody Mb2 variants. Electrostatic potential cutoff was set to 1 kT/e. Positive charges and negative charges are colored blue and red, respectively. Numbers in parentheses represent changes in positive and negative charges between constructs. (a) the scFv Mb2.1 shows a positive patch in blue over the CDR3. Dotted curves denote the CDRs boundaries over a space-filling model. Heavy chain CDR1, CDR2 and CDR3 are depicted in dark orange, midtone orange and light orange, respectively. Light chain CDR1, CDR2 and CDR3 are in dark violet, midtone violet, and light violet. (b) similar to the scFv of Mb1.1, a large positive cluster on LC-FR2 is observable comprising residues LC-K47, K50, K53. (c) and (d) ex-vivo kidney and liver biodistributions of minibody Mb2 variants at 24 h p.i. (e) mutation of CDR residues lead to a further improvement in kidney uptake. K110G, on LC-CDR3 of Mb2.3. And neutralization of HC-K73 in scFv Mb2.4 which exposes E53. (f) introduction of a negative charge at position HC-73 leads to the formation of a negative patch in scFv Mb2.5. Data are shown as mean ± SEM. One-way ANOVA followed by Bonferroni’s post hoc test. ***p < .001 vs. Mb2.1; ꝉp < .05 vs. Mb2.2; ⊥p < .05 vs. Mb 2.3; ##p < .01, ####p < .001 vs. Mb2.5.

Although in our model of Mb2.2 the LC-CDR3 K110 was partially buried near the LC-CDR1, neutralization of this residue in Mb2.3 reduced the kidney activity below 40%ID/gr (). HC-K73 on the CDR2 is frequent across the IGVH1 germlines and is in close proximity to the cluster of HC-R45, R77, R97. Neutralization of K73 in Mb2.4 further reduces the kidney uptake by an additional 41% (). Of note, the mutation of HC-K73 in Mb2.5 to a negatively charged residue formed an additional negative patch probably due to its proximity to E53 on the HC-FR2 (Mb2.5 in ). This did not contribute to any further reduction in kidney activity but resulted in a small increase in liver uptake (). Mutations at K50Q and K53Q, which neutralized the positive patch on light-chain FR2, led to a reduction in the isoelectric point (pI) (Suppl. Table S1), and did not significantly affect the thermal stability and binding affinity for the antigens (Suppl. Figure S1).
To rule out the possibility that minibodies would interact with mouse blood serum proteins (such as albumin), which could facilitate indirect recycling of the minibody via FcRn, we tested Mb1.1 and Mb2.1 by ELISA for binding to mouse albumin and blood serum. As shown in Suppl. Figure S2, no measurable interactions were observed. Finally, we tested the potential binding of these minibodies to blood cells by incubating 89Zr radiolabeled Mb1.1 and Mb2.1 with cells isolated from mice blood. For both minibodies 99% of the activity was located in the supernatant, indicating no meaningful uptake in murine blood cells.
Binding to megalin and FcRn
To test if the minibody localization at the proximal tubules was mediated by the megalin receptor, we tested binding to LRP2 by ELISA. Human megalin is a large membrane protein with a 4398 residues extracellular domain, and 36 cysteine-rich complement-type repeats (CR) divided into four clusters.Citation14 Recombinant production of active fragments of megalin have been reported.Citation15 LRP2 is a natural receptor for the receptor-associated protein (RAP), a chaperone for LRPs, and antagonist protein which inhibit the interaction of LRP ligands with this family of receptors.Citation16 Constructs corresponding to the four megalin CR clusters, CR1–7, CR8–15, CR16–25, and CR26–36, were produced from transient transfections and tested by ELISA. We tested minibody Mb1.1, together with RAP and a human IgG1 antibody as positive controls (Suppl. Figure S3a–d).
The full-length immunoglobulin showed weak but saturable binding to each megalin construct. This is consistent with reported binding data by surface plasmon resonance where IgGs were shown to bind megalin and cubilin with affinities in the range µM to nM.Citation17 Comparable affinities were also reported for light chains, which show micromolar affinity for megalin.Citation18 For the minibody, the binding was practically undetectable for each of the four clusters. Conversely, RAP demonstrated the expected stronger affinity in the single digit nM to each megalin construct.
Since minibodies do not have a functional Fc, they are not expected to bind to FcRn. However, electrostatic mediated secondary interactions with FcRn have been reported for positively charged antibodies.Citation19 Such interaction could be important in the nephron, considering that FcRn was reported to play a role in IgG recycling at the podocytes and proximal tubules.Citation20,Citation21 We tested the positively charged minibody Mb1.1 for binding to FcRn, proving that no residual interaction with FcRn was observable for the minibody (Suppl. Figure S3e,f).
