ABSTRACT
Streptococcus equi subsp. zooepidemicus (SEZ) is a major equine pathogen that causes pneumonia, abortion, and polyarthritis. It can also cause invasive infections in humans. SEZ expresses the M-like protein SzM, which recruits host proteins such as fibrinogen to the bacterial surface. Equine SEZ strain C2, which binds only comparably low amounts of human fibrinogen in comparison to human SEZ strain C33, was previously shown to proliferate in equine and human blood. As the expression of SzM_C2 was necessary for survival in blood, this study investigated the working hypothesis that SzM_C2 inhibits complement activation through a mechanism other than fibrinogen and non-immune immunoglobulin binding. Loss-of-function experiments showed that SEZ C2, but not C33, binds C1q via SzM in IgG-free human plasma. Furthermore, SzM C2 expression is necessary for recruiting purified human or equine C1q to the bacterial surface. Flow cytometry analysis demonstrated that SzM expression in SEZ C2 is crucial for the significant reduction of C3b labelling in human plasma. Addition of human plasma to immobilized rSzM_C2 and immobilized aggregated IgG led to binding of C1q, but only the latter activated the complement system, as shown by the detection of C4 deposition. Complement activation induced by aggregated IgG was significantly reduced if human plasma was pre-incubated with rSzM_C2. Furthermore, rSzM_C2, but not rSzM_C33, inhibited the activation of the classical complement pathway in human plasma, as determined in an erythrocyte lysis experiment. In conclusion, the immunoglobulin-independent binding of C1q to SzM_C2 is associated with complement inhibition.
Introduction
S. equi subsp. zooepidemicus (SEZ) causes diseases in horses, pigs, ruminants, dogs, and humans [Citation1,Citation2]. In horses, SEZ often colonizes mucosal surfaces of the upper respiratory tract and genital tract. However, it is also a major equine pathogen. Ascending or invasive infection may lead to pathologies such as pneumonia, arthritis, endometritis and abortion [Citation3–5]. Outbreaks of severe disease occurred in the Icelandic horse population [Citation6] but also in pigs in China and North America [Citation7–9]. Horses are an important source of zoonotic infections [Citation10]. In humans, severe diseases such as septicaemia, endocarditis and meningitis have been observed [Citation11–13].
SEZ expresses at least one dimeric coiled-coil M-like protein, designated SzM and SzP, on the bacterial surface, similar to the human pathogen S. pyogenes [Citation14]. SzM showed high heterogeneity, but human SEZ isolates grouped into distinct szm clusters, indicating the high zoonotic potential of strains of these clusters [Citation15]. Distinct variants of SzM have been shown to bind equine fibrinogen (Fg) [Citation15–17]. Recently, we demonstrated that two SzM proteins (SzM_C2 and SzM_C33) bind to the Fc region of human IgG [Citation15].
M-protein-mediated Fg binding is important for complement evasion in group A streptococci (GAS) [Citation18]. Sandin et. al. [Citation19] showed through functional analysis of mutants expressing variants of the M5 protein still binding FHL-1 (factor H) and human serum albumin but deficient in Fg binding, that the B-repeat region is crucial for phagocytosis resistance due to its role in Fg binding. However, many M proteins do not bind Fg but interfere with complement deposition and phagocytosis by recruiting human C4b-binding protein (C4BP), an inhibitor of the classical complement pathway [Citation20,Citation21]. An older study [Citation22] showed that C1q directly binds to two proteins within the M family in GAS M5 and M76. However, the functional consequences of this interaction have not yet been investigated further and a motif for C1q binding has not been described.
The prominent binding of human Fg is a characteristic phenotype of zoonotic SEZ isolates. Accordingly, zoonotic strain C33 recruits high amounts of human Fg to the bacterial surface. However, the equine strain C2 binds significantly lower amounts of human Fg but still proliferates in human blood [Citation15]. As expression of SzM is crucial for the survival of SEZ in equine and human blood [Citation15], the SzM-mediated interaction between SEZ and host proteins was investigated in this study. The finding that SEZ C2 binds C1q, the main activator of the classical complement pathway, independent of IgG via SzM led us to investigate the working hypothesis that C1q binding to SzM_C2 leads to inhibition of the complement system.
Results
SEZ strain C2 directly binds human complement component C1q via SzM
To identify further interactions of SzM with host proteins, SEZ strains C2, C2ΔSzM, C33, and C33ΔSzM were incubated in human plasma and IgG-free human plasma (IgG was depleted by passing plasma through a protein G column). Host proteins recruited to the bacterial surface were eluted and subjected to SDS-PAGE and Western blot analyses (). In SDS-PAGE analysis under reducing conditions, SEZ wt strain C2, but not its isogenic szm mutant or the wt strain C33, showed numerous bands between 25 kDa and 35 kDa, as well as a band clearly below 25 kDa after incubation in human plasma. Although less prominent, these bands were also recorded in the eluate of SEZ C2 after incubation with IgG-free human plasma. We hypothesized that the 26 kDa, 24 kDa and 20 kDa fragments of C1q were among these bands and conducted western blotting [Citation23]. This analysis showed that the SEZ strain C2, but not the szm deletion mutant C2ΔSzM or wt SEZ C33, bound human C1q (). This binding was also observed in the absence of IgG. Binding of C1q to SEZ strain C33 was not detectable. Notably, SzM-dependent binding of human IgG to the bacterial surface was recorded under these conditions for both SEZ wt strains ().
