ABSTRACT
The controlled expression of two or more proteins at a defined and stable ratio remains a substantial challenge, particularly in the bi- and multispecific antibody field. Achieving an optimal ratio of protein subunits can facilitate the assembly of multimeric proteins with high efficiency and minimize the production of by-products. In this study, we propose a solution based on alternative splicing, enabling the expression of a tunable and predefined ratio of two distinct polypeptide chains from the same pre-mRNA under the control of a single promoter. The pre-mRNA used in this study contains two open reading frames situated on separate exons. The first exon is flanked by two copies of the chicken troponin intron 4 (cTNT-I4) and is susceptible to excision from the pre-mRNA by means of alternative splicing. This specific design enables the modulation of the splice ratio by adjusting the strength of the splice acceptor. To illustrate this approach, we developed constructs expressing varying ratios of GFP and dsRED and extended their application to multimeric proteins such as monoclonal antibodies, achieving industrially relevant expression levels (>1 g/L) in a 14-day fed-batch process. The stability of the splice ratio was confirmed by droplet digital PCR in a stable pool cultivated over a 28-day period, while product quality was assessed via intact mass analysis, demonstrating absence of product-related impurities resulting from undesired splice events. Furthermore, we showcased the versatility of the construct by expressing two subunits of a bispecific antibody of the BEAT® type, which contains three distinct subunits in total.
Introduction
Many mature proteins, including commercially available biotherapeutics (e.g., antibodies, antibody-derived bispecific or multispecific formats), require assembly of multiple subunits to form a fully functional protein structure. For recombinant expression of such molecules, the relative expression of the subunits has an impact on overall production levelsCitation1–3 but also on product quality (e.g., levels of product-related impurities).Citation4 A simple method commonly used for the generation of high-expressing stable cell lines used for manufacturing of therapeutic antibodies is the co-transfection of monocistronic single-gene vectors,Citation5 which is followed by integration in the genome of concatemerized plasmids in one or few integration sites. This random integration event may lead to expression of the desired ratio of protein subunits, resulting in some transfectants producing high level of the protein product. However, in other transfectants, the cells may express the subunits in an unbalanced manner, which may occur due to an unfavorable ratio of plasmids stably integrated in the genome, thereby expressing at suboptimal levels or with undesired quality. The resulting screening efforts to select the few cell populations that express the subunits at the desired levels can be considered a major drawback of this approach.
The use of a single vector carrying expression cassettes for all subunits may help to overcome this limitation, while allowing high expression levelsCitation6 and potential use of targeted integration or semi-targeted integration using transposons.Citation7 However, the relative expression of the subunits needs to be adjusted at the DNA level, for example, by adjusting the promoter strength or the codon usage, which requires additional development work. A downside of both approaches is that different expression cassettes may undergo different levels of silencing, possibly due to different sensitivity of the promoters to silencing or a different genetic environment of the integrated plasmids, and as a result, the subunit ratio expressed within the cell populations may vary over time. Further, the possibility to control the expression level of the multiple expression cassettes (e.g., by using inducible promoters) is limited. The use of a polycistronic construct under control of a single promoter reduces the likelihood of silencing events affecting specific subunits and simplifies controlled expression. For example, the use of 2A self-cleaving peptides allows the generation of two proteins from a single mRNA transcript due to ribosomal skipping during translation.Citation8 However, this requires the presence of a 2A peptide sequence on the coding sequence, which may not always be possible or desirable. Moreover, this system always leads to an equimolar ratio of the subunits. Alternatively, on the mRNA level, internal ribosome entry sites (IRES) allow translation initiation from more than one open-reading frame (ORF) on the same primary transcript,Citation9 but at a fixed and non-tuneable ratio of subunits. In general, polycistronic approaches lead to lower titers (below 1 g/L)Citation8,Citation10 compared to monocistronic approaches with titers in the multigram per liter range.
A different approach to generate a polycistronic construct is offered by alternative splicing, a cellular mechanism that allows generation of different mature mRNAs from the same pre-mRNA. In this case, the pre-mRNA contains competing splice donor and acceptor sites that allow for alternative inclusion of different exons. This mechanism is used in nature to change the expression level of proteins or in order to modify protein activity during development.Citation10 Alternative splicing is usually controlled by complex interactions of many factors, including intron and exon size,Citation11 binding of regulatory proteinsCitation12,Citation13 and consensus sequences (i.e., branch point, splice donor and splice acceptor regions),Citation14–18 In the field of recombinant expression, alternative splicing has been described to generate variants of the same proteinCitation19 or to allow expression of two different proteins from the same pre-mRNA,Citation20 including the expression of antibody heavy and light chain resulting in secretion of fully functional antibody, but with very low titers (in the µg/L range).Citation21,Citation22 Although splicing is well known in the literature and consensus sequences of the splice sites have been published, as described above, the precise outcome of alternative splicing events depends on integration of multiple factors and this inherent complexity may be the reason for the low expression levels described so far for standard monoclonal antibodies (mAbs) using alternative splicing.
Here, we describe the generation of an alternative splicing construct based on the alternative chicken troponin exon 5 and its surrounding introns 4 (cTNT-I4) and 5 (cTNT-I5), allowing expression of multiple proteins from the same pre-mRNA. These sequences were used as a starting point because exon 5 is a well-characterized alternative exon.Citation10,Citation14 Troponin is expressed exclusively in cardiac muscle and embryonic skeletal muscle. Over 90% of the mRNAs include the exon 5 in early embryonic heart and skeletal muscle, whereas over 95% of mRNAs in the adult chicken exclude this exon.Citation10 In contrast to the natural constructCitation10 or previous approaches,Citation21,Citation22 we show that flanking the alternative exon by similar intron sequences (e.g., cTNT-I4 before and after the exon) allows modulation of the ratio of alternative splicing by consensus site modifications (e.g., modulation of the poly(Y) content of the splice acceptor).Citation18,Citation21,Citation22 The resulting bicistronic expression cassette allowed the expression of the desired ratios of proteins encoded by the two ORFs. The generation of stable cell lines expressing heavy chain and light chain of a mAb with industrially relevant expression levels demonstrated the utility of this expression cassette. Further, examples are provided for the expression of bispecific antibodies, demonstrating the versatility of the expression construct. A patent application relating to this work has been filed.Citation23
Materials and methods
Cloning of constructs expressing DsRed and GFP
Construct pGLEX3-ASC-DsRed-GFP (see supplementary information for cloning steps) is a pGLEX3 (a descendant of pcDNA3.1 (Invitrogen))-derived vector that contains a single expression cassette under control of the human CMV promoter. Modifications were introduced by amplification of the chicken troponin intron 4 or 5 using modified primers. These amplicons were then cloned in the backbone of pGLEX3-ASC-DsRed-GFP-Final using the restriction enzymes NheI and EcoRV for cloning in the position of Intron 2 and EcoRI and AgeI for cloning in the position of Intron 3 (see for intron numbering). shows all constructs that were cloned expressing GFP and DsRed, a summary of primers and PCR reactions is provided in supplementary Table S1 and Table S2, respectively.