Electrostatic properties of germline pairs
Electrostatic features of Fvs comprising all combinations of VH and VL frameworks were compared in terms of pI, total number of positively and negatively charged residues, number of proximities of residue with equal charge, and number and length of clusters of positively and negatively charged residues. As a proxy for electrostatic patches, we detected proximities of equally charged residues, where a proximity was counted if the centers of mass of the side chains fell within an arbitrary distance. In our analysis, only the framework regions were considered so that only the impact of the germline on the Fv electrostatics would be assessed, excluding the CDRs. Results from these calculations are reported in Supplementary figures 4–14.
Theoretical, sequence-based pIs were in the range 4.99 to 9.73, with most of the pI values concentrated in the basic range, between 7.5 and 9.5 (Suppl. Figure S4a and S6). The most acidic germlines were as follows: IGKV2–28, IGKV2–40, IGKV2D–28, IGKV2D–40, IGKV5–2 and IGLV3–1, IGLV3–10, IGLV3–16, IGLV3–19, IGLV3–22, IGLV3–25, IGLV8–61 among the light chains, and IGHV1-69-2, IGHV3–35 and IGHV8-51-1 among the heavy chains. Overall, pI values were uniformly distributed across all Fv combinations, although the lowest pI was concentrated among a few lambda chains (families IGLV3 and IGLV8) and the IGKV5–2.
On average, heavy-chain germlines in the group IGHV4 through IGHV8 have fewer positive and fewer negative charges than germlines in the group IGHV1 through IGHV3 (Suppl. Figure S4b). Similarly, the lambda VLs have fewer positive and fewer negative charges than kappa VLs (Suppl. Figure S4c). When paired, the total number of positive and negative charges for VH/VL pairs were in the range 9 to 23 and 7 to 19, respectively (Suppl. Figures S7, S8 and S4d,e). Moving down the families of VH germlines, from IGHV1–2 to IGHV8-51-1, a slight trend is visible where the total number of positive and negative charges decreases (Suppl. Figures 4d,e, S7 and S8). The IGHV3–16 family stands out with a count of ≥ 17 positive charges regardless of the VL it was paired with.
The number of proximities was in the range 1 to 31 for positively charged residues (Suppl. Figure S4f and S9), and 1 to 15 for negatively charged residues (Suppl. Figures S4g and S10). On average, VH germline families in the group IGHV1 through IGHV3 show a tendency to form more positively charged proximities than families in the group IGHV4 through IGHV8. Lambda light chains show an average tendency to form fewer positively charged proximities and more negatively charged proximities than kappa VL. The IGHV3–16 family stands out showing ≥ 23 positively charged proximities regardless of the VL it was paired with. Combinations of heavy chains IGHV4–31*01, IGHV5–51*07 and IGHV7–81*01 with IGLV8–61*02, only led to 1 positively charged proximity. The highest number of positive proximities (31) was recorded for IGHV3–16*01 and IGHV3–16*02, in combination with IGKV2–30*01 and IGKV2–30*02 light chains. On the other hand, the highest number of negative contacts (15) was found in the IGLV3–10*02 and IGLV3–10*03 in combination with the VH germlines IGHV1–3*02, IGHV1–3*03 and IGHV1–24*01. The lowest number of proximities of negatively charged residues (1) was counted for the IGHV3–30, IGHV3–38 and IGHV8–51 heavy chains in combination with the IGLV2–33 family.
Finally, we calculated the length of the longest cluster of positively and negatively charged residues for each germlines pair, and the total number of clusters (Suppl. Figure S5, and S11–S14). Heavy-chain frameworks in the group IGHV1 through IGHV3 had on average a higher number of positively charged clusters than the group of families IGHV4 through IGHV8 (Suppl. Figure S5c and S13). Lambda light chains, on average, show longer clusters of negatively charged residues regardless of the heavy-chain framework they were paired with (Suppl. Figure S5b and S12). The IGHV3–16 family stands out for having the longest cluster of positively charged residues (≥13).
Discussion
The rapid clearance of antibody fragments can be advantageous for the delivery of toxic payloads for which prolonged circulation times may result in undesirable systemic exposure. Several sub-compartments at clearance organs can retain charged proteins. In nephrons, both the glomeruli and the proximal tubules bear negatively charged moieties. The GBM contains three heparan sulfate proteoglycans, agrin, perlecan and collagen XVIII, which confer a negative charge to the GBM. The role of the GBM as a charge barrier (permselectivity), has been the subject of debate.Citation9 In conditional knock-out mice for perlecan and podocyte agrin, for example, the clearance of charged carriers was not affected, suggesting that the glomerular selectivity is attributable predominantly to conformation and size, and to a lesser extent to electrostatic charges.Citation22
In the proximal tubules, a known site of reabsorption for immunoglobulins as well as other molecules, this charge selectivity seems to be more pronounced. Isoelectric focusing experiments on urinary IgGs revealed that, in patients with dysfunctions of the proximal tubules, the proportion of cationic IgGs in the urine and serum is comparable, while in glomerular disorders only anionic or neutral immunoglobulins were found in the urine.Citation23
Preferential reabsorption of cationic proteins at the proximal tubules was proposed to occur both via endocytic receptors or through interactions with strong negative electrostatic charges on the filamentous brush border membrane.Citation24 The latter could be facilitated by the acidic urinary pH, which can approach pH 6 in this segment of the nephron.