Figure 1. SDS-PAGE and Western blot analysis of host plasma proteins recruited to the bacterial surface. (a) Bacteria were incubated with normal human plasma (1) or IgG-free human plasma (2). Host proteins bound to the bacterial surface were eluted and subjected to SDS-PAGE and Western blot analysis. SEZ strain C2 but not the mutant C2ΔSzM bound C1q.
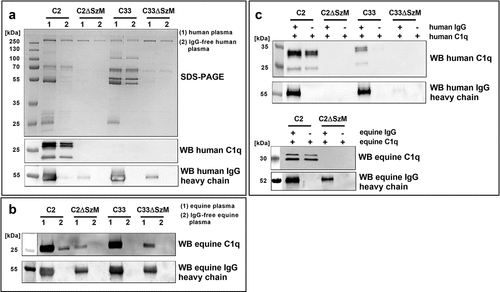
In addition, plasma absorption assays with normal and IgG-free equine plasma were performed, and C1q and IgG binding was analysed by western blotting (). The wild-type strain C2, but not the mutant C2ΔSzM, bound equine C1q in the absence of IgG. As expected, horses carried specific IgG antibodies against SEZ, indicated by prominent IgG signals in the case of the szm deletion mutants C2ΔSzM and C33ΔSzM. Accordingly, antigen-bound IgG recruited C1q to the surface of the szm deletion mutants.
To show direct C1q binding and exclude the contribution of IgM to C1q binding, SEZ strains C2, C2ΔSzM, C33, and C33ΔSzM were incubated with purified human IgG and human C1q or C1q alone (). The same experimental setup was used to analyse the binding of equine C1q (). Host proteins bound to the bacterial surface were eluted and subjected to western blotting analysis. SEZ C2 bound human and equine C1q directly, and no IgG was necessary. Direct C1q binding to SEZ C2 was mediated by the M-like protein SzM_C2, as the szm deletion mutant C2ΔSzM did not show this phenotype. SEZ strain C33 did not bind human or equine C1q in the absence of immunoglobulins. In conclusion, immunoglobulin-independent binding of human and equine C1q to SEZ C2 is a distinct phenotype of this strain mediated by its SzM protein.
SzM expression reduces C3b deposition on the bacterial surface of SEZ in human plasma
As the expression of SzM in SEZ C2 and C33 is necessary for survival in equine and human blood [Citation15] and SzM_C2, but not SzM_C33, binds C1q (), we asked whether these phenotypes are associated with reduced complement C3b deposition on the bacterial surface. Specifically, flow cytometric analysis of C3b deposition in wild-type and mutant strains after incubation in human and equine plasma was conducted. The apathogenic bacterium Lactococcus lactis was included for comparison. EDTA-treated plasma, in which the complement system is inactivated, served as a negative control. In human plasma, wild-type SEZ strain C2 showed significantly lower C3b deposition than C33, and C3b deposition was significantly increased in Lactococcus lactis compared to both SEZ strains (). In contrast, no significant differences in C3b deposition were recorded for the various investigated strains after incubation in equine plasma under the chosen experimental conditions (). Importantly, C3b deposition was significantly enhanced in both isogenic szm mutants in comparison to the wild-type SEZ strains C2 and C33 in human plasma (). This result indicates that SzM expression in SEZ strains C2 and C33 is involved in complement evasion in the human blood. As SEZ strain C2 binds comparably low amounts of hFg [Citation15] and human blood generally does not contain SEZ-specific IgG antibodies, the results suggest that C1q binding of SzM_C2 is involved in the reduction of C3b deposition.
Figure 2. Expression of SzM in SEZ C2 and C33 is crucial for significant reduction of C3b labelling in human but not in equine plasma. Flow cytometry analysis of C3b deposition on bacteria incubated with human (a and b) or equine plasma (c) at 37°C for 30 min. After incubation in plasma, the bacteria were stained with a FITC-labelled goat F(ab)2 anti-human C3 antibody, which also detects equine C3. The deposition of C3 at the bacterial surface is shown as the geomean of the fluorescence intensity of all gated bacteria. EDTA-treated plasma, in which the complement system is inactivated, served as a negative control. The apathogenic bacterium Lactococcus lactis was used as a positive control for complement activation (n = 3).
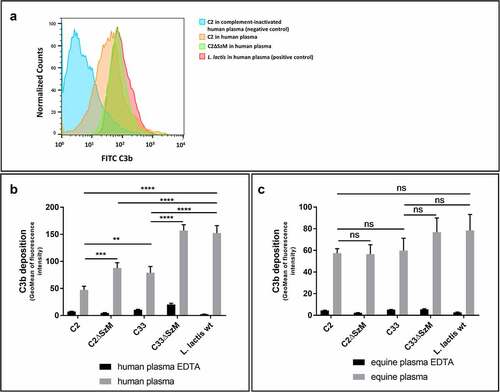
Recombinant SzM_C2 but not rSzM_C33 binds human C1q and inhibits activation of the human classical complement pathway
To analyse C1q binding by SzM and its subsequent interaction with the complement cascade, recombinant M proteins were coated on ELISA plates and incubated with human plasma. Immobilized rSzM_C2 showed dose-dependent binding of C1q, but complement activation was not observed, as indicated by C4 analysis (). Immobilized heat-aggregated human IgG, used as a positive control, recruited C1q and activated the complement system, as shown by C4 deposition (). The immobilized rSzM_C33 did not bind to C1q and did not induce C4 deposition (). To further investigate the inhibition of complement activation by rSzM_C2, human plasma was pre-incubated with rSzM and subsequently incubated in the wells of an ELISA plate coated with heat-aggregated human IgG. Activation of the complement system was again detected by the deposition of C4. rSzM_C2, but not rSzM_C33, led to dose-dependent inhibition of complement activation, as recorded by C4 deposition induced by immobilized aggregated IgG (). In conclusion, rSzM_C2, but not rSzM_C33, binds to C1q and inhibits complement activation induced by aggregated IgG. This suggests that rSzM_C2 interferes with the activation of the classical complement pathway by binding to C1q to prevent its activation.