Figure 1. Schematic drawing of the alternative splicing construct containing four exons. Exons 1,2 and 4 are constitutive, while Exon 3 may be excluded from the mRNA as part of an alternative intron (Intron 2’). (a) If Exon 3 is included in the spliced mRNA, the light chain will be expressed (on top of the drawing). If Exon 3 is spliced out from the mRNA, heavy chain will be expressed (on the bottom of the drawing). (b) Schema of the adapted construct expressing DsRed and GFP.
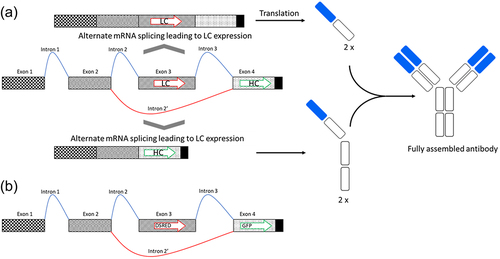
Table 1. List of constructs in pGLEX3 backbone expressing GFP and DsRed. Available constructs are indicated by a cross.
For stable expression, the alternative splicing cassette with I4(5Y–5) in Intron 2 and cTNT-I4 in Intron 3 was cut out of the backbone pGLEX3 using the enzymes NheI (R3131, New Englands Biolabs) and BstBI (R0519, New England Biolabs) and cloned into the backbone of pGLEX41 opened using the same enzymes and CIPed (M0525, New England Bioloabs). In this vector the alternative splicing cassette is driven by the mouse CMV promoter and a constitutive intron sequence.Citation24 The resulting vector was called pGLEX41-ASC-DsRed-GFP. The vector conferring the resistance genes against the antibiotic puromycin was a pGL3 (Promega)-derived vector, called pPuro. The puromycin resistance in this vector is under control of the SV40 promoter.
Cloning of constructs expressing antibodies
The codon optimized (for CHO expression) coding sequences for heavy and light chains of an anti-HER2 antibody were cloned in selected splice constructs described in replacing the genes coding for GFP and DsRed. A list of all plasmids generated is available in . A summary of primers and PCR reactions is provided in supplementary Table S1 and Table S2, respectively. In order to obtain stable expression of the reporter anti-HER2 antibody in CHO cells, the alternative splicing construct I4(0Y) in Intron 2 and I4 in Intron 3 was cut of pGLEX3 and cloned into expression vector pGLEX41 under control of the 2G UNicTM promoter (ProteoNic Biosciences) and a constitutive intron sequence.Citation24 A second expression cassette was cloned in this vector, coding for the glutamine synthetase gene under control of a weak promoter, leading to vector pGLEX41-2 G-anti-HER2-LC-HC. The constructs for the bispecific CD3/HER-2 BEAT® antibody were generated similarly (using a mouse CMV promoter instead of the 2G UNicTM promoter).
Table 2. List of constructs in pGLEX3 or pGLEX41 backbone expressing an anti-HER2 antibody. Available constructs are indicated by a cross.
Cultivation of cells
As described previously,Citation23 CHO cells were cultivated for routine passaging in PowerCHO2 (Lonza, Cat. No. BEBP12-771Q), 4 mM Glutamine (Sartorius, Cat. No. 03–020-1B) and HEK293 cells in Ex-cell293 (Sigma-Aldrich, Cat.No. 14571C), 4 mM Glutamine (Sartorius, Cat. No. 03–020-1B). Cells were seeded at a cell density of 0.5 × 106 cells/ml twice a week and incubated in a shaken incubator in an atmosphere of 5% CO2 and 80% humidity at 37°C.
Transient transfection of cells
As described previously,Citation23 the transfection was done in 12 or 24 well plates (TPP, Cat. No. 92012 and 92,024) using a final volume of 1 ml or 2 ml, respectively, or in 50 ml bioreactor tubes (TPP, Cat. No. 87050) using a final medium volume of 10 ml. For transfection, the cells were seeded at a density of 1.0 × 106 cells/ml prior to the day of transfection. The day of transfection, the cells were resuspended in either Opti-MEM (CHO; ThermoFisher, Cat. No. 31985070) or RPMI (HEK; ThermoFisher, Cat. No. 52400017) and transfected with JetPEI™ (Polyplus-transfection, Cat. No. 101000053) according to the manual of the manufacturer. After 5 h one volume of the respective growth medium supplemented with Pluronic F68 (ThermoFisher, Cat. No 24,040,032) was added. The cells were analyzed three to 5 days after transfection by flow cytometry for GFP and DsRed expression or using the Octet QK device (Fortebio) for mAb constructs.
Stable transfection
For the generation of stable cell populations expressing the two fluorescent reporter proteins, the DNA cocktail used was a mix of 95% of expression vector pGLEX3-ASC-DsRed-GFP-Final and 5% of pPuro. CHO cells were transfected and then incubated for 1 day on an orbital shaker. The following day, the cells were plated in different dilutions on 96 well plates under selection pressure (puromycin (ThermoFisher, Cat. No A1113802) at a concentration that reliably yields stable populations). After 1 week the selection pressure was refreshed. Screening for wells containing growing cells (mini pools) was performed after 2 weeks using an ELISA plate reader. Cells showing high levels of GFP/DsRed signal were expanded to 24 well plate scale. To obtain clonally derived populations, one mini pool was chosen for a second round of limiting dilution. For this, the cells were diluted at different concentrations and plated in 96-well plates. Clonally derived populations were selected and expanded based on the number of colonies growing on a plate and the absence of multiple growth centers in a well.
For the generation of stable cell pools expressing anti-HER2, the DNA cocktail used was a mix of 91% of expression vector pGLEX41-2G-anti-HER2-LC-HC (containing a second expression cassette for glutamine synthetase) and 9% of a vector coding for wild-type PiggyBac transposase. CHO cells lacking the gene for glutamine synthetase were seeded at a density of 1.0 × 106 cells/ml prior to the day of transfection. The day of transfection, the cells were resuspended in 50 ml bioreactor tubes in 5 ml CD CHO (ThermoFisher, Cat. No. 10743029) supplemented with 2 mM Glutamine and transfected with PEIproR (Polyplus-transfection, Cat. No. 101000017), according to the manual of the manufacturer. After 4 h, 5 ml of the growth medium supplemented with 2 mM of Glutamine was added. The following day, cells were centrifuged at 300 g for 5 min and resuspended into PowerCHO under selection pressure (methionine sulfoxide (Sigma-Aldrich, Cat. No. J62873.14) at a concentration that reliably yields stable populations). Three days later, the cells were passaged in bioreactor tubes at a concentration of 0.5 × 106 cells/mL in PowerCHO2 every two or three days. When cells reached 95% of viability, the resulting bulk pools were used for inoculation of a fed-batch in 125 mL shake flask (TriForrest, Cat. No. FPC0125S) at a cell density of 0.8 × 106 cells/mL. During the time of the fed-batch, glucose was kept constant around a setpoint of 5 g/L and feeding was initiated from day 3 onwards (using commercially available feeds).
Splice ratio assessment over multiple generations
The bulk pool population was passaged every two or three days at 0.5 × 106 cells/mL for 28 days (approximately 30 population doubling levels). On days 0, 14, 28, cell pellets were frozen in order to determine the mRNA splice ratio and sent on dry ice to Microsynth. As a statistical test, we used the Tukey–Kramer test performed with JMP software (Version 15.1.3, SAS)
Quantification of antibody
As described previously,Citation23 the secreted antibody was quantified 3–6 days after transient transfection using the Octet QK system (Fortebio) with Protein A bioprobes (Sartorius, Cat. No. 18–5010) according to the specifications of the manufacturer. The calibration curve was done using Herceptin (trastuzumab) as reference. For stable production, anti-HER2 was assessed using the Cedex Bio HT Analyzer IgG assay (Custom Biotech, Cat. No. 06 608 540 001).
