Abstract
The influence of ethylenediamine and bis(3-aminopropyl)amine insertion into graphite oxide using toluene and ethanol–water media was studied by elemental analysis, X-ray diffraction, X-ray photoelectron spectroscopy, thermogravimetry, temperature-programmed desorption and diffuse reflectance infrared Fourier transform spectroscopy. In every case, amine was incorporated between the basal planes and on the edges of the material through different interactions with epoxy, hydroxyl, carboxyl and carbonyl groups resulting in new-layered materials. Results show that incorporation was homogeneous for ethylenediamine irrespective of the solvent and that the higher level of intercalation in non-polar media was probably a consequence of the inhibition of competitive reactions with the solvent. For bis(3-aminopropyl)amine, the higher reaction times required to obtain satisfactory degrees of intercalation in the non-polar solvent suggests that with this solvent the process is kinetically controlled for longer chain amines.
1. Introduction
Graphite oxide (GO) is a pseudo-two-dimensional layered carbonaceous material rich in oxygen-containing functional groups whose composition depends on the level of oxidation. It is generally accepted that it consists of randomly distributed intact graphitic regions and regions of aliphatic six-membered rings.[Citation1] Epoxy and hydroxyl functionalities lie above and below these carbon layers while carbonyl groups and carboxylic acid groups having strong acidic character decorate the sheet edges.[Citation2] This variety of functional groups bound to the carbon sheets makes the material strongly hydrophilic and gives rise to interesting properties like cation exchange capacity and rich intercalation ability. In this sense, great attention in recent years has been paid to the preparation of intercalated GO composites by intercalation of polar organic molecules, polymers or inorganic materials inside the nanospace between the layers by different methods.[Citation3–6] Furthermore, the exfoliation and subsequent reduction of GO has been proposed as a promising route for large-scale production of graphene sheets and graphene-based materials.[Citation7,Citation8] The electrical, optical and mechanical properties have urged the application of these materials in the fabrication of microelectronic devices.
Most of the reported works are based on the ability of GO to form stable colloidal suspensions in aqueous media.[Citation9,Citation10] However, in some applications, it would be more favourable to improve the dispersion of the material in organic media, as polar aprotic solvents, and to increase its compatibility with polymeric matrices. Therefore, several methods have been explored to attach some molecules to GO as a previous step of chemical functionalization.[Citation6,Citation11,Citation12] Similar approaches have also been applied to reduce the aggregation and restacking of graphene sheets due to the van der Waals interactions. These strategies provide a path to improve the synthesis of the quasi-two-dimensional carbon nanosheets and to tune the properties or even the number of graphene sheets. On the other hand, the use of bifunctional molecules can provide materials with good dispersibility in both aqueous and organic media[Citation13] and could lead to materials with free functional groups or pillared structures which open its applicability as catalytic supports or adsorbents.
With this in mind, we have explored the incorporation of two different amines, a diamine and a triamine, inside the nanospace of a lab-prepared GO. In order to get a better knowledge of the solvent effect on the intercalation process, the preparation of the materials was carried out in two solvents with different polarities, ethanol and toluene, and the materials were characterized by a variety of complementary techniques.
2. Experimental
GO was synthesized from natural graphite powder (99.999% stated purity, −200 mesh, Alfar Aesar) following a modification of Brodie's method.[Citation14] The graphite was added to a reaction flask containing fuming nitric acid (HNO3, 20 ml/g of support) which was previously cooled to 273 K in an iced bath, after that potassium chlorate (8 g/g of support) was slowly added. The reaction was left to proceed for 21 h under stirring, and the final solid was filtered, extensively washed with deionized water until neutral pH and dried under vacuum at 323 K overnight.
Incorporation of ethylenediamine (E) and bis(3-aminoethyl)amine (T) was performed according to a reported method.[Citation15] For each experiment, 0.02 mol amine per gram of GO was dissolved in ethanol and added dropwise to a suspension of GO in water at room temperature. The mixture was stirred for 24 h and then the solution was filtered off, the solid washed with ethanol/water and finally dried under vacuum at 333 K overnight. Samples obtained by this procedure were named GOE and GOT. A second procedure was also studied where the amine and the GO were dispersed in toluene (t). The mixture was stirred for 24 h for GOE-t and GOT-t and for 96 h for GOT-t-96, the solution was filtered off, and the solid washed with toluene and finally dried under vacuum at 333 K.