Figure 3. (a) rSzm binds C1q but does not activate the complement system. ELISA plates were coated with rSzM and heat aggregated human IgG (positive control) and incubated with the indicated amounts of human plasma. Bound C1q and C4 were detected with a goat anti-human complement C1q and a goat anti-human complement C4 antibody, respectively. Heat-aggregated human IgG was recognised by C1q and led to an activation of the complement system, shown as C4 deposition. rSzM_C2 bound C1q but did not activate the complement system as no C4 deposition was detected. (b) rSzM_C2 leads to an inhibition of complement activation. One percent human plasma was pre-incubated with the indicated amounts of rSzM at 37°C for 1 h. Then, pre-incubated plasma was incubated in the wells of an ELISA plate, coated with heat-aggregated human IgG. Activation of the complement system was detected by the deposition of C4. rSzM_C2 showed a dose-dependent inhibition of complement activation as shown by detection of C4 deposition, whereas rSzM_C33 did not influence C4 deposition. (c) rSzM_C2 but not rSzM_C33 inhibits activation of the classical complement pathway in porcine serum. Porcine anti-sheep erythrocyte serum (of three different pigs) was pre-incubated with recombinant SzM proteins at 37 °C for 30 min. Pre-incubated serum was mixed with washed sheep erythrocytes and incubated for 2 h at 37 °C. Haemolysis of erythrocytes was determined by measuring the absorbance at 405 nm of the supernatant. (d) rSzM_C2 but not rSzM_C33 inhibits activation of the classical complement pathway in human plasma. Sheep erythrocytes were antibody labelled with EDTA-treated porcine anti-sheep erythrocyte serum. afterwards, antibody-labelled erythrocytes were washed and incubated with human plasma (source of active complement system), which was pre-incubated with the indicated rSzM variants. (n = 3). (e) rSzM_C2 but not rSzM_C33 inhibits IgG-mediated activation of the complement system in human plasma. Sheep erythrocytes were antibody labelled with purified porcine anti-sheep erythrocyte IgG. Afterwards, IgG-labelled erythrocytes were washed and incubated with human plasma (source of active complement system), which was pre-incubated with the indicated rSzM variants. (n = 3).
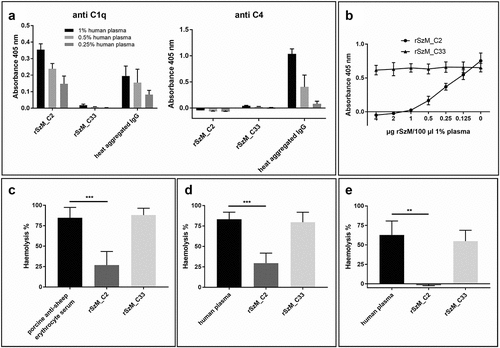
The classical complement activation pathway was further studied in haemolysis assays using sera containing antibodies directed against erythrocytes. Specifically, we comparatively investigated the impact of rSzM_C2 and rSzM_C33 on complement activation in a haemolysis assay using sera drawn from piglets vaccinated with sheep erythrocytes [Citation24]. Pre-incubation of porcine anti-sheep erythrocyte serum with rSzM_C2 resulted in a significant reduction in erythrocyte haemolysis, indicating that rSzM_C2 interferes with activation of the classical complement pathway (). In contrast, rSzM_C33 did not inhibit immunoglobulin-mediated haemolysis. shows a modified haemolysis assay designed to analyse the interaction of SzM with the human complement system. Briefly, sheep erythrocytes were incubated with EDTA-inactivated porcine anti-sheep erythrocyte serum, and erythrocytes were antibody labelled. After washing the antibody labelled erythrocytes, human heparinized plasma (as a source of the active complement system) pre-incubated with rSzM_C2 or rSzM_C33 proteins was added. Pre-incubation of human plasma with rSzM_C2, but not rSzM_C33, significantly inhibited the human complement-mediated lysis of erythrocytes (). Additionally, inhibition of IgG-mediated activation of the complement system in human plasma by rSzM was analysed (). Sheep erythrocytes were labelled with purified porcine anti-sheep erythrocyte IgG. After washing the IgG-labelled erythrocytes, human plasma pre-incubated with rSzM protein was added. rSzM_C2 completely inhibited IgG-mediated activation of the complement system, whereas rSzM_C33 did not significantly inhibit lysis. In conclusion, rSzM_C2 inhibits the activation of the classical complement pathway in porcine serum and human heparin plasma.
Discussion
SEZ is an important pathogen in horses and a neglected zoonotic agent that causes invasive infections. Survival and proliferation in the blood are important steps in the pathogenesis of various pathologies caused by SEZ. Our previous study demonstrated that the expression of the M-like protein SzM in SEZ strains C2 and C33 is crucial for survival in equine and human blood [Citation15]. The data presented in the former and current study indicate that the two strains use different strategies to prevent complement activation on the bacterial surface in human plasma. The SEZ strain C33 recruits large amounts of human fibrinogen to the bacterial surface [Citation15]. It is likely that SzM_C33 mediated binding of human fibrinogen to the bacterial surface leads to reduced C3b deposition (). This is in accordance with previous studies showing that fibrinogen binding by M-like proteins or M-proteins is a complement evasion mechanism in GAS [Citation18,Citation25]. However, SEZ strain C2 binds much less human fibrinogen and still shows the lowest value for labelling with C3b in human plasma. As C3b deposition on SEZ_C2 was significantly reduced by SzM expression, we postulated that a further function of SzM_C2 is crucial for complement inhibition.