Tubular recycling of immunoglobulins can also occur through specific endocytic multi-ligand receptors, such as the cubam complex (comprising megalin, cubilin, and amnionless), and FcRn. All these are known to mediate the promiscuous reabsorption of a diverse range of molecules, including IgGs.Citation10,Citation17 Interestingly, receptors of the LRP family, like megalin, have repeat clusters of acidic residues that have been proposed to interact with cationic proteins.Citation25 Similarly, FcRn was also reported to play a role in the renal reabsorption of IgGs at proximal tubules, and was shown to have electrostatic binding to the variable domain of positively charged antibodies, in addition to the well-known pH-dependent interaction with the IgG Fc.Citation19–21
In the liver, sinusoid endothelial cells and Kupffer cells act as specialized scavengers, mostly through clathrin-rich caveolae and scavenger receptors.Citation26 Several of the hepatic scavenger receptors are reported to mediate the endocytosis of anionic macromolecules.Citation11 Endocytosis of negatively charged macromolecules was also found in the sinusoidal endothelial lining.Citation27 Using electron microscopy and particles of different size and charge, anionic macromolecules could be found in coated pits, colocated with positively charged glycocalyces extending tens of angstroms away from the negatively charged cell surface.Citation27
Small biologics are exquisitely sensitive to the presence of electrostatic charges. Using immunofluorescence microscopy, we show that minibodies bearing positively charged patches in the Fv can be located at the proximal tubules after 24 h p.i., when the protein had mostly cleared from circulation (). Binding experiments with the megalin endocytic receptor and FcRn showed no appreciable interaction with these minibodies (Suppl. Figure S3), confirming that the renal uptake for this class of proteins is predominantly mediated by electrostatic interactions.
Next, we tested if neutralizing charged residues would affect the biodistribution of these minibodies. We show that the cationic nature of the IGKV1–39*01 framework resulted in an increase in renal uptake and retention, and we narrowed down the effect to a positively charged patch very conserved across the IGKV1 family, comprising residues K47, K50 and K53 on the LC-FR2 (Suppl. Figure S16).
Neutralization of this patch was sufficient to trigger a drastic reduction in kidney uptake, which was more evident when the scFv did not carry additional positive charges on the CDRs. Engineering of nearby negative patches did not lead to any further reduction in the kidney uptake but resulted in a slight increase in liver activity.
These results are of particular interest considering that the IGKV1–39*01 germline is the most frequently used light-chain framework in commercially approved biologics, and the IGKV1 and IGHV1 families appear in 53% and 31% of 119 approved biologics (Suppl. Figure S19 and S20). These germlines were also reported to be frequently represented in the human repertoire. Next-generation sequencing of leucocytes from 637 donors indicated IGHV1–69*01 and IGKV1–39*01 to be the second most popular germlines within their respective pools of VH and VL (with usage frequencies of 9.97% and 11.69%, respectively).Citation28 Also, the combination of IGHV1–69*01 and IGKV1–39*01 was reported to have high stability and proposed as an attractive target for humanization.Citation29
In agreement with our finding, frameworks from the IGKV1 family have been reported to affect the PK of full-length antibodies. Igawa et al. showed that mutations that decreased the pI in an IgG4 antibody humanized with IGKV1/IGHV4 frameworks resulted in a reduction of its clearance rate.Citation30 Similarly, Li et al. observed higher liver and spleen catabolism in rats for an antibody humanized with IGKV1/IGHV3 germlines. Reformatting this antibody to IGKV4/IGHV1 decreased the pI, leading to a slower serum clearance.Citation31 In both studies, all variants had identical affinities for FcRn. These reports are in line with the broader finding that, on average, antibodies with higher pI have increased tissue uptake and more rapid clearance.
Historically, pI has been used as a physicochemical parameter to describe the electrostatic bias of proteins. PK studies on antibodies with different pI allowed defining basic rules asserting that decreases in net positive charge are usually associated with lower tissue uptake and accelerated whole-body clearance.Citation12 However, the pI only represents a high-level view of the electrostatic nature of a protein and does not provide structural details about the effect of clustering charges into surface-exposed patches.
To understand how different germline combinations affect the electrostatic features of Fv, we conducted a simple analysis of all framework combinations derived from 307 VH germlines and 187 kappa and lambda VL germlines, for a total of 57,409 pairs (Suppl. Figure S4–S14). CDRs were intentionally excluded from our analysis to account solely for the contribution from the framework regions.