C, H and N contents of the samples were determined by elemental analysis with a LECO CHNS-932 system. The crystalline phases present in these samples were determined from the X-ray diffraction patterns (XRD). The diffractograms were recorded on a Polycristal X'Pert Pro PANalytical apparatus using Ni-filtered Cu Kα radiation ( nm) and a graphite monochromator. For each sample, Bragg's angles between 4° and 90° were scanned at a rate of 0.04 deg/s.
The amount of oxygen groups present on the surface was determined by thermogravimetric analysis (TG) in a CI Electronics microbalance (MK2-MC5). The sample was purged under flowing He for 2 h and then heated at a 5 K·min−1 rate up to 1023 K. The chemical nature of these functional groups was evaluated by temperature-programmed desorption (TPD) experiments under vacuum in a conventional volumetric system connected to a SRS RGA-200 mass spectrometer. The sample (0.010 g) was evacuated for 30 min at room temperature and ramped to 1023 K at a 5 K·min−1 rate.
Infrared spectra were collected by using a VARIAN 670 spectrometer equipped with a diffuse reflectance accessory. The diffuse reflectance infrared Fourier transform (DRIFT) spectra were recorded by a mercury–cadmium–telluride detector from 256 scans and with a resolution of 4 cm−1. GO and its derivatives were thoroughly ground and mixed with pre-dried potassium bromide to a final concentration of approximately 1% (w/w).
The surface modification was also analysed by X-ray photoelectron spectroscopy (XPS). The spectra were obtained on a ESCAPROBE P spectrometer from OMICROM equipped with a EA-125 hemispherical multichannel Electronics analyzer. The pressure in the analysis chamber was kept below 10−9 Pa. The excitation source was the Mg Kα line (hν=1253.6 eV, 150 W). The binding energy was referenced to the C 1s line at 284.6 eV. The error in determination of electron binding energies and the line widths did not exceed 0.2 eV.
3. Results
3.1. Structural characterization
Elemental analysis of the samples () corroborates the incorporation of amine. In ethanol, the degree of molecular incorporation was similar for both amines, and comparing with parent GO, a reduction in the amount of oxygen groups was evident. In toluene, the amount of triamine incorporated was much lower than that of diamine, and the oxygen groups were reduced to a lower extent than for the ethanol series.
Table 1. Elemental analysis.
Alterations in the structure of GO after the modification treatment are observed in the XRD patterns of the amine-modified derivatives shown in . The layer-to-layer distance or d-spacing for the prepared GO, obtained from the position of the 001 reflection, was 0.55 nm. Since interlayer spacing depends on the degree of oxidation and hydration level, this low value compared with those reported in the literature might suggest a relatively low degree of oxidation and hydration.[Citation15,Citation16] The interlayer distance increased from 0.55 nm for the parent GO to 0.62 and 0.70 nm for GOE and GOT samples, respectively. This is consistent with previous results reporting that the longer the chain of alkylamines, the longer the interlayer spacing.[Citation15] For samples prepared in toluene, the patterns showed the presence of several peaks consequence of layers with different d-spacings. The 001 reflection peak for GOE-t and GOT-t-96 was shifted to lower angles compared to those for samples prepared in ethanol (GOE and GOT), which implied higher interlayer spacing (0.75 and 0.85 nm). The shoulder at 13.3° could be due to residual solvent molecules intercalated between the layers. Moreover, the patterns showed the peak at 15.8°, the same value that for the parent GO, indicating that some galleries remained unoccupied. The relative intensity of this peak can be related to the incorporation degree of the amine molecule and therefore, for longer chain molecules and in apolar media, longer intercalation times are needed. On the other hand, the broad hump that appeared in the range 2θ=20–26° for some of the patterns, and that corresponds to a d-spacing of 0.36 nm, was close to the value for natural graphite, 2 ((a)), and suggested the presence of random or disordered graphitic platelets (turbostratic platelets). For OGT-t-96, the presence of this hump could also be related to the contraction of unoccupied layers caused by the expansion of adjacent layers where amine molecules are intercalated [Citation17] and not only to GO reduction.
