ABSTRACT
Understanding the composition and community of airborne mycobiota associated with fungal diseases was critical for mushroom production and disease control. In this study, we first investigated airborne fungi concentrations in different function areas of a Chinese shiitake factory. Then, high-throughput sequencing was employed to characterise the airborne fungal compositions and communities of this factory during high and low incidence seasons of shiitake artificial log rot disease (SLRD). The lowest concentration of culturable airborne fungi was observed in spawn-running greenhouse (250–450 CFU/m3), while the airborne fungi concentrations in cultivation greenhouses were up to 7,000–8,500 CFU/m3. The airborne fungal communities across distinct function areas showed convergence. The four major mushroom contaminants, Trichoderma, Penicillium, Cladosporium, and Aspergillus were the stable core fungi. Seasonal shifts in compositions, abundances, and communities of airborne fungi were systematically analysed and found to be associated with SLRD. Compared with the SLRD low incidence season, the airborne fungal communities were rich but uneven in the SLRD high incidence season, in which Trichoderma was dominant. Further analysis indicated that the favourable temperature and humidity caused the proliferation of Trichoderma and then affected the fungal communities, which presented an SLRD-seasonal variation. SLRD incidence could be significantly reduced by reasonably increasing the ambient temperature. For the first time, we comprehensively characterised the dynamics of airborne fungal communities across the SLRD occurrence in the shiitake factory. Findings here also provided valuable information for fungal exposures in the mushroom industry.
1. Introduction
Mushrooms are of great importance in ecology, agriculture, industry, and medicine (Zhang et al. Citation2021). Owing to the multiple health-promoting properties and cost-effective production, global mushroom production has increased rapidly in recent decades (Grimm and Wösten Citation2018). Especially, the intensive production of edible mushrooms in well-controlled greenhouses (also called mushroom factories) has greatly promoted the efficiency of mushroom farming by offering a standardised and all-year-round production mode (Chen et al. Citation2022). Of particular note, the mushroom greenhouses contain abundant organic substrates (wet wood sawdust, cornstalk, and wheat bran, etc.), which provide not only favourable environments for mushroom growth but also the growth of mushroom contaminants such as fungi and bacteria (Ficociello et al. Citation2019). Under the intensive production of mushrooms, many microbes may contaminate mushrooms and cause huge losses.
Among cultivated edible mushrooms, Lentinula edodes (shiitake) is one of the most widely grown species worldwide, contributing to about 22% of the world’s total production (Zhang et al. Citation2021). In recent years, Shandong Qihe Biotechnology Co., Ltd. (hereafter as Qihe) in China has realised year-round fruiting of shiitake in the temperature and humidity-controlled cultivation greenhouses (CGs). Shiitake artificial log rot disease (SLRD) (Figure S1), also called green mold disease, is a common disease during spawn running and fruiting in shiitake cultivation (Wang et al. Citation2016; Ma et al. Citation2021). During the occurrence of SLRD, the infected mycelia of L. edodes in cultivated artificial logs became wilted and finally died, while the artificial logs initially became soft and finally became rotten and loose (Cao et al. Citation2015; Wang et al. Citation2016). Mainly caused by Trichoderma spp. infections, SLRD is one of the most serious fungal diseases that severely affect yield and quality of shiitake (Wang et al. Citation2016; Ma et al. Citation2021). Since 2017, SLRD has occurred annually in CGs of Qihe in autumn and winter of China, with an incidence rate of up to 40%. For shiitake production and disease control, it is important to characterise the composition and community of airborne mycobiota associated with fungal diseases, including SLRD.
Culture-dependent approaches have only been able to capture a small fraction of microbes. Thereby, the composition of the microbiome could not be sufficiently investigated by culture-dependent approaches (Gao et al. Citation2022). The development of high-throughput sequencing provided robust tools for the fine-scale molecular characterisation of microbial diversity and community (Nilsson et al. Citation2018). In recent years, high-throughput sequencing has been widely used to investigate microbial communities in a variety of environments (Małek et al. Citation2021; Xue et al. Citation2023). In edible mushrooms, such as Agaricus bisporus and Morchella spp. (Ban et al. Citation2023; Zhang et al. Citation2023), relevant studies mainly focused on the dynamics of microbial communities in compost substrates or soils and their impacts on mushroom growth and development. Of these, only a few have been conducted on the airborne microbial communities. Based on the culture-dependent approach, Cai et al. (Citation2020) showed that Penicillium and Cladosporium were the dominant fungi in the cooling and inoculation rooms of the Flammulina filiformis factory. While in the Pleurotus eryngii factory, the dominant fungal genera were Aspergillus and Cladosporium (Qin et al. Citation2020). At present, the use of high-throughput sequencing to characterise the composition and community of airborne mycobiota in the production environment of edible mushrooms is rare, but it is urgently needed.
Currently, little is known about airborne mycobiota during the occurrence of fungal diseases and their impacts on mushroom production and workers’ health. Here in this study, we first investigated the airborne fungi concentrations in a shiitake factory. Then, high-throughput sequencing was employed to investigate the shift of airborne fungal communities in different function areas of this factory across the occurrence of SLRD. The relationships between fungal communities, incidence rates of SLRD, and environmental parameters were further explored. The present study aimed to characterise the dynamics of airborne fungal communities in a shiitake factory, and to explore the pathogenesis of SLRD. Findings here would be of great significance for shiitake production and disease control, and also provide new insight on occupational exposure to fungal bioaerosols in the mushroom industry.
