ABSTRACT
The genus Agrocybe, situated within the Strophariaceae family, class Agaricomycetes, and phylum Basidiomycota, encompasses a myriad of species exhibiting significant biological activities. This review presents an integrative overview of the secondary metabolites derived from Agrocybe species, elucidating their respective biological activities and potential pharmacological applications. The metabolites under scrutiny encompass a diverse array of biological macromolecules, specifically polysaccharides and lectins, as well as a diverse group of 80 documented small molecular chemical constituents, classified into sterols, sesquiterpenes, volatile compounds, polyenes, and other compounds, their manifesting anti-inflammatory, anticancer, antioxidant, hepatoprotective, antimicrobial, and antidiabetic activities, these metabolites, in which polysaccharides exhibit abundant activities, underscore the potential of the Agrocybe genus as a valuable source of biologically active natural products. The present review emphasises the need for escalated research into Agrocybe, including investigations into the biosynthetic pathways of these metabolites, which could foster the development of novel pharmaceutical therapies to address various health challenges.
1. Introduction
The initial classification of the genus Agrocybe was credited to the Swiss mycologist Victor Fayod in 1889 (Fayod Citation1889). Fayod’s description of Agrocybe was notably succinct. In 1971, Egon Horak, an Austrian fungal taxonomist, provided a comprehensive summary based on a substantial amount of data on Agaricales fungi from New Zealand. According to Horak, the pileus of Agrocybe species is fleshy and can be ranged in colour from white to brown, while the spores are brown and possess thick walls (Horak Citation1971).
In 1987, Scottish mycologists Watling and Taylor provided a detailed description of the Agrocybe genus. According to Watling, the fruiting bodies of Agrocybe species are medium to large, pale white to cream in colour, and fleshy in texture. The pileus, or cap, is semiglobate in shape, fleshy, and either smooth or slightly wrinkled. While the pileus is typically not plicate-striate (with distinct radial lines), exceptions with pronounced striations do exist (Watling and Taylor Citation1987). The centre of the stipe can be white, buff, or ochraceous in colour, generally darkening upwards from the base, with a distinct ring, or in some cases, no veil. The flesh is white or pale-coloured, often darkening upwards in the stipe with age, and exhibits a mealy (farinaceous) taste and smell. Basidiospores are typically smooth, thick-walled, and either possess or lack a prominent germ pore. Most of them are saprophytic, thriving in diverse environments such as forests, pastures, wilderness, and on dung, plant remains, refuse, and wood ().
Figure 1. Biomorphology of some Agrocybe species. (a) A. cylindracea in the wild. (b) Cultivated A. cyclindraceae. (c) A. praecox in the wild.
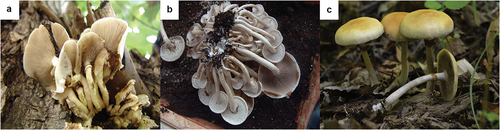
The genus Agrocybe belongs to Basidiomycota, Agaricomycetes, Agaricales, and Strophariaceae, lacked a unified standard for morphological classification in the last century. This situation prevailed until 1975 when German mycologist Rolf Singer established a standard for the genus and categorised it under the family Bolbitiaceae (Singer Citation1975). Based on whether the spores have a germ-pore or not, Agrocybe can be bifurcated into two subgenera: Subgen Agrocybe and Aporus. Subgen Agrocybe is further subdivided into five sections: Agrocybe, Pediades, Microsporae, Allocystides, and Evelatae. Conversely, Subgen Aporus consists of two sections: Aporus and Velatae. Singer’s classification undoubtedly represents a milestone contribution to the study of Agrocybe. In later work, Watling conducted a thorough taxonomic study of Agrocybe and proposed revisions to Singer’s classification system. In Watling (Citation1982) revised system, the classification still retained two subgenera. However, Sect Evelatae was moved from Subgen Agrocybe to Subgen Aporus, and Sect Allocystides was removed from Subgen Agrocybe. This classification system has proven influential and continues to be utilised by scholars in the field to this day. However, modern research on the anamorphs of Bolbitiaceae indicated that Agrocybe did not belong to the family Bolbitiaceae (Walther and Weiss Citation2006). According to recent molecular phylogenetic analyses, Agrocybe belongs to the family Strophariaceae (Matheny et al. Citation2006), comprising three species branches: A. Praecox (Pers.) Fayod, A. Smithii and A. Pediades. The contemporary taxonomy of basidiomycete definitively classifies Agrocybe within the Strophariaceae (He et al. Citation2019). The genus continues to be enriched with new species, thanking to the ongoing contributions and findings of mycologists worldwide, leading to an ever-increasing discovery of new species.
Several species within the Agrocybe genus are known for being significant sources of natural products with biological and pharmacological activities. Over the previous three decades, polysaccharides, lectins, and more than 80 small-molecule compounds have all been isolated and identified by researchers as belonging to this genus. Some of them, like ceramide, have inhibitory effects on COX-1 and 2 (Diyabalanage et al. Citation2008), alkaloids can scavenge free radicals (Kim et al. Citation1997), Sterols to prevent osteoporosis (Choi et al. Citation2009), and polysaccharides have antiobesity properties (Zhu et al. Citation2022a), lectins with immunomodulatory activity (Ye et al. Citation2021), etc. These active substances have attracted widespread attention in today’s society. Despite the intricate taxonomy of Agrocybe species and the diverse structures and activities of their produced secondary metabolites, there is currently no comprehensive systematic review available. In the present article, we conducted an extensive and systematic review of the chemical structures of secondary metabolites and their biosynthesis in Agrocybe, along with their associated biological activities and pharmacological effects. The objective of this review is to enhance our understanding of the active compounds found within these species and to facilitate the transition of these bioactive compounds into clinical applications. This review will serve as a reference for the better utilisation of these medicinal and edible fungi.
2. Polysaccharides
Polysaccharides play crucial roles as primary active constituents in mushrooms. Unlike plant and animal polysaccharides, mushroom polysaccharides are primarily constituted of β-glucan, characterised by a β-1,3/1,6 glycosidic linkage (Rosdan Bushra and Nurul Citation2022). This specific bond is resistant to the α-1,4-amylase enzyme in humans, rendering β-glucan indigestible. Consequently, mushroom polysaccharides can potentially stimulate the immune system and be absorbed in the gastrointestinal tract. Additionally, mushroom polysaccharides typically exhibit lower molecular weights compared to polysaccharides from other sources, facilitating their absorption and utilisation by the body (Hyder and Dutta Citation2021). Mushroom polysaccharides also exhibit antioxidant properties, containing compounds capable of scavenging free radicals and mitigating oxidative stress, which is beneficial in preventing or decelerating the progression of chronic diseases such as cardiovascular diseases and cancers. Noteworthy examples include Lentinan from Lentinula edodes (Wu et al. Citation2023; Xiao et al. Citation2023), polysaccharides from Ganoderma lucidum (Reishi) (Jia et al. Citation2022), and Hericium erinaceus (Lion’s Mane) (Yang et al. Citation2022). These substances are known for their medicinal properties, including anti-tumour and immune regulatory effects. Similarly, polysaccharides derived from the genus Agrocybe exhibited significant medicinal values. It has been reported that they engaged in physiological processes like immunological regulation, hypoglycaemic properties, inhibition of lipid peroxidation, anti-tumour effects, anti-ageing effects, and in vitro antioxidant activity (Jing et al. Citation2018b) (). Kiho et al. (Citation1989) isolated an insoluble linear (1→3)-α-D-glucan (AG-AL) from the fruiting body of A. cylindracea. Carboxymethylated derivatives of AG-AL, known as O-(carboxymethyl) AG-AL, exhibited remarkable anti-tumour activity. In a study by Xu et al. (Citation2005), it was reported that polysaccharides derived from A. aegerita (AAP) showed that transplanted sarcoma S180 and S180 ascites tumours in mice were significantly inhibited. Additionally, AAP was found to prolong the survival period and counteract leucopenia induced by the chemotherapy drug Cyclophosphamide. Ji et al. (Citation2013) examined the effect of AAP on the expression of tumour necrosis factor (TNF-α) and interferon (IFN-γ) in rat esophageal carcinoma (EC) to further understand the anti-tumour mechanism of this compound. Their research revealed that AAP downregulated TNF-α and upregulated IFN-γ, thereby promoting immune function restoration and enhancing anti-tumour capabilities. Liu et al. (Citation2023) obtained two different polysaccharides, ACP and ACAP, from A. cylindracea. ACP was found to inhibit the proliferation and induce cell death in human gastric cancer cells (MGC-803), with an IC50 of 240.71 µg/mL after 24 hours. The heteroglycan SACP-80, derived from A. cylindracea, was primarily composed of a backbone consisting of β-(1→6)-glucopyranosyl, α-(1→6)-galactopyranosyl, and α-(1→2,6)-glucopyranosyl units, with a molecular ratio of 3:1:1 (Wang et al. Citation2021), exhibited significant inhibition of colorectal cancer cell growth with an IC50 value of 490 µg/mL (Wang et al. Citation2023). These studies suggested that polysaccharides from Agrocybe were able to inhibit tumour growth and metastasis, prolong survival, and enhance immune function, providing a basis for utilisation as potential antitumour therapeutic agents.