We organized VH and VL germline into four groups, comprising VH families IGHV1 through IGHV3 and IGHV4 through IGHV8, respectively, paired with kappa and lambda light chains. From this analysis, some interesting trends emerge. The values of pI ranged in the interval 4.99–9.79, with a preference for basic pI (Suppl. Figure S4a). This confirms the known fact that IgGs tend to possess basic pIs.Citation12
On average, germlines from the group IGHV1 through IGHV3 had more positively and more negatively charged residues than families IGHV4 through IGHV8 (Suppl. Figure S4b). They exhibited a higher number of proximities of positively charged residues, and a higher number of positively charged clusters than the group of families IGHV4 through IGHV8.
Lambda light chains had a lower average number of positively and negatively charged residues than kappa VLs (Suppl. Figure S4c). They tended to form fewer positively charged proximities and more negatively charged proximities, and they had longer negatively charged clusters than kappa VLs. Some lambda light chains of the IGLV3 and IGLV8 families stood out as showing remarkably acidic features leading to the lowest values of pI, nearly with any IGHV framework they were paired with (Suppl. Figure S6). A close examination of the lambda sequences reveals a higher density of Glu and Asp in the FR3 (Suppl. Figure S17). Of note, although less frequently used, lambda light chains appear in 10% of the approved biologics in our list (Suppl. Figures S19 and S20).
Admittedly, our analysis has limitations. For instance, only one static 3D model was used to mimic all germline pairs, not accounting for protein conformational dynamics, and structural differences probably occurring for different VH/VL pairs. To that end, averages from molecular dynamics simulation on individually generated homology models could have produced more accurate results, although at a high computational cost. However, the loose cutoff distance which we set at 11 Å was probably sufficient to accommodate for such structural variability.
Also, our analysis only considered unmodified frameworks, void of back-mutations, and only one J segment was used: IGHJ1*01 for the VH, and IGK1J*01 and IGL1J*01 for the kappa and lambda VL. It is conceivable that a different choice of frameworks 4 could alter the results to some degree. For example, IGHJ1*02, *03 and *04 carry an additional cationic residue at position 141, closely located to the VH/VL interface. When paired with an IGKV1 light chain, this additional positive charge would fall in proximity to the positive patch LC-K47, K50, K53, further expanding the extent of this charged cluster. Conversely, the IGLJ7*01 and *02 segments lack the lysine at position 145 and could contribute to a lower pI.
Also of note, our approach only considers positive and negative charges in isolation. It does not consider cases where interspersed opposite charges prevent the formation of continuous patches. Finally, although pairing of VH/VL germlines appears to be promiscuous and does not show an intrinsic pairing preference,Citation32–35 many of the germline combinations we tested are probably not of interest for biologics discovery because of poor manufacturability or because they rarely occur in nature.
In conclusion, our work suggests that additional criteria of selection during humanization of small biologics could be useful to avoid generating leads with an electrostatic bias that promotes off-target uptake. The impact of concentrated charges on PK and polyreactivity of antibodies has been recently reviewed.Citation36 Excessive positive charges can affect the off-target uptake promoting pinocytosis as well as electrostatic interactions with FcRn.Citation19 We show that in smaller biologics, with a molecular weight below the sieving threshold of the GBM, and for which the binding to FcRn is silenced, clustering of charged residues can result in increased tissue accumulation, with cationic proteins mostly homing to the kidney, and anionic proteins favoring hepatic accumulation. This effect can be more pronounced than in full-length IgGs, which experience much lower passage through the GBM and for which FcRn remains the main factor affecting their blood clearance rate.
It is conceivable that avidity could also play a role in exacerbating the effect of electrostatic patches on the variable regions. In this regard, the same charged patch on both scFv in the minibody could result in a compounded affinity for the electrostatic nonspecific binding due to a bivalent interaction.
Our analysis further stresses the importance of complementing the engineering process with a structure-based investigation of the protein electrostatics, rather than limiting the analysis to using the protein pI. At a minimum, the selection of leads with non-charged CDRs, combined with frameworks that do not carry large electrostatic patches, or extreme values of pI, would be preferable. This is of particular relevance for applications aimed at the delivery of cytotoxic payloads.
Materials and methods
Protein engineering
Minibodies Mb1 and Mb2 were engineered from parental murine antibodies derived from two separate immunization campaigns with different antigens. The murine antibodies were humanized by grafting the parental CDRs onto the framework of closest matching human germlines IGKV1–39*01 and IGHV1–69*01 and the IGHJ4*01 and IGKJ4*01 J-segments. The sequence of the scFv was fused into the scaffold of a minibody.