3.2. Thermal analyses
The original GO and the modified samples were characterized by thermal analyses, and displays the TG curves. The profile for GO sample showed a strong weight loss of 30% at 535 K that corresponded to the release of different gases, H2O, CO and CO2, during the reductive exfoliation of GO and that was a consequence of the decomposition of the oxygen-containing functionalities, mainly epoxy and OH groups. For higher temperatures, the weight loss followed a slower rate, and a total mass loss of about 40% was eventually reached. The thermograms for the amine-modified samples also showed a pronounced drop but at lower temperature, 430 K, and a continuous or slower decay for higher temperatures. This shift of the main weight loss to lower temperatures is consistent not only with the increase in the interlayer distance observed by XRD, but also with the reducing character of the intercalated molecules. It has been reported that the higher the reducing character of the intercalated molecule, the lower the exfoliation temperature.[Citation17] The total weight loss was in all cases lower than that for GO sample, although the one for the GOT-t-96 comparing with that for GOT-t was consequent with a higher amount of amine incorporated.
Figure 2. Thermograms in He for the amine-modified samples (A) prepared in ethanol (a) GO, (b) GOE and (c) GOT, and (B) prepared in toluene, (a) GO, (b) GOE-t, (c) GOT-t and (d) GOT-t-96.
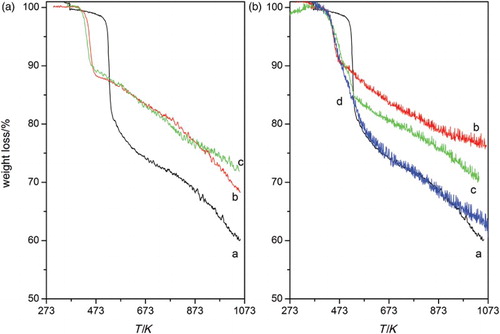
The analysis of the gases evolved during the TPD experiments was also carried out. The TPD profiles for GO and the amine-modified samples are displayed in and , respectively. The profile of the GO sample showed intense signals at 530 K for m/z=18, 44 and 28, which are assigned to H2O, CO2 and CO, coming from the decomposition of the oxygen functional groups. For the amine-modified samples, the fragmentation patterns of the studied amine were considered, and therefore, some other mass/charge ions were monitored during the TPD to study their contribution to the general spectra. To asses for evolution of molecularly adsorbed amine, we have followed signals m/z=30 and 73 for diamine and triamine, respectively. Mass 73 was selected because major fragment for the triamine molecule is m/z=44 and can be masked by the CO2 contribution. The lack of m/z 30 and 73 could be taken as a proof that other kinds of interactions were responsible of the amine attachment. The first point to remark when analysing the TPD profiles was that an intense peak at 470 K and a small shoulder at higher temperatures, 573 K, were observed for samples prepared in ethanol (). Contributions to the main peak of m/z=18, 17, 16 (not shown), 44 and 28 were identified. The intense and wide peak obtained for m/z=18 was assigned to water desorption. The presence of masses m/z=44 and 28 seemed to indicate that the amine thermal decomposition contributed to the profile. In addition, the appearance of a shoulder at 723 K with contributions of m/z 17 and 44 seemed to reflect the additional linkage of the amine through stronger bonds. This peak was probably due to decomposition of the amine in NH3 and fragments leading to m/z=44. The evolution of m/z=2 assigned to H2 was observed at high temperatures and could be in line with the dehydrogenation of the amine or of its decomposition products.
Figure 4. TPD profiles for amine-modified samples prepared in toluene (a) GO, (b) GOE-t, (c) GOT-t and (d) GOT-t-96.