2. Material and methods
2.1. Sampling sites and schedules
In this study, all the air samples were collected from Shandong Qihe Biotechnology Co., Ltd. (117°54′ E, 36°39′ N), a shiitake factory located in Zichuan district of Shandong Province in China. In Qihe, compared with other seasons (incidence rate less than 10%), the incidence of SLRD in cultivation greenhouses increased greatly in autumn and winter of China, up to 40%. The FA-1 Andersen six-stage cascade impactor (Ju Chuang Group Co., Ltd., China) was used to collect airborne mycobiota from four sites [outdoor space (OS), spawn-running greenhouse (SG), cultivation greenhouse A (CGA), and cultivation greenhouse B (CGB)] in Qihe (). OS was a windward site without obstruction, SG was a greenhouse for shiitake mycelia growth, and CGA and CGB were two cultivation greenhouses for shiitake fruiting. The DHM2 mechanical ventilation hygrometer was used to measure the temperature and relative humidity of each sampling site.
To explore the seasonal discrepancy of airborne fungal communities, samples were collected in two periods (SLRD high incidence season: From September to December 2021; and SLRD low incidence season: From May to July 2022 in China). Since the whole harvest time of shiitake in CGs lasts a long time, the fruiting period of shiitake was further subdivided into the first flush period (FF), the interval period between two fruiting flushes (IBF), and the second flush period (SF). All four sites were sampled once every seven days. The detailed sampling sites, schedules, as well as temperature and humidity parameters were shown in Table S1.
For simplicity, we have added the prefix HI (high incidence) to the names of samples collected from September to December 2021. Correspondingly, samples collected from May to July 2022 were prefixed with LI (low incidence). For instance, the sample was named HI_A_FF1, meaning that it was the first air sample collected during the first flush period in cultivation greenhouse A (CGA) from September to December 2021 (, Table S1).
2.2. Sampling method
The air samples were collected by using the FA-1 Andersen six-stage sampler with a flow rate of 28.3 L/min (Yu et al. Citation2013). For cultivable fungi, on each layer of the sampler, one 9.0 cm Petri dish containing 15 mL potato dextrose agar medium (PDA, potatoes infusion 200 g/L, dextrose 20 g/L, and agar 20 g/L) supplemented with streptomycin was used to collect airborne fungi. Each sampling lasted about 5 min with three replicates at each site. The airborne fungi were then cultured in PDA media at 25 °C for 72 h. After that, the number of airborne fungal colonies at each stage was counted and statistically corrected using the Positive-hole method (Lu et al. Citation2017). The concentration of airborne fungi was calculated as c = N × 1,000/t × Q, where c was the airborne fungal concentrations (CFU/m3); N represented the total number of colonies after correction (CFU); Q was the sampler air flow rate (L/min), and t was the sampling time (min) (Yu et al. Citation2013).
For airborne microbiome analysis, the cellulose acetate membrane filters (47 mm diameter and 0.22 µm pore size) were placed on the first stage of the FA-1 sampler. Each sampling lasted 10 min with three replicates. After completing air sampling, the samples from three replicates were pooled in a cryopreservation tube and stored in liquid nitrogen until high-throughput sequencing. The samples collected in SLRD HI and LI seasons were sequenced respectively. For SG, the environmental conditions (temperature and humidity) remained constant on an annual basis; therefore, only the samples in one shiitake mycelia growth cycle (84 days) in SLRD HI season were used for high-throughput sequencing.
2.3. DNA extraction, PCR amplifications, and high-throughput sequencing
DNA was extracted from each filter using the TGuide S96 Magnetic Soil/Stool DNA Kit (ID: DP812, Tiangen Biotech Co., Ltd., Beijing, China), according to the manufacturer’s instructions. The DNA concentrations of the samples were measured with the Qubit dsDNA HS Assay Kit and Qubit 4.0 Fluorometer (Invitrogen, Thermo Fisher Scientific, Eugene, OR, USA). The ITS2 regions of the DNA samples were then amplified with ITS2F (5‘-GCATCGATGAAGAACGCAGC-3‘) and ITS2R (5’-TCCTCCGCTTATTGATATGC-3’) primers (Ding et al. Citation2015). Each 10 mL PCR reaction system included 5–50 ng of genomic DNA, 0.3 mL each of ITS2 primers, 0.2 mL KOD FX Neo, 5 mL KOD FX Neo Buffer (Beijing Biolink Biotechnology Co., Ltd., Beijing, China), and 2 mL dNTPs. PCR reactions were carried out with the following thermal profile: 95 °C for 5 min, followed by 35 cycles of 95 °C for 30 s, 50 °C for 30 s, 72 °C for 40 s, followed by a cycle of 72 °C for 7 min, and a hold of 4 °C. The PCR products from the target region (10 mL system) were mixed with VAHTSTM DNA Clean Beads (Vazyme Biotech Co., Ltd., Nanjing, China) in a 1:1 ratio, and the beads were then used to select the sizes of the amplicons to generate high-quality libraries. The libraries were sequenced using the Illumina MiSeq system with the paired-end strategy (Biomarker Technologies, Beijing, China).
2.4. Bioinformatic and statistical analyses
During data processing, FLASH v1.2.11 with default settings was used to merge the paired-end reads and convert them to FASTA format (Magoc and Salzberg Citation2011). Mothur v.1.45.3 was then used to remove the low-quality sequences (average quality score ≤ 20, containing N, homopolymers > 10 bp, primer mismatches ≥ 4 bp, less than 200 bp, over 500 bp) and the primer sequences (Schloss et al. Citation2009). After removing chimeras and singletons by UCHIME V4.2 (Edgar et al. Citation2011), the filtered sequences were clustered into operational taxonomic units (OTUs) at 97% similarity criterion using USEARCH v11.0.667 (Edgar Citation2013). Histograms of relative abundances of airborne fungi at the phylum and genus level were visualised using ggplot2 (https://github.com/tidyverse/ggplot2). The fungi with relative abundance > 1% in at least two samples were defined as the dominant genus.