Table 1. Molecular weight, monosaccharide composition, molar ratio, and activity of Agrocybe polysaccharides.
Two polysaccharides were isolated from the fruiting bodies of A. cylindracea using hot water extraction by Kiho et al. (Citation1994). The high molecular weight glucan, known as AG-HN1, primarily consisted of β-D-glucan. The molar ratio of the galactose, glucose, fucose, and mannose in the low molecular weight heteroglycans, AG-HN2, was 36:27:17:14. In both healthy and mice with diabetes induced by streptozotocin (STZ), AG-HN1 demonstrated significant hypoglycaemic activity when given at a dose of 50 mg/kg after 6 hours. In contrast to AG-HN2, it displayed more activity. In a separate study, Lee et al. (Citation2010) isolated β-D-Glucan (polysaccharide) from A. chaxingu. In addition to drastically lowering blood sugar levels in STZ-induced diabetic mice, this polysaccharide also improved the pancreatic β-cells’ tolerance to STZ injury. These results suggested that they had potential as a diabetic therapy medication.
When the rate of free radical generation exceeds the capacity of the antioxidant defence system, it leads to an imbalance and accumulation of oxidative stress, resulting in cellular damage. One of the main contributing factors of ageing is this imbalance (Başaran et al. Citation2017). In a study by Jing et al. (Citation2018a), two polysaccharides, named Ac-MPS and Al-MPS, were extracted from the mycelium of A. aegerita (Brig.) Sing. Gas chromatography (GC) analysis showed that Ac-MPS consisted of fucose, arabinose, mannose, galactose, and glucose in a molar ratio of 1.5:1:4.7:5.7:117.2, while Al-MPS consisted of fucose, mannose, galactose, and glucose in a molar ratio of 1:4:2.3:23.2. Ac-MPS had a hydroxyl scavenging capacity of 68% and a DPPH scavenging rate of 85% at a dose of 4.5 mg/mL, according to an in vitro antioxidant investigation. AAPS is a novel polysaccharide of A. aegirita, which mainly consists of rhamnose, fucose, arabinose, xylose, mannose, glucose, and galactose with a molar ratio of 2.90:10.25:0.78:0.77:3.70:38.27:0.26. It was found that at a concentration of 2 mg/mL enhanced telomerase activity, facilitated cell entry into the G2 phase of the cell cycle, and decreased mitochondrial membrane potential. Therefore, AAPS might have certain anti-ageing properties (Liu et al. Citation2020).
Zhao et al. (Citation2017) utilised the 95% ethanol precipitation method to extract extracellular polysaccharide (EPS) and its components EPS-1, EPS-2, and EPS-3 from the fermentation broth of A. cylindracea. EPS was found to consist of rhamnose and glucan in a molar ratio of 2.8:1, which had antioxidant activity in vitro and anti-ageing action in vivo in mice given D-galactose to promote ageing. By lowering the levels of malondialdehyde (MDA) and total cholesterol (TC) and raising the activities of superoxide dismutase (SOD), glutathione peroxidase (GPx), and total antioxidant capacity (T-AOC), the EPS demonstrated potential anti-ageing effects. Furthermore, Jing et al. (Citation2018b) reported on the antioxidant, anti-ageing, and organ protection effects of water-extracted mycelium polysaccharide (MPS) and enzyme-extracted mycelium polysaccharide (En-MPS) from A. aegerita (Brig.) Sing. These polysaccharides displayed promising results in mitigating age-related effects in mice induced with D-galactose. Previous studies have suggested that trace elements possessed the potential to enhance the scavenging of free radicals by biological macromolecules such as polysaccharides, as well as antioxidant proteins (Lian et al. Citation2018; Lin et al. Citation2019). In line with these findings, Liu et al. (Citation2016) isolated mycelium selenium polysaccharide (MSPS) from the mycelium of A. cylindracea SL-02. This isolation was achieved by incorporating Na2SeO3 into the SL-02 medium. MSPS was found to be composed of rhamnose, arabinose, mannose, glucose, and galactose in a molar ratio of 29:3:1:18.8:2.7. Antioxidant experiments demonstrated the potential of MSPS in scavenging free radicals and enhancing the ability to reduce reactive oxygen species.
A polysaccharide called ACP’ was isolated from the fruiting body of A. cylindracea. ACP’ was composed of mannose, ribose, rhamnose, glucuronic acid, glucose, galactose, and xylose, with a molar ratio of 0.29:0.47:0.25:1.72:1.51:0.94:1. This study demonstrated that ACP’ exhibits significant benefits in high-calorie diet-induced obese mice (Zhu et al. Citation2022a, Citation2022b). It successfully reduced weight gain, improved insulin resistance, and alleviated lipid disorders. These research findings provided evidences for the positive role of ACP’ in combating diet-induced obesity and highlighted its potential as a promising probiotic formulation for obesity prevention.
Inhibiting the arginase (ARG) of Leishmania spp. is effective in the treatment of leishmaniasis. Motoshima et al. (Citation2018) isolated a heteropolysaccharide fucogalactan (FG-Aa) from the substrates of the A. aegerita, which inhibited the catalytic activity of ARG by 60.5% at a concentration of 10 µmol/L. The IC50 potency was 5.82 ± 0.57 µmol/L, showing excellent efficacy against leishmaniasis.
3. Lectins
Fungi are known to contain significant quantities of lectins, which are proteins or glycoproteins with the ability to agglutinate cells and selectively bind to carbohydrates. This binding ability enables lectins to cause agglutination of different cell types by targeting specific monosaccharides or oligosaccharides on the cell surface. Lectins were vital in several industries, like biotechnology, diagnostic/pharmacological research, and therapeutic applications (Singh et al. Citation1999). More than 400 different species of mushrooms were subjected to lectin assays by Pemberton (Citation1994), who discovered that around half of them carried lectins. Many of these lectin-containing mushrooms were edible, including Tricholoma mongolicum, Pleurotus ostreatus, and A. aegerita (Singh et al. Citation2014). Most of the edible mushroom lectins were obtained from their substrates and mycelium with molecular weights ranging from 12–68 kDa. Precipitation, adsorption chromatography, ion exchange chromatography, affinity chromatography, crystallisation, ultrafiltration, gel filtration chromatography, high-performance liquid chromatography (HPLC), and fast protein liquid chromatography (FPLC) were generally used to isolate and purify lectins (Yagi et al. Citation1997; Singh et al. Citation2014). A. aegerita Lectin was relatively stable, had good activity at 60 °C, and could still maintain activity in extremely acidic (pH 2.42) or extremely alkaline (pH 12.22) environments, which was relatively rare among lectin (Sun et al. Citation2003). The primary structure of A. cylindracea lectin was similar to that of fungal galectins, with 37% sequence homology to Coprinus galectins and only 19% sequence homology to human galectin-1 (Yagi et al. Citation2001; Liu et al. Citation2008).