In GAS, motifs for immunoglobulin, fibrinogen or plasminogen binding have been described in different M-proteins [Citation26]. Amino acids 276 to 311 of the SzM_C2 protein show high homology to the IgG-binding S-region of the M1-protein of GAS [Citation27] and the M-protein FOG of group G streptococci (GGS) [Citation28] (Fig. S1). Accordingly, we previously showed that rSzM_C33 and rSzM_C2 bind to the Fc fragment of human IgG [Citation15]. This study confirmed the SzM-dependent binding of IgG to the bacterial surface of both SEZ strains via plasma absorption assays (). The hexamer formation of IgG on surfaces recruits C1q and constitutes an efficient way to activate the classical complement pathway [Citation29]. Staphylococcal protein A blocks IgG hexamerization on the bacterial surface by competitive binding to the Fc-Fc interaction interface on IgG monomers [Citation30]. Accordingly, one might speculate that non-immune binding of IgG to the surface of the SEZ caused by SzM expression also prevents IgG hexamerization and subsequent complement activation. This could explain the enhanced C3b deposition on the bacterial surfaces of both szm mutants observed after incubation in human plasma (C2ΔSzM and C33ΔSzM in ). However, it has been shown that the orientation of the IgG antibody on the surface of GAS depends on the IgG concentration [Citation31]. At high IgG concentrations, as present in plasma, IgG is mostly bound to the bacterial surface via Fab and not through Fc binding of M and M-like proteins.
Strikingly, SzM-dependent differences in C3b deposition were not observed after incubation in equine plasma. The difference between human and equine plasma might be explained by the presence of SEZ-specific antibodies in equine plasma, but not in human plasma. The level of SEZ-specific antibodies in equine plasma may be too high to reduce C3b deposition through SzM-mediated complement evasion. This interpretation is in accordance with the finding that IgG antibodies bind mainly via the Fab part to streptococci incubated in the plasma [Citation31].
The isogenic mutants C2ΔSzM and C33ΔSzM showed significantly increased deposition of C3b on the bacterial surface compared to the respective wt strains. We agree that it is important to show the gain-of-function through complementation in such experiments. However, we failed to complement these mutants for unknown reasons. As M and M-like proteins are the main virulence and complement evasion factors in different pathogenic streptococci [Citation18,Citation32–34], it appears very likely that this phenotype is due to the in-frame deletion of szm and not a polar effect.
We demonstrated that SEZ C2 recruits human C1q, independent of Ig, to the bacterial surface via SzM_C2 expression. The following findings indicate that SzM-mediated binding of C1q to SzM_C2 is involved in reduced C3b deposition on the bacterial surface: (i) binding of C1q to immobilized rSzM_C2 does not lead to complement activation as determined by C4 deposition (); (ii) soluble rSzM_C2 inhibits complement activation initiated by immobilized heat-aggregated IgG (), and (iii) rSzM_C2, but not rSzM_C33, inhibits the classical complement pathway as measured in different haemolysis assays (). The latter cannot be explained by IgG Fc binding of SzM_C2, as SzM_C33 also binds to the Fc region of IgG [Citation15]. The described functions of rSzM_C2 are in accordance with the hypothesis that the SzM_C2-mediated, Ig independent binding of C1q to SEZ_C2 () contributes to complement evasion in this strain.
Agarwal et al. [Citation35] showed that secreted endopeptidase O (PepO) of S. pneumoniae binds to C1q. Similar to our results for SzM_C2, PepO inhibited the classical complement pathway, as determined in a haemolysis assay with antibodies directed against erythrocytes and human complement. However, binding of C1q to immobilized PepO was associated with C3b deposition, whereas we did not observe deposition of C4 after binding of C1q to immobilized SzM_C2 (). Accordingly, PepO secretion is associated with complement activation and, as a consequence, complement consumption. In contrast, we postulate that the binding of C1q to SzM_C2 prevents complement activation of bound C1q.
Expression of the cell wall-anchored protein SntA by S. suis leads to reduced C3b deposition and MAC formation on the bacterial surface [Citation36]. This complement evasion function is associated with the SntA-mediated binding of C1q. Similar to SzM_C2, SntA interferes with activation of the classical complement pathway, as determined in the haemolysis assay. Thus, different unrelated bacterial proteins are involved in complement evasion through the binding of C1q. However, C1q binding is in comparison to other mechanisms, such as recruitment of the complement inhibitor factor H or fibrinogen, a rather uncommon mechanism of complement evasion in bacteria [Citation18,Citation32,Citation37]. In contrast, numerous parasites, such as Trypanosoma cruzi, Haemonchus contortus and Trichinella spiralis, express the C1q binding protein calreticulin to inhibit the classical complement activation pathway on the parasite surface [Citation38–40]. In T. spiralis, this binding is associated with reduced neutrophil killing of newborn larvae [Citation40], indicating that C1q binding is involved in immune evasion.
Non-immune binding of IgG by M- and M-like proteins is considered to be an immune evasion mechanism, but differences have been described regarding the binding of C1q to this complex. Binding of equine IgG4 and IgG7 to the M protein of S. equi subsp. equi disrupts C1q binding and antibody-mediated activation of the classical complement pathway [Citation41]. Accordingly, we recorded SzM-dependent recruitment of human IgG to the bacterial surface of the SEZ strain C33, but were unable to detect C1q on its surface (). In contrast, IgG bound via Fc to the M-like protein FOG of GGS was still capable of binding C1q.