To comprehensively characterise the airborne fungal communities, data of L. edodes was removed from the subsequent analysis. Alpha diversity of airborne fungal communities was analysed using Shannon and Chao1 indices using Mothur v.1.45.3 (Schloss et al. Citation2009). The significant differences in fungal richness and compositions between samples were analysed by Kruskal-Wallis tests with a 0.05 p-value threshold. The beta diversity of fungal OTUs was estimated using principal coordinate analysis (PCoA) based on Bray Curtis metrics (Liu et al. Citation2018). The ANOSIM (analysis of similarities) test was performed to examine the significance by using the vegan package of R software (http://mirrors.ustc.edu.cn/CRAN/web/packages/vegan/). Biomarkers between samples were identified by the linear discriminant analysis effect size (LEfSe), with the LDA threshold of 4.0 (Segata et al. Citation2011). Finally, the distribution characteristics of Trichoderma abundance, temperature, and relative humidity across SLRD high- and low-incidence seasons were visualised by constructing a three-dimensional map.
2.5. Influence of air temperature on SLRD incidence rate
To verify our hypothesis that temperature in CGs was the key factor causing the high incidence of SLRD, a fruiting trial was performed. A total of 1,200 artificial logs of two shiitake varieties (L2 and L9, with 600 logs. respectively) were prepared by the standard cultivation procedures of shiitake (Gui Citation2022). Briefly, the substrates (consisting of 79% hardwood sawdust, 20% wheat bran, and 1% gypsum, with a moisture content of 60%) were mixed and filled into plastic culture bags (15 cm in fold diameter, 55 cm in length). These culture bags were then autoclaved at 121 °C for 120 min, cooled to 25 °C, and then inoculated with shiitake spawn (Gong et al. Citation2014). The weight proportion of inoculated spawn to sawdust medium was approximately 3% (w/w). Then, these shiitake artificial logs (culture bags) were incubated at 24 °C in darkness. After these artificial logs were fully colonised, formed light-induced mycelial brown film (Tang et al. Citation2013), and were under 4 °C low-temperature stimulation for 12 h, they were transferred into the temperature and humidity-controlled CGs. During fruiting, all the logs of L2 and L9 were placed into three CGs, with 200 logs per variety in each CG. To assess the influence of temperature on SLRD incidence rate, under the same relative humidity, the temperature in the three CGs was set at 21–23 °C, 24–26 °C, and 26–28 °C, respectively. After the conventional fruiting management, the SLRD incidence rate was calculated by the proportion of rotten logs to the total logs.
3. Results
3.1. Environmental parameters in shiitake factory
In the sampled shiitake factory, both temperature and relative humidity presented seasonal and regional variations (Table S1). The temperatures in sampling sites during the SLRD HI season were significantly lower than those in the SLRD LI season. The temperature in CGs ranged from 11.0 °C to 20.0 °C in the SLRD HI season, and from 17.5 °C to 23.0 °C in the SLRD LI season. The temperature in SG was well controlled at 23.0 °C to 25.0 °C. But for OS, the temperature was greatly affected by the external environment and presented great variation (ranging from 4.0 °C to 22.5 °C in the SLRD HI season, and from 23.0 °C to 33.0 °C in the SLRD LI season).
Overall, the relative humidities in sampling sites during the SLRD HI season were significantly higher than those in the SLRD LI season (Table S1). The relative humidity in CGs was significantly higher than that in SG and OS. For details, the relative humidity in CGs was 80%–100% in the SLRD HI season, and 48%–85% in the SLRD LI season. The relative humidity in SG varied from 29% to 73%. In OS, the relative humidity ranged from 74% to 90% in the SLRD HI season, while from 21% to 67% in the SLRD LI season.
3.2. Airborne fungi concentration
There were significant differences in airborne fungi concentrations among the four sampling sites (). The lowest concentration of culturable airborne fungi was observed in SG (approximately 250–450 CFU/m3), while the airborne fungal concentrations in CGs were up to 7,000–8,500 CFU/m3. Both in SLRD HI and LI seasons, airborne fungal concentrations in CGs were significantly higher than those in SG and OS (). The airborne fungal concentrations in CGA and CGB during the SLRD LI season were higher than those in the SLRD HI season (). However, in SG and OS, airborne fungal concentrations did not differ significantly between SLRD HI and LI seasons (). Trichoderma, Penicillium, Cladosporium, and Aspergillus were the dominant fungal genera in CGs both in the SLRD HI and LI seasons.
Figure 2. Differences of airborne fungal concentrations in the four sampling sites during shiitake artificial log rot disease (SLRD) high and low incidence seasons. (a) and (b) Represented the differences in airborne fungal concentrations of the four sampling sites [cultivation greenhouse A (CGA), cultivation greenhouse B (CGB), outdoor space (OS), and spawn-running greenhouse (SG)] in SLRD high and low incidence seasons, respectively. (c–f) Represented the difference in airborne fungal concentrations between high and low incidence seasons in CGA, CGB, OS, and SG, respectively.
![Figure 2. Differences of airborne fungal concentrations in the four sampling sites during shiitake artificial log rot disease (SLRD) high and low incidence seasons. (a) and (b) Represented the differences in airborne fungal concentrations of the four sampling sites [cultivation greenhouse A (CGA), cultivation greenhouse B (CGB), outdoor space (OS), and spawn-running greenhouse (SG)] in SLRD high and low incidence seasons, respectively. (c–f) Represented the difference in airborne fungal concentrations between high and low incidence seasons in CGA, CGB, OS, and SG, respectively.](/cms/asset/e90b5b72-03fb-4990-8b58-16ea4b0baf5c/tmyc_a_2323714_f0002_oc.jpg)
3.3. Abundance and composition of airborne fungi
During the entire sampling period, a total of 64 air samples (16, 15, 21, and 12 from CGA, CGB, OS, and SG, respectively) were collected and sequenced (Table S1). After quality trimming, over four million high-quality ITS sequences were obtained. These obtained sequences were finally annotated to 17,899 OTUs at a 97% similarity threshold. All sequences have been deposited in the China National GeneBank Database with the accession number CNP0004496.