The extracellular domain of human megalin (low-density lipoprotein receptor-related protein 2, LRP2, UniProt P98164) was divided into four constructs corresponding to the four clusters of complement-type repeats: CR1–7 (residues 26–365), CR8–15 (residues 970–1433), CR16–25 (residues 2646–3196), and CR26–36 (residues 3466–4053) and expressed recombinantly. Similarly, human RAP, residues 35–357 (Uniprot P30533) was synthesized as C-terminal his-tag. Human FcRn (residues 24–297, UniProt P55899) was synthesized as C-terminal His tag and co-transfected transiently with the human beta-2-microglobulin (residues 21–119, UniProt P61769). All proteins were expressed and purified from transient transfections using the Gibco Expi293™ Expression System (ThermoFisher Scientific, cat# A14524). Mouse albumin was purchased from Sigma Aldrich (cat# A3139).
In silico modeling and electrostatics analysis
Sequences of approved biologics were derived from The Antibody Society database (Suppl. Figures S1 and S2).Citation37 All sequence numbering in these articles are based on the Honegger numbering system (AHo),Citation38 and IMGT definition for the CDRs.Citation39 Sequences of human germlines were obtained from the IMGT repository (Suppl. Figure S15-S18).Citation40 Only functional and open reading frame sequences were included. Pseudogenes, orphons and suspected nonfunctional genes were not included in the analysis. Eight subgroups were included for the IGHV gene (307 sequences), 6 subgroups for the IGKV (91 sequences including proximal and distal clusters), and 11 subgroups for the IGLV (96 sequences), leading to 57,409 combinations. The J-segments were kept fixed using the IGHJ1*01, IGKJ1*01 and IGLJ1*01 sequences.
To estimate the extent of clustering of equally charged residues in each combination of VH and VL frameworks, a model of Fv was used to create a Boolean proximity matrix: . In this matrix, two residues were marked as in proximity to each other if the centers of mass of the sidechains fell within an arbitrary distance of 11 Å. The framework sequences where then converted to Boolean diagonal matrices, σ, where only positively charged or negatively charged residues were marked as true. Clusters of positively charged or negatively charged residues were then identified through the dot product:
Values of pIs for all combinations of HC/LC germline pairs were calculated in Biopython. The CDR regions were intentionally excluded from these calculations to isolate the contribution of the framework.
Three-dimensional models of the scFvs were constructed in SabPred – ABodyBuilder-ML.Citation41 Electrostatic potentials were calculated with the Adaptive Poisson-Boltzmann Solver (APBS) within PyMol (Schrödinger), and plotted as iso-potential surfaces with a cutoff at ± 1.0 kT/e.Citation42
In-vivo experiments
All in-vivo experiments were conducted in accordance with ImaginAb’s Institutional Animal Care and Use Committee policy and procedures, and in strict accordance with recommendations in the Guide for the Care and Use of Laboratory Animals of the National Research Council. All treatments were administered by intravenous injection (i.v.), and euthanasia was performed under isoflurane anesthesia. All efforts were made to minimize discomfort.
In-vivo biodistributions were performed in Nu/J mice (The Jackson Laboratory strain #002019). The deferoxamine-conjugated minibodies, radiolabeled with 89Zr to a target-specific activity of 5 µCi/µg were injected by lateral tail vein injection, at a radiation dose of 50 µCi per animal in 100 µL of phosphate-buffered saline (PBS). After 24 h, the animals were euthanized and organs collected, weighed, and activity counted in a gamma counter (Hidex AMG). Organ uptake was presented as the density of the %ID per gram of the organ (%ID/gr).
Binding to blood cells was tested on blood extracted from Nu/J mice in an EDTA-coated tube. Cells were isolated by centrifugation (2000 rpm, 20 min, 4°C), plasma was removed, and the cells washed with PBS. 89Zr radiolabeled Mb1.1 and Mb2.1 were added to the cells and incubated at 37°C for 1 h. The cells were then centrifuged and washed three times with PBS. The activities of the supernatant and the cell pellet were measured in a gamma counter.
Immunofluorescence microscopy
Mice were injected with 500 µg unlabeled minibody and after 24 h the kidneys were removed and frozen in OCT embedding matrix (Scigen, cat# 4586). Ten µm cryosections were mounted on glass slides and the OCT was removed with PBS. Kidney sections were then fixed in 10% formalin (Millipore Sigma, cat# HT5012) for 3 min and washed in PBS. Autofluorescence was quenched using TrueView Autofluorescence quenching agent (Vector Laboratories, cat# SP8400) according to manufacturer’s instruction.
Primary antibodies, rat anti-mouse-uromodulin (Thermo Fisher, cat# MA5–24374) and rabbit anti-mouse-megalin (Abcam, cat# ab76969), were applied sequentially at 100 nM with 1 h incubation. DAPI (Thermo Fisher, cat# D1306) was applied for 1 h in PBS. Secondary antibodies were applied for 1 h in PBS at the following ratio: goat anti-rat-Alexa488 (Thermo Fisher, cat# A11006) 1:200, goat anti-rabbit-DyLightTM 680 1:200 (Thermo Fisher, cat# 35568), goat anti-human-Alexa546 1:200 (Thermo Fisher, cat# A21089). Each step was followed by three washes in PBS. Before images were collected, slides were covered with mounting medium and coverslipped.