Flow cytometric analysis
As described previously,Citation23 the cells were gated on living cells using forward and side scatter. For the analysis of the ratio of DsRed and GFP expressing cells, compensation was performed using DsRed transfected cells and GFP transfected cells. For the estimation of the shift from DsRed to GFP expressing cells, non-transfected cells were excluded by adding a gate. As a positive control, vectors expressing only DsRed (GSD636, an in-house vector based on pGLEX3, derived from pDsRed Express 1 (Clontech)) and pEGFP-N1 (Clontech) were transfected into the host cells, respectively.
Intracellular staining
Intracellular staining of antibody heavy and light chains was used to estimate cell culture populations expressing fully assembled antibodies and cells expressing antibody fragments. A day after transfection, cells were collected, washed in 2% bovine serum albumin (Sigma Aldrich, Cat. No. BSAV-RO in phosphate-buffered saline (PBS) (ThermoFisher, J61196.AP) twice, and fixed in D Cytofix/Cytoperm 1X solution (BD Bioscience, Cat.No. BD 554,714) for 20 min at 4°C. After fixing, cells were resuspended in Perm/Wash 1X solution (BD Bioscience, Cat. No. 557885), washed, and dissolved again in Perm/Wash Solution. APC-mouse anti-Human Ig kappa Light Chain (BD Pharmingen, Cat. No. 561323) and PE-conjugated goat anti-human IgG (Fc gamma specific) (eBioscience, Cat. No. 12-4998-82) were added to the cells in Perm/Wash solution and incubated in the dark at room temperature for 20 min. Labeled cells were washed two more times by centrifugation and resuspension in Perm/Wash solution. Stained cells with labeled antibodies or antibody fragments were separated and analyzed with Cytoflex cytometer (Beckman Coulter).
Protein purification
The cell suspension was spun down at 1000 g during 10 min. The supernatant was collected and incubated overnight at 4°C with Protein A beads (GE Healthcare) on a roller mixer. The supernatant with the beads was passed through Econo-Pac Chromatography Columns (Biorad, Cat. No. 7321010) that retains the beads. After a washing step with cold PBS, the antibody is eluted with Glycine pH 3.5 (Sigma Aldrich, Cat. No. G5417) and directly neutralized with a Tris solution pH 8.7 (Sigma Aldrich, Cat. No. 1083861000). The concentration was determined using the Nano drop (Thermo Scientific) device.
Mass spectrometry
Intact mass analysis consists of the direct mass measurement of the intact antibody. The experiment was performed on deglycosylated and non-deglycosylated antibody samples. For deglycosylation, 20 µg of sample were treated with PNGase-F (New England Biolabs) for at 3 hours at 37°C. Liquid chromatography was performed injecting 4 µg of sample on a Thermo Vanquish UHPLC system using an online desalting cartridge (Massprep microdesalting polyphenyl column, 2.1 × 5 mm, 1000 Å, 20 µm, Waters) coupled to an Electrospray quadrupole-orbitrap mass spectrometer with an extended mass range (Q-Exactive Biopharma, Thermo). The MS was operated in the positive ion mode and with the High Mass Range on. The spectra were acquired in the m/z 1500–6000 range with a 17.5 K resolution at m/z 200. Ten microscans were averaged. The in-source collision energy was set to 10 eV. The mobile-phase system consisted of 0.1% formic acid (FA) (mobile phase A) and 0.1% FA in acetonitrile (mobile phase B). The column outlet was connected directly to the electrospray ionization source. Column temperature was set to 40°C. The whole LC-MS system was controlled using the Chromeleon console. As control for the anti-HER 2 antibody, a commercial lot of the antibody with the same primary sequence was used (Herceptin® Lot. #N7631B03, Roche).
Results
Design of starting constructs
In order to express monoclonal antibodies using alternate splicing, a basic construct was designed containing four exons (Exon 1–Exon 4) and three introns (Intron 1 to Intron 3), as schematically depicted in . Exon 1 and Exon 2 contain the 5’ untranslated region (5’UTR) and are separated by Intron 1, which is constitutively cut out by the splice machinery of the cell. Exon 3 (the alternative exon containing the ORF for the light chain) and Exon 4 (containing the ORF for the heavy chain) are separated by Intron 3. In the alternative splicing event, Exon 3 is part of a larger intron, called Intron 2’composed by Intron 2, Exon 3 and Intron 3. Exon 4 is constitutively included in the mRNA but will only express the heavy chain if it is the first ORF on the mature mRNA. Therefore, if Exon 3 is present in the mRNA (splicing of Introns 1, 2, 3), only light chain will be translated (see , on top of the drawing). If Exon 3 is spliced out (splicing of Intron 1 and Intron 2’), the heavy chain will be expressed (see , on the bottom of the drawing). Of course, the position of heavy chain and light chain can also be inversed (heavy chain on Exon 3 and light chain on Exon 4). Both splicing events occur within every cell and the ratio can be adjusted as shown in the following. The resulting ratio of the mRNAs will then impact the translation of the encoded subunits.
In order to assess the expression of two alternative ORFs located on two different exons of the same primary transcript, a first construct with fluorescence markers DsRed (in Exon 3) and GFP (in Exon 4) was generated (). Both proteins, GFP and DsRed, can be intracellularly expressed at high levels, are well tolerated by cells and can be easily distinguished in a flow cytometric analysis or under a fluorescent microscope. These constructs allow simple visualization of the expression levels coming from Exon 3 and 4 and are extremely useful for the initial optimization of the molecular constructs. The initial construct was designed using intron 4 from chicken cardiac troponin T (cTNT-I4) as Intron 2 and intron 5 from chicken cardiac troponin T (cTNT-I5) for intron 3, because the small cTNT exon 5 naturally present between these introns is a well characterized alternative exon.Citation10,Citation14 In transfected CHO or HEK293 cells, the construct shows expression of both DsRed and GFP (see supplementary Figure S1 for CHO expression and for the gating strategy), but DsRed (first ORF) expression was largely favored over GFP (second ORF) expression.
Modifications of splice site consensus sequences (changes in poly(Y) content in Introns 2 and 3, in branch point and in intron-exon consensus).
In order to shift the splice ratio in favor of GFP expression, sequence modifications were introduced in the initial construct. It has been shown that the abundance of pyrimidine bases C or T (“Y”) between the branch point and the intron-exon border (the so-called poly(Y) tract) is important for the strength of the splice acceptor site.Citation11,Citation18 Different constructs with decreasing number of Ys in the poly(Y) tract of Intron 2 (from 29 in a modified version of the basic construct cTNT-I4 down to 0; see for alignment) were transfected in CHO and HEK293 cells using constructs listed in . After 3–6 days the cells were analyzed using flow cytometry, but no statistically significant shift in GFP expression could be detected (see and statistical assessment in supplementary Table S6).