Lectins represented a large and extensive family of proteins that shared a specific amino acid sequence motif in their carbohydrate recognition structural domain (CRD) (Thijssen et al. Citation2015; Sanjurjo et al. Citation2022), which included His-44, Arg-48, Val-59, Asn-61, Trp-68, Glu-71, and Arg-73, and which specifically recognised β-galactose (Yang et al. Citation2005). These proteins could be found in many species ranging from fungi to mammals and are involved in a diverse array of processes, including growth regulation, cell adhesion, cell migration, and immune reactions (Camby et al. Citation2001; Kaltner et al. Citation2017). Lectins typically possessed multiple hydrophilic and shallow binding pockets or boxes that allowed them to weakly interact with carbohydrates. However, to enhance the strength and specificity of carbohydrate-binding, many lectins existed as oligomers, which were complexes formed by several similar or identical monomers. This oligomeric structure enabled lectins to engage in multivalent binding and bound multiple carbohydrates simultaneously (Thakur et al. Citation2007; Singh et al. Citation2014). Yagi et al. (Citation1997) first reported the isolation of a lectin from A. cylindracea substrates, which was composed of two identical 15.8 kDa subunits polymerised to interact strongly with glycoconjugates such as lactose, N-Acetyl-D-lactosamine (LacNAc), NeuAcα2, 3Galβ1 and 3GlcNAc-/GalNAc (Yang et al. Citation2005). According to the report by Zhao et al. (Citation2003), they successfully isolated an anti-tumour lectin called AAL from the fruiting body of A. aegerita. Similar to the lectin found in A. cylindracea, AAL has a molecular weight of 15.8 kDa and is made up of two identical subunits. AAL demonstrated a potent inhibitory effect against SGC-7901, SW480, MGC80-3, BGC-823, S-180, and HeLa cells at a dose of 100 µg/mL, which was comparable to the inhibitory rate seen with adriamycin (also at a concentration of 100 µg/mL). In addition, Ye et al. (Citation2021) reported the immune-activating properties of AAL, which promoted the infiltration of T cells, NK cells, and macrophages into the liver, inhibited liver tumour growth, and extended the lifespan of tumour-bearing subjects. Mincle, a major receptor on leukocytes, recognises and binds to lectin-like carbohydrates (Zhang et al. Citation2015). Upon binding with AAL, Mincle mediated Syk phosphorylation and triggered the downstream Card9/NF-κB pathway. This induced macrophage polarisation, leading to the secretion of various pro-inflammatory cytokines and chemokines, including CXCL9, CCL2, CCL3, CCL5, and IL-15. These chemokines subsequently directed the migration of activated T cells and NK cells into hepatic tumour cells, significantly inhibiting tumour growth (). Wang et al. (Citation2002) reported on the isolation of another lectin with two distinct subunits (16.1 and 15.3 kDa) that displayed potent mitogenic activity against mouse splenocytes. Both subunits exhibited specificity for sialic acid and could be purified using ion exchange chromatography. Jiang et al. (Citation2012) isolated a new lectin called AAL-2 from A. aegerita, with a molecular weight of 43.175 kDa. AAL-2 showed a highly selective binding affinity for the terminal non-reducing GlcNAc residue. In vitro, AAL-2 could bind to the surface of liver cancer cells, and induce cell apoptosis, and in vivo, it demonstrated anti-liver cancer effects by slowing the growth of tumours and extending the survival period of mice with tumours. In addition to its anti-tumour effects, Lectin (AAL) has also demonstrated anti-metastatic activity in breast cancer. Yang et al. (Citation2018) demonstrated that AAL dramatically decreased the lung metastasis of 4T1 cells when delivered at doses of 1.5 mg/kg, and increased the survival time of mice with tumours.
4. Steroids
Steroids play a significant role as secondary metabolites in Agrocybe, and a total of eight steroids have been identified. In 2003, the sterol compound 5,8-epidioxy-ergosta-6,22-dien-3β-ol (1) and the known compound ergosterol (2) were first isolated from the fruiting body of A. aegerita. Compounds 1 and 2 both possessed COX inhibitory and antioxidant activities, with inhibition rates of 57% and 19% inhibition of COX-1 enzyme and inhibition rates of 22% and 28% inhibition of COX-2 enzyme at 100 µg/mL (Zhang et al. Citation2003). Kawagishi et al. (Citation2007) extracted six types of degraded sterols, which were formed by ergostane-type steroids through 5,6- and 9,10 oxidative cleavages resulting in the loss of the A ring, from the fruiting body of A. chaxingu. These sterols included chaxines A–E (3–7) (Kawagishi et al. Citation2007; Choi et al. Citation2009), and demethylincisterol A3 (8) (), in which the 2’S and 5’S stereochemistry structure of the A ring of compound 4 was initially determined to be wrong, and later revised to 2’R,5’S (Niki et al. Citation2020; Zhabinskii et al. Citation2022). Compound 8 showed inhibitory activity against Staphylococcus aureus, Vibrio parahaemolyticus, V. anguillarum, S. epidermidis, and Bacillus cereus. The inhibitory activity was significant against S. epidermidis and B. cereus, exhibiting a minimum inhibitory concentration (MIC) value of 3.13 µmol/L (Chen et al. Citation2015). Furthermore, 8 showed significant cytotoxicity against human cancer cell lines Hela, A549, and HepG2 with IC50 values of 0.17 nmol/L, 11.14 nmol/L, and 14.16 nmol/L, respectively, and the flow cytometer showed that 8 mainly inhibited the cell cycle in the G0/G1 phase, thereby apparently inducing apoptosis (Zhou et al. Citation2018). 3–7 had a strong inhibitory effect on osteoclasts, which could be used for the prevention and control of osteoporosis. The rate of osteoclast formation was reduced to 6.7% at a concentration of 1.6 µg/mL for 3, 4, and 5 significantly inhibited osteoclast formation to 66% and 0% at 3.1 mg/mL, respectively (Choi et al. Citation2009). 5 exhibited significant cytotoxicity against human cancer cell lines including Hela, HT29, HCT116, MCF-7, and Vero, with IC50 values ranging from 2.24 to 3.77 µg/mL (McCloskey et al. Citation2017).
5. Terpenoids
5.1. Volatile terpenoids
Based on the development process of the fruiting body of A. aegerita, a total of 22 volatile sesquiterpenes were detected. These sesquiterpenes served as precursors for different bioactive non-volatile substances, including ten cadinane-type sesquiterpenoids, γ-muurolene (9), δ-cadinene (10), α-Muurolene (11), Cadina-3,5-diene (12), Cubenene (13), Calamenene (14), Epicubenol (15), Cubenol (16), δ-Cadinol (17), and Epizonarene (18), two eudesmane-type sesquiterpenes, α-Selimene (19) and β-Selinene (20), and other types of sesquiterpenes β-Elemene (21), Germacrene D (22), β-Copaene (23), Viridiflorene (24), Longifolene (25), Δ6-protoilludene (26), Caryophyllene (27), α-Humulene (28), α-lsocomene (29) and trans-β-Ocimene (30) (Orban et al. Citation2020; Zhang et al. Citation2020) ().