The full-length antibody was labeled with Alexa594 (Thermo Fisher, cat# A20104) and 360 µg was injected into the mice. At 24 h, the kidneys were frozen and sectioned as described above. Sections were treated with 10% formalin (Millipore Sigma, cat# HT5011-1CS) for 3 min then washed in PBS and treated with TrueBlack (Biotium, cat# NC1125051) to quench background fluorescence. Sections were incubated with primary antibodies for 1 h in PBS 3% bovine serum albumin (BSA): hamster anti-mouse-podoplanin (Novus Biologicals, cat# NB600-1015SS) at 100 nM and rabbit anti-mouse-megalin at 100 nM (Abcam, cat# ab76969). Following three washes with PBS, the sections were incubated with secondary antibodies, goat anti-hamster-Alexa488 (Thermo Fisher, cat# A21110), goat anti-rabbit-DylightTM 680 (Thermo Fisher, cat# 35568), and DAPI for 1 h in PBS 3% BSA. Control slides were prepared with mice injected with saline using secondary antibodies only to establish the higher exposure time that yielded no visible background on each channel.
Statistical analyses
All data are presented as mean ± SEM and were analyzed by one-way ANOVA followed by a Bonferroni post hoc multiple comparison test. All analyses were performed using Prism 10.1 (GraphPad, USA) and the differences were considered statistically significant at p value < 0.05.
Abbreviations
ADC | = | antibody-drug conjugate |
APBS | = | Adaptive Poisson-Boltzmann Solver |
CDR | = | complementary-determining region |
CR | = | complement-type repeat |
BSA | = | bovine serum albumin |
DARPin | = | designed ankyrin repeat protein |
FcRn | = | Fc neonatal receptor |
FDC | = | fragment drug conjugate |
FR | = | framework |
GBM | = | glomerular basement membrane |
HC | = | Heavy chain |
LC | = | Light chain |
LE | = | late endosome |
VH | = | Variable heavy chain |
VL | = | Variable light chain |
PBS | = | phosphate-buffered saline |
PK | = | pharmacokinetics |
p.i. | = | post-injection |
pI | = | isoelectric point |
RAP | = | receptor-associated protein |
scFv | = | single-chain Fragment variable domain |
SEC | = | size exclusion chromatography |
Supplemental Material
Download PDF (2.6 MB)Disclosure statement
No potential conflict of interest was reported by the author(s).
Supplementary material
Supplemental data for this article can be accessed online at https://doi.org/10.1080/19420862.2024.2311991
Correction Statement
This article has been corrected with minor changes. These changes do not impact the academic content of the article.
Additional information
Funding
References
- Ryman JT, Meibohm B. Pharmacokinetics of monoclonal antibodies. CPT Pharmacomet Syst Pharmacol. 2017;6(9):576–9. doi:10.1002/psp4.12224.
- Hamano Y, Grunkemeyer JA, Sudhakar A, Zeisberg M, Cosgrove D, Morello R, Lee B, Sugimoto H, Kalluri R. Determinants of vascular permeability in the kidney glomerulus. J Biol Chem. 2002;277(34):31154–62. doi:10.1074/jbc.M204806200.
- Carter LM, Poty S, Sharma SK, Lewis JS. Preclinical optimization of antibody-based radiopharmaceuticals for cancer imaging and radionuclide therapy—Model, vector, and radionuclide selection. J Label Compd Radiopharm. 2018;61(9):611–35. doi:10.1002/jlcr.3612.
- Deonarain MP. Miniaturised ‘antibody’-drug conjugates for solid tumours? Drug Discov Today Technol. 2018;30:47–53. doi:10.1016/j.ddtec.2018.09.006.
- Deonarain MP, Yahioglu G, Stamati I, Pomowski A, Clarke J, Edwards BM, Diez-Posada S, Stewart AC. Small-Format Drug Conjugates: A Viable Alternative to ADCs for Solid Tumours? Antibodies. 2018;7(2):16. doi:10.3390/antib7020016.
- Datta-Mannan A, Lu J, Witcher DR, Leung D, Tang Y, Wroblewski VJ. The interplay of non-specific binding, target-mediated clearance and FcRn interactions on the pharmacokinetics of humanized antibodies. MAbs. 2015;7(6):1084–93. doi:10.1080/19420862.2015.1075109.
- Huotari J, Helenius A. Endosome maturation. EMBO J. 2011;30(17):3481–500. doi:10.1038/emboj.2011.286.
- Pyzik M, Sand KMK, Hubbard JJ, Andersen JT, Sandlie I, Blumberg RS. The neonatal fc receptor (FcRn): a misnomer? Front Immunol. 2019;10:1540. doi:10.3389/fimmu.2019.01540.