Figure 2. Sequences of the Introns of the different splicing constructs used in this study. The different splice acceptor sequences parts are shown (branch point, polypyrimidine tract (poly(Y) tract), intron-exon border consensus sequence (C*)), the pyrimidines in the poly(Y) tract are highlighted in green, the sequence modifications are highlighted in red and underlined and the Start codon in Exon 3 or 4 is underlined). (a) Modifications in the splice acceptor site of Intron 2: modifications in the number of pyrimidines (Ys; the bases C and T) in the poly(Y) tract, modifications in the branch point region and modifications in the intron-exon consensus sequence. (b) Modifications in the poly(Y) tract of Intron 3. (c) Elimination of the splice donor site of cTNT-I4 located downstream of Exon 3. Shown is an alignment of the native cTNT-I4 sequence and the shortened version I4(sh), that lacks the splice donor consensus sequence (cTNT-I4 Intron is only displayed partially for better visibility).
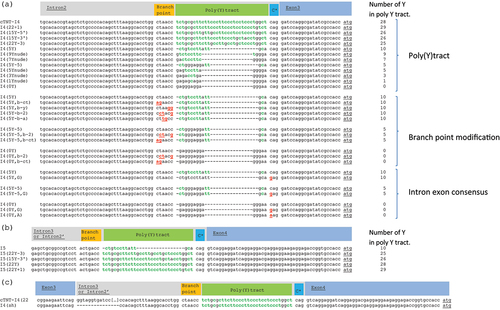
Figure 3. Heat map summarizing the optimization of the construct using fluorescent proteins in (a) CHO cells and (b) HEK293 cells. Modification of the intron arrangements allowed to shift the ratio. The cell population with Exon 3 being flanked by the same introns shows a higher level of double staining. The elimination of the splice donor site combined with the reduction of the poly(Y) tract (only performed for CHO) allowed to identify constructs with an even higher shift toward GFP expression. Every column is the mean of three individual transfections.
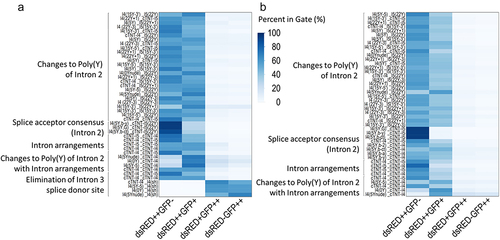
In these constructs, the splice acceptor of Intron 3 (which is also the splice acceptor of the alternative Intron 2’) has a poly(Y) tract containing only 10 Ys. Modified cTNT-I5 intron sequences with a strengthened splice acceptor containing up to 29 Ys in Intron 3 were transfected in CHO and HEK293 cells using constructs listed in (see for alignment). However, with one exception (DsRed++GFP+ showing a minor slope in CHO, but not in HEK293), no statistically significant shift to GFP expression was observed (see and statistical assessment in Table S6).
In order to decrease the strength of the splice acceptor region, modifications were introduced in the branch point region and in the intron-exon consensus region of Intron 2, upstream of alternative Exon 3 (constructs listed in , see for alignment). However, none of these modifications led to a significant shift to GFP expression (see for CHO and HEK293 cells) in transient transfection. On the contrary, they seemed to slightly reduce the alternative splicing event (DsRed population is higher compared to control).
Using the same intron flanking the alternative exon and re-evaluation of previous changes
The introns cTNT-I4 and cTNT-I5 were arranged around Exon 3 in all four possible permutations. Surprisingly, flanking the alternative exons with two identical introns slightly increased the double positive (DsRed and GFP) population (see ) in a modest, but statistically significant, manner. The construct with cTNT-I4 in both, Introns 2 and 3 showed the highest amount of double positive cells in CHO cells and was used as basis for further constructs. In order to analyze whether the reduction of the number of Ys in the poly(Y) tract shifts the splice ratio when Exon 3 is flanked by similar introns, different poly(Y) modifications of Intron 2 were used (cTNT-I4(5Y–5), cTNT-I4(0Y), cTNT-I4(5Ynude); constructs listed in , see for alignment). The combination of poly(Y) tract reduction and the use of cTNT-I4 for Intron 2 and Intron 3 showed a shift of the splice ratio toward GFP expression for all three constructs in HEK293 and CHO cells (). This shift was statistically significant for CHO. In HEK, the sample cTNT-I4(5Ynude) was an outlier. When removed, the shift was also statistically significant for HEK293 cells (see supplementary Table S7), with the exception of the gate DsRed-GFP++.
Additional modifications in the branch point regions in Intron 2 did not lead to a significant shift from DsRed to GFP (see , the constructs evaluated are listed in ).
In order to shift the splice ratio even further to GFP expression, the splice donor site of cTNT-I4 in Intron 3 was eliminated (constructs are listed in , see for alignment) by deleting the exon-intron consensus region (including the splice donor) of Intron 3. Importantly, the splice acceptor region (branch point, poly(Y) and the intron-exon consensus were not modified. This elimination increased the shift from DsRed expression to GFP expression. In some of the constructs this led to almost predominant GFP expression (e.g., cTNT-I4(5Ynude), see ).
Generation of stable cell populations
The pGLEX41-ASC-DsRed-GFP vector construct with cTNT-I4(5Y–5) in Intron 2 and cTNT-I4 in Intron 3 was used for the generation of stable CHO populations (see supplementary Figure S2). A comparison of the relative expression levels of DsRed and GFP of eight randomly chosen clonally derived cell populations showed a very similar ratio of DsRed to GFP expression, although the overall expression level varies between cell populations. All cell populations were double positive for DsRed and GFP and no cell populations were observed that expressed only GFP or DsRed. The similar splicing ratio of different cell populations derived from the same parental mini pool indicates that the splice ratio remains stable over multiple generations, without shifts toward one of the two exons.
Transient expression of a model antibody using alternative splicing constructs
After the successful generation of stable cell lines using the splicing construct coding for fluorescent molecules, an evaluation of the alternative splicing constructs for expression of antibodies was performed. An anti-HER2 antibody (for sequence, see supplementary Table S8) was used as a model protein. The ORFs coding for the anti-HER2 antibody heavy and light chains (HC and LC) were cloned in the expression construct, replacing the two fluorescence markers GFP and DsRed. Expression constructs with varying amounts of poly(Y) were selected from the preliminary study using GFP and DsRed (see ) based on the absolute expression level and the shift in the expression from the first (DsRed) to the second ORF (GFP). These were combined with the full-length cTNT-I4 or the shortened version (cTNT-I4sh) in Intron 3 generating the constructs listed in and transiently transfected in CHO cells. The amount of accumulated anti-HER2 antibody in the supernatant on day 6 was quantified, as well as the amount of intracellular IgG and the ratio of the alternative mRNAs (with and without Exon 3).
In all constructs, the anti-HER2 antibody expression level increases when the poly(Y) tract contains less than 7 Ys, independently whether Intron 3 is fully functional (cTNT-I4) or whether the splice donor (cTNT-I4(sh)) is disabled (, Titer). In agreement with this, the mRNA ratio in the constructs with the heavy chain in Exon 3 is shifted in constructs with orientation LC:HC toward a lower ratio of mRNA1 (expressing exon 3) to mRNA2 (expressing exon 4) as shown (mRNA ratio). Constructs with increased mRNA2 level will thus translate to higher levels of the subunit encoded by mRNA2 (and less from mRNA1), allowing optimization of the ratio of these subunits.