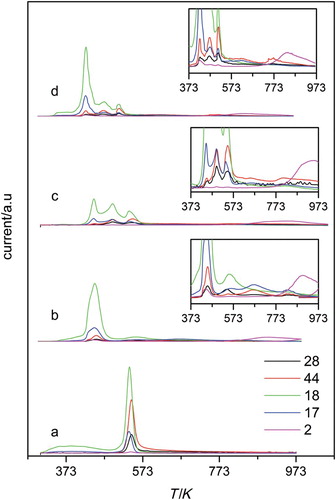
The general spectra were clearly different for samples prepared in toluene (). While for the diamine-modified sample, the TPD showed one broad peak at 450 K and a small one at 560 K, for the triamine samples they showed three main peaks at 440, 490 and 530 K. Again, the masses that mainly contributed to the profile were 18, 17, 28 and 44 whose relative contribution increased considerably in the two peaks at higher temperatures. The temperature range of the latter peak, i.e. 530–540 K, corresponded to the temperature of GO exfoliation. Thus, this peak must be attributed to the decomposition of functional groups in the residual not intercalated GO structures, which were also detected by XRD. In addition, as occurred for samples prepared in ethanol, a small shoulder at 680 K with contributions of masses 17 and 44 was detected. This indicated that amine was strongly adsorbed on other type of oxygen-surface groups different from hydroxyl and epoxy groups. An increase in m/z=2 for temperatures starting at 873 K can reveal, as suggested above, the dehydrogenation of the adsorbed amine.
3.3. DRIFT
The infrared spectra of GO also changed after modification (). Peaks appearing in the range 3000–3600 and at 1380 cm−1 which were assigned to the stretching and bending vibrations of the acidic ‒ OH groups [Citation12,Citation15,Citation18] seemed to be significantly attenuated for both modified series. For samples prepared in ethanol, the band at 1713 cm−1 due to C˭ O stretching vibration of carbonyl and/or carboxyl groups situated at the edges of the lamellae disappeared, while the peak at 1578 cm−1 could indicate the presence of un-oxidized graphitic domains.[Citation18] New bands at 1410 and 1216 cm−1 could be assigned to δ CH2 and ρ NH2, respectively,[Citation19] and would confirm the presence of amine. On the other hand, new features related to the presence of amine could be detected in the samples prepared in toluene, i.e. peaks at 1667 and 1565 cm−1.[Citation12] The appearance of these two peaks added to the fact that the C˭ O stretching vibration was absent or shifted to lower wavenumber could indicate the formation of an amide bond. Therefore, these peaks would correspond to the amide carbonyl stretching mode and the coupling of the C‒ N stretching vibration with the CHN deformation vibration, respectively.[Citation15] However, these two bands could also be assigned to asymmetric and symmetric deformation bands of hydrogen-bonded NH2.[Citation20] This would indicate that amine molecules reacted with acidic hydroxyl groups forming hydrogen-bonded NH2 groups. Therefore, DRIFT spectra of the functionalized-samples are not conclusive concerning amidation, but they have allowed the identification of amine functionalities and of differences in the interaction depending on the solvent used in the synthesis.
3.4. XPS analysis
XPS analyses were carried out to asses the type of functional groups on the prepared samples. The relative amounts of the different species, C, O and N, were calculated from the corresponding peak areas divided by the sensitivity factors (1.00 for C, 2.85 for O and 1.77 for N) and are shown in . The O/C ratios obtained for the GO series showed a small decrease in the oxygen content after the functionalization with amines at expenses of an increase in the N/C ratio, which suggested the incorporation of the amine. Moreover, the N/C ratios for the toluene series were higher than for the ethanol series implying a greater proportion of amines over the surface of the samples of that series. Comparing the data of the two amines in each series, the higher N/C ratios for the triamine samples were consequent taking into account the number of amine groups in the molecule. The N/C ratio obtained for GOT-t-96 was also remarkable.
Table 2. XPS results.
According to the possible components contributing to the C 1s region in carbon materials, the position of the maximum for the high-resolution C 1s spectra of the GO sample (supplementary material, Figure SI 1), 285.1 eV and the absence of the peak characteristic of large polyaromatic structures were coherent with the structure of the GO. Thus, the creation of epoxy and hydroxyl groups induced this loss in the aromatic character of the graphene sheets. Besides, the asymmetry of the peak was also consequence of the high proportion of oxygen groups. After incorporation of the amines, the maxima of the C 1s spectra shifted to lower BE, which indicated that the contribution due to graphitic structures had increased. Furthermore, a shoulder at intermediate BE has developed and, in fact, was maximum for GOT-t-96 sample, and could be ascribed to the presence of C‒ N structures and to a change in the concentration of the different type of oxygen groups.
