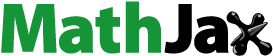
Abstract
To investigate cannabinoid content and profiles, 16 cannabinoids were quantified in 30 commercial hemp seed edible oils. In addition, one hemp seed oil was subjected to thermal processing up to 200 °C for up to 60 min. UHPLC-MS/MS was used for analysis. The content of cannabinoids in the samples ranged from 9 to 279 mg kg−1 (sum) and for Δ9-tetrahydrocannabinol (Δ9-THC) from 0.2 to 6.7 mg kg−1. Three samples exceeded the EU Δ9-THC equivalent maximum levels of 7.5 mg kg−1 for hemp seed oils. Cannabinoid profiles can provide indications of different product characteristics (e.g. degree of processing, variety of plant material). Furthermore, intense thermal processing (200 °C, 60 min) led to 38% decrease in sum cannabinoid content (sum of all analysed cannabinoids in this study), 99% decrease in cannabinoid acids, and 22% increase in Δ9-THC.
Introduction
Hemp, botanically known as Cannabis sativa L., is a culturally important crop. As part of its secondary metabolism, C. sativa produces phytocannabinoids, of which 130 compounds have been identified in C. sativa extracts (Lange and Zager Citation2021). The predominant products of the biosynthetic pathway are cannabigerolic acid (CBGA), cannabidiolic acid (CBDA), tetrahydrocannabinolic acid (THCA) and cannabichromenic acid (CBCA). Cannabinoid acids are formed in glandular trichomes, which are found in large quantities in the female flower, but also in the leaves and other organs (Tahir et al. Citation2021). The testa of hemp seeds is generally not covered with trichomes and thus, the seeds are virtually free of cannabinoids. However, during harvest, they may come into contact with other plant parts covered with cannabinoid-containing resin and thus, become contaminated with cannabinoids on the testa (Ross et al. Citation2000).
Hemp-containing food has been a trend in Germany for years (Dräger et al. Citation2022). Data from the German National Monitoring Programme for food, compiled by the German Federal Office of Consumer Protection and Food Safety (BVL), reveal that the sample numbers for hemp-containing foods increased from 34 samples in 2015 to 466 samples in 2019 (FragDenStaat Citation2021). A wide range of food products containing hemp can be found in grocery stores, with flours, seeds and edible oils accounting for an important share of the market (Lachenmeier, Bock, et al. Citation2019; Lachenmeier, Habel, et al. Citation2019). Hemp seed edible oils (hereafter: hemp seed oils) in particular are an established product on the German market and have been available since 1995 (Lachenmeier et al. Citation2004). Due to the fatty acid profile and high levels of antioxidants, hemp seed oil is considered potentially health-promoting (Da Porto et al. Citation2012; Teh and Birch Citation2013).
In the plant, cannabinoids are mostly present as carboxylic acids, which decarboxylate to neutral cannabinoids mainly under thermal influence (Gülck and Møller Citation2020). The decarboxylation of THCA, extensively studied in plant material, is particularly important as it yields the psychoactive Δ9-THC (Perrotin-Brunel et al. Citation2011; Wang et al. Citation2016; Moreno et al. Citation2020; Gigopulu et al. Citation2022; Seo et al. Citation2022). Δ9-THC and cannabidiol (CBD) are important neutral cannabinoids with partially opposite pharmacological effects. However, of the two, only Δ9-THC is considered to have psychoactive relevance (Schwilke et al. Citation2009; Bhattacharyya et al. Citation2010; Lachenmeier, Bock, et al. Citation2019; Lachenmeier, Habel, et al. Citation2019). Therefore, Δ9-THC has been restricted with maximum levels, calculated as Δ9-THC equivalents (sum of neutral and decarboxylated acidic form: Δ9-THC + 0.877*THCA) in the EU since 1 January 2023. The levels in edible hemp seeds and products derived thereof are restricted to 3.0 mg kg−1 and hemp seed oils to 7.5 mg kg−1 (European Commission Citation2022, Citation2023b).
By studying the cannabinoid profile of hemp, it is possible to differentiate industrial (CBDA dominant) and medicinal (THCA dominant) varieties (Andre et al. Citation2016). The ratio of the content of psychoactive, incl. cannabinol (CBN), and non-psychoactive cannabinoids, using the equation (Δ9-THC + CBN)/CBD, the so-called phenotype ratio, can be used to distinguish industrial (ratio < 1) from medicinal hemp (ratio > 1) (United Nations Office on Drugs and Crime, Citation2022).
Gas chromatographic (GC) separation of cannabinoids is possible, but due to high temperatures in GC, acidic and neutral cannabinoids cannot be distinguished without derivatisation (Fodor and Molnár-Perl Citation2017). Thermal decarboxylation does not occur in liquid chromatography (LC) due to lower temperatures, thus allowing differentiation of acidic and neutral cannabinoids without derivatisation (Cardenia et al. Citation2018). Coupling LC with tandem mass spectrometry (MS/MS) offers high sensitivity, specificity and low limits of detection and quantification (Capriotti et al. Citation2021) and is therefore often used in recent methods dealing with food matrices such as beverages, hemp seeds or hemp seed oil (Di Marco Pisciottano et al. Citation2018; Christinat et al. Citation2020).
Previous studies on cannabinoids showed various limitations in regard to food safety concerns. On the one hand, phytochemical or forensic issues are prioritised and the focus of the analyses is placed on plants or plant material (Ross and Elsohly Citation1997; Lange and Zager Citation2021; Meija et al. Citation2022). On the other hand, analyses in food matrices are often limited to a limited profile of cannabinoids, usually consisting of only CBD, THC, CBN and their corresponding acidic forms (Petrović et al. Citation2015; Jang et al. Citation2020; Kladar et al. Citation2021) or a small number of typical food samples (e. g. oils, seeds, protein isolates, n = 1–10) (Citti et al. Citation2019; Christinat et al. Citation2020). Several studies were not able to distinguish between acidic and neutral cannabinoids due to the nature of GC analysis (Petrović et al. Citation2015; Jang et al. Citation2020; Kladar et al. Citation2021). In addition, the decarboxylation of cannabinoid acids has been studied mainly in plant material (Gigopulu et al. Citation2022; Meija et al. Citation2022; Seo et al. Citation2022) or in hemp seed oil, focusing only on individual cannabinoid acids and reaction kinetics (Citti et al. Citation2018).