Figure 4. Anti-HER2 antibody expression with constructs having different splice ratios of mRNAs coding for subunits assembling the HER2 antibody. Expression results (“Titer”) and the splice ratio of mRNA 1 (with Exon 3) to mRNA 2 (without Exon 3) determined by ddPCR (“mRNA ratio”) are shown as a function of the orientation (light chain (LC) in Exon 3, heavy chain (HC) in Exon 4 or the other way round) and of the nature of Intron 3 (poly(Y) tract and cTNT-I4 vs cTNT-I4(sh)).
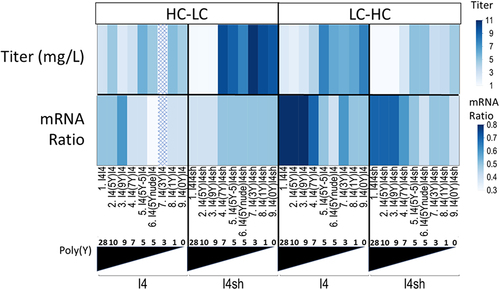
The configuration HC:LC shows less shifting in the mRNA ratio. A potential explanation for this phenomenon is the different length of the exons. The heavy chain ORF is about two times longer than the light chain ORF (~1500bps vs ~ 750bps). The distance between the splice donor site of Intron 2 and the splice acceptor site of Intron 3 is thus longer in the HC:LC construct as compared to the LC:HC construct. The resulting longer Intron 2’ may be less likely to be recognized by the splice machinery, irrespective of the number of Ys in the poly(Y) tract.Citation25 An additional explanation may be that the increase in strength of the poly(Y) tract leads to an overexpression of heavy chains. Misbalanced overexpression of heavy chain may represent a major challenge to the secretory pathway of the cell, as heavy chains require an assembly with light chain for correct folding.Citation26 In contrast to this, an excess of light chain can be secreted in the form of light-chain dimers and does not lead to a folding challenge for the cells.Citation27 Therefore, the lack of the shift in mRNA ratio may be a cellular response to the folding challenge in the endoplasmic reticulum, potentially activating the unfolded protein response mechanism.Citation28
The intracellular staining signal of the transfected population reflects the trends identified above. With less Ys in Intron 2 the cellular population with an excess of expression of the ORF on exon 4 decreases. In the configuration LC:HC (Figure S3), the fraction of the cellular population overexpressing light chain decreases with the decreasing number of Ys. In the configuration HC:LC (Figure S3), this decreases the fraction of the cellular population overexpressing heavy chain. It should be noted that the observed heterogeneity of the cell population (with cells expressing an excess of light chain or heavy chain, respectively) is not considered contradictory to the concept of the predetermined splice ratio. It merely reflects the lack of sensitivity of the assay for the low expressing subunit.
In summary, the modifications allowing the splice ratio in the GFP/dsRED-expressing constructs to be shifted also allowed a shift of the mRNA levels coding for heavy and light chain of the anti-HER2 antibody. This resulted (as only assembled antibody was measured) in a shift in expression levels. The threshold in Ys allowing significant expression may be related to a required minimum level of both subunits that allows efficient assembly and secretion of antibody or to the length of the ORFs of the different subunits. The shortening of Intron 3 seems to have no or little impact on this ratio. This result is in contrast to what was observed for DsRed and GFP constructs, highlighting again the importance of post-translational events, such as multimer assembly, which is not required with the fluorescent markers. The highest expression levels were obtained with the heavy chain on Exon 3 and light chain in Exon 4 using construct cTNT-I4(3Y) in Intron 2 and the shortened cTNT-I4(sh) in Intron 3. High expression was also achieved with light chain on Exon 3 and heavy chain on Exon 4. The best construct here was cTNT-I4(0Y) in Intron 2 and cTNT-I4 in Intron 3. This expression construct was cloned into the expression vector used for stable cell-line development. A reevaluation of intron-exon consensus sequence and branch-point mutations did not allow further increases in the expression levels (see supplementary Figure S4). It is worth noting that the best construct (combination of ORF orientation, poly(Y) strength, shortening of Intron 3) might differ from one antibody to the other (due to different optimal ratios between heavy chain and light chain) and thus needs to be identified and fine-tuned on a case-to-case basis.
Alternative splicing is enhanced if the alternative exon is flanked by similar introns, independently from the intron sequence
The use of the same intron sequence upstream and downstream of the alternative exon was found to shift the splice ratio toward higher levels of protein expression from the second ORF. To assess whether this is only true for introns naturally involved in alternative splicing, a constitutive intron from the human EF1alpha geneCitation29 was used for the expression of the anti-HER2 antibody. The EF1alpha intron was cloned in Intron 2 and Intron 3. Intermediate constructs with EF1alpha in Intron 2 and cTNT-I4 in Intron 3 were cloned as well. Constructs with identical introns flanking the alternative exon 5’ and 3’ show higher expression levels compared with constructs having different introns, independent on whether the heavy or light chains of the anti-HER2 antibody are expressed on the alternative exon (). Using the cTNT introns, the expression level is higher compared to the EF1alpha introns. It can be speculated that introns involved naturally in alternative splicing allow higher expression of the second exon and better expression of multimeric proteins like antibodies. This insight may be useful in order to design alternative constructs.
Creation of stable cell lines expressing anti-HER2 antibody
In order to obtain stable expression of the anti-HER2 antibody in CHO cells, vector pGLEX41-2 G-anti-HER2-LC-HC with cTNT-I4(0Y) in Intron 2 and cTNT-I4 in Intron 3 in orientation LC-HC was used to generate a stable pool population using PiggyBac-mediated transfection. This pool was assessed in a 14-day supplemented batch. The highest titer obtained at the end of the batch culture was 1.3 g/L (see ). These titers represent the first industrially relevant production level of a stable cell line producing an antibody based on alternative splicing technology. Further improvements to this titer may be possible by optimizing the cell line (e.g., clonal derivation) and upstream process development process.Citation30
Product quality
Transient and stable material was generated with cTNT-I4(0Y) in Intron 2 and cTNT-I4 in Intron 3 in orientation LC-HC. The quality of purified antibody material (glycosylated and aglycosylated) was assessed by mass spectrometry ( for stable material and Figure S5 for transient material) and compared to product manufactured using a monocistronic expression cassette in a commercial fed-batch process.
Figure 7. Intact mass measurement by mass spectrometry comparing glycosylated (a) and aglycosylated (b) anti-HER2 antibody generated using alternative splicing and glycosylated (c) and aglycosylated (d) anti-HER2 antibody generated in traditional fed-batch. The measured molecular masses matched the expected ones for the anti-HER2 antibody, with its major N-glycosylations (G0F/G1F for anti-HER2 antibody generated in traditional fed-batch and G0F/G0F for the anti-HER2 antibody generated using alternative splicing) and major post-translational modifications (C-terminal lysine clipped, Glycation), within an accepted error of ±5 Da (±50 ppm).