5.2. Nonvolatile terpenoids
Illudins are a class of sesquiterpene antibiotics renowned for their diverse antibacterial and antitumour activities. Liu et al. (Citation2011) isolated a total of 10 illudin-type sesquiterpene compounds, agrocybins A–G (31–37), and compounds (2R)-2,3-dihydro-7-hydroxy-2-(hydroxymethyl)-2,4,6-trimethyl-1 H-indene-5-ethanol (38), illudin T (39), and illudin I (40) (). Their structures were established by spectrum data analysis and comparison with data from the existing literature (Wang et al. Citation2011). Interestingly, compounds 32–33 and 39–40 had similar spirocyclic structures, while 31 was a hexacyclic sesquiterpene which was highly cyclised and contained seven chiral carbons. The cytotoxicity assay was performed on five human cancer cell lines (HL-60, SMMC-7712, A549, MCF-7, and SW480) using MTT assay, and compounds 31–39 did not show significant activity at a concentration of 40 µmol/L. In earlier research, it was noted that a cyclopropane ring and a leaving group, such as a hydroxyl, on C-2 or C-4 were thought to be necessary for illudins to demonstrate cytotoxicity (Yamada et al. Citation2007). However, it is worth noting that in the case of the tested compounds mentioned, none of them satisfied this specific structural requirement. Compound 40 had moderate activity against B. subtilis, Escherichia coli, and S. aureus, and also showed certain cytotoxicity (Reina et al. Citation2004). Dai et al. (Citation2020) successfully isolated 13 illudin derivatives from the cultural broth of A. salicacola, including agrocybins H–K (41–44) and nine analogues, dihydrogranuloinden (45), echinolactone D (46), (2S,3S,9R)-14-hydroxy-5-desoxy-illudosin (47), sterostrein O (48), agrocybins D–G (34–37), and (2R)-2,3-dihydro-7-hydroxy-2-(hydroxymethyl)-2,4,6-trimethyl-1 H-indene-5-ethanol (49) (). Structurally, the carbon skeleton of compound 41 has never been described and was probably derived from the protoilludane skeleton by carbon-carbon bond breaking, and compounds agrocybins I–K (42–44) were aromatised illudin racemates, which were further isolated to give single enantiomers 42R/S and 43R/S. Among the illudin derivatives tested, compounds 41, 45, 47, and 48 demonstrated varying degrees of cytotoxicity against five different human cancer cell lines (HL60, SMMC-7721, A549, MCF-7, and SW480). Compound 41 displayed a particularly noteworthy cytotoxic effect on the SW480 cell line, with an IC50 value of 8.7 µmol/L. Compound 47 also exhibited significant cytotoxic effects on multiple cancer cell lines, including, IC50 values ranging from 7.6 to 21.7 µmol/L. Three aromadendrane-type sesquiterpenes 50–52 have been isolated from the culture of A. salicacola by (Zhu et al. Citation2010a). Notably, compound 50 differed from the common aromadendrane-type sesquiterpene and was a 2,3-secoaromadendrane-type sesquiterpene with a 5/7-ring cis-fusion. Biogenetically, Fomannosane-type sesquiterpenoids (FS) were classified as the largest group of sesquiterpenes derived from humulene, with the presence of a cyclobutane and a cyclopentane linked by carbon-carbon bonds. Liu et al. (Citation2012) obtained two new FS agrocybins H–I (53, 54) and illudosin (55) from the fermentation broth of A. salicacola, of which compound 55 might be an intermediate in the biosynthesis of the sesquiterpene fomannosin. Six marasmone type sesquiterpenes isolated from Agrocybe sp.: 1α,2β-dihydroxymarasmone (56), 2β,3α-dihydroxymarasmone (57),14-hydroxyoreadone (58), agrocybolacton (59), marasmene (60), 14-hydroxymarasmene (61) (Zheng et al. Citation2012). Compound 59 showed moderate activity against B. subtilis ATCC 6633 and Mycobacteria smegmatis SG987 at 50 µg/mL (Berg et al. Citation2002). From A. cylindracea Maire K3793 isolated protoilludene sesquiterpene metabolites, pasteurestin A–C (62–64). 62 and 63 exhibited significant inhibitory activity against the pathogenic bacterium Pasteurella haemolytica, which caused bovine pneumonia, with minimum inhibitory concentrations of 0.39 µg/mL and 0.78 µg/mL, respectively (Takeuchi et al. Citation2002; Surup et al. Citation2019).
5.3. Dimeric sesquiterpenes
The compound agrocybone (65) was a bis-sesquiterpene that was isolated from the fermentation broth of the fungus A. salicacola (). It is worth noting that the skeleton of 65 consisted of two spirocyclic carbons, which are highly unique in the realm of natural products. 65 had no inhibitory activity against cancer cell lines, but showed a weak antiviral activity against respiratory syncytial virus (RSV) with an IC50 value of 100 µmol/L (Zhu et al. Citation2010b). Surup et al. (Citation2019) isolated three compounds, bovistol A–C (66–68) from the liquid cultures of Cyclocybe aegerita. The compound bovistol was believed to be a dimeric sesquiterpene derived from the heterodimeric Diels-alder reaction of two sesquiterpenes. Compound 66 exhibited weak cytotoxic effects with IC50 values of 15 µg/mL for L929 and 7 µg/mL for KB3.1.
6. Alkyne compounds
The Agrocybe genus is known to produce plant growth regulators, including alkyne compounds produced by A. praecox that could stimulate abnormal fruit enlargement. Additionally, Agrocybe sp. has been found to cause shrinkage of strawberry fruits (Suzui et al. Citation1980). These findings demonstrated that different Agrocybe species and their compounds could have diverse effects on plant growth and development. Five analogues of polyacetylene, Agrocybynes A–E (69–73) (), were isolated from the culture broth of A. praecox by Fushimi et al. (Citation2012). Although Agrocybyne D (72) has been chemically synthesised, this is the first time it has been isolated from a natural source. Compounds 69–72 inhibited hypocotyl growth (29.6%, 70.4%, 38.4%, and 12.0% of the rate of length of growth compared to the control). 69, 71, and 72 inhibited root growth at 1 µmol/L (20.7%, 17.3%, and 6.67%). In the study conducted by Zheng et al. (Citation2016), a compound known as 8-Hydroxy-2,4,6-octatriynamide (74) was isolated from the fermentation broth of Agrocybe sp. The structure of 74 was determined through NMR, ESI, and X-ray single-crystal diffraction. 74 was identified as a natural polyacetylene and exhibited inhibitory effects against rice pathogens. Specifically, it displayed significant growth inhibition against Xanthomonas oryzae, with a minimum inhibitory concentration (MIC) of 53.1 µmol/L.
7. Other compounds
More and more studies have shown that there is a close relationship between brain ageing or related neurodegenerative diseases and oxidative stress (an imbalance between free radicals and antioxidant reserves) (Kandlur et al. Citation2020; Ionescu-Tucker and Cotman Citation2021), for instance, Alzheimer’s disease (Cabral-Costa and Kowaltowski Citation2020). Kim et al. (Citation1997) obtained two new indole radical scavengers, 6-hydroxy-1 H-indole-3-carboxaldehyde (75) and 6-hydroxy-1 H-indole-3-acetamide (76), from the MeOH extract of A. cylindracea that could inhibit lipid peroxidation in rat liver microsomes with IC50 values of 4.1 and 3.9 µg/mL, respectively. A structurally unique alkaloid Agrocybenine (77) was isolated from the fruiting body of A. cylindracea by CHCl3-MeOH gradient elution (Koshino et al. Citation1996). And, 2-formyl-3,5-dihydroxybenzyl acetate (78) and o-orsellinaldehyde (79) were isolated from the culture of A. praecox (Fushimi et al. Citation2012) (). DNA fragment analysis using flow cytometry and the presence of significant sub-G1 peaks suggested that compound 79 might mediate its cytotoxicity through cell apoptosis. According to the viability of Hep 3B and MRC-5 cells, the IC50 values were 3.6 and 33.1 µg/mL, respectively (Lin and Liu Citation2006). Diyabalanage et al. (Citation2008) isolated a ceramide (80) from the fruiting body of A. aegerita, which had a significant inhibitory effect on COX enzymes. At a concentration of 25 μg/mL, the inhibition rates of COX-1 and COX-2 enzymes reached 43% and 92.3%, respectively.