Binding of C1q to pneumococci mediates not only complement evasion but also adhesion and invasion of epithelial and endothelial cells [Citation42]. Whether C1q is also a bridging molecule to host cells for the SEZ is the subject of further studies.
In this study, we compared the two SzM proteins. Both proteins mediate the survival of SEZ in the human blood [Citation15]. Although the expression of SzM_C33 leads to the decoration of the bacterial surface with human fibrinogen, only SzM_C2 binds directly to C1q. We postulate that both interactions are involved in complement evasion and survival in the human blood. The results of this study are in line with the concept that the diversity of SzM proteins is associated with important differences among SEZ strains in host – pathogen interactions and that SzM is a multifunctional protein.
Materials and methods
Bacterial strains and growth conditions
SEZ was cultured in Todd-Hewitt broth supplemented with 0.5% yeast extract. The isogenic mutants C2ΔSzM and C33ΔSzM carrying in-frame deletions of the respective szm genes were generated in our previous study [Citation15]. E. coli strain XL1 blue carrying pQE30_SzM_C2 or pQE30_SzM_C33 [Citation15] was grown in Luria Bertani (LB) broth or on LB agar including ampicillin (100 µg/ml). M15 medium was used for cultivation of Lactococcus lactis. Bacteria were cultured at 37 °C.
Expression and purification of recombinant his-tagged proteins
Recombinant His-tagged SzM_C2 and SzM_C33 were expressed in E. coli harbouring pQE30_SzM_C2 and pQE30_SzM_C33 [Citation15], respectively, and purified using Protino Ni-TED columns (Macherey-Nagel), as previously described [Citation15].
Plasma and C1q absorption assays with Western blot analysis
Plasma absorption assays of SEZ with equine or human plasma were conducted using acidic glycine buffer, as previously outlined [Citation15]. For the C1q absorption assay, washed bacteria were incubated in PBS with 2 µg human C1q ±10 µg human IgG and 5 µg equine C1q ±20 µg equine IgG.
Supernatants were subjected to SDS-PAGE analysis and staining with InstantBlue (Expedeon), as described [Citation15]. Western blot analysis was conducted after transfer of proteins to nitrocellulose membranes and blocking of membranes with 5 % (w/v) skim milk powder in TBS-T.
Goat IgG anti-horse IgG HRP (Dianova, 108-035-003) and goat IgG anti-human IgG HRP (Dianova, 109-035-088) were used as antibodies against equine and human IgG, respectively. Human and equine C1q were detected using goat IgG anti-human complement 1q/C1q (Calbiochem, Cat. #234390), and rabbit F(ab)2 anti-goat IgG (horradish peroxidase HRP (A24452; Invitrogen). Membranes were developed after washing three times with TBS-T using WesternBright Chemiluminescence Substrate (Biozym).
Equine C1q purification
Equine C1q was purified from horse serum by affinity chromatography using Sepharose-bound rabbit IgG, as described by McKay [Citation43]. Chromatography was conducted using the FPLC system ÄKTAprime plus (code no:03009481) and Sepharose products from GE Healthcare Bio-Sciences AB (Uppsala, Sweden) according to the manufacturer’s instructions.
IgG isolated from rabbit serum with protein G-Sepharose (HiTrap Protein G, 1 mL) was bound to NHS-Sepharose (HiTrap NHS-activated HP, 1 mL) at a concentration of 10 mg/mL. After equilibration of the IgG-Sepharose gel with 50 mM Tris/HCl, 0.1 M NaCl, 20 mM EDTA (pH 7.2), 45 mL horse serum containing 20 mM EDTA was passed through the column at a flow rate of 1 °mL/min. After washing the gel with 30 mL of the mentioned Tris buffer, elution was conducted with a continuous NaCl gradient ranging from 0.1 M to 1.5 M NaCl.
The samples with prominent elution peaks were concentrated to 2 ml via centrifugal filtration (Vivaspin, Sartorius, exclusion limit 30 kDa). The concentrated eluate was fractionated using size-exclusion chromatography (HiLoad 16/600, Superdex 200 pg, code no:28-9893-35) with PBS (pH 7.35) at a flow rate of 1 mL/min. Fractions 24–26 (2 mL per fraction) of the known molecular size range of C1q between 300 and 500 kDa showed the highest purity of C1q and were used for further analysis. Only C1q fractions free of IgG and IgM, which were tested using western blotting, were used in the experiments.
IgG purification with Protein G columns
IgG purification from plasma or sera was performed using Cytiva HiTrap Protein G HP Prepacked Columns, according to the manufacturer’s instructions. The eluted IgG was dialysed against PBS. IgG-free plasma was obtained by applying the plasma multiple times through a HiTrap™ Protein G HP Prepacked Column. Between each run, IgG was eluted from the column and the column was equilibrated with PBS. IgG-free plasma was analysed by western blotting for the complete removal of IgG.