- Miner JH. The Glomerular Basement Membrane. Exp Cell Res. 2012;318(9):973–78. doi:10.1016/j.yexcr.2012.02.031.
- Christensen EI, Birn H, Storm T, Weyer K, Nielsen R. Endocytic receptors in the renal proximal tubule. Physiol Bethesda Md. 2012;27(4):223–36. doi:10.1152/physiol.00022.2012.
- Terpstra V, van Amersfoort ES, van Velzen AG, Kuiper J, van Berkel TJC. Hepatic and extrahepatic scavenger receptors. Arterioscler Thromb Vasc Biol. 2000;20(8):1860–72. doi:10.1161/01.ATV.20.8.1860.
- Boswell CA, Tesar DB, Mukhyala K, Theil F-P, Fielder PJ, Khawli LA. Effects of charge on antibody tissue distribution and pharmacokinetics. Bioconjug Chem. 2010;21(12):2153–63. doi:10.1021/bc100261d.
- Hu S, Shively L, Raubitschek A, Sherman M, Williams LE, Wong JY, Shively JE, AM W. Minibody: a novel engineered anti-carcinoembryonic antigen antibody fragment (single-chain fv-CH3) which exhibits rapid, high-level targeting of xenografts. Cancer Res. 1996;56:3055–61.
- De S, Kuwahara S, Saito A. The endocytic receptor megalin and its associated proteins in proximal tubule epithelial cells. Membranes. 2014;4(3):333–55. doi:10.3390/membranes4030333.
- Vash B, Phung N, Zein S, DeCamp D. Three complement-type repeats of the low-density lipoprotein receptor-related protein define a common binding site for RAP, PAI-1, and lactoferrin. Blood. 1998;92(9):3277–85. doi:10.1182/blood.V92.9.3277.
- Bu G, Rennke S. Receptor-associated protein is a folding chaperone for low density lipoprotein receptor-related protein. J Biol Chem. 1996;271(36):22218–24. doi:10.1074/jbc.271.36.22218.
- Bryniarski MA, Zhao B, Chaves LD, Mikkelsen JH, Yee BM, Yacoub R, Shen S, Madsen M, Morris ME. Immunoglobulin G is a novel substrate for the endocytic protein megalin. AAPS J. 2021;23(2):40. doi:10.1208/s12248-021-00557-1.
- Klassen RBS, Allen PL, Batuman V, Crenshaw K, Hammond TG. Light chains are a ligand for megalin. J Appl Physiol Bethesda Md. 2005;98(1):257–63. doi:10.1152/japplphysiol.01090.2003.
- Schoch A, Kettenberger H, Mundigl O, Winter G, Engert J, Heinrich J, Emrich T. Charge-mediated influence of the antibody variable domain on FcRn-dependent pharmacokinetics. Proc Natl Acad Sci U S A. 2015;112(19):5997–6002. doi:10.1073/pnas.1408766112.
- Kobayashi N, Suzuki Y, Tsuge T, Okumura K, Ra C, Tomino Y. FcRn-mediated transcytosis of immunoglobulin G in human renal proximal tubular epithelial cells. Am J Physiol Renal Physiol. 2002;282(2):F358–365. doi:10.1152/ajprenal.0164.2001.
- Akilesh S, Huber TB, Wu H, Wang G, Hartleben B, Kopp JB, Miner JH, Roopenian DC, Unanue ER, Shaw AS. Podocytes use FcRn to clear IgG from the glomerular basement membrane. Proc Natl Acad Sci U S A. 2008;105(3):967–72. doi:10.1073/pnas.0711515105.
- Goldberg S, Harvey SJ, Cunningham J, Tryggvason K, Miner JH. Glomerular filtration is normal in the absence of both agrin and perlecan–heparan sulfate from the glomerular basement membrane. Nephrol Dial Transplant. 2009;24(7):2044–51. doi:10.1093/ndt/gfn758.
- Takahashi S, Wada N, Harada K, Nagata M. Cationic charge-preferential IgG reabsorption in the renal proximal tubules. Kidney Int. 2004;66(4):1556–60. doi:10.1111/j.1523-1755.2004.00920.x.
- Takahashi S. Origin of proteinuria as observed from qualitative and quantitative analysis of serum and urinary proteins. Child Kidney Dis. 2015;19(2):65–70. doi:10.3339/chikd.2015.19.2.65.
- Daly NL, Scanlon MJ, Djordjevic JT, Kroon PA, Smith R. Three-dimensional structure of a cysteine-rich repeat from the low-density lipoprotein receptor. Proc Natl Acad Sci. 1995;92(14):6334–38. doi:10.1073/pnas.92.14.6334.
- Bhandari S, Larsen AK, McCourt P, Smedsrød B, Sørensen KK. The scavenger function of liver sinusoidal endothelial cells in health and disease. Front Physiol. 2021;12:757469. doi:10.3389/fphys.2021.757469.