8. Biosynthesis
Terpenoids are a highly diverse group of natural products that exhibit a wide range of structures. They were found to be applied in various industries such as food, cosmetics, industrial raw materials, and pharmaceuticals. Terpene synthases were characterised by a wide variety of functions and products, resulting in a large number of terpenoids and complex structural types (Wu et al. Citation2022). Zhang et al. (Citation2020) identified 11 sesquiterpene synthases (STSs) of the fungus A. aegerita, by their engineered E. coli chassis strains designed for high terpene production, Agr1–Agr9 could biosynthesize one or more sesquiterpenes in E. coli.
Fungal sesquiterpene synthases could be divided into clades I–IV (). Comparing these 11 STS from A. aegerita with other fungal STS that have been characterised in previous studies [Clitopius pseudopinsitus (CpSTS), Coniophora puteana (Copu), Coprinopsis cinerea (Cop), Lignosus rhinocerotus (GME), Omphalotus olearius (Omp), Postia placenta (PpSTS), Stereum hirsutum (ShSTS), Termitomyces albuminosus (STC), Fomitopsis pinicola (Fompi1), Armillaria gallica (Pro1), Lactarius deliciosus (LdSTS)] were analysed together for phylogenetic tree analysis (). Results showed that Agr1 clustered with Cop1 and STC15, which were classified as highly promiscuous terpene synthases with α-muurolene as the major product [clade I (Light green colour)]. However, Agr1 had δ-cadinene as the major product, in which Cop1 had β-elemene as the major product and δ-cadinene as the minor product, and STC15 produced germacrene D-4-ol. Agr3 clustered with Cop3, CpSTS3, and Omp3, which also belong to the highly promiscuous terpene synthases group, with α-muurolene as their primary product (clade I, light green colour). Agr2, Agr5, CpSTS13, CpSTS12, and CpSTS9 were classified as terpene synthases belonging to clade II_A (green colour) and known to produce viridiflorol and viridiflorene as their main products. Agr4 clustered with Cop4 and CpSTS2, which were classified as highly promiscuous terpene synthases with δ-cadinene as the major product (clade II _B, apricot colour). Both Agr6 and Agr7 had Δ6-protoilludene as their unique product. These two enzymes, along with Omp6, CpSTS4, PpSTS08, ShSTS15, ShSTS16, ShSTS17, ShSTS18, and Pro1 were classified as Δ6-protoilludene synthetases (clade III, purple colour). Agr8 and Agr9 were not categorised in several of the above clades. These two enzymes were capable of producing several sesquiterpene compounds. γ-Muurolene and β-cadinene were the main products of Agr8, and β-selinene was also detected, as well as small amounts of α-selinene, β-elemene, and δ-cadinene. The main product of Agr9 was an unknown sesquiterpene, with small amounts of γ-Muurolene, β-cadinene, and δ-cadinene. By examining the evolutionary relationships and clustering patterns of known terpenoid synthase (TPS) enzymes through phylogenetic analysis, researchers can identify candidate genes or proteins that share sequence similarities with characterised TPS enzymes. This approach offers valuable insights into the potential functions and substrate specificities of these newfound TPS candidates. It helps in the exploration and discovery of novel terpenoid biosynthetic pathways within the fungal kingdom.
9. Conclusions and prospect
Edible macrofungi from the Agrocybe genus, such as A. aegerita, have increasingly become a significant component of daily diets and health supplements (Li et al. Citation2023). The bioactive compounds extracted from the fruiting bodies of these mushrooms are recognised for their high safety profile. However, their applications were hindered by several challenges, including the labour-intensive cultivation process, lengthy growth periods, and the low yield of secondary metabolites, which are typically not easily accessible. For instance, chaxine A, a compound employed in osteoporosis prevention, could only be isolated at a rate of 1 mg/kg of dried A. chaxingu fruiting bodies (Kawagishi et al. Citation2007). Despite these limitations, significant advancements in the synthesis of certain mushroom-derived secondary metabolites have been seen in recent years (Anke Citation2020). A notable example is ganoderic acid, a compound with potent anti-cancer properties. It is now produced via heterologous synthesis in yeast under optimised conditions, resulting in higher yields than direct extraction from mushrooms (Yuan et al. Citation2022).
Although this review introduced over 80 natural products from Agrocybe (), it is crucial to highlight that most of these compounds originate from the fermentation broth of A. salicacola and are present in minuscule quantities, complicating their isolations. However, we can enhance the production of these secondary metabolites through ab initio synthesis by judiciously modifying the host organism. Moreover, there is substantial potential to identify more compounds from the Agrocybe genus via biosynthesis or heterologous expression. This approach will not only deepen our understanding of Agrocybe, but also pave the way for further drug development and other potential applications.
Table 2. Biological activity of Agrocybe secondary metabolites and their producers.
Author contributions
LZ and CL conceived and designed the research. LZ, MY, and CL surveyed the scientific literature. LZ analysed data and wrote the draft manuscript. MY and CL interpreted the data and reviewed the manuscript. CL revised the manuscript. All authors read and approved the manuscript.
Disclosure statement
No potential conflict of interest was reported by the author(s).
Additional information
Funding
References
- Anke T. 2020. Secondary metabolites from mushrooms. J Antibiot (Tokyo). 73(10):655–656. doi: 10.1038/s41429-020-0358-6.
- Başaran N, Bacanlı M, Ahmet Başaran A. 2017. Chapter 28-lycopenes as antioxidants in gastrointestinal diseases. In: Gracia-Sancho J, and Salvadó J, editors. Gastrointestinal tissue. New York (NY): Academic Press; pp. 355–362.
- Berg A, Dörfelt H, Kiet TT, Schlegel B, Gräfe U. 2002. Agrocybolacton, a new bioactive metabolite from Agrocybe sp. HKI 0259. J Antibiot (Tokyo). 55(9):818–820. doi: 10.7164/antibiotics.55.818.
- Cabral-Costa JV, Kowaltowski AJ. 2020. Neurological disorders and mitochondria. Mol Aspects Med. 71:100826. doi: 10.1016/j.mam.2019.10.003.
- Camby I, Belot N, Rorive S, Lefranc F, Maurage CA, Lahm H, Kaltner H, Hadari Y, Ruchoux MM, Brotchi J, et al. 2001. Galectins are differentially expressed in supratentorial pilocytic astrocytomas, astrocytomas, anaplastic astrocytomas and glioblastomas, and significantly modulate tumor astrocyte migration. Brain Pathol. 11(1):12–26. doi: 10.1111/j.1750-3639.2001.tb00377.x.
- Chen M, Wang KL, Liu M, She ZG, Wang CY. 2015. Bioactive steroid derivatives and butyrolactone derivatives from a gorgonian-derived Aspergillus sp. fungus. Chem Biodivers. 12(9):1398–1406. doi: 10.1002/cbdv.201400321.
- Choi JH, Ogawa A, Abe N, Masuda K, Koyama T, Yazawa K, Kawagishi H. 2009. Chaxines B, C, D, and E from the edible mushroom Agrocybe chaxingu. Tetrahedron. 65(47):9850–9853. doi: 10.1016/j.tet.2009.09.064.
- Dai Q, Zhang FL, Du JX, Li ZH, Feng T, Liu JK. 2020. Illudane sesquiterpenoids from edible mushroom Agrocybe salicacola and their bioactivities. ACS Omega. 5(34):21961–21967. doi: 10.1021/acsomega.0c03314.