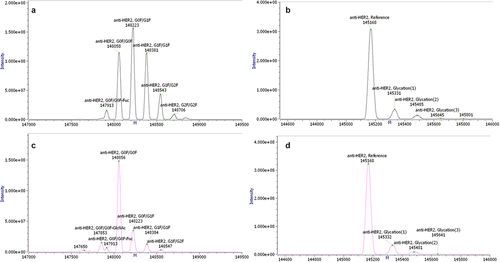
The measured molecular masses matched the expected ones for the anti-HER2 molecule, with its major N-glycosylations and major post-translational modifications (C-terminal lysine clipped, C-terminal glycine loss and proline amidation,Citation31,Citation32 glycation), within an accepted error of ±5 Da (±50 ppm). Importantly, the measured intact mass confirmed the presence of only fully assembled antibody with correct primary sequence. No antibody variants that may be the result of erroneous aberrant splicing could be detected.
Stability of expression
The pool population was cultivated for 28 days (this corresponds to approximately 29 population doubling levels). The relative expression of the two splice variants (mRNA1/mRNA2) was assessed using cells harvested on day 0, 14 and 28. As shown in , there is no statistically significant change in splice ratio over time (Tukey–Kramer test), confirming the initial observations made using the fluorescent constructs.
Expression of bispecific antibodies using alternative splicing constructs
In order to assess the expression of bispecific antibodies with the alternative splicing construct described here, a bispecific antibody of the BEAT® format was chosen. The bispecific antibody used in this experiment is of the [Fab’scFv]-heteroFc typeCitation33 (for sequences, see Table S8) composed of the three subunits heavy chain, light chain (OKT3) and the Fc-scFv (binder against HER-2) using the BEAT® interfaceCitation34 for efficient heterodimerization. The different subunits have to be expressed at a certain molar ratio in order to get the best possible expression and heterodimerization levels.
Heavy chain and the Fc-scFv were cloned in both orientations into the alternative splicing construct carrying cTNT-I4(7Ynude) in Intron 2 and cTNT4-I4(sh) in Intron 3. The vectors with the alternative splicing construct and the vector for the light chain were co-transfected in CHO cells using a three-fold excess of the vector coding for the light chain over the vector coding for the alternate splicing construct. The expression levels of resulting Protein A binding species are shown in . The presence of bispecific antibodies was confirmed by ELISA (specific for the two arms of the bispecific antibody).
Figure 9. Expression level of the anti-CD3/anti-HER-2 bispecific antibody in transient transfection. (A) Schematic drawing of the bispecific antibody. (B) The alternative splicing construct in orientation HC:Fc-scFv (black) or in orientation Fc-scFv:HC (yellow) was co-transfected at a threefold molar excess of light chain expression vector to alternative splicing vector.
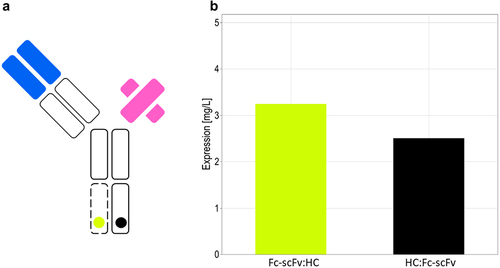
While this proof-of-concept experiment demonstrates that bispecific antibodies can be expressed using splice constructs, further optimization would be required to identify the best construct with regard to product quality and quantity. No optimization was performed to optimize the ratio in the splice constructs, for example, by varying the poly(Y) tract in Intron 2, which may prove useful to shift the ratio for higher expression or lower levels of contaminating by-products.
Discussion
This work describes the development of an alternative splicing construct that allows the expression of two proteins at defined ratios from a single pre-mRNA with the purpose of expressing multimeric proteins like monoclonal antibodies. The basic construct is composed of 4 exons and 3 introns. Exon 1 and Exon 2 contain the 5’untranslated region (5’UTR), Exon 3 contains a first ORF and Exon 4 a second ORF. Intron 1 is constitutively cut out by the splice machinery of the cell, whereas the following intron may exclude (Intron 2) or include Exon 3 (Intron 2’) from the final mRNA depending on the alternative splicing event that is occurring.
First, DsRed and GFP were used as reporter proteins in Exon 3 and 4, respectively, and it was shown that the expression construct allowed the generation of mRNAs coding for both fluorescent proteins using alternative splicing. The basic construct (cTNT-I4 in Intron 2 and cTNT-I5 in Intron 3) showed a preference for inclusion of the alternative exon and expressed mainly DsRed (coding sequence present on Exon 3).
The shift in expression from DsRed to GFP by modifications in the poly(Y) expected according to data described in the literatureCitation21 could not be achieved. Xu et al.Citation35 described that artificial exons smaller than 49 nucleotides are not recognized by the splice machinery if they lack a splice enhancer element (not present in the exons used in this study) and they demonstrate that exons with a size between 49 and 119 nucleotides are alternatively spliced, albeit at lower efficiency with increasing exon size. The Exon with DsRed used in this study had a size of 718 nucleotides (6 times the maximum exon size analyzed by Xu et al.). Therefore, the observed preference for expression of the first exon might be simply due to the size of the exon.
Flanking the alternative exon (containing the first ORF) with the same intron up- and downstream, in particular with the cTNT-I4, allowed the expression of both ORFs to equilibrate. This interesting observation was confirmed with three different introns (cTNT-I4, cTNT-I5 and the EF1alpha first intron), including a constitutively removed intron (EF1alpha). For cTNT-I4, this allowed a shift in the splice ratio toward higher expression of the second ORF by reducing the amount of Ys in the poly(Y) tract of Intron 2. The splice event is heavily influenced by proteins binding to introns. It could be speculated that having the same sequences and protein-binding sites on the introns flanking the alternative intron removes all bias based on the different splice factors and renders the splice event more susceptible for variations in splice acceptor strength. Finally, the deletion of the splice donor site downstream of the alternative exon (Intron 3 in our construct) led to further exclusion of the alternative exon. The cells transfected with the DsRed/GFP constructs with the splice donor deletion seemed to express mainly GFP.
These different modifications of intron sequences allowed generation of a pool of alternative splicing constructs covering both extremes of alternative splicing (mainly inclusion of the alternative exon to mainly exclusion of the alternative exon), as well as intermediate ratios.
Similar observations were made with expression constructs coding for a protein requiring assembly from two subunits, the anti-HER2 antibody. Using the cTNT-I4 Intron in Intron 2 and Intron 3, reducing the strength of the poly(Y) tract of Intron 2 (and removing the splice donor of intron 3) allowed a reduction in the amount of mRNA1 (expressing Exon 3) and an increase in the amount of mRNA 2 (expressing Exon 4). As a result, the expression of heavy chain and light chain becomes more balanced, leading to a clear increase in the expression level. This tuning of the expression of subunits to achieve secretion of increasing amounts of fully assembled antibody was achieved with constructs carrying the subunits in both orientations (HC-LC or LC-HC). Within this study, only one antibody was expressed. Different antibodies may have a different optimal ratio of heavy chain to light chain, which may influence the expression levels observed with the alternative splicing constructs in this study.
The stability of the splice event over time was assessed using stable cell lines expressing DsRed and GFP. Mini pools and clones generated with the same construct showed similar splice ratios of GFP and DsRed, indicating that the splice ratio is stable over multiple generations. This was confirmed with a cell pool expressing the anti-HER antibody. A 28-day stability study showed no statistically significant changes in the splice ratio.