In this study, it was hypothesised that thermal processing of commercial hemp seed oils at temperatures between 130 °C and 200 °C for 10–60 min will lead to transformation reactions of acidic and neutral cannabinoids (e.g. decarboxylation, oxidation), resulting in changes in the cannabinoid profile. Specifically, we expected to observe alterations in THCA and Δ9-THC ratios and contents. By quantifying 16 cannabinoids in both native and processed hemp seed oils, we aim to provide a comprehensive overview of cannabinoid profiles that may not have previously been focused on. Overall, it was intended to provide data that can be used for risk assessment purposes of hemp seed oil and other hemp-containing foods.
Material and methods
Chemicals
Cannabinoids were purchased as certified reference material dissolved in methanol at a concentration of 1 mg mL−1, unless otherwise noted. Cannabichromene (CBC, >98%), CBCA (>95%), CBD (>99%), CBDA (>98%), cannabidivarin (CBDV, >99%), cannabidivarinic acid (CBDVA, > 98%), cannabigerol (CBG, >99%), CBGA, (>99%), cannabicyclol (CBL, >99%), cannabicyclolic acid (CBLA, >98%, 0.5 mg mL−1), CBN, (>99%), cannabinolic acid (CBNA, >98%), Δ9-tetrahydrocannabinol (Δ9-THC, >94%, 100 µg mL−1 in heptane), (THCA, >99%, in acetonitrile), tetrahydrocannabidivarin (THCV, > 98%), tetrahydrocannabidivarinic acid (THCVA, >92%), CBD-D3 (>99%, 100 µg mL−1) and THCA-D3 (>98%, 100 µg mL−1) were purchased from Sigma-Aldrich Corp. (St. Louis, MO). CBN-D3 (> 97%, 100 µg mL−1) and Δ9-tetrahydrocannabinol-D3 (>98%, 100 µg mL−1) were purchased from Th. Geyer GmbH & Co. KG (Berlin, Germany). Structures for all these cannabinoids are shown in Figure S1. Acetonitrile (‘Hypergrade for LC-MS’) and acetone (‘for liquid chromatography’) were purchased from Merck KGaA (Darmstadt, Germany). Purified water was prepared with a Milli-Q® purification system with a Millipak® Express 20 (0.22 µm) filter (Merck KGaA, Darmstadt, Germany). Formic acid was purchased from Sigma-Aldrich Chemie GmbH (Taufkirchen, Germany).
Preparation of standard solutions
Reference standards for each cannabinoid were available. The individual analytes were obtained in dissolved form in ampoules. Stock solutions with concentrations of 10–100 µg mL−1 (depending on the respective analyte) were prepared for each analyte from the supplied ampoules. A standard working solution containing each analyte at a concentration of 1000 ng mL−1 was prepared from the stock solutions of the individual analytes. Acetonitrile was used for dilution.
Four isotope-labelled reference standards were available: CBD-D3, CBN-D3, Δ9-THC-D3 and THCA-D3. The individual isotope-labelled substances were obtained in dissolved form in ampoules. Stock solutions with concentrations of 10 µg mL−1 were prepared for each analyte from the supplied ampoules. A working solution containing each substance at a concentration of 500 ng mL−1 was prepared from the stock solutions of each substance by dilution with acetonitrile. The working solution was added to measurement vials to monitor the MS device performance.
Sample preparation
A total of 30 commercially available hemp seed oils were randomly purchased in online-shops, supermarkets, drug stores, pharmacies and hemp product shops in Germany. According to the product declaration, oils originated from Germany (n = 22), Czech Republic (n = 4), Austria (n = 3) and Lithuania (n = 1).
The sample preparation approach used in this study is based on an analytical method for the quantification of cannabinoids in edible oils proposed for standardisation within the framework of §64 of the German Food and Feed Code (LFGB) (working group ‘Plant Toxins’) hosted by the Federal Office of Consumer Protection and Food Safety (BVL) (Amtliche Sammlung von Untersuchungsverfahren nach §64 LFGB Citation2024). The same sample preparation was used both for commercial and thermally processed hemp seed oils. Approximately 200 mg of oil were accurately weighed into a 10 mL volumetric flask. Vials containing heated oil samples were vortexed for 5 s beforehand. The volumetric flask was filled to the calibration mark with acetone and vortexed for 10 s. The solutions were diluted five- and ten-fold by placing 10 µL of isotope-labelled reference standard working solution in a HPLC vial (to monitor MS device performance), adding 200 or 100 µL of solution, and then diluting with 790 or 890 µL of acetonitrile/water (50/50 v/v). The 10-fold dilution was used in addition to the usual 5-fold dilution in order to be able to quantify some highly concentrated cannabinoids such as CBD. The exact sample weight was employed for the quantification calculations.
Thermal processing
For thermal processing of the hemp seed oils, approximately 700 mg of oil were weighed into an HPLC vial. Depending on the experiment, vials were either capped or remained open during heating. The vials were heated in a drying oven (Heraeus UT 6120) with the airflow turned on. The oven was preheated to each selected temperature (130 °C, 160 °C, 180 °C and 200 °C) for 1 h. At each temperature, three vials were heated for 10, 30 and 60 min, removed from the oven, and cooled down to room temperature for approximately 1 h. The non-capped vials were then capped after cooling down. All vials were stored at room temperature until further use in the following two days.
Calibration and quality control
A calibration series was prepared consisting of 11 solutions covering a range of 0.1–100 ng mL−1. After incorporating a fixed volume of isotope-labelled reference standard working solution (to monitor MS device performance) in HPLC vials, standard working solution was added and the necessary volume of acetonitrile/water (50/50, v/v) was added to give a total volume of 1000 µL. For example, to produce a calibration solution with a concentration of 10 ng mL−1, 10 µL of isotope-labelled reference standard working solution, 10 µL of standard working solution, and 980 µL of acetonitrile/water were mixed. The concentrations of the calibration solutions were 0.1, 0.25, 0.5, 1, 2.5, 5, 10, 25, 50, 75 and 100 ng mL−1 (0.005–5 mg kg−1). The concentration range used for the external calibration was substance-specific, starting at the lowest concentration corresponding to the limit of quantification (LOQ) () up to 100 ng mL−1 (5 mg kg−1). The linearity of the cannabinoid-specific calibration function was checked within each measurement sequence. Quantification was performed using the external calibration series. Peak areas for the analytes in the calibration solutions were plotted against their respective concentrations, and a weighted linear regression analysis was employed to establish a calibration curve. Concentrations of the analytes in the samples were then obtained by substituting the respective peak areas in the linear calibration function. Additionally, a quality control sample (QC) was prepared. The QC was a fortified sunflower seed oil sample. To prepare, 200 mg of oil were weighed into a 10 mL volumetric flask. Then, 200 µL of standard working solution were added and the volumetric flask was filled to the calibration mark with acetone and vortexed for 10 s. Finally, 200 µL of this extract, 10 µL of isotope-labelled reference standard working solution and 790 µL of acetonitrile/water (50/50, v/v) were added to a measuring vial resulting in a concentration of 4 ng mL−1 for each analyte. The recoveries of cannabinoids were considered acceptable between 80% and 130% in accordance with the guideline followed (European Commission Citation2023a). No concentration corrections were made for the cannabinoids with regards to the recovery. Furthermore, a 5- and 10-fold dilution of the QC made it possible to verify that no matrix effects influenced the measurements. The quality control parameters are listed in . As additional identification parameters, the RT tolerance (±0.1 min) and the ion ratio (±30%) were checked in each sequence according to the Guidance document on identification of mycotoxins and plant toxins in food and feed (EURL/NRL Mycotoxin/Plant Toxin Network Citation2023).