- Diyabalanage T, Mulabagal V, Mills G, DeWitt DL, Nair MG. 2008. Health-beneficial qualities of the edible mushroom, Agrocybe aegerita. Food Chem. 108(1):97–102. doi: 10.1016/j.foodchem.2007.10.049.
- Fayod V. 1889. Prodrome d’une histoire naturelle des agaricinés. [prodrome of a natural history of the Agaricales.]. Ann Sci Nat, Bot, Sér 4. 9:181–411.
- Fushimi K, Anzai K, Tokuyama S, Kiriiwa Y, Matsumoto N, Sekiya A, Hashizume D, Nagasawa K, Hirai H, Kawagishi H. 2012. Agrocybynes A–E from the culture broth of Agrocybe praecox. Tetrahedron. 68(4):1262–1265. doi: 10.1016/j.tet.2011.11.049.
- He MQ, Zhao RL, Hyde KD, Begerow D, Kemler M, Yurkov A, McKenzie EHC, Raspé O, Kakishima M, Sánchez-Ramírez S, et al. 2019. Notes, outline and divergence times of Basidiomycota. Fungal Divers. 99(1):105–367. doi: 10.1007/s13225-019-00435-4.
- Horak E. 1971. Contributions to the knowledge of the Agaricales s.L. (fungi) of New Zealand. N Z J Bot. 9(3):463–493. doi: 10.1080/0028825X.1971.10430194.
- Hyder MS, Dutta SD. 2021. Mushroom-derived polysaccharides as antitumor and anticancer agent: A concise review. Biocatal Agric Biotechnol. 35:102085. doi: 10.1016/j.bcab.2021.102085.
- Ionescu-Tucker A, Cotman CW. 2021. Emerging roles of oxidative stress in brain aging and Alzheimer’s disease. Neurobiol Aging. 107:86–95. doi: 10.1016/j.neurobiolaging.2021.07.014.
- Ji Y, Zheng MF, Ye SG, Wu XB, Chen JY. 2013. Agrocybe aegerita polysaccharide combined with chemotherapy improves tumor necrosis factor-α and interferon-γ levels in rat esophageal carcinoma. Dis Esophagus. 26(8):859–863. doi: 10.1111/j.1442-2050.2012.01397.x.
- Jia XW, Ma BJ, Xue F, Xing YQ, Wu P, Li TX, Shi FC, Xu CP. 2022. Structure characterization and anti-inflammatory activity of polysaccharides from Lingzhi or reishi medicinal mushroom ganoderma lucidum (Agaricomycetes) by microwave-assisted freeze-thaw extraction. Int J Med Mushrooms. 24(11):49–61. doi: 10.1615/IntJMedMushrooms.2022045268.
- Jiang S, Chen YJ, Wang M, Yin YL, Pan YH, Gu BL, Yu GJ, Li YM, Wong BH, Liang Y, et al. 2012. A novel lectin from Agrocybe aegerita shows high binding selectivity for terminal N-acetylglucosamine. Biochem J. 443(2):369–378. doi: 10.1042/bj20112061.
- Jing HJ, Li J, Zhang JJ, Wang WS, Li SS, Ren ZZ, Gao Z, Song XL, Wang XX, Jia L. 2018a. The antioxidative and anti-aging effects of acidic-and alkalic-extractable mycelium polysaccharides by Agrocybe aegerita (Brig.) Sing. Int J Biol Macromol. 106:1270–1278. doi: 10.1016/j.ijbiomac.2017.08.138.
- Jing HJ, Zhang Q, Liu M, Zhang JJ, Zhang C, Li SS, Ren ZZ, Gao Z, Liu XT, Jia L. 2018b. Polysaccharides with antioxidative and antiaging activities from enzymatic-extractable mycelium by Agrocybe aegerita (Brig.) Sing. Evid Based Complementary Altern Med. 2018:1584647. doi: 10.1155/2018/1584647.
- Kaltner H, Toegel S, Caballero GG, Manning JC, Ledeen RW, Gabius HJ. 2017. Galectins: Their network and roles in immunity/tumor growth control. Histochem Cell Biol. 147(2):239–256. doi: 10.1007/s00418-016-1522-8.
- Kandlur A, Satyamoorthy K, Gangadharan G. 2020. Oxidative stress in cognitive and epigenetic aging: A retrospective glance. Front Mol Neurosci. 13:41. doi: 10.3389/fnmol.2020.00041.
- Kawagishi H, Akachi T, Ogawa T, Masuda K, Yamaguchi K, Yazawa K, Takahashi M. 2007. Chaxine A, an osteoclast-forming suppressing substance, from the mushroom Agrocybe chanxingu. ChemInform. 38(19). doi: 10.1002/chin.200719169.
- Kiho T, Sobue S, Ukai S. 1994. Structural features and hypoglycemic activities of two polysaccharides from a hot-water extract of Agrocybe cylindracea. Carbohydr Res. 251:81–87. doi: 10.1016/0008-6215(94)84277-9.
- Kiho T, Yoshida I, Nagai K, Ukai S, Hara C. 1989. (1→3)-α-D-glucan from an alkaline extract of agrocybe cylindracea, and antitumor activity of its O-(carboxymethyl)ated derivatives. Carbohydr Res. 189:273–279. doi: 10.1016/0008-6215(89)84103-5.
- Kim WG, Lee IK, Kim JP, Ryoo IJ, Koshino H, Yoo ID. 1997. New indole derivatives with free radical scavenging activity from Agrocybe cylindracea. J Nat Prod. 60(7):721–723. doi: 10.1021/np970150w.
- Koshino H, Lee IK, Kim JP, Kim WG, Uzawa J, Yoo ID. 1996. Agrocybenine, novel class alkaloid from the Korean mushroom Agrocybe cylindracea. Tetrahedron Lett. 37(26):4549–4550. doi: 10.1016/0040-4039(96)00900-8.
- Lee BR, Lee YP, Kim DW, Song HY, Yoo KY, Won MH, Kang TC, Lee KJ, Kim KH, Joo JH, et al. 2010. Amelioration of streptozotocin-induced diabetes by Agrocybe chaxingu polysaccharide. Mol Cells. 29(4):349–354. doi: 10.1007/s10059-010-0044-9.
- Li JX, Yang WQ, Ren JW, Cao B, Zhu XY, Lin L, Ye W, Zhao RL. 2023. A new species Agrocybe striatipes, also a newly commercially cultivated mushroom with highly nutritional and healthy values. J Fungi. 9(3):383. doi: 10.3390/jof9030383.
- Lian KX, Zhu XQ, Chen J, Liu G, Gu XL. 2018. Selenylation modification: enhancement of the antioxidant activity of a Glycyrrhiza uralensis polysaccharide. Glycoconj J. 35(2):243–253. doi: 10.1007/s10719-018-9817-8.
- Lin JT, Liu WH. 2006. ο-orsellinaldehyde from the submerged culture of the edible mushroom Grifola frondosa exhibits selective cytotoxic effect against hep 3B cells through apoptosis. J Agric Food Chem. 54(20):7564–7569. doi: 10.1021/jf0616762.
- Lin TY, Chang CC, Tseng AJ, Chao CH, Lu MK. 2019. Sodium thiosulfate enhances production of polysaccharides and anticancer activities of sulfated polysaccharides in Antrodia cinnamomea. Carbohydr Polym. 216:204–212. doi: 10.1016/j.carbpol.2019.04.017.
- Liu C, Dai KY, Li ZT, Liu AJ. 2023. Comparison of two kinds of Agrocybe cylindracea polysaccharides: structural characteristic and antitumor activity. Food Bioprod Process. 140:160–171. doi: 10.1016/j.fbp.2023.06.001.
- Liu C, Zhao X, Xu XC, Li LR, Liu YH, Zhong SD, Bao JK. 2008. Hemagglutinating activity and conformation of a lactose-binding lectin from mushroom Agrocybe cylindracea. Int J Biol Macromol. 42(2):138–144. doi: 10.1016/j.ijbiomac.2007.10.017.