The same stable pool allowed titers of 1.3 g/L in a non-optimized fed-batch. Process improvements may further increase the current titer (e.g., clonal derivation, optimized fed-batch process). This titer is at the upper end compared to other polycistronic technologies and can be considered within the range, albeit at the lower end, reported for industrial production of antibody constructs.Citation36,Citation37 With regard to product quality, the primary sequence of the assembled product was fully congruent with the theoretical sequence, demonstrating that the alternative splice events are highly controlled without detectable side products from aberrant splicing. The antibody was fully assembled, demonstrating that the two mRNAs can be successfully translated. Alternate splicing is located in the nucleus. Therefore, an assessment of transiently generated material seemed adequate, as there is no difference in processing compared to stable integration, and no other post-translational product quality attributes were assessed, as these merely depend on the activity cellular enzymes (e.g., terminal lysine removal, glycosylation), the chemical environment, or the residence time prior to harvest (e.g., glycation, deamidation).
In contrast to monocistronic constructs, polycistronic constructs offer a simplified vector architecture because there is only one expression cassette. This enables strategies related to controlled expression, which may be used for expression of toxic products. A unique advantage of the presented alternate splicing constructs is that they allow tuning of the expression of subunits, which may be needed, for example, because an excess of light chain is required for efficient expression.
With these features, the proposed bicistronic construct seems to be of interest for high-throughput screening and antibody engineering applications, where researchers need to rapidly express and evaluate multiple-antibody variants that need to have a specific ratio of subunits. For such a use, the expression levels are in a range that could be considered for early clinical supply and would thus also support a very rapid transition from discovery to clinical stages.
Other than for the expression of single IgGs, the construct may be used for the expression of subunits of bispecific antibodies, as exemplified with a BEAT® antibody targeting CD3 and HER-2. There may be a potential use in the generation of multispecific CAR constructs, as shown in the recent development of a bicistronic anti-CD19/CD20 CAR construct.Citation38 Also, such a construct may allow expression of a mix of two antibodies (or even a mix of bispecific antibodies) in the same cell population (by carrying heavy chain 1 and heavy chain 2 on the alternative splicing construct). The advantage here would be that the ratio of antibody 1 to antibody 2 would be defined by the molecular construct, overcoming a problem in the generation of antibody mixtures.Citation39
As mentioned previously, further optimization work may allow increases in the expression levels of the construct and it may allow the limitation of allowing only bicistronic expression to be overcome. If titer levels are not of concern, it may be possible to add further exons to the expression cassette to build not only bicistronic, but tricistronic constructs, allowing, for example, expression of all three subunits of a bispecific antibody similar to the CD3/HER-2 BEAT® expressed as part of the study.
In summary, our data suggest that the described expression construct with an alternate exon flanked by identical introns (in particular introns based on cTNT-I4) allows tuneable alternative splicing. The presented bicistronic construct is believed to be useful for high-throughput screening and antibody engineering applications requiring rapid expression and evaluation of multiple antibody variants that need to have a specific ratio of subunits. The expression levels are in a range that could be considered for early clinical supply allowing a rapid transition from discovery to clinical stages.
KMAB_2023_0114R4_supp material.docx
Download MS Word (40.2 KB)Acknowledgments
The authors would like to thank Dr David Hacker for critically reading the manuscript and Dr Maria Tsachaki for valuable technical support.
Disclosure statement
No potential conflict of interest was reported by the author(s).
Supplemental material
Supplemental data for this article can be accessed online at https://doi.org/10.1080/19420862.2024.2342243
Additional information
Funding
References
- Dorai H, Csirke B, Scallon B, Ganguly S. Correlation of heavy and light chain mRNA copy numbers to antibody productivity in mouse myeloma production cell lines. Hybridoma (Larchmt). 2006;25(1):1–14. doi:10.1089/hyb.2006.25.1. PMID: 16475875.
- Schlatter S, Stansfield SH, Dinnis DM, Racher AJ, Birch JR, James DC. On the optimal ratio of heavy to light chain genes for efficient recombinant antibody production by CHO cells. Biotechnol Progress. 2005;21(1):122–33. doi:10.1021/bp049780w.
- Ho SCL, Koh EYC, van Beers M, Mueller M, Wan C, Teo G, Song Z, Tong YW, Bardor M, Yang Y. Control of IgG LC: HC ratio in stably transfected CHO cells and study of the impact on expression, aggregation, glycosylation and conformational stability. J Biotechnol. 2013;165(3–4):157–66. doi:10.1016/j.jbiotec.2013.03.019.
- Blanco N, Williams AJ, Tang D, Zhan D, Misaghi S, Kelley RF, Simmons LC. Tailoring translational strength using Kozak sequence variants improves bispecific antibody assembly and reduces product-related impurities in CHO cells. Biotechnol Bioeng. 2020;117:1946–60. doi:10.1002/bit.27347. PMID: 32246763.
- Von Kreudenstein TS, Escobar-Carbrera E, Lario PI, D’Angelo I, Brault K, Kelly J, Durocher Y, Baardsnes J, Woods RJ, Xie MH. et al. Improving biophysical properties of a bispecific antibody scaffold to aid developability: quality by molecular design. Mabs-austin. 2013;5(5):646–54. doi:10.4161/mabs.25632. PMID: 23924797.
- Rajendra Y, Peery RB, Hougland MD, Barnard GC, Wu X, Fitchett JR, Bacica M, Demarest SJ. Transient and stable CHO expression, purification and characterization of novel hetero-dimeric bispecific IgG antibodies. Biotechnol Prog. 2017;33:469–77. doi:10.1002/btpr.2414. PMID: 27977915.
- Matasci M, Baldi L, Hacker DL, Wurm FM. The PiggyBac transposon enhances the frequency of CHO stable cell line generation and yields recombinant lines with superior productivity and stability. Biotechnol Bioeng. 2011;108:2141–50. doi:10.1002/bit.23167. PMID: 21495018.
- Chng J, Wang T, Nian R, Lau A, Hoi KM, Ho SC, Gagnon P, Bi X, Yang Y. Cleavage efficient 2A peptides for high level monoclonal antibody expression in CHO cells. Mabs-austin. 2015;7(2):403–12. doi:10.1080/19420862.2015.1008351.
- Ho SCL, Bardor M, Feng H, Tong YW, Song Z, Yap MGS, Yang Y. IRES-mediated Tricistronic vectors for enhancing generation of high monoclonal antibody expressing CHO cell lines. J Biotechnol. 2012;157(1):130–39. doi:10.1016/j.jbiotec.2011.09.023.
- Cooper TA, Ordahl CP. A single cardiac troponin T gene generates embryonic and adult isoforms via developmentally regulated alternate splicing. J Biol Chem. 1985;260(20):11140–48. doi:10.1016/S0021-9258(17)39158-5. PMID: 2993302.
- Dominski Z, Kole R. Selection of splice sites in pre-mRnas with short internal exons. Mol Cell biol. 1991;11(12):6075–83. doi:10.1128/MCB.11.12.6075. PMID: 1944277.
- Ladd AN, Charlet N, Cooper TA. The CELF family of RNA binding proteins is implicated in cell-specific and developmentally regulated alternative splicing. Mol Cell biol. 2001;21:1285–96. doi:10.1128/MCB.21.4.1285-1296.2001. PMID: 11158314.
- Ho TH, Charlet BN, Poulos MG, Singh G, Swanson MS, Cooper TA. Muscleblind proteins regulate alternative splicing. Embo J. 2004;23(15):3103–12. doi:10.1038/sj.emboj.7600300. PMID: 15257297.