The modification in the type of groups that occurred after intercalation of both amines was reflected by the shift of the maximum of the O 1s spectra to lower BE. Therefore, information about the nature of the surface oxygen groups was obtained by deconvolution of the peak in four components: carbonyl oxygen (peak A, 531.4); oxygen atoms in C‒ O bonds like hydroxyl and ether groups (peak B, 532.6); oxygen atoms in acidic carboxyl groups (peak C, 534.6) and adsorbed water (peak D, 535.6). shows the relative area of the different peaks. The data suggested a decrease in the proportion of carboxyl groups that was slightly higher for samples prepared in toluene. This went along with an increase in the C˭ O type groups.
The N1s peak could also be deconvoluted in two components at 399.5 (N1) and 400.5 eV (N2) (supplementary material, Figure SI 2). The assignation of species was complicated but a tentative assignment from the literature could be hydrogen-bonded and hydrogen-bonded protonated amines, respectively, species that seemed to coexist and that were in equilibrium.[Citation19] For the samples prepared in toluene, the N1/N2 ratio decreased, which would point to a higher proportion of protonated species.
4. Discussion
The incorporation of amine molecules to the GO interlayer has been assessed by all the characterization techniques applied in this study and seems to depend on the solvent used in the preparation and on the size or length chain of the molecule. The relative orientation of molecules intercalated on GO depends on their structure and functionalities, and on the type of interaction with the GO.[Citation19,Citation21,Citation22] According to these variables, some of the possible conformations are shown in . Briefly, with the alkyl chain parallel to the layer forming a one- or double-layer structure; with the chain titled leading to a tail conformation; or bridge or loop conformations in the case of bifunctional molecules.[Citation18] The d-spacing values of our samples vary in the range 0.62–0.85 nm and seem to indicate that amine molecules are probably arranged in a bilayer or tail orientation to yield such values. The amount of amine molecules incorporated when the samples were prepared in the polar solvent was similar for both ethylenediamine (E) and bis(3-aminoethyl)amine (T) and their distribution very homogeneous as the single peak detected in the XRD spectra suggested. In apolar media where interferences due to the solvent–solid interactions should be absent, the level of incorporation of E was higher, while it decreased for T. In addition, the remaining peak at 2 in the XRD spectra of OGT-t and OGT-t-96 reflects the existence of unoccupied galleries. Furthermore, the discrepancy between the N content from elemental analysis and the XPS results, where the N/C ratios showed that T amine was incorporated in a higher extent on the samples prepared in toluene, also confirms the heterogeneity in OGT-t and OGT-t-96 samples. All these facts can only be rationalized if it is considered that T molecules are located close to or at the edges of GO layers in the toluene samples. Consequently, these amine molecules could be hindering the access of other molecules to the interlayer. Besides, the higher levels of incorporation obtained for sample treated during 96 h would be indicating that the intercalation is a kinetically controlled process that depends on the chain length and number of functionalities of the amine and on the solvent.