Flow cytometric analysis of C3b deposition on streptococci
Complement deposition on the streptococcal surface was assessed as follows: Streptococci were grown to an OD600 of 0.35, centrifuged, and washed once with PBS. The OD600 value was adjusted again to 0.35. One millilitre of this bacterial suspension was incubated with 100 µL human or equine plasma at 37° C for 30 min. Staining of C3 labelled bacteria was conducted with 250 µL of a 1:250 diluted FITC-conjugated goat F(ab)2 anti-human C3 antibody (Protos Immunoresearch) for 1 h at 4 °C [Citation44]. EDTA-treated plasma was used as the negative control. Samples were measured using a FACSCalibur (BD, Heidelberg, Germany) and analysed using FlowJoTM_V10 software.
rSzM interaction with complement components (ELISA C1q and C4 deposition)
To assess the ability of rSzM to interact with the complement pathway, microtiter plates were coated with 2 µg/well rSzM or heat aggregated human IgG (positive control) in PBS overnight at 4°C. The plates were washed three times with 200 µL TBS-T at each step. The plates were blocked with 200 µL 2 % BSA in TBS-T for 1 h at 37 °C. After blocking, the plates were washed and human plasma (1 %, 0.5 %, and 0.25 % in PBS) was added to the plates and incubated at 37 °C for 1 h. Following the incubation, deposited complement proteins were detected using specific goat IgG anti- human complement 1q/C1q (Calbiochem, Cat.#234390), goat anti-human complement 4/C4 (Calbiochem Cat. #204886), and rabbit F(ab)2 anti-goat IgG HRP (Invitrogen, A24452) in TBS-T 0.2 % BSA. Wells were washed four times with 200 µL TBS-T. One hundred microlitre ABTS solution was added and plates were incubated at 37 °C for 30 min. OD405 nm was measured photometrically. Three independent experiments were conducted in triplicate.
To investigate inhibition of the classical complement pathway by rSzM, 1% human plasma was incubated with increasing concentrations of rSzM (0.125 µg − 4 µg per 100 µL 1 % plasma) at 37°C for 1 h. After pre-incubation, mixtures were added to heat aggregated human IgG-coated plates (2 µg/well) and incubated at 37 °C for 1 h. Deposited complement C4 was detected, as described above.
Haemolysis assay
The interference of rSzM with the activation of the classical complement pathway was investigated in a haemolysis assay with porcine serum directed against sheep erythrocytes [Citation24]. In all experiments, erythrocytes were washed three times with PBS and adjusted to 1 % dilution. To investigate complement inhibition in haemolysis assays, 80 µg rSzM protein was pre-incubated with 200 µL 10% porcine anti-sheep erythrocyte serum at 37 °C for 30 min. After pre-incubation, 50 µL of the 1 % erythrocyte dilution was added and incubated at 37 °C for 1 h. Following the incubation, the samples were centrifuged at 1000 × g to pellet intact cells, and the haemolytic activity (i.e. the number of lysed erythrocytes and released haemoglobin) was determined by spectrophotometric measurement of absorbance at 405 nm. Lysis obtained using ddH2O was defined as 100% haemolytic activity. Three different porcine serum samples, each in triplicate, were used.
Furthermore, a modified haemolysis assay was developed to analyse the interaction of SzM with the human complement system. Briefly, 1% sheep erythrocyte solution was incubated with EDTA (final concentration: 15 mM)-inactivated porcine anti-sheep erythrocyte serum, and erythrocytes were antibody labelled. After washing, 50 µL of the antibody labelled erythrocytes was incubated with 200 µL of 10% human heparinized plasma (as a source of active complement system) pre-incubated with 80 µg rSzM proteins (37 °C for 30 min). Erythrocyte lysis was determined as described previously. Notably, the modified haemolysis assay did not work with equine plasma, as erythrocytes labelled with porcine antibodies and incubated with equine plasma showed no haemolysis (data not shown).
Purified porcine IgG anti-sheep erythrocyte antibodies were used for the haemolysis assay as follows. Briefly, 1 % sheep erythrocyte solution was incubated with porcine IgG anti-sheep erythrocyte antibodies in 15 mM EDTA in PBS. After washing, 50 µL of the antibody-labelled erythrocytes was incubated with 200 µL of 10 % human heparinized plasma (as a source of active complement system) pre-incubated with 80 µg rSzM proteins (37 °C for 30 min). Erythrocyte haemolysis was determined as described previously.
Statistical Analysis
Statistical analysis was performed using Prism software, version 7 (GraphPad, San Diego, CA, United States). Differences between multiple groups were determined using ANOVA followed by Sidak´s or Tukey´s multiple comparisons test. All figures represent the means and SD. Significant differences are indicated by asterisks. Probabilities were considered as follows p < 0.05 *, p < 0.01 **, p < 0.001 ***.
Accession number(s)
Nucleotide sequences of szm of SEZ strains C2 and C33 were deposited in GenBank under the accession numbers MH286972.1 and MH286987.1, respectively.
Availability of data
The data that support the findings of this study are available from the corresponding author [CGB] upon reasonable request.
Supplemental Material
Download PDF (32.3 KB)Acknowledgements
Mark van der Linden (German National Reference Center for Streptococci, Institute of Medical Microbiology, University Hospital RWTH Aachen, Aachen, Germany) kindly provided SEZ strain C33.
Disclosure statement
No potential conflict of interest was reported by the author(s).