- Ghitescu L, Fixman A. Surface charge distribution on the endothelial cell of liver sinusoids. J Cell Biol. 1984;99(2):639–47. doi:10.1083/jcb.99.2.639.
- Glanville J, Zhai W, Berka J, Telman D, Huerta G, Mehta GR, Ni I, Mei L, Sundar PD, Day GMR. et al. Precise determination of the diversity of a combinatorial antibody library gives insight into the human immunoglobulin repertoire. Proc Natl Acad Sci. 2009;106(48):20216–21. doi:10.1073/pnas.0909775106.
- Teplyakov A, Obmolova G, Malia TJ, Luo J, Muzammil S, Sweet R, Almagro JC, Gilliland GL. Structural diversity in a human antibody germline library. MAbs. 2016;8(6):1045–63. doi:10.1080/19420862.2016.1190060.
- Igawa T, Tsunoda H, Tachibana T, Maeda A, Mimoto F, Moriyama C, Nanami M, Sekimori Y, Nabuchi Y, Aso Y. et al. Reduced elimination of IgG antibodies by engineering the variable region. Protein Eng Des Sel. 2010;23(5):385–92. doi:10.1093/protein/gzq009.
- Li B, Tesar D, Boswell CA, Cahaya HS, Wong A, Zhang J, Meng YG, Eigenbrot C, Pantua H, Diao J. et al. Framework selection can influence pharmacokinetics of a humanized therapeutic antibody through differences in molecule charge. MAbs. 2014;6(5):1255–64. doi:10.4161/mabs.29809.
- Jayaram N, Bhowmick P, Martin ACR. Germline VH/VL pairing in antibodies. Protein Eng Des Sel PEDS. 2012;25(10):523–30. doi:10.1093/protein/gzs043.
- Ahmed L, Gupta P, Martin KP, Scheer JM, Nixon AE, Kumar S. Intrinsic physicochemical profile of marketed antibody-based biotherapeutics. Proc Natl Acad Sci U S A. 2021;118(37):e2020577118. doi:10.1073/pnas.2020577118.
- DeKosky BJ, Kojima T, Rodin A, Charab W, Ippolito GC, Ellington AD, Georgiou G. In-depth determination and analysis of the human paired heavy- and light-chain antibody repertoire. Nat Med. 2015;21(1):86–91. doi:10.1038/nm.3743.
- DeKosky BJ, Lungu OI, Park D, Johnson EL, Charab W, Chrysostomou C, Kuroda D, Ellington AD, Ippolito GC, Gray JJ. et al. Large-scale sequence and structural comparisons of human naive and antigen-experienced antibody repertoires. Proc Natl Acad Sci U S A. 2016;113(19):E2636–2645. doi:10.1073/pnas.1525510113.
- Cunningham O, Scott M, Zhou ZS, Finlay WJJ. Polyreactivity and polyspecificity in therapeutic antibody development: risk factors for failure in preclinical and clinical development campaigns. MAbs. 2021;13(1):1999195. doi:10.1080/19420862.2021.1999195.
- Antibody therapeutics approved or in regulatory review in the EU or US. [Internet]. Antib. Soc. [cited 2022 Jul 11]; Available from: https://www.antibodysociety.org/resources/approved-antibodies/
- Honegger A, Plückthun A. Yet another numbering scheme for immunoglobulin variable domains: an automatic modeling and analysis tool. J Mol Biol. 2001;309(3):657–70. doi:10.1006/jmbi.2001.4662.
- Lefranc M-P, Pommié C, Ruiz M, Giudicelli V, Foulquier E, Truong L, Thouvenin-Contet V, Lefranc G. IMGT unique numbering for immunoglobulin and T cell receptor variable domains and Ig superfamily V-like domains. Dev Comp Immunol. 2003;27(1):55–77. doi:10.1016/S0145-305X(02)00039-3.
- Giudicelli V, Duroux P, Ginestoux C, Folch G, Jabado-Michaloud J, Chaume D, Lefranc M-P. IMGT/LIGM-DB, the IMGT comprehensive database of immunoglobulin and T cell receptor nucleotide sequences. Nucleic Acids Res. 2006;34(90001):D781–784. doi:10.1093/nar/gkj088.
- Dunbar J, Krawczyk K, Leem J, Marks C, Nowak J, Regep C, Georges G, Kelm S, Popovic B, Deane CM. SAbPred: a structure-based antibody prediction server. Nucleic Acids Res. 2016;44(W1):W474–8. doi:10.1093/nar/gkw361.
- Jurrus E, Engel D, Star K, Monson K, Brandi J, Felberg LE, Brookes DH, Wilson L, Chen J, Liles K. et al. Improvements to the APBS biomolecular solvation software suite. Protein Sci Publ Protein Soc. 2018;27(1):112–28. doi:10.1002/pro.3280.