Scheme 1. Representation of possible conformations for intercalated mono- and bifunctional molecules on graphite oxide: a) monolayer, b) bilayer, c) tail, d) bridge e) loop. •: functional group
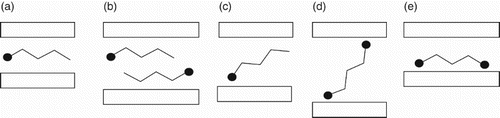
On the other hand, the elemental analysis and the O/C ratios obtained by XPS prove that the incorporation of amine reduces the amount of oxygen groups. The decrease in the DRIFT bands associated to the oxygen groups, added to the decrease in the weight loss observed for all the TG profiles of the modified samples, seems to confirm the elimination of some of the oxygen-containing groups due to reaction with the amine or to the partial reduction forming disordered carbon. The ratios obtained by XPS () have also pointed to partial reduction of the GO structure that is higher in the samples prepared in ethanol. The small development in the XP spectra of the 291 eV peak that is associated with the polyaromatic character and the D/G intensity ratios obtained by Raman (not shown) seem to indicate that the reduction implies not the generation of a continuous sp2 phase, but most likely the creation of sp2 domains isolated by disordered domains.[Citation23]
The question of the type of interaction between the amine and the oxygen groups is not trivial to explain. The amine must be mainly adsorbed either by hydrogen bonding interactions between amine moieties and the OH groups or by nucleophilic attack on the epoxy groups, although the exchange of protons with acidic groups at the periphery of the GO layers cannot be disregarded. Furthermore, contrary to what it has been reported for graphite and carbon nanofibers series also modified with bis(3-aminoethyl)amine,[Citation24] m/z=27 was absent in the TPD profile for the amine-modified GO samples. The presence of mass m/z=27, which is assigned to desorption of HCN during the TPD, was associated in that case to the interaction of the amine with the carboxylic acid groups. Then, its absence in the profiles of amine-modified GO samples suggests a small number of amide species, consistent with the low amount of carboxylic groups, or that the amine undergoes different chemical transformations during the TPD run and leads to desorption of other products. The formation of amide bonds has been reported after SOCl2 activation of the carboxylic groups.[Citation25] So, other groups present on the GO different from carboxylic groups seem to be involved in the attachment of the amine molecule. Several groups based in XRD and infrared analysis have proposed the nucleophilic attack to the epoxy groups as the main route of insertion.[Citation15,Citation26] Our results seem to be more in agreement with the interaction via hydrogen bonding forming hydrogen-bonded and hydrogen-bonded protonated species. Furthermore, the deconvolution of the N 1s region of the XPS has shown that the solvent used in the synthesis influences the ratio between both types of species. The lower N1/N2 ratios for samples prepared in toluene would also reflect a higher proportion of hydrogen-bonded protonated species that would be in line with the favoured acid–base interaction between the amine and the surface acid groups, i.e. the hydroxyl groups and/or the carboxylic groups located on the borders of the graphene sheets. This interaction is favoured in this case because the solvent used for the preparation does not interfere or react with the solid surface. However, it is also important to highlight that those assignments were suggested for alkylamines,[Citation19] and in our case, we are studying amines with two and three NH2 groups. Therefore, the presence of some additional amine groups should also be considered.
5. Conclusion
In conclusion, the method of preparation of amine-modified GO derivatives is crucial in determining the properties of the material, i.e. the amount of amine incorporated and the type of interaction. Specifically, the temperature and time of reaction, the solvent, the alkyl chain of the amine molecule to be incorporated and the previous reduction or not of the GO nanosheets are the main parameters to be controlled. The incorporation of amines into GO with a polar solvent, ethanol–water, implies a homogeneous distribution, but the partial reduction of the GO structure. The resulting material does not contain large size sp2 domains, but abundant and isolated by disordered domains. The degree of incorporation when the apolar solvent, toluene, is used depends on the amine chain length: the larger the molecule the lower the amount of amine incorporated and the homogeneity. Comparing both solvents, toluene gives better levels of intercalation than ethanol for ethylendiamine due to the lack of interferences caused by the solvent and the GO. Finally, the amine molecules inserted in the GO interlayers seem to be mainly retained by hydrogen bonding interactions with the OH species.
Supplementary online material. A more detailed information on experiments is available at http://dx.doi.org/10.1080/21642311.2014.913817.
Supplementary material
Download MS Word (132.5 KB)Acknowledgement
The authors acknowledge the MICINN of Spain (Projects CTQ-2011-29272-C04-01 and -03) for financial support.
References
- He H, Klinowski J, Forster M, Lerf A. A new structural model for graphite oxide. Chem Phys Lett. 1998;287:53–56. doi: 10.1016/S0009-2614(98)00144-4
- Jeong H-K, Lee YP, Lahaye RJWE, Park M-H, An KH, Kim IJ, Yang C-W, Park CY, Ruoff RS, Lee YH. Evidence of graphitic AB stacking order of graphite oxides. J Am Chem Soc. 2008;130:1362–1366. doi: 10.1