- Liu LY, Li ZH, Dong ZJ, Li XY, Su J, Li Y, Liu JK. 2012. Two novel fomannosane-type sesquiterpenoids from the culture of the basidiomycete Agrocybe salicacola. Natur Prod Bioprosp. 2(3):130–132. doi: 10.1007/s13659-012-0031-2.
- Liu LY, Zhang L, Feng T, Li ZH, Dong ZJ, Li XY, Su J, Li Y, Liu JK. 2011. Unusual illudin-type sesquiterpenoids from cultures of Agrocybe salicacola. Natur Prod Bioprosp. 1(2):87–92. doi: 10.1007/s13659-011-0018-4.
- Liu M, Jing HJ, Zhang JJ, Che G, Zhou M, Gao Z, Li SS, Ren ZZ, Hao L, Liu Y, et al. 2016. Optimization of mycelia selenium polysaccharide extraction from Agrocybe cylindracea SL-02 and assessment of their antioxidant and anti-ageing activities. PLoS One. 11(8):e0160799. doi: 10.1371/journal.pone.0160799.
- Liu XY, Liu D, Chen YH, Zhong RT, Gao LY, Yang CF, Ai C, El-Seedi HR, Zhao C. 2020. Physicochemical characterization of a polysaccharide from Agrocybe aegirita and its anti-ageing activity. Carbohydr Polym. 236:116056. doi: 10.1016/j.carbpol.2020.116056.
- Matheny PB, Curtis JM, Hofstetter V, Aime MC, Moncalvo JM, Ge ZW, Slot JC, Ammirati JF, Baroni TJ, Bougher NL, et al. 2006. Major clades of Agaricales: A multilocus phylogenetic overview. Mycologia. 98(6):982–995. doi: 10.1080/15572536.2006.11832627.
- McCloskey S, Noppawan S, Mongkolthanaruk W, Suwannasai N, Senawong T, Prawat U. 2017. A new cerebroside and the cytotoxic constituents isolated from Xylaria allantoidea SWUF76. Nat Prod Res. 31(12):1422–1430. doi: 10.1080/14786419.2016.1258559.
- Motoshima RA, Rosa TDF, Mendes LDC, Silva EVD, Viana SRF, Amaral BSD, de Souza DHF, Lião LM, Corradi da Silva ML, de Sousa LRF, et al. 2018. Inhibition of leishmania amazonensis arginase by fucogalactan isolated from Agrocybe aegerita mushroom. Carbohydr Polym. 201:532–538. doi: 10.1016/j.carbpol.2018.08.109.
- Niki M, Hirata Y, Nakazaki A, Wu J, Kawagishi H, Nishikawa T. 2020. Biomimetic synthesis of chaxine and its related compounds. J Org Chem. 85(7):4848–4860. doi: 10.1021/acs.joc.9b03482.
- Orban A, Hennicke F, Rühl M. 2020. Volatilomes of cyclocybe aegerita during different stages of monokaryotic and dikaryotic fruiting. Biol Chem. 401(8):995–1004. doi: 10.1515/hsz-2019-0392.
- Pemberton RT. 1994. Agglutinins (lectins) from some British higher fungi. Mycol Res. 98(3):277–290. doi: 10.1016/S0953-7562(09)80455-3.
- Reina M, Orihuela JC, González-Coloma A, de Inés C, de la Cruz M, González Del Val A, Torno JR, Fraga BM. 2004. Four illudane sesquiterpenes from Coprinopsis episcopalis. Phytochemistry. 65(4):381–385. doi: 10.1016/j.phytochem.2003.10.023.
- Rosdan Bushra SM, Nurul AA. 2022. Bioactive mushroom polysaccharides: the structure, characterization and biological functions. J Liq Chromatogr Relat Technol. 45(13–16):174–190. doi: 10.1080/10826076.2023.2182317.
- Sanjurjo L, Broekhuizen EC, Koenen RR, Thijssen V. 2022. Galectokines: the promiscuous relationship between galectins and cytokines. Biomolecules. 12(9). doi: 10.3390/biom12091286.
- Singer R 1975. Agaricales in modern taxonomy. 3rd ed. Vaduz: Cramer.
- Singh RS, Tiwary AK, Kennedy JF. 1999. Lectins: sources, activities, and applications. Crit Rev In Biotechnol. 19(2):145–178. doi: 10.1080/0738-859991229224.
- Singh SS, Wang H, Chan YS, Pan W, Dan X, Yin CM, Akkouh O, Ng TB. 2014. Lectins from edible mushrooms. Molecules. 20(1):446–469. doi: 10.3390/molecules20010446.
- Sun H, Zhao CG, Tong C, Qi YP. 2003. Purification and characterization of a novel lectin AAVP from fruiting bodies of the edible fungus, Agrocybe aegerita. Chin J Biochem Mol Biol. 1:96–102. doi: 10.13865/j.cnki.cjbmb.2003.01.022.
- Surup F, Hennicke F, Sella N, Stroot M, Bernecker S, Pfütze S, Stadler M, Rühl M. 2019. New terpenoids from the fermentation broth of the edible mushroom cyclocybe aegerita. Beilstein J Org Chem. 15:1000–1007. doi: 10.3762/bjoc.15.98.
- Suzui K, Makino T, Otani Y. 1980. Agrocybe species causing stunt syndrome of strawberries. Ann Phytopathol Soc Jpn. 46:396. doi: 10.3186/jjphytopath.46.46.
- Takeuchi T, Iinuma H, Momose I, Matsui S. 2002. New antibiotics pasteurestin a and B, and method for producing the same. JP 2001–9452 20010117.
- Thakur A, Pal L, Ahmad A, Khan MI. 2007. Complex carbohydrate specificity of lectin from fruiting body of Ganoderma lucidum. A surface plasmon resonance study. Iubmb Life. 59(12):758–764. doi: 10.1080/15216540701663463.
- Thijssen VL, Heusschen R, Caers J, Griffioen AW. 2015. Galectin expression in cancer diagnosis and prognosis: a systematic review. Biochim Biophys Acta Rev Cancer. 1855(2):235–247. doi: 10.1016/j.bbcan.2015.03.003.
- Walther G, Weiss M. 2006. Anamorphs of the Bolbitiaceae (Basidiomycota, Agaricales). Mycologia. 98(5):792–800. doi: 10.1080/15572536.2006.11832650.
- Wang G, Liu LY, Zhu YC, Liu JK. 2011. Illudin T, a new sesquiterpenoid from basidiomycete Agrocybe salicacola. J Asian Nat Prod Res. 13(5):430–433. doi: 10.1080/10286020.2011.566218.
- Wang H, Ng TB, Liu Q. 2002. Isolation of a new heterodimeric lectin with mitogenic activity from fruiting bodies of the mushroom Agrocybe cylindracea. Life Sci. 70(8):877–885. doi: 10.1016/S0024-3205(01)01463-1.
- Wang YX, Zhang T, Huang XJ, Yin JY, Nie SP. 2021. Heteroglycans from the fruiting bodies of agrocybe cylindracea: fractionation, physicochemical properties and structural characterization. Food Hydrocoll. 114:106568. doi: 10.1016/j.foodhyd.2020.106568.
- Wang ZW, Chen KY, Zhang K, He KH, Zhang DD, Guo XH, Huang TW, Hu JL, Zhou XT, Nie S. 2023. Agrocybe cylindracea fucoglucogalactan induced lysosome-mediated apoptosis of colorectal cancer cell through H3K27ac-regulated cathepsin D. Carbohydr Polym. 319:121208. doi: 10.1016/j.carbpol.2023.121208.
- Watling R. 1982. British fungus flora: agarics and Boleti. 3/Bolbitiaceae: Agrocybe, bolbitius & conocybe. Edinburgh: Royal Botanic Gardens.