- Cooper TA. In vitro splicing of cardiac troponin T precursors. Exon mutations disrupt splicing of the upstream intron. J Biol Chem. 1992;267(8):5330–38. doi:10.1016/S0021-9258(18)42770-6. https://www.ncbi.nlm.nih.gov/pubmed/1544914 PMID: 1544914.
- Cooper TA, Ordahl CP. Nucleotide substitutions within the cardiac troponin T alternative exon disrupt pre-mRNA alternative splicing. Nucleic Acids Res. 1989;17(19):7905–21. doi:10.1093/nar/17.19.7905.
- Cooper TA, Cardone MH, Ordahl CP. Cis requirements for alternative splicing of the cardiac troponin T pre–mRNA. Nucl Acids Res. 1988;16(17):8443–65. https://www.ncbi.nlm.nih.gov/pubmed/3419923. PMID: 3419923.
- Cooper TA. Use of minigene systems to dissect alternative splicing elements. Methods. 2005;37(4):331–40. doi:10.1016/j.ymeth.2005.07.015. PMID: 16314262.
- Dominski Z, Kole R. Cooperation of pre-mRNA sequence elements in splice site selection. Mol Cell biol. 1992;12(5):2108–14. doi:10.1128/MCB.12.5.2108. PMID: 1569943.
- Aebischer-Gumy C, Moretti P, Ollier R, Ries Fecourt C, Rousseau F, Bertschinger M. SPLICELECT™: an adaptable cell surface display technology based on alternative splicing allowing the qualitative and quantitative prediction of secreted product at a single-cell level. Mabs-austin. 2020;12(1):1709333. doi:10.1080/19420862.2019.1709333.
- Lucas BK, Giere LM, DeMarco RA, Shen A, Chisholm V, Crowley CW. High-level production of recombinant proteins in CHO cells using a dicistronic DHFR intron expression vector. Nucleic Acids Res. 1996;24:1774–79. https://www.ncbi.nlm.nih.gov/pubmed/8649999. PMID: 8649999.
- Fallot S, Ben Naya R, Hieblot C, Mondon P, Lacazette E, Bouayadi K, Kharrat A, Touriol C, Prats H. Alternative-splicing-based bicistronic vectors for ratio-controlled protein expression and application to recombinant antibody production. Nucleic Acids Res. 2009;37(20):e134. doi:10.1093/nar/gkp716. PMID: 19729510.
- Prentice inventor. Methods and constructs for expressing polypeptide multimers in eukaryotic cells using alternative splicing.
- Aebischer-Gumy C, Bertschinger M, Moretti P (Inventors). Glenmark pharmaceuticals SA/Ichnos sciences SA s assignee.^assignees. Expression constructs and methods for expressing polypeptides in eukaryotic cells. WO2015018832.
- Gorman CM, Gies DR, McCray G. Transient production of proteins using an adenovirus transformed cell line. DNA Protein Eng Tech. 1990;2:1–28.
- Bell MV, Cowper AE, Lefranc MP, Bell JI, Screaton GR. Influence of intron length on alternative splicing of CD44. Mol Cell biol. 1998;18(10):5930–41. doi:10.1128/MCB.18.10.5930. PMID: 9742110.
- Feige MJ, Buchner J. Principles and engineering of antibody folding and assembly. Biochim Biophys Acta. 2014;1844(11):2024–31. doi:10.1016/j.bbapap.2014.06.004. PMID: 24931831.
- Spooner J, Keen J, Nayyar K, Birkett N, Bond N, Bannister D, Tigue N, Higazi D, Kemp B, Vaughan T. et al. Evaluation of strategies to control Fab light chain dimer during mammalian expression and purification: A universal one-step process for purification of correctly assembled Fab. Biotechnol Bioeng. 2015;112(7):1472–77. doi:10.1002/bit.25550. PMID: 25619171.
- Read A, Schroder M. The unfolded protein response: an overview. Biology (Basel). 2021;10(5):384. doi:10.3390/biology10050384. PMID: 33946669.
- Running Deer J, Allison DS. High-level expression of proteins in Mammalian Cells using transcription regulatory sequences from the Chinese Hamster EF-1α Gene. Biotechnol Prog. 2004;20(3):880–89. doi:10.1021/bp034383r. PMID: 15176895.
- Peltret M, Vetsch P, Farvaque E, Mette R, Tsachaki M, Frank J, Vaxeliare E, Moritz B, Aillerie C, Giovannini R. et al. Development of a 10 g/l process for a difficult-to-express multispecific antibody format using a holistic process development approach. Submitted To J Biotechnol. 2023.
- Tsubaki M, Terashima I, Kamata K, Koga A. C-terminal modification of monoclonal antibody drugs: amidated species as a general product-related substance. Int J Biol Macromol. 2013;52:139–47. doi:10.1016/j.ijbiomac.2012.09.016. PMID: 23022270.
- Beck A, Liu H. Macro- and micro-heterogeneity of natural and recombinant IgG antibodies. Antibodies (Basel). 2019;8(1):18. doi:10.3390/antib8010018. PMID: 31544824.
- Biswas R, Belouski E, Graham K, Hortter M, Mock M, Tinberg CE, Russell AJ. VERITAS: Harnessing the power of nomenclature in biologic discovery. Mabs-austin. 2023;15(1):2207232. doi:10.1080/19420862.2023.2207232. PMID: 37162235.
- Skegro D, Stutz C, Ollier R, Svensson E, Wassmann P, Bourquin F, Monney T, Gn S, Blein S. Immunoglobulin domain interface exchange as a platform technology for the generation of Fc heterodimers and bispecific antibodies. J Biol Chem. 2017;292(23):9745–59. doi:10.1074/jbc.M117.782433. PMID: 28450393.
- Xu R, Teng J, Cooper TA. The cardiac troponin T alternative exon contains a novel purine-rich positive splicing element. Mol Cell biol. 1993;13(6):3660–74. doi:10.1128/MCB.13.6.3660. PMID: 8388541.
- Guo C, Chen F, Xiao Q, Catterall HB, Robinson JH, Wang Z, Mock M, Hubert R. Expression liabilities in a four-chain bispecific molecule. Biotechnol Bioeng. 2021;118(10):3744–59. doi:10.1002/bit.27850. PMID: 34110008.
- Magistrelli G, Poitevin Y, Schlosser F, Pontini G, Malinge P, Josserand S, Corbier M, Fischer N. Optimizing assembly and production of native bispecific antibodies by codon de-optimization. Mabs-austin. 2017;9(2):231–39. doi:10.1080/19420862.2016.1267088. PMID: 28001485.
- Lam N, Finney R, Yang S, Choi S, Wu X, Cutmore L, Andrade J, Huang L, Amatya C, Cam M. et al. Development of a bicistronic anti-CD19/CD20 CAR construct including abrogation of unexpected nucleic acid sequence deletions. Mol Ther Oncolytics. 2023;30:132–49. doi:10.1016/j.omto.2023.07.001. PMID: 37654973.
- Rasmussen SK, Naested H, Muller C, Tolstrup AB, Frandsen TP. Recombinant antibody mixtures: production strategies and cost considerations. Arch Biochem Biophys. 2012;526(2):139–45. doi:10.1016/j.abb.2012.07.001. PMID: 22820097.