Table 1. Overview of the quality control parameters.
UHPLC-MS/MS conditions
Chromatographic separation was performed on an Agilent 1290 Infinity II system (Agilent Technologies Inc., Santa Clara, CA). The chromatographic column was a Waters Acquity UPLC® BEH C18 (150 mm × 2.1 mm, 1.7 µm particle size) model (Waters Corp., Milford, MA). The binary gradient consisted of water (A) and acetonitrile (B), each containing 0.1% (v/v) formic acid. The gradient was as follows: 0 min with 40% B, increase to 70% B at 1.5 min, increase to 100% B at 15.5 min, decrease to 40% B at 16 min (total run time: 18 min). The eluate was diverted to waste between 0–4 min and 14–18 min. The column temperature was equilibrated at 30 °C, the injection volume was 5 µL and the flow rate was 0.3 mL min−1. The autosampler was maintained at a temperature of 10 °C. An exemplary chromatogram of a standard working solution is shown in Figure S2.
Mass spectrometric analysis was carried out on a Sciex QTRAP® 6500+ (AB Sciex LLC, Framingham, MA) triple quadrupole system. All analytes were ionised using electrospray ionisation (ESI) in positive mode. The source parameters were as follows: ion spray voltage 5500 V, capillary temperature 500 °C, curtain gas 35 mL min−1, GS1 60 mL min−1 and GS2 60 mL min−1. An overview of the substance-specific parameters of the LC-MS/MS method is shown in Table S1.
Statistical analysis
The statistical analysis of the effects of capped/uncapped, processing temperature and processing time on the cannabinoid concentration was performed by using the function lm() in R 4.2.2 (R Core Team Citation2022).
Results and discussion
Quantification of cannabinoid contents and profiles in hemp seed oils
In this study, the cannabinoid contents in hemp seed oils were analysed, revealing a range of 9.2–279.4 mg kg−1. This content is the sum of the concentrations of all 16 cannabinoids from the analyte spectrum. The mean () cannabinoid content was 63.2 mg kg−1, with a median (
) of 51.1 mg kg−1. The values presented here for profiling and contents are in agreement with recent research on the topic (Chang et al. Citation2017; Citti et al. Citation2018, Citation2019). For example, Citti et al. (Citation2018) analysed seven cannabinoids in 13 hemp seed oils and found sum cannabinoid contents similar to the ones presented here in most samples (12–68 mg kg−1). At the same time, their highest found value was seven times greater than our maximum (1960 mg kg−1), proving the high variability of the sum cannabinoid content in hemp seed oils. provides an overview of the observed cannabinoid contents in the examined samples.
Figure 1. Cannabinoid contents in mg kg−1 in the 30 investigated hemp seed oils. For each oil, the error bars represent the sum of the standard deviations for each individual cannabinoid from a duplicate workup, each measured at two dilution levels (n = 4). The individual values are listed in Table S3.

The samples with the lowest cannabinoid contents were samples No. 1 and No. 2 with contents of 9.2 and 16.2 mg kg−1, respectively. Both samples were the only hemp seed oils being produced from hulled hemp seeds according to the manufacturer declaration. Hemp seeds contain no or minimal cannabinoids, but can be contaminated with cannabinoid resin on the seed surface, if there is contact with other parts of the plant during harvest (Ross et al. Citation2000). If this seed coat is peeled off before oil pressing, it can be assumed that the cannabinoid contents in the corresponding oil are lower than in oils pressed from unpeeled seeds. The comparatively low cannabinoid contents can therefore be a first indication for the specially declared production of the hemp seed oil. Sample No. 30 had by far the highest cannabinoid content (279.4 mg kg−1). There was no noteworthy manufacturer declaration indicating any special treatment of this sample, but given the arguments above, it can be assumed that the hemp seeds were exposed to other cannabinoid-containing plant parts during harvest. Cannabinoid contents in the other samples between the extremes (sample No. 3 to No. 29) ranged from 24.2 to 114.1 mg kg−1. None of the sample declarations indicated special treatment that would influence these contents.
THCA and Δ9-THC are the most important cannabinoids in terms of psychoactive effects in humans that are present in relevant amounts in hemp seed oils. While THCA itself is not psychoactive, it can be transformed to Δ9-THC by decarboxylation. In the samples, THCA contents ranged from 0.04 to 8.1 mg kg−1 ( = 2.0 mg kg−1,
= 1.4 mg kg−1) and Δ9-THC contents from 0.1 to 6.7 mg kg−1 (
= 1.9 mg kg−1,
= 1.3 mg kg−1). The EU maximum levels for Δ9-THC in hemp seeds and products derived therefrom refer to the sum parameter ‘Δ9-THC equivalents’, which assumes the complete decarboxylation of THCA to Δ9-THC (European Commission Citation2023b). In the investigated samples, the Δ9-THC equivalents ranged from 0.3 to 13.8 mg kg−1 (
= 3.7 mg kg−1,
= 2.9 mg kg−1). Three samples exceeded the maximum levels of 7.5 mg kg−1 for Δ9-THC equivalents in hemp seed oils, namely sample No. 7 (13.8 mg kg−1), sample No. 13 (8.3 mg kg−1) and sample No. 29 (7.7 mg kg−1).