Supplemental data
Supplemental data for this article can be accessed online at https://doi.org/10.1080/21505594.2023.2235461
Additional information
Funding
References
- Velineni S, Timoney JF, Russell K, et al. Clones of Streptococcus zooepidemicus from outbreaks of hemorrhagic canine pneumonia and associated immune responses. Clin Vaccin Immunol. 2014;21(9):1246–11. doi: 10.1128/CVI.00222-14
- Las Heras A, Vela AI, Fernández E, Legaz E, Domínguez L, Fernández-Garayzábal JF. Unusual outbreak of clinical mastitis in dairy sheep caused by Streptococcus equi subsp. zooepidemicus.J Clin Microbiol. 2002;40(3):1106–1108. doi: 10.1128/JCM.40.3.1106-1108.2002
- Rasmussen CD, Haugaard MM, Petersen MR, et al. Streptococcus equi subsp. zooepidemicus isolates from equine infectious endometritis belong to a distinct genetic group. Vet Res. 2013;44(1):26. doi: 10.1186/1297-9716-44-26
- Petersen MR, Skive B, Christoffersen M, et al. Activation of persistent Streptococcus equi subspecies zooepidemicus in mares with subclinical endometritis. Vet Microbiol. 2015;179(1–2):119–125. doi: 10.1016/j.vetmic.2015.06.006
- Firth EC, Dik KJ, Goedegebuure SA, et al. Polyarthritis and bone infection in foals. Zentralblatt für Veterinärmedizin Reihe B. 1980;27(2):102–124. doi: 10.1111/j.1439-0450.1980.tb01644.x
- Björnsdóttir S, Harris SR, Svansson V, et al. Genomic dissection of an Icelandic epidemic of respiratory disease in horses and associated zoonotic cases. MBio. 2017;8(4):e00826–17. doi: 10.1128/mBio.00826-17
- de Costa MO, Lage B. Streptococcus equi subspecies zooepidemicus and sudden deaths in swine, Canada. Emerg Infect Dis. 2020;26(10):2522–2524. doi: 10.3201/eid2610.191485
- Chen X, Resende-De-Macedo N, Sitthicharoenchai P, et al. Genetic characterization of Streptococcus equi subspecies zooepidemicus associated with high swine mortality in the United States. Transbound Emerg Dis. 2020;67(6):2797–2808. doi: 10.1111/tbed.13645
- Feng H. Outbreak of swine streptococcosis in Sichan province and identification of pathogen. Anim Husbandry Vet Med Lett. 1977;2:7–12.
- Pelkonen S, Lindahl SB, Suomala P, et al. Transmission of Streptococcus equi subspecies zooepidemicus infection from horses to humans. Emerg Infect Dis. 2013;19(7):1041–1048. doi: 10.3201/eid1907.121365
- Watson JR, Leber A, Velineni S, et al. Recurrent Streptococcus equi subsp. zooepidemicus bacteremia in an infant. J Clin Microbiol. 2015;53:3096–3099. doi: 10.1128/JCM.01306-15
- Ural O, Tuncer I, Dikici N, et al. Streptococcus zooepidemicus meningitis and bacteraemia. Scand J Infect Dis. 2003;35(3):206–207. doi: 10.1080/00365540310000076
- Martinez-Luengas F, Inclan GM, Pastor A, et al. Endocarditis due to Streptococcus zooepidemicus. Can Med Assoc J. 1982;127:13.
- Timoney JF, Walker J, Zhou M, et al. Cloning and sequence analysis of a protective M-like protein gene from Streptococcus equi subsp. zooepidemicus. Infect Immun. 1995;63(4):1440–1445. doi: 10.1128/iai.63.4.1440-1445.1995
- Bergmann R, M-CMC J, Uhlig A, et al. Prominent binding of human and equine fibrinogen to Streptococcus equi subsp. zooepidemicus is mediated by specific SzM types and is a distinct phenotype of zoonotic isolates. Infect Immun. 2020;88(1):e00559–19. doi: 10.1128/IAI.00559-19
- Velineni S, Timoney JF. Identification of novel immunoreactive proteins of Streptococcus zooepidemicus with potential as vaccine components. Vaccine. 2013;31(38):4129–4135. doi: 10.1016/j.vaccine.2013.06.100
- Velineni S, Timoney JF. Characterization and protective immunogenicity of the SzM protein of Streptococcus zooepidemicus nc78 from a clonal outbreak of equine respiratory disease. Clin Vaccin Immunol. 2013;20(8):1181–1188. doi: 10.1128/CVI.00069-13
- Carlsson F, Sandin C, Lindahl G. Human fibrinogen bound to Streptococcus pyogenes M protein inhibits complement deposition via the classical pathway. Mol Microbiol. 2005;56(1):28–39. doi: 10.1111/j.1365-2958.2005.04527.x
- Sandin C, Carlsson F, Lindahl G. Binding of human plasma proteins to Streptococcus pyogenes M protein determines the location of opsonic and non-opsonic epitopes. Mol Microbiol. 2006;56(1):28–39. doi: 10.1111/j.1365-2958.2005.04913.x
- Ermert D, Weckel A, Magda M, et al. Human IgG increases virulence of Streptococcus pyogenes through complement evasion. J Immunol. 2018;200(10):3495–3505. doi: 10.4049/jimmunol.1800090
- Thern A, Stenberg L, Dahlbäck B, et al. Ig-binding surface proteins of Streptococcus pyogenes also bind human C4b-binding protein (C4BP), a regulatory component of the complement system. J Immunol. 1995;154(1):375–386. doi: 10.4049/jimmunol.154.1.375
- Koroleva IV, Sjöholm AG, Schalén C. Binding of complement subcomponent C1q to Streptococcus pyogenes: evidence for interactions with the M5 and FcRA76 proteins. FEMS Immunol Med Microbiol. 2006;20(1):11–20. doi: 10.1111/j.1574-695X.1998.tb01106.x