- Watling R, Taylor GM. 1987. Observations on the Bolbitiaceae: 27. Preliminary account of the Bolbitiaceae of New Zealand. Bibliotheca Mycologica Band. 117:1–61.
- Wu JJ, Yang XY, Duan YC, Wang PC, Qi JZ, Gao JM, Liu CW. 2022. Biosynthesis of sesquiterpenes in basidiomycetes: A review. J Fungi. 8(9):913. doi: 10.3390/jof8090913.
- Wu TJ, Cai Z, Niu FD, Qian B, Sun P, Yang N, Pang J, Mei HL, Chang XA, Chen F, et al. 2023. Lentinan confers protection against type 1 diabetes by inducing regulatory T cell in spontaneous non-obese diabetic mice. Nutr Diabetes. 13(1):4. doi: 10.1038/s41387-023-00233-7.
- Xiao HL, Wei C, Liu HY, Li ZQ, Zheng CH, Luo J. 2023. Lentinan alleviates sciatic nerve injury by promoting autophagy to remove myelin fragments. Phytother Res. 37(9):4042–4058. doi: 10.1002/ptr.7862.
- Xu JJ, Wu MC, Chen P. 2005. The influence of Agrocybe aegerita polysaccharides (AAP) on bearing tumor animal model. Food Sci. 6:246–248. doi: 10.1111/j.1744-7909.2005.00136.x.
- Yagi F, Hiroyama H, Kodama S. 2001. Agrocybe cylindracea lectin is a member of the galectin family. Glycoconj J. 18(10):745–749. doi: 10.1023/a:1021193432577.
- Yagi F, Miyamoto M, Abe T, Minami Y, Tadera K, Goldstein IJ. 1997. Purification and carbohydrate-binding specificity of Agrocybe cylindracea lectin. Glycoconj J. 14(2):281–288. doi: 10.1023/a:1018558225454.
- Yamada K, Ojika M, Kigoshi H. 2007. Ptaquiloside, the major toxin of bracken, and related terpene glycosides: chemistry, biology and ecology. Nat Prod Rep. 24(4):798–813. doi: 10.1039/b614160a.
- Yang N, Tong X, Xiang Y, Zhang Y, Liang Y, Sun H, Wang DC. 2005. Molecular character of the recombinant antitumor lectin from the edible mushroom Agrocybe aegerita. J Biochem. 138(2):145–150. doi: 10.1093/jb/mvi109.
- Yang Q, Yin YL, Pan YF, Ye XD, Xu B, Yu WH, Zeng HL, Sun H. 2018. Anti-metastatic activity of Agrocybe aegerita galectin (AAL) in a mouse model of breast cancer lung metastasis. J Funct Foods. 41:163–170. doi: 10.1016/j.jff.2017.12.058.
- Yang Y, Li JH, Hong Q, Zhang XH, Liu ZM, Zhang TH. 2022. Polysaccharides from Hericium erinaceus fruiting bodies: structural characterization, immunomodulatory activity and mechanism. Nutrients. 14(18):3721. doi: 10.3390/nu14183721.
- Ye XD, Wang XQ, Yu WH, Yang Q, Li Y, Jin YX, Su YT, Song JQ, Xu B, Sun H. 2021. Synergistic effects of AAGL and anti-PD-1 on hepatocellular carcinoma through lymphocyte recruitment to the liver. Cancer Biol Med. 18(4):1092–1108. doi: 10.20892/j.issn.2095-3941.2020.0278.
- Yuan W, Jiang CJ, Wang Q, Fang YB, Wang J, Wang M, Xiao H. 2022. Biosynthesis of mushroom-derived type II ganoderic acids by engineered yeast. Nat Commun. 13(1):7740. doi: 10.1038/s41467-022-35500-1.
- Zhabinskii VN, Drasar P, Khripach VA. 2022. Structure and biological activity of ergostane-type steroids from fungi. Molecules. 27(7). doi: 10.3390/molecules27072103.
- Zhang CQ, Chen XX, Orban A, Shukal S, Birk F, Too HP, Rühl M. 2020. Agrocybe aegerita serves as a gateway for identifying sesquiterpene biosynthetic enzymes in higher fungi. ACS Chem Biol. 15(5):1268–1277. doi: 10.1021/acschembio.0c00155.
- Zhang Y, Mills GL, Nair MG. 2003. Cyclooxygenase inhibitory and antioxidant compounds from the fruiting body of an edible mushroom, Agrocybe aegerita. Phytomedicine. 10(5):386–390. doi: 10.1078/0944-7113-00272.
- Zhang ZJ, He L, Hu S, Wang Y, Lai QH, Yang P, Yu QL, Zhang S, Xiong F, Simsekyilmaz S, et al. 2015. AAL exacerbates pro-inflammatory response in macrophages by regulating Mincle/Syk/Card9 signaling along with the Nlrp3 inflammasome assembly. Am J Transl Res. 7(10):1812–1825.
- Zhao C, Sun H, Tong X, Qi YP. 2003. An antitumor lectin from the edible mushroom Agrocybe aegerita. Biochem J. 374(Pt 2):321–327. doi: 10.1042/bj20030300.
- Zhao HJ, Li J, Zhang JJ, Wang XX, Hao L, Jia L. 2017. Purification, in vitro antioxidant and in vivo anti-aging activities of exopolysaccharides by agrocybe cylindracea. Int J Biol Macromol. 102:351–357. doi: 10.1016/j.ijbiomac.2017.04.039.
- Zheng YB, Lu CH, Xu L, Sun WJ, Shen YM. 2012. Three new sesquiterpenoids from Agrocybe sp. Chem Res Chin Univ. 28:976–979. doi: 10.1055/s-1327871.
- Zheng YB, Xu XP, Wu YB. 2016. Inhibitory activities against rice pathogens of 8-hydroxy-2,4,6-octatriynamide from Agrocybe sp. World J Microbiol Biotechnol. 32(3):35. doi: 10.1007/s11274-015-2004-1.
- Zhou J, Li G, Deng Q, Zheng DY, Yang XB, Xu J. 2018. Cytotoxic constituents from the mangrove endophytic Pestalotiopsis sp. induce G (0)/G (1) cell cycle arrest and apoptosis in human cancer cells. Nat Prod Res. 32(24):2968–2972. doi: 10.1080/14786419.2017.1395431.
- Zhu YC, Wang G, Liu JK. 2010a. Two new sesquiterpenoids from basidiomycete Agrocybe salicacola. J Asian Nat Prod Res. 12(6):464–469. doi: 10.1080/10286020.2010.489822.
- Zhu YC, Wang G, Yang XL, Luo DQ, Zhu QC, Peng T, Liu JK. 2010b. Agrocybone, a novel bis-sesquiterpene with a spirodienone structure from basidiomycete Agrocybe salicacola. Tetrahedron Lett. 51(26):3443–3445. doi: 10.1016/j.tetlet.2010.04.128.
- Zhu ZJ, Huang R, Huang AH, Wang J, Liu W, Wu SJ, Chen MF, Chen MT, Xie YZ, Jiao CW, et al. 2022a. Polysaccharide from Agrocybe cylindracea prevents diet-induced obesity through inhibiting inflammation mediated by gut microbiota and associated metabolites. Int J Biol Macromol. 209(Pt A):1430–1438. doi: 10.1016/j.ijbiomac.2022.04.107.
- Zhu ZJ, Huang R, Liu W, Wang J, Wu SJ, Chen MF, Huang AH, Xie YZ, Chen MT, Jiao CW, et al. 2022b. Whole Agrocybe cylindracea prevented obesity linking with modification of gut microbiota and associated fecal metabolites in high-fat diet-fed mice. Mol Nutr Food Res. 66(7):e2100897. doi: 10.1002/mnfr.202100897.