The CBDA/CBD ratio is an indicator of heating or storage of hemp and hemp products, as CBDA converts to CBD by decarboxylation, even at room temperature conditions (Citti et al. Citation2019; Meija et al. Citation2022). As all cannabinoid acids decarboxylate, the acid/neutral form ratio (A/N) can be considered in general. This A/N ratio ranged from 0.6 to 7.0 for all samples ( = 2.4,
= 1.8) as listed in detail in Table S3. The values are in agreement with previously published literature (Citti et al. Citation2019; Christinat et al. Citation2020). Sample No. 1 had the lowest A/N ratio of 0.6. This means that a higher proportion of neutral cannabinoids compared to cannabinoid acids was present. In addition, it was the only hemp seed oil declared to be made from roasted seeds. It can be assumed that the oil was exposed to significantly higher temperature stresses during production than the other oils, which are usually declared as ‘cold pressed’ (or do not contain any additional information on the production process). Therefore, thermal conversion of the cannabinoid acids to neutral cannabinoids is possible, which would be expressed in the smaller A/N ratio observed. The highest A/N ratio of 7.0 was found in sample 16, which was declared as ‘cold-pressed’ by the manufacturer. Possibly, the oil was pressed particularly gently or at a comparatively low temperature compared to other cold-pressed oils. It should be noted that ‘cold-pressed’ oils may be exposed to temperatures of up to 100 °C during production and that actual process temperatures may vary between manufacturers (Aladić et al. Citation2015). Thus, the A/N ratios determined can provide initial information on the production processes and storage conditions of hemp seed oils.
Moreover, the ratio of the content of psychoactive and non-psychoactive cannabinoids in the form of (Δ9-THC + CBN)/CBD, the so-called phenotype ratio, can be used to distinguish industrial (ratio < 1) from medical hemp (ratio > 1) (United Nations Office on Drugs and Crime Citation2022). The calculated phenotype ratios for all hemp seed oil samples are shown in Table S3. In general, the analysed hemp seed oil samples had a phenotype ratio < 1, indicating the use of hemp seeds from industrial hemp plants for the production of hemp seed oil. Exceptions were samples No. 4 and No. 7 (same manufacturer) with phenotype ratios of 3.8 and 2.4, respectively, indicating a possible origin of the hemp seeds from medical hemp varieties. This is in good agreement with the literature. In a study described by Petrović et al. analysing CBD, THC and CBN in 11 Croatian hemp seed oils, phenotype ratios varied from 0.03 to 0.2 for industrial hemp seed oils and from 1.0 to 15.0 for samples classified as medical hemp seed oils (Petrović et al. Citation2015). In our sample No. 13, the phenotype ratio was 0.3. However, its profile closely resembled that of sample No. 4 and sample No. 7. Notably, samples No. 7 and No. 13 were purchased from the same shop and they share matching product labels and ‘best before dates’. Still, the oils have different cannabinoids profile. Due to conflicting indicators, an unambiguous classification cannot be made for sample No. 13.
Although the cannabinoid contents in the hemp seed oils studied herein varied, they generally had similar cannabinoid profiles. An overview of the profiles for all samples is shown in .
In general, CBDA and CBD were the two cannabinoids with the largest proportions of the cannabinoid fraction in the samples studied. CBDA and CBD accounted for an average of 78% of the cannabinoid fraction in hemp seed oils, with CBDA accounting for 50.6% ( = 53.9%) and CBD for 27.7% (
= 28.2%) of the cannabinoid fraction. When samples Nos. 4, 7, and 13 were excluded, the proportion of CBDA and CBD increased to 83%. THCA and Δ9-THC in sum accounted for the second largest proportions with an average of 7.7% (
= 6.0%) of the cannabinoid fraction. These relative proportions averaged 4.1% for THCA (
= 2.9%) and 3.6% for Δ9-THC (
= 2.8%). All other cannabinoids accounted for smaller proportions of the cannabinoid fraction. Calculated as a pair, the mean proportions were 5.0% (
= 4.8%) for CBCA and CBC, 3.1% (
= 3.2%) for CBDVA and CBDV, 2.2% (
= 0.3%) for THCVA and THCV, 1.3% (
= 1.3%) for CBLA and CBL, 1.2% (
= 1.0%) for CBGA and CBG, and 1.2% (
= 0.8%) for CBNA and CBN.
The relative abundance of CBDA and CBD was more than ten times higher than that of the second most abundant pair, which is to be expected from industrial hemp (Andre et al. Citation2016). Samples Nos. 4, 7 and 13, which were assumed to originate from medical hemp due to their phenotype ratios, showed different cannabinoid profiles (). The high proportions of THCA and Δ9-THC at the expense of CBDA and CBD are the desired characteristics in medical hemp (Andre et al. Citation2016). In the mentioned samples of this study, THCVA and THCV were present in noticeable amounts. As the C3-side chain equivalents of THCA and THC, this was expected, as THCA synthase not only converts CBGA to THCA, but also CBGVA to THCVA (Walsh et al. Citation2021).
Thermal processing of hemp seed oils
Influence of thermal treatment on cannabinoid content and profile
For the thermal processing experiments, an oil sample with a typical cannabinoid profile and relatively high cannabinoid content was selected so that changes in the cannabinoid content could be quantified using the target method shown here and potential effects with respect to the cannabinoids investigated in this study could be described. The chosen sample No. 26 was heated to 130 °C, 160 °C, 180 °C and 200 °C for 10, 30 and 60 min at each temperature setting to investigate the potential effects of thermal processing on hemp seed oils and its cannabinoid contents and profiles. The heating was carried out in both non-capped and capped vials. Thus, it would have been possible to a) identify effects of thermal processing under conditions similar to, for example, household processing (e.g. frying hemp seed oils in a pan) and compare these results to b) an approach with a limited access to oxygen.
An overview of the cannabinoid contents in the a) non-capped and b) capped approaches is shown in and the details are listed in Tables S4 and S5. In the non-processed hemp seed oil sample, the cannabinoid content was 87.3 mg kg−1. The cannabinoid content decreased significantly with both increasing temperature and processing time (p < 0.05, Table S2).
Figure 3. Sum of and individual cannabinoid contents in mg kg−1 in thermally processed hemp seed oils heated in vials that were a) non-capped and b) capped. The black dots represent the ratio of acidic and neutral cannabinoids (A/N) for the given samples (see secondary y-axis). For each sample, the error bars represent the sum of the standard deviations for each individual cannabinoid from a duplicate workup, each measured in two dilution levels (n = 4). The individual values are listed in Table S4 for a) and in Table S5 for b). ‘n. p.’ is short for ‘not processed’.