The amounts of the shared and unique fungal OTUs of the samples were visualised by the Venn diagram (Figure S2). More OTUs were identified in the SLRD HI season than in the SLRD LI season. At the genus level, there were 466, 434, and 604 OTUs in CGA, CGB, and OS during the SLRD HI season, and 372, 351, and 400 OTUs in these three sites during the SLRD LI season, respectively. The airborne microbiome in SG was investigated only in the SLRD HI season, and 637 OTUs were identified. During the SLRD HI season, 249 fungal OTUs were commonly shared in the four sites, and 48 OTUs, 37 OTUs, 89 OTUs, and 103 OTUs were unique in CGA, CGB, OS, and SG, respectively (Figure S2A). As shown in Figure S2B, in CGA, CGB, and OS, the number of fungal OTUs shared between SLRD HI and LI season was 223, 234, and 267, respectively, accounting for 36.3%, 42.2%, and 37.2% of the total OTUs in each comparison. The high proportion of shared OTUs indicated the existence of stable core airborne mycobiota between SLRD HI and LI seasons.
Based on OTUs annotation, the airborne fungi from the 64 samples were annotated to 33 phyla and 1,010 genera. At the phylum level, Ascomycota, Basidiomycota, and Chytridiomycota were the three dominant phyla within the samples (Figure S3). To profile the genus-level composition, we collected taxa that had a relative abundance of more than 1% in at least two samples (). During the SLRD HI season, the most abundant genera in samples were Trichoderma (2.5%–24.3% in CGA, 7.5%–69.7% in CGB, 2.0%–66.3% in SG, and 2.0%–66.4% in OS), Penicillium (14.0%–36.0% in CGA, 8.1%–21.1% in CGB, 1.8%–34.7% in SG, and 1.8%–34.7% in OS), Cladosporium (1.4%–14.6% in CGA, 0.2%–9.3% in CGB, 0.6%–6.3% in SG, and 0.6%–6.4% in OS), and Aspergillus (1.1%–6.7% in CGA, 0.2%–12.4% in CGB, 0.6%–36.5% in SG, and 0.6%–36.5% in OS). During the SLRD LI season, these four pathogens remained the dominant fungal genera (). However, the relative abundances of Trichoderma decreased significantly in the sampling sites (0.1%–2.0% in CGA, 0%–2.1% in CGB, 0%–2.1% in OS). Moreover, the abundances of Phlyctochytrium were noticeably increased in SLRD LI season samples (). These results were consistent with those of the culture-dependent method, indicating that the four main mushroom pathogens (Trichoderma, Penicillium, Cladosporium, and Aspergillus) were the stable core fungi in all the sampling sites of the shiitake factory.
Figure 3. Relative abundances of airborne fungi in different sampling sites at genus level. Cultivation greenhouse a (CGA) (a), cultivation greenhouse B (CGB) (b), spawn-running greenhouse (SG) (c), and outdoor space (OS) (d) respectively. Different colors represent the different fungal genera and the height of the column represents the relative fungal abundance.
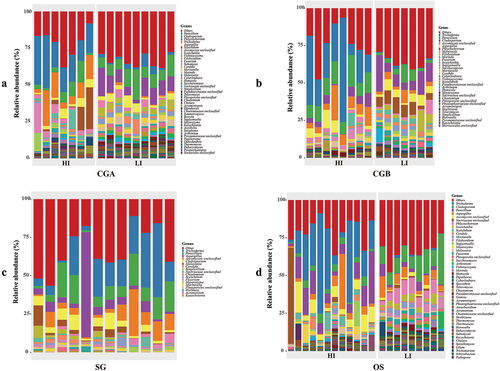
3.4. Diversity of fungal communities in shiitake factory
The Shannon and Chao1 indices were used to characterise the diversity and richness of airborne fungi in different sampling sites of the shiitake factory. Overall, Shannon indices of SLRD LI season samples were higher than those in the HI season (, Table S2). For the samples from CGB and OS, Shannon indices differed significantly between SLRD LI and HI seasons. Oppositely, Chao1 indices of SLRD LI season samples were lower than those in HI season (, Table S2). Taken together, the fungal communities in CGA, CGB, and OS samples from the SLRD HI season showed relatively high richness but lower diversity than those in the LI season. According to the results of relative abundance, this phenomenon may be due to the proliferation of dominant fungi during the SLRD HI season. Among different sampling sites in the SLRD HI season, no significant difference was found in the richness and diversity of taxa (p > 0.05). Further, there was no significant difference in Shannon and Chao1 indices between samples from different fruiting stages (Table S2).
Figure 4. The Shannon and Chao1 indices of cultivation greenhouse a and b (CGA, CGB) and outdoor space (OS) in high and low incidence seasons. Difference of Shannon index between high and low incidence seasons in CGA (a), CGB (b), and OS (c) respectively; difference of Chao1 index between high and low incidence seasons in CGA (d), CGB (e), and OS (f), respectively.
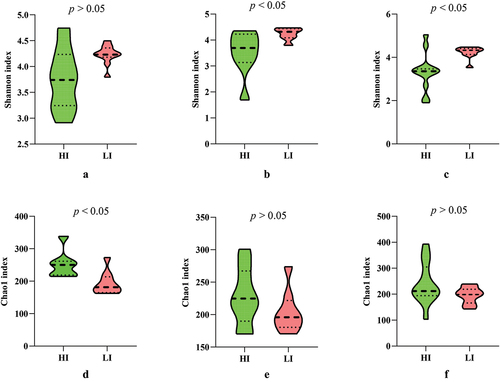
PCoA and ANOSIM based on OTUs further assessed the overall differences in airborne fungal communities in distinct sampling sites across seasons. The airborne fungal communities across SLRD HI and LI seasons were clearly distinguished (p < 0.05) (, Figure S4A). Seasonal changes and SLRD occurrence might be the main factors causing the variations in airborne fungal communities. Within the SLRD HI or LI season, there was no significant difference in fungal communities in different sampling sites (p > 0.05). The airborne fungal communities also showed no significant difference among fruiting periods (, Figure S4B).
Table 1. Similarity analysis of samples between different groups.