- Pohl DA, Gibbons JJ, Tsai CC, et al. Isolation and purification of human C1q from plasma. J Immunol Methods. 1980;36(1):13–27. doi: 10.1016/0022-1759(80)90090-3
- Seele J, Beineke A, Hillermann LM, et al. The immunoglobulin M-degrading enzyme of Streptococcus suis, IdeSsuis, is involved in complement evasion. Vet Res. 2015;46(1):1–14. doi: 10.1186/s13567-015-0171-6
- Whitnack E, Beachey EH. Inhibition of complement-mediated opsonization and phagocytosis of Streptococcus pyogenes by D fragments of fibrinogen and fibrin bound to cell surface M protein. J Exp Med. 1985;198; 162(6):1983–1997. doi: 10.1084/jem.162.6.1983
- Sanderson-Smith M, De Oliveira DMP, Guglielmini J, et al. A systematic and functional classification of Streptococcus pyogenes that serves as a new tool for molecular typing and vaccine development. J Infect Dis. 2014;210(8):1325. doi: 10.1093/infdis/jiu260
- Khakzad H, Happonen L, Karami Y, et al. Structural determination of Streptococcus pyogenes M1 protein interactions with human immunoglobulin G using integrative structural biology. PLoS Comput Biol. 2021;17(1):e1008169. doi: 10.1371/journal.pcbi.1008169
- Nitsche-Schmitz PD, Johansson HM, Sastalla I, et al. Group G Streptococcal IgG binding molecules FOG and protein G have different impacts on opsonization by C1q. J Biol Chem. 2007;282(24):17530–17536. doi: 10.1074/jbc.M702612200
- Diebolder CA, Beurskens FJ, de Jong RN, et al. Complement is activated by IgG hexamers assembled at the cell surface. Science. 1979;343(6176):1260–1263. 2014. doi: 10.1126/science.1248943
- Cruz AR, den Boer MA, Strasser J, et al. Staphylococcal protein a inhibits complement activation by interfering with IgG hexamer formation. Proc Natl Acad Sci USA. 2021;118(7):e2016772118. doi: 10.1073/pnas.2016772118
- Nordenfelt P, Waldemarson S, Linder A, et al. Antibody orientation at bacterial surfaces is related to invasive infection. J Exp Med. 2012;209(13):2367–2381. doi: 10.1084/jem.20120325
- Whitnack E, Poirier TP, Beachey EH. Complement-mediated opsonization of group A streptococci inhibited by the binding of fibrinogen to surface M protein fibrillae. In: Cabello Felipe, Pruzzo Carla, editors. Bacteria, complement and the phagocytic cell. Springer, Berlin, Heidelberg; 2012. (NATO ASI Series; vol.24). doi: 10.1007/978-3-642-85718-8_15
- Meehan M, Lynagh Y, Woods C, et al. The fibrinogen-binding protein (FgBP) of Streptococcus equi subsp. equi additionally binds IgG and contributes to virulence in a mouse model. Microbiology (N Y). 2001;147(12):3311–3322. doi: 10.1099/00221287-147-12-3311
- Fulde M, Rohde M, Polok A, et al. Cooperative plasminogen recruitment to the surface of Streptococcus canis via M protein and enolase enhances bacterial survival. MBio. 2013;4(2):e00629–12. doi: 10.1128/mBio.00629-12
- Agarwal V, Sroka M, Fulde M, et al. Binding of Streptococcus pneumoniae endopeptidase O (PepO) to complement component C1q modulates the complement attack and promotes host cell adherence. J Biol Chem. 2014;289(22):15833–15844. doi: 10.1074/jbc.M113.530212
- Deng S, Xu T, Fang Q, et al. The surface-exposed protein SntA contributes to complement evasion in zoonotic Streptococcus suis. Front Immunol. 2018;9:1063. doi: 10.3389/fimmu.2018.01063
- Moore SR, Menon SS, Cortes C, et al. Hijacking factor H for complement immune evasion. Front Immunol. 2021;12:602277. doi: 10.3389/fimmu.2021.602277
- Ramírez-Toloza G, Ferreira A. Trypanosoma cruzi evades the complement system as an efficient strategy to survive in the mammalian host: The specific roles of host/parasite molecules and Trypanosoma cruzi calreticulin. Front Microbiol. 2017;8:1667. doi: 10.3389/fmicb.2017.01667
- Suchitra S, Joshi P. Characterization of Haemonchus contortus calreticulin suggests its role in feeding and immune evasion by the parasite. Biochim Biophys Acta. 2005;1722(3):293–303. doi: 10.1016/j.bbagen.2004.12.020
- Shao S, Hao C, Zhan B, et al. Trichinella spiralis calreticulin S-domain binds to human complement C1q to interfere with C1q-mediated immune functions. Front Immunol. 2020;11:572326. doi: 10.3389/fimmu.2020.572326
- Lewis MJ, Meehan M, Owen P, et al. A common theme in interaction of bacterial immunoglobulin-binding proteins with immunoglobulins illustrated in the equine system. J Biol Chem. 2008;283(25):17615. doi: 10.1074/jbc.M709844200
- Agarwal V, Ahl J, Riesbeck K, et al. An alternative role of C1q in bacterial infections: Facilitating Streptococcus pneumoniae adherence and invasion of host cells. J Immunol. 2013;191(8):4235–4245. doi: 10.4049/jimmunol.1300279
- McKay EJ. A simple two-step procedure for the purification of plasma C1q from different animal species. Immunol Lett. 1981;3(5):303–308. doi: 10.1016/0165-2478(81)90010-9
- De Jong NWM, Vrieling M, Garcia BL, et al. Identification of a staphylococcal complement inhibitor with broad host specificity in equid Staphylococcus aureus strains. J Biol Chem. 2018;293(12):4468–4477. doi: 10.1074/jbc.RA117.000599