As the trends were similar for both approaches, only one approach (non-capped) will be discussed and then the differences between the approaches. A decrease in cannabinoid content was evident when, for example, comparing the untreated oil with the most heated oil (200 °C, 60 min). There, a decrease in cannabinoid content from 87.3 to 54.1 mg kg−1 was observed (−38%). Moreover, this behaviour was evident when comparing different heating durations at a constant temperature. For example, at 180 °C the cannabinoid content decreased to 77.5 mg kg−1 (−11%) after 10 min, to 68.2 mg kg−1 (−22%) after 30 min, and to 57.6 mg kg−1 (−34%) after 60 min. The same trend was observed at the other temperatures (Table S4). For the sum of CBDA and CBD, a decrease from 70.4 to 44.0 mg kg−1 (−38%) was observed in the comparison of untreated oil and the strongest heating (200 °C, 60 min). With one exception, the other pairs showed decreases of the same magnitude: CBCA and CBC by −64%, CBDVA and CBDV by −45%, CBGA and CBG by −31%, CBLA and CBL by −47%, THCA and Δ9-THC by –32%, and THCVA and THCV by −42%. Only the total of CBNA and CBN showed an increase, of +16%, which is most likely caused by oxidative degradation of Δ9-THC to CBN.
THCA and Δ9-THC were also affected by thermal processing of the hemp seed oils and behave similarly to the other cannabinoids. After the strongest heating, the content of THCA decreased from 2.5 mg kg−1 to < LOQ (0.025 mg kg−1) (–100%). Simultaneously, Δ9-THC increased from 3.7 to 4.5 mg kg−1 (+22%). The Δ9-THC equivalents decreased from 5.5 to 4.5 mg kg−1 (–28%) and the proportion of Δ9-THC + THCA in the cannabinoid fraction increased slightly from 7.6% to 8.3%. Although the Δ9-THC equivalent level (which is set by the EU) decreased, an increase in Δ9-THC content is possible when hemp seed oils are heated strongly, which in turn should be taken into account when considering health risks.
In the study by Seo et al. (Citation2022), psychoactive THC isomers, other than Δ9-THC, were detected after a heat treatment of cannabis extracts. In this study, the occurrence of Δ8-THC was used as an example to study whether similar mechanisms could occur during the heating of hemp seed oils. As a pre-requisite, Δ8-THC could not be detected in native or thermally processed oil samples.
In contrast to the approach a) with non-capped vials where an exchange of fresh air and oxygen was possible in the oven with active air circulation, this was not possible in the approach b) with capped vials. Resulting effects are discussed below for approach b). Previous studies in different hemp matrices have shown that there were direct and indirect influences of oxygen on the degradation of cannabinoids when open and closed containers were thermally processed and compared (Citti et al. Citation2018; Moreno et al. Citation2020; Gigopulu et al. Citation2022; Seo et al. Citation2022). In this study and in accordance with the literature, a reduction of cannabinoid content was more pronounced in the non-capped approach with an increase in thermal stress. For example, comparing both approaches after the treatment at 200 °C for 60 min, the cannabinoid contents decreased from 87.3 to 54.1 mg kg−1 (–38%) and 66.8 mg kg−1 (–23%), respectively. Furthermore, in the non-capped vials, neutral cannabinoid levels increased by only 34%, whereas in the capped vials the increase was 62% when comparing non-processed and 200 °C/60 min oils. Most of the increase was most likely caused by decarboxylation of acidic forms into their corresponding neutral forms. Also, the relatively low number of neutral cannabinoids in non-capped vials compared to capped vials might be due to evaporation of neutral cannabinoids under open conditions. In this context, capped vials would retain the substances. The boiling points of CBDA, CBD, THCA and Δ9-THC are reported to be 130 °C, 160–180 °C, 120 °C and 157 °C, respectively (McPartland and Russo Citation2001; Birenboim et al. Citation2022). Boiling points for the other cannabinoids investigated in the scope of the present study are not available in the literature. Potential loss of neutral cannabinoids due to evaporation after heat treatment in C. sativa inflorescence extracts and plant material has been suggested previously (Moreno et al. Citation2020; Seo et al. Citation2022). Evaporation of Δ9-THC and CBD has also been shown to occur upon heating marihuana extracts (Veress et al. Citation1990). Assuming that the other cannabinoids also boil at similar temperatures (< 200 °C), it is possible that there is an evaporation of cannabinoids from hemp seed oils during thermal processing. This could partly explain the greater loss of cannabinoid contents in non-capped vials compared to capped vials.
Decarboxylation and degradation of cannabinoids during thermal processing of hemp seed oils
The purpose of this section is to discuss possible decarboxylation and degradation of cannabinoids during thermal processing of hemp seed oils and the resulting effects on cannabinoid contents and profile.
In the following, the non-capped approach is considered. With an increasing temperature the sum of cannabinoid acids significantly decreased (p < 0.05, Table S2), while the sum of neutral cannabinoids significantly increased (p < 0.05, Table S2). Comparing the non-processed oil with the processing at 200 °C/60 min, a decrease of the cannabinoid acids content from 47.3 to 0.4 mg kg−1 (–99%) was observed. Simultaneously, the sum of neutral cannabinoids increased from 40.0 to 53.7 mg kg−1 (+34%). The acid/neutral ratio (A/N) decreased from 1.18 to 0.01. The decrease of the A/N implies that acidic cannabinoids decarboxylate to their corresponding neutral counterparts (). Comparing all temperatures applied (130 °C, 160 °C, 180 °C and 200 °C) at 30 min processing time, the decrease in acids was –6%, –25%, –73% and –95%. For the neutral forms the increases were +3%, +18%, +39% and +43%. As a specific example, the contents of CBDA decreased from 38.7 to 0.4 mg kg−1 (−99%) and of CBD increased from 31.8 to 43.6 mg kg−1 (+37%). The CBDA/CBD ratio decreased from 1.22 to 0.01. For THCA contents, decreases from 2.9 mg kg−1 to < LOQ (0.025 mg kg−1) (–100%) and increased for Δ9-THC from 3.7 to 4.5 mg kg−1 (+22%). Both pairs showed changes representative for most other cannabinoid acid/neutral pairs, CBNA and CBN being the notable exception as mentioned above. Details on the percent changes for each compound are listed in Table S7.