3.5. Analysis of fungal differences between SLRD HI and LI seasons
To explore the effect of SLRD occurrence on airborne fungal communities, the data from CGA and CGB were further combined to identify the differential fungi between SLRD HI, and LI seasons. Biomarkers of CGs varied according to SLRD incidence. Four fungal genera, including Phlyctochytrium, Pseudaleuria, Lichtheimia, and Issatchenkia (LDA values > 4) contributed significantly to the difference between groups and were the biomarkers of CGs in the SLRD LI season. In contrast, Trichoderma spp. was significantly enriched during the SLRD HI season (Figure S5), which was consistent with the fact that SLRD was mainly caused by the infection of Trichoderma spp.
Further, we characterised the variations in the abundance of Trichoderma, temperature, and humidity in the two CGs across seasons (). Relatively, the SLRD HI season was characterised by low temperature (16.6 °C), high humidity (93.3%), and high abundance of Trichoderma (20.3%), while the SLRD LI season was characterised by high temperature (21.1 °C), low humidity (74.6%), and low abundance of Trichoderma (0.7%) (Table S3). The high incidence of SLRD was caused by the increased abundance of Trichoderma spp., which may be associated with the decrease in temperature and increase in relative humidity in CGs.
3.6. Influence of temperature on SLRD
The influence of temperature on SLRD in CGs was assessed by a fruiting trial using two shiitake varieties. For the two varieties, SLRD incidence was significantly lower at 24–26 °C (5.2% for L2 and 26.5% for L9) and 26–28 °C (6.5% for L2 and 30.0% for L9) than that at 21–23 °C (15.0% for L2 and 39.5% for L9). Results indicated that SLRD incidence could be significantly reduced by appropriately increasing the temperature of cultivation greenhouses (). Regarding the performance of different varieties, under the same temperature condition, the SLRD incidence of L2 was significantly lower than that of L9. The resistance of shiitake varieties also had a significant influence on the occurrence of fungal diseases ().
Figure 6. Shiitake artificial log rot disease (SLRD) incidence of two Lentinula edodes varieties under different temperatures. Difference of incidence rate of SLRD under different interval period between two fruiting flushes (IBF) temperature (a); difference of incidence rate of different L. edodes varieties at the same IBF temperature (b).
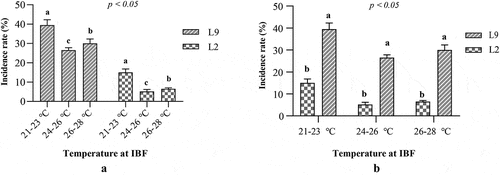
4. Discussion
4.1. Air quality assessment of shiitake factory
Occupational exposure to fungal propagules could cause various respiratory and infectious diseases and have major public health impacts (Douwes et al. Citation2003; Tarigan et al. Citation2017). Fungal diseases are common in mushroom factories, which are rich in organic materials and with a highly intensive production mode. The workplace-related hypersensitivity pneumonitis was also well documented in the mushroom industry (Jackson and Welch Citation1970; Yoshikawa et al. Citation2007; Tarigan et al. Citation2017). Therefore, the assessment of fungal concentrations in shiitake factories is not only related to the safe production of shiitake mushrooms but also related to the health of workers.
Here in this study, using the culture-dependent method, we assessed culturable fungi concentrations in a shiitake factory during SLRD high/low occurrence seasons. Due to the installation of an air filtration system and well-controlled temperature and humidity, the lowest concentration of culturable airborne fungi was observed in the spawn-running greenhouse (approximately 250–450 CFU/m3), which was comparable to that of family homes (Lou et al. Citation2023). In the cultivation greenhouses, the airborne fungi concentrations presented seasonal variation related to the occurrence of SLRD, and up to 7,000–8,500 CFU/m3. This airborne fungal concentration was comparable to that in cultivation rooms of oyster mushrooms (Chun et al. Citation2012). The significantly higher concentrations of culturable airborne fungi in CGs than those in SG and OS may be because unwrapping the shiitake logs during the fruiting stage provided favourable environments for the growth of diverse fungi (Penicillium, Trichoderma, and Cladobotryum, etc.) (Wang et al. Citation2016; Wu et al. Citation2019). Meanwhile, spraying and injecting water into shiitake logs caused the fungal spores to spread around.
Several national and international institutions have suggested standards for acceptable fungi concentration in indoor environments. The World Health Organization (WHO) has recommended a fungal bioaerosol concentration threshold (500 CFU/m3) as a residential guideline (WHO Citation1988). The American Industrial Hygiene Association (AIHA) has limited the threshold for fungal spore concentration in indoor environments of residential buildings below 500 CFU/m3 (Yuan et al. Citation2022). In China, the threshold value of 1,500 CFU/m3 for bacterial bioaerosol concentrations in residential and office buildings in the Indoor Air Quality Standard was issued in 2022 (https://openstd.samr.gov.cn/bzgk/gb/newGbInfohcno=6188E23AE55E8F557043401FC2EDC436). Taking the recommended 500 CFU/m3 (total fungi) as a baseline, the airborne fungal concentrations in CGs were far beyond the air quality standard, revealing the serious fungal contamination in SLRD HI and LI seasons. In addition, the results of relative abundance showed that some allergenic fungi (such as Aspergillus and Penicillium) were dominant species within the fungal community either in SLRD HI or in LI season. Taken together, the air quality in shiitake factories, especially in cultivation greenhouses is unsatisfactory. The workers and stakeholders in the mushroom industry should be fully aware of the health risks associated with occupational exposure to fungal contaminants. After the end of harvesting, it was necessary to perform in-depth environmental cleaning and disinfection of cultivation greenhouses.
4.2. Seasonal discrepancy of airborne fungal communities related to SLRD
The nonculturable microbes account for a large part of the microbiome, and cannot be detected by culture-based method (Nakamura et al. Citation2016). Therefore, high-throughput sequencing was further employed to comprehensively characterise the dynamics of airborne fungal communities related to SLRD.