When studying the decarboxylation of seven different cannabinoid acids (CBCA, CBDA, CBDVA, CBGA, CBNA, THCA and THCVA) in dried cannabis flowers at temperatures up to 40 °C, it was found that the temperature-dependent decarboxylation rate constants of the cannabinoid acids studied were ‘mostly indistinguishable from one another’ (Meija et al. Citation2022). This study shows that decarboxylation of cannabinoid acids occurs at temperatures ≥130 °C in the food matrix hemp seed oil. The more intense the thermal processing, the more similar all acids behave. While the decrease of individual acids ranges from 8% to 37% (R = 29%) after processing at 130 °C for 60 min, the range narrows to 49–77% (R = 28%) at 160 °C/60 min, to 90–100% (R = 10%) at 180 °C/60 min, and to 99–100% (R = 1%) at 200 °C/60 min. This behaviour is expected given the similar decarboxylation rate constants mentioned. Gigopulu et al. (Citation2022) found that after thermal processing, the THCA/THC ratio of 26.7 in raw cannabis flower decreased to 0.04 at 133 °C (about 54 min total), and to 0 at 160 °C (about 70 min total). These observations are in good agreement with the trends of this study concerning the THCA/Δ9-THC ratio in the non-capped approach. Here, a decrease from 0.80 to 0.52 and 0.15 was observed after treatment at 130 °C and 160 °C for 60 min each. It should be noted that the THCA/Δ9-THC ratios in the non-processed products are vastly different as the flowers originate from medicinal hemp while the hemp seed oils originate from industrial hemp. In terms of matrix effects on decarboxylation, Gigopulu et al. (Citation2022) found that when a powdered THCA standard was compared with THCA in plant material, decarboxylation in the plant material was comparatively quick and completed at a lower temperature. In addition, Seo et al. (Citation2022) highlighted the influence of matrix on decarboxylation by showing that decarboxylation of CBDA in inflorescence extracts was faster compared to isolated CBDA. Pacifici et al. (Citation2017) achieved complete decarboxylation of cannabinoid acids in an olive oil extract of flowering buds, which was slower than the decarboxylation conducted by Seo et al. (Citation2022). In summary, matrix effects appear to play a relevant role in cannabinoid acid decarboxylation processes.
The mass fraction of bound CO2 in the carboxyl groups of the cannabinoid acid in the cannabinoid fraction, calculated by molar masses, is 6.7%. Assuming that all acids would decarboxylate completely after intense thermal processing, the complete loss of CO2 can explain only part of the 38% decrease in cannabinoid content after treatment at 200 °C for 60 min (Table S6). Conversely, in addition to decarboxylation, transformation of cannabinoids after thermal processing may play a relevant role in hemp seed oils.
A greater decrease in cannabinoid content in non-capped vials compared to capped vials implies that the presence of oxygen supports the degradation of cannabinoids. The degradation of cannabinoids by heat exposure in cannabis extracts and plant material has already been described in the literature. For example, after heating an inflorescence extract at 130 °C for 30 min, seven degradation products of CBDA were identified, mainly resulting from decarboxylation, hydration (addition of H2O to a double bond), and cyclisation of CBD (Seo et al. Citation2022). While the thermal degradation of major cannabinoids to common minor cannabinoids is known, the degradation to less common cannabinoids or other substance classes is not yet well understood. In the future, investigations using high-resolution mass spectrometry (HRMS), e.g. in the form of suspect screening, should be carried out to investigate possible as yet unknown chemical pathways resulting from the thermal treatment of hemp-containing food. Known reactions include the formation of Δ9-THC from CBD and CBN from Δ9-THC, or the thermal degradation of CBDA to CBEA (cannabielsoinic acid) and CBE in inflorescence extracts (García-Valverde et al. Citation2022; Seo et al. Citation2022).
The oxidative degradation of Δ9-THC to CBN is a known reaction that is enhanced by heat (Russo et al. Citation2008; Petrović et al. Citation2015). In both processing approaches, CBNA could not be quantified (LOQ 0.013 mg kg−1) after treatment at 180 °C/60 min and 200 °C/30 min but the contents of CBN kept rising after the complete degradation of CBNA. Therefore, it can be assumed that CBN was formed from Δ9-THC in sufficient amounts that might counteract the simultaneous thermal degradation of the substance. More CBN was formed in the capped vials without free airflow, where CBN content increased from 0.7 to 1.3 mg kg−1 (+103%) compared to 1.2 mg kg−1 (+85%) in the a) non-capped vials. Therefore, it can be assumed that, on the one hand, no constant supply of oxygen is required for the degradation of Δ9-THC and that, on the other hand, cannabinoids are better retained in the capped vials, possibly due to less evaporation.
Conclusion
In this study, a HPLC-MS/MS method was used to quantify 16 cannabinoids in both native and processed hemp seed oils. We have provided a comprehensive overview of cannabinoid profiles in this food matrix. The analysis revealed that CBDA and CBD quantitatively were the most relevant cannabinoids, comprising an average of 78% of the cannabinoid fraction in the hemp seed oils, followed by THCA and Δ9-THC with an average of 8%. Thus, on average, these commonly researched cannabinoids are the main cannabinoids in hemp seed oil in regards to their relative contents. Our hypothesis that thermal processing of commercial hemp seed oils at temperatures between 130 °C and 200 °C for 10–60 min would lead to transformation reactions and changes in the cannabinoid profile has been supported. With increasing processing temperatures and time, cannabinoids acids are decreasing while neutral forms are increasing. In regards to Δ9-THC, processing at 200 °C for 60 min led to an increase in Δ9-THC by 22%, while THCA and Δ9-THC equivalents decreased by 100% and 28%, respectively. This must be kept in mind for risk assessments when oils are processed during production or by consumers. The findings underline the need for an analytical differentiation of THCA and Δ9-THC and other potentially relevant pairs in food. Differentiated profiles are essential for the evaluation of possible pharmacological effects from cannabinoids in hemp-containing foods, both native and processed, and may support risk assessment.
CRediT authorship contribution statement
Niklas Lindekamp: Conceptualisation, Methodology, Validation, Formal analysis, Investigation, Writing – Original Draft, Visualisation. Nadja Triesch: Conceptualisation, Methodology, Validation, Writing – Review & Editing, Supervision, Project administration. Sascha Rohn: Writing – Review & Editing, Supervision. Stefan Weigel: Conceptualisation, Writing – Review & Editing, Supervision, Project administration, Funding acquisition.
Supplemental Material
Download PDF (140.5 KB)Acknowledgements
The authors thank Sebastian Kemter and Michael Weiß for their support.
Disclosure statement
The authors declare that they have no known competing financial interests or personal relationships that could have appeared to influence the work reported in this paper.
Additional information
Funding
References
- Aladić K, Jokić S, Moslavac T, Tomas S, Vidović S, Vladić J, Šubarić D. 2015. Cold pressing and supercritical CO2 extraction of hemp (Cannabis sativa) seed oil. ChemBiochemEngQ. 28(4):481–490. doi: 10.15255/CABEQ.2013.1895.
- Amtliche Sammlung von Untersuchungsverfahren nach §64 LFGB. 2024. Band I (L), L 13.04-9: Bestimmung von Cannabinoiden in pflanzlichen Ölen und Spirituosen mittels LC-MS/MS.
- Andre CM, Hausman JF, Guerriero G. 2016. Cannabis sativa: the plant of the thousand and one molecules [review]. Front Plant Sci. 7:19. doi: 10.3389/fpls.2016.00019.