Regarding different sampling sites of the shiitake factory, the airborne fungal communities showed convergence. PCoA analysis indicated that there was no significant difference in fungal communities in different sampling sites within the same season. The major fungal contaminants (including Trichoderma, Penicillium, Cladosporium, and Aspergillus) were the stable core fungi, which was consistent with the previous studies in F. filiformis and P. eryngii factories (Cai et al. Citation2020; Qin et al. Citation2020). Under external detachment force (such as high-speed airflow), fungal spores could be aerosolized and spread out as aerosols, thereby affecting the surrounding fungal community (Li et al. Citation2022). In this study, the diffusion of dominant pathogens and their spores may be the main reason for the convergence of fungal communities in different function areas of the shiitake factory.
In terms of seasonal discrepancy associated with SLRD, the airborne fungal communities differed significantly between SLRD high- and low-incidence seasons in the shiitake factory. The samples from the SLRD HI season showed a higher relative abundance of airborne fungi than the SLRD LI season samples. Alpha diversity also indicated that fungal communities were richer but uneven in the SLRD HI season than in the LI season. Seasonal discrepancy of airborne fungal communities was found to be related to SLRD. This discrepancy may be due to the proliferation of Trichoderma during the SLRD HI season, in which the relative abundance of Trichoderma in CGs and OS was up to 69.7% and 66.4%, respectively, remarkably higher than those in the SLRD LI season. The enrichment of Trichoderma in the SLRD HI season was also confirmed by LEfSe analysis.
Environmental factors (such as temperature and humidity) had a large influence on the diversity and composition of mycobiota (Souza et al. Citation2020). Trichoderma species are widely spread in soils and have been reported to grow in a wide range of soil temperatures varying from 0 °C to 40 °C (Dutta et al. Citation2022). Earlier studies reported that the competitive colonisation of T. harzianum was highest at temperatures below the optimal growth temperature and its saprophytic ability was great at 15–21 °C (Eastburn and Butler Citation1991). Moreover, the population of Trichoderma increased with an increase in rainfall and relative humidity (Mohan and Gopal Citation2021). Indeed, SLRD HI season was characterised by low temperature (16.6 °C) and high humidity (93.3%), while SLRD LI season was characterised by relatively high temperature (21.1 °C) and low humidity (74.6%). Collectively, the favourable temperature and humidity caused the proliferation of Trichoderma, and then affected the airborne fungal communities, which presented an SLRD-seasonal variation.
4.3. Causes of artificial log rot disease in Qihe
Uncovering the main causes of fungal diseases and preventing their spread are the key issues for the safe production of mushrooms. As one of the most serious diseases in shiitake, SLRD severely affects the yield of shiitake mushrooms (Ma et al. Citation2021). In this study, Koch’s postulates confirmed that T. harzianum was the pathogen causing SLRD in Qihe (data not shown), which was consistent with our previous results (Cao et al. Citation2015). Previous studies have shown that Trichoderma spp. infect mushroom mycelia by competing for nutrition and growth space, coiling or mycoparasitism, and secreting harmful metabolites (Wang et al. Citation2016; Ma et al. Citation2019). Under the high-temperature stress (nearly 40 °C for dozens of hours), shiitake mycelia were damaged and lost the resistance to Trichodema spp. (Cao et al. Citation2015). The difference in the surveyed shiitake factory was that SLRD occurred more frequently at a relatively low temperature (less than 20 °C). The optimal temperature for shiitake mycelia growth was at 24–27 °C (Xiang et al. Citation2021). We speculated that the shiitake mycelia were weak at temperatures below 20 °C (low temperature seasons) and were susceptible to aggressive T. harzianum strains, leading to the proliferation of Trichoderma, and eventually resulting in the outbreak of SLRD. This hypothesis was also supported by the results of the fruiting trial, in which SLRD incidence could be significantly reduced by reasonably increasing the ambient temperature.
5. Conclusions
For the first time, we comprehensively characterised the airborne fungal communities in one shiitake factory across the occurrence of artificial log rot disease. The airborne fungal concentrations observed in shiitake fruiting greenhouses were up to 7,000–8,500 CFU/m3. The major fungal contaminants, including Trichoderma, Penicillium, Cladosporium, and Aspergillus were the stable core mycobiota in the shiitake factory. The concentrations, richness, diversity, and community structure of airborne fungi in the shiitake factory differed significantly across SLRD high- and low-incidence seasons, whereas the fungal community in distinct function areas showed convergence. SLRD high incidence was labelled with a high abundance of Trichoderma. Temperature and relative humidity in cultivation greenhouses were important factors influencing the occurrence of SLRD. SLRD incidence could be significantly reduced by reasonably increasing the ambient temperature during the fruiting process. Findings here provide valuable information on the dynamics of airborne fungal communities across the occurrence of fungal diseases in shiitake. Fungal exposures and their impacts on mushroom production and workers’ health deserve more attention in the mushroom industry.
Author contributions
Wenbing Gong: Formal analysis, Writing-original draft, Writing-review and editing, Investigation. Kaixiao Qiu: Investigation, Formal analysis, Writing-original draft. Zhen Zhuang: Data curation. Lujun Zhang: Conceptualization. Xueqing Liu: Investigation. Xiao Yu: Resources. Yinbing Bian: Funding acquisition, Supervision. Yang Xiao: Conceptualization, Supervision, Funding acquisition, Writing-review and editing.
Supplemental Material
Download MS Word (3.9 MB)Disclosure statement
No potential conflict of interest was reported by the author(s).
Supplemental data
Supplemental data for this article can be accessed online at https://doi.org/10.1080/21501203.2024.2323714.
Additional information
Funding
References
- Ban GH, Kim JH, Kim SA, Rhee MS, Choi SY, Hwang IJ, Kim SR. 2023. Microbial succession during button mushroom (Agaricus bisporus) production evaluated via high-throughput sequencing. Food Microbiol. 114:104307. doi: 10.1016/j.fm.2023.104307.
- Cai J, Chang K, Bian YB, Xiao Y. 2020. Dynamic monitoring of airborne microorganisms in clean area of Flammulina filiformis factory. Acta Edulis Fungi. 27:52–60.
- Cao XT, Bian YB, Xiao XJ, Li JS, Wang GZ. 2015. Effect of heat stress on Lentinula edodes mycelial growth recovery and resistance to Trichoderma harzianum. Acta Edulis Fungi. 22:81–85.
- Chen L, Qian L, Zhang X, Li J, Zhang Z, Chen X. 2022. Research progress on indoor environment of mushroom factory. Int J Agric Biol Eng. 15(1):25–32. doi: 10.25165/j.ijabe.20221501.6872.
- Chun SC, Ahn Y, Khan SM, Chung IM, Won HY, Jhune CS, Park Y. 2012. The microbial population in the air of cultivation facility of oyster mushrooms. J Microbiol. 50(6):1053–1057. doi: 10.1007/s12275-012-2195-1.
- Ding N, Xing F, Liu X, Selvaraj JN, Wang L, Zhao Y, Wang Y, Guo W, Dai X, Liu Y. 2015. Variation in fungal microbiome (mycobiome) and aflatoxin in stored in-shell peanuts at four different areas of China. Front Microbiol. 6:1055. doi: 10.3389/fmicb.2015.01055.
- Douwes J, Thorne PS, Pearce N, Heederik DJ. 2003. Bioaerosol health effects and exposure assessment: progress and prospects. Ann Occup Hyg. 47(3):187–200. doi: 10.1093/annhyg/meg032.
- Dutta P, Deb L, Pandey AK. 2022. Trichoderma- from lab bench to field application: looking back over 50 years. Front Agron. 4:932839. doi: 10.3389/fagro.2022.932839.
- Eastburn DM, Butler EE. 1991. Effects of soil moisture and temperature on the saprophytic ability of Trichoderma harzianum. Mycologia. 83(3):257–263. doi: 10.1080/00275514.1991.12026009.
- Edgar RC. 2013. UPARSE: Highly accurate OTU sequences from microbial amplicon reads. Nat Methods. 10(10):996–998. doi: 10.1038/nmeth.2604.
- Edgar RC, Haas BJ, Clemente JC, Quince C, Knight R. 2011. UCHIME improves sensitivity and speed of chimera detection. Bioinformatics. 27(16):2194–2200. doi: 10.1093/bioinformatics/btr381.
- Ficociello B, Masciarelli E, Casorri L, Cichelli A, Pacioni G. 2019. The onset of occupational diseases in mushroom cultivation and handling operators: A review. Italian J Mycol. 48:26–38. doi:10.6092/issn.2531-7342/9409.
- Gao M, Yu A, Chen M, Qiu T, Guo Y, Sun X, Wang X. 2022. Airborne fungi and human exposure in different areas of composting facilities. Ecotoxicol Environ Saf. 243:113991. doi: 10.1016/j.ecoenv.2022.113991.
- Gong WB, Xu R, Xiao Y, Zhou Y, Bian YB. 2014. Phenotypic evaluation and analysis of important agronomic traits in the hybrid and natural populations of Lentinula edodes. Sci Hortic (Amsterdam). 179:271–276. doi: 10.1016/j.scienta.2014.09.044.
- Grimm D, Wösten HAB. 2018. Mushroom cultivation in the circular economy. Appl Microbiol Biotechnol. 102(18):7795–7803. doi: 10.1007/s00253-018-9226-8.
- Gui Y 2022. Studies on breeding excellent hybrid strains of Lentinula edodes suitable for industrial cultivation [ master’s thesis]. Wuhan (Hubei): Huazhong Agricultural University.
- Jackson E, Welch KM. 1970. Mushroom worker’s lung. Thorax. 25(1):25–30. doi: 10.1136/thx.25.1.25.
- Li X, Liu D, Yao J. 2022. Aerosolization of fungal spores in indoor environments. Sci Total Environ. 820:153003. doi: 10.1016/j.scitotenv.2022.153003.
- Liu J, Abdelfattah A, Norelli J, Burchard E, Schena L, Droby S, Wisniewski M. 2018. Apple endophytic microbiota of different rootstock/scion combinations suggests a genotype-specific influence. Microbiome. 6(1):18. doi: 10.1186/s40168-018-0403-x.
- Lou X, Yang Q, Xie J, Fang Z. 2023. Concentration characteristics of culturable airborne microbes in family homes in Hangzhou, China. Aerobiologia. 39(1):119–131. doi: 10.1007/s10453-023-09779-6.
- Lu R, Li WX, Song Y. 2017. Characteristics of culturable bioaerosols in various weather in Xi’an city, China. Res Environ Sci. 30:1012–1019.
- Ma XL, Fan XL, Wang GZ, Xu RP, Yan LL, Zhou Y, Gong YH, Xiao Y, Bian YB. 2021. Enhanced expression of thaumatin-like protein gene (LeTLP1) endows resistance to Trichoderma atroviride in Lentinula edodes. Life. 11(8):863. doi: 10.3390/life11080863.
- Magoc T, Salzberg SL. 2011. FLASH: fast length adjustment of short reads to improve genome assemblies. Bioinformatics. 27(21):2957–2963. doi: 10.1093/bioinformatics/btr507.
- Małek S, Ważny R, Błońska E, Jasik M, Lasota J. 2021. Soil fungal diversity and biological activity as indicators of fertilization strategies in a forest ecosystem after spruce disintegration in the Karpaty Mountains. Sci Total Environ. 751:142335. doi: 10.1016/j.scitotenv.2020.142335.
- Ma XL, Wang GZ, Fan XL, Bian YB. 2019. Interaction between edible fungi and Trichoderma spp.: A review. Wei Sheng Wu Xue Tong Bao. 46:184–191. doi:10.13344/j.microbiol.china.180265.