- Bhattacharyya S, Morrison PD, Fusar-Poli P, Martin-Santos R, Borgwardt S, Winton-Brown T, Nosarti C, O' Carroll CM, Seal M, Allen P, et al. 2010. Opposite effects of delta-9-tetrahydrocannabinol and cannabidiol on human brain function and psychopathology. Neuropsychopharmacology. 35(3):764–774. doi: 10.1038/npp.2009.184.
- Birenboim M, Fallik E, Kengisbuch D, Shimshoni JA. 2022. Quantitative and qualitative spectroscopic parameters determination of major cannabinoids. J Lumin. 252:119387. doi: 10.1016/j.jlumin.2022.119387.
- Capriotti AL, Cannazza G, Catani M, Cavaliere C, Cavazzini A, Cerrato A, Citti C, Felletti S, Montone CM, Piovesana S, et al. 2021. Recent applications of mass spectrometry for the characterization of cannabis and hemp phytocannabinoids: from targeted to untargeted analysis. J Chromatogr A. 1655:462492. doi: 10.1016/j.chroma.2021.462492.
- Cardenia V, Toschi TG, Scappini S, Rubino RC, Rodriguez-Estrada MT. 2018. Development and validation of a Fast gas chromatography/mass spectrometry method for the determination of cannabinoids in Cannabis sativa L. J Food Drug Anal. 26(4):1283–1292. doi: 10.1016/j.jfda.2018.06.001.
- Chang CW, Tung CW, Tsai CC, Wu YT, Hsu MC. 2017. Determination of cannabinoids in hemp nut products in Taiwan by HPLC-MS/MS coupled with chemometric analysis: quality evaluation and a pilot human study. Drug Test Anal. 9(6):888–897. doi: 10.1002/dta.2062.
- Christinat N, Savoy MC, Mottier P. 2020. Development, validation and application of a LC-MS/MS method for quantification of 15 cannabinoids in food. Food Chem. 318:126469. doi: 10.1016/j.foodchem.2020.126469.
- Citti C, Linciano P, Panseri S, Vezzalini F, Forni F, Vandelli MA, Cannazza G. 2019. Cannabinoid profiling of hemp seed oil by liquid chromatography coupled to high-resolution mass spectrometry [original research]. Front Plant Sci. 10:120. doi: 10.3389/fpls.2019.00120.
- Citti C, Pacchetti B, Vandelli MA, Forni F, Cannazza G. 2018. Analysis of cannabinoids in commercial hemp seed oil and decarboxylation kinetics studies of cannabidiolic acid (CBDA). J Pharm Biomed Anal. 149:532–540. doi: 10.1016/j.jpba.2017.11.044.
- Da Porto C, Decorti D, Tubaro F. 2012. Fatty acid composition and oxidation stability of hemp (Cannabis sativa L.) seed oil extracted by supercritical carbon dioxide. Ind Crops Prod. 36(1):401–404. doi: 10.1016/j.indcrop.2011.09.015.
- Di Marco Pisciottano I, Guadagnuolo G, Soprano V, De Crescenzo M, Gallo P. 2018. A rapid method to determine nine natural cannabinoids in beverages and food derived from Cannabis sativa by liquid chromatography coupled to tandem mass spectrometry on a QTRAP 4000. Rapid Commun Mass Spectrom. 32(19):1728–1736. doi: 10.1002/rcm.8242.
- Dräger H, Barthlott I, Golombek P, Walch SG, Lachenmeier DW. 2022. Time trends of tetrahydrocannabinol (THC) in a 2008–2021 German National survey of hemp food products. Foods. 11(3):486. doi: 10.3390/foods11030486.
- EURL/NRL Mycotoxin/Plant Toxin Network. 2023. Guidance document on identification of mycotoxins and plant toxins in food and feed.
- European Commission. 2022. COMMISSION REGULATION (EU) 2022/1393 of 11 August 2022 amending Regulation (EC) No 1881/2006 as regards maximum levels of delta-9-tetrahydrocannabinol (Δ9-THC) in hemp seeds and products derived therefrom. 2022/1393. Brussels, Belgium: European Commission.
- European Commission. 2023a. COMMISSION IMPLEMENTING REGULATION (EU) 2023/2783 of 14 December 2023 laying down the methods of sampling and analysis for the control of the levels of plant toxins in food and repealing Regulation (EU) 2015/705. In: european Commission, editor. (EU) 2023/2783. Official Journal of the European Union. Brussels, Belgium: European Commission.
- European Commission. 2023b. COMMISSION REGULATION (EU) 2023/915 of 25 April 2023 on maximum levels for certain contaminants in food and repealing Regulation (EC) No 1881/2006. In: european Commission, editor. 2023/915. Official Journal of the European Union. Brussels, Belgium: European Commission.
- Fodor B, Molnár-Perl I. 2017. The role of derivatization techniques in the analysis of plant cannabinoids by gas chromatography mass spectrometry. Trend Anal Chem. 95:149–158. doi: 10.1016/j.trac.2017.07.022.
- FragDenStaat. 2021. THC in Lebensmitteln. Anfrage an: bundesamt für verbraucherschutz und lebensmittelsicherheit. in: fragdenstaat.de; [updated 25.08.2021; accessed]. https://fragdenstaat.de/anfrage/thc-in-lebensmitteln/.
- García-Valverde MT, Sánchez-Carnerero Callado C, Díaz-Liñán MC, Sánchez de Medina V, Hidalgo-García J, Nadal X, Hanuš L, Ferreiro-Vera C. 2022. Effect of temperature in the degradation of cannabinoids: from a brief residence in the gas chromatography inlet port to a longer period in thermal treatments. Front Chem. 10:1038729. doi: 10.3389/fchem.2022.1038729.
- Gigopulu O, Geskovski N, Stefkov G, Gjorgievska VS, Spirevska IS, Huck CW, Makreski P. 2022. A unique approach for in-situ monitoring of the THCA decarboxylation reaction in solid state. Spectrochim Acta A Mol Biomol Spectrosc. 267(Pt 2):120471. doi: 10.1016/j.saa.2021.120471.
- Gülck T, Møller BL. 2020. Phytocannabinoids: origins and biosynthesis. Trends Plant Sci. 25(10):985–1004. doi: 10.1016/j.tplants.2020.05.005.
- Jang E, Kim H, Jang S, Lee J, Baeck S, In S, Kim E, Kim YU, Han E. 2020. Concentrations of THC, CBD, and CBN in commercial hemp seeds and hempseed oil sold in Korea. Forensic Sci Int. 306:110064. doi: 10.1016/j.forsciint.2019.110064.