- Mohan M, Gopal S. 2021. Impact of climatic variables on the population of Trichoderma sp. in the rhizosphere of black pepper. Environ Conserv J. 222(1&2):45–48. doi: 10.36953/ECJ.2021.221208.
- Nakamura K, Iizuka R, Nishi S, Yoshida T, Hatada Y, Takaki Y, Iguchi A, Yoon DH, Sekiguchi T, Shoji S, et al. 2016. Culture-independent method for identification of microbial enzyme-encoding genes by activity-based single-cell sequencing using a water-in-oil microdroplet platform. Sci Rep. 6(1):22259. doi:10.1038/srep22259.
- Nilsson RH, Anslan S, Bahram M, Wurzbacher C, Baldrian P, Tedersoo L. 2018. Mycobiome diversity: High-throughput sequencing and identification of fungi. Nat Rev Microbiol. 17(2):95–109. doi: 10.1038/s41579-018-0116-y.
- Qin WD, Rong CB, Song S, Wang SX, Liao YL, Liu Y. 2020. Study on airborne microbial community in a Pleurotus eryngii commercial cultivation facility. Biotechnology. 30:579–583.
- Schloss PD, Westcott SL, Ryabin T, Hall JR, Hartmann M, Hollister EB, Lesniewski RA, Oakley BB, Parks DH, Robinson CJ, et al. 2009. Introducing mothur: open-source, platform-independent, community-supported software for describing and comparing microbial communities. Appl Environ Microbiol. 75(23):7537–7541. doi:10.1128/AEM.01541-09.
- Segata N, Izard J, Waldron L, Gevers D, Miropolsky L, Garrett WS, Huttenhower C. 2011. Metagenomic biomarker discovery and explanation. Genome Biol. 12(6):1–18. doi: 10.1186/gb-2011-12-6-r60.
- Souza FF, Mathai PP, Pauliquevis T, Balsanelli E, Pedrosa FD, Souza EM, Baura VA, Monteiro RA, Cruz LM, Souza RA, et al. 2020. Influence of seasonality on the aerosol microbiome of the Amazon rainforest. Sci Total Environ. 760:144092. doi: 10.1016/j.scitotenv.2020.144092.
- Tang LH, Jian HH, Song CY, Bao DP, Shang XD, Wu DQ, Tan Q, Zhang XH. 2013. Transcriptome analysis of candidate genes and signaling pathways associated with light-induced brown film formation in Lentinula edodes. Appl Microbiol Biotechnol. 97(11):4977–4989. doi: 10.1007/s00253-013-4832-y.
- Tarigan YG, Chen RY, Lin H, Jung CY, Kallawicha K, Chang T, Hung P, Chen C, Chao HJ. 2017. Fungal bioaerosol exposure and its effects on the health of mushroom and vegetable farm workers in Taiwan. Aerosol Air Qual Res. 17(8):2064–2075. doi: 10.4209/aaqr.2016.09.0401.
- Wang GZ, Cao XT, Ma XL, Guo MP, Liu CH, Yan LL, Bian YB. 2016. Diversity and effect of Trichoderma spp. associated with green mold disease on Lentinula edodes in China. Microbiologyopen. 5(4):709–718. doi: 10.1002/mbo3.364.
- WHO. 1988. World Health Organization Regional Publications European Series, No. 31: indoor air quality: biological contaminants. report on a WHO meeting. Available from: WHO, Copenhagen, Denmark.
- Wu XY, Fu YP, Li Y. 2019. Biological characteristics of Cladobotryum dendroides, a causal pathogen of Lentinula edodes cobweb disease. Mycosystema. 38:646–657. doi:https://doi.org/10.13346/j.mycosystema.180310.
- Xiang Q, Adil B, Chen Q, Gu Y, Zeng X, Li X. 2021. Shiitake mushroom (Lentinula edodes (Berk.) sing.) breeding in China. In: Al-Khayri J, Jain S Johnson D, editors Advances in plant breeding strategies: vegetable crops. Cham, Switzerland: Springer; pp. 443–476.
- Xue F, Yang Y, Lai S, Xiao Y, Yao Y, Zhang Y, Zou S. 2023. Airborne microbiomes at a subtropical island in southern China: importance of the northwest and southeast monsoons. Atmos Environ. 307:119842. doi: 10.1016/j.atmosenv.2023.119842.
- Yoshikawa S, Tsushima K, Yasuo M, Fujimoto K, Kubo K, Kumagai T, Yamazaki Y. 2007. Hypersensitivity pneumonitis caused by Penicillium citrinum, not enoki spores. Am J Ind Med. 50(12):1010–1017. doi: 10.1002/ajim.20535.
- Yuan C, Wang X, Pecoraro L. 2022. Environmental factors shaping the diversity and spatial-temporal distribution of indoor and outdoor culturable airborne fungal communities in Tianjin University campus, Tianjin, China. Front Microbiol. 13:928921. doi: 10.3389/fmicb.2022.928921.
- Yu J, Hu Q, Xie Z, Kang H, Li M, Li Z, Ye P. 2013. Concentration and size distribution of fungi aerosol over oceans along a cruise path during the fourth chinese arctic research expedition. Atmosphere. 4(4):337–348. doi: 10.3390/atmos4040337.
- Zhang L, Gong WB, Li C, Shen N, Gui Y, Bian YB, Kwan HS, Cheung MK, Xiao Y. 2021. RNA-Seq-based high-resolution linkage map reveals the genetic architecture of fruiting body development in shiitake mushroom, Lentinula edodes. Comput Struct Biotechnol J. 19:1641–1653. doi: 10.1016/j.csbj.2021.03.016.
- Zhang C, Shi X, Zhang JQ, Zhang Y, Wang W. 2023. Dynamics of soil microbiome throughout the cultivation life cycle of morel (Morchella sextelata). Front Microbiol. 14:979835. doi: 10.3389/fmicb.2023.979835.