- Kladar N, Čonić BS, Božin B, Torović L. 2021. European hemp-based food products – health concerning cannabinoids exposure assessment. Food Control. 129:108233. doi: 10.1016/j.foodcont.2021.108233.
- Lachenmeier DW, Bock V, Deych A, Sproll C, Rajcic de Rezende T, Walch SG. 2019. Hanfhaltige Lebensmittel - Ein Update. Deutsche Lebensmittel-Rundschau. 115(8):351–372. doi: 10.5281/zenodo.3384640.
- Lachenmeier DW, Habel S, Fischer B, Herbi F, Zerbe Y, Bock V, Rajcic de Rezende T, Walch SG, Sproll C. 2019. Are adverse effects of cannabidiol (CBD) products caused by tetrahydrocannabinol (THC) contamination? [version 6; peer review: 2 approved. F1000Res. 8:1394. doi: 10.12688/f1000research.19931.6.
- Lachenmeier DW, Kroener L, Musshoff F, Madea B. 2004. Determination of cannabinoids in hemp food products by use of headspace solid-phase microextraction and gas chromatography-mass spectrometry. Anal Bioanal Chem. 378(1):183–189. doi: 10.1007/s00216-003-2268-4.
- Lange BM, Zager JJ. 2021. Comprehensive inventory of cannabinoids in Cannabis sativa L.: can we connect genotype and chemotype? Phytochem Rev. 21(4):1273–1313. doi: 10.1007/s11101-021-09780-2.
- McPartland JM, Russo EB. 2001. Cannabis and cannabis extracts: greater than the sum of their parts? J Cannabis Ther. 1(3–4):103–132. doi: 10.1300/J175v01n03_08.
- Meija J, McRae G, Miles CO, Melanson JE. 2022. Thermal stability of cannabinoids in dried cannabis: a kinetic study. Anal Bioanal Chem. 414(1):377–384. doi: 10.1007/s00216-020-03098-2.
- Moreno T, Dyer P, Tallon S. 2020. Cannabinoid decarboxylation: a comparative kinetic study. Ind Eng Chem Res. 59(46):20307–20315. doi: 10.1021/acs.iecr.0c03791.
- Pacifici R, Marchei E, Salvatore F, Guandalini L, Busardo FP, Pichini S. 2017. Evaluation of cannabinoids concentration and stability in standardized preparations of cannabis tea and cannabis oil by ultra-high performance liquid chromatography tandem mass spectrometry. Clin Chem Lab Med. 55(10):1555–1563.
- Perrotin-Brunel H, Buijs W, Spronsen J, Roosmalen MJEv, Peters CJ, Verpoorte R, Witkamp G-J. 2011. Decarboxylation of Δ9-tetrahydrocannabinol: kinetics and molecular modeling. J Mol Struct. 987(1–3):67–73. doi: 10.1016/j.molstruc.2010.11.061.
- Petrović M, Debeljak Ž, Kezić N, Džidara P. 2015. Relationship between cannabinoids content and composition of fatty acids in hempseed oils. Food Chem. 170:218–225. doi: 10.1016/j.foodchem.2014.08.039.
- R Core Team. 2022. R: a language and environment for statistical computing Vienna, Austria: R Foundation for Statistical Computing.
- Ross SA, Elsohly MA. 1997. CBN and D9-THC concentration ratio as an indicator of the age of stored marijuana samples. Bull Narcotics. 49–50(1):139–147. https://www.unodc.org/unodc/en/data-and-analysis/bulletin/bulletin_1997-01-01_1_page008.html.
- Ross SA, Mehmedic Z, Murphy TP, ElSohly MA. 2000. GC-MS analysis of the total d9-THC content of both drug- and fiber-type cannabis seeds. J Anal Toxicol. 24(8):7C5–717. doi: 10.1093/jat/24.8.715.
- Russo EB, Jiang HE, Li X, Sutton A, Carboni A, del Bianco F, Mandolino G, Potter DJ, Zhao YX, Bera S, et al. 2008. Phytochemical and genetic analyses of ancient cannabis from Central Asia. J Exp Bot. 59(15):4171–4182. doi: 10.1093/jxb/ern260.
- Schwilke EW, Schwope DM, Karschner EL, Lowe RH, Darwin WD, Kelly DL, Goodwin RS, Gorelick DA, Huestis MA. 2009. Delta9-tetrahydrocannabinol (THC), 11-hydroxy-THC, and 11-nor-9-carboxy-THC plasma pharmacokinetics during and after continuous high-dose oral THC. Clin Chem. 55(12):2180–2189. doi: 10.1373/clinchem.2008.122119.
- Seo C, Jeong M, Lee S, Kim EJ, Rho S, Cho M, Lee YS, Hong J. 2022. Thermal decarboxylation of acidic cannabinoids in Cannabis species: identification of transformed cannabinoids by UHPLC-Q/TOF–MS. J Anal Sci Technol. 13(1):42. doi: 10.1186/s40543-022-00351-4.
- Tahir MN, Shahbazi F, Rondeau-Gagné S, Trant JF. 2021. The biosynthesis of the cannabinoids. J Cannabis Res. 3(1):7. doi: 10.1186/s42238-021-00062-4.
- Teh SS, Birch J. 2013. Physicochemical and quality characteristics of cold-pressed hemp, flax and canola seed oils. J Food Compos Anal. 30(1):26–31. doi: 10.1016/j.jfca.2013.01.004.
- United Nations Office on Drugs and Crime. 2022. Recommended methods for the identification and analysis of cannabis and cannabis products. Vienna, Austria: United Nations Office on Drugs and Crime.
- Veress T, Szanto JI, Leisztner L. 1990. Determination of cannabinoid acids by high-performance liquid chromatography of their neutral derivatives formed by thermal decarboxylation: i. study of the decarboxylation process in open reactors. J Chromatogr A. 520:339–347. doi: 10.1016/0021-9673(90)85118-F.
- Walsh KB, McKinney AE, Holmes AE. 2021. Minor cannabinoids: biosynthesis, molecular pharmacology and potential therapeutic uses. Front Pharmacol. 12:777804. doi: 10.3389/fphar.2021.777804.
- Wang M, Wang Y-H, Avula B, Radwan MM, Wanas AS, van Antwerp J, Parcher JF, ElSohly MA, Khan IA. 2016. Decarboxylation study of acidic cannabinoids: a novel approach using ultra-high-performance supercritical fluid chromatography/photodiode array-mass spectrometry. Cannabis Cannabinoid Res. 1(1):262–271. doi: 10.1089/can.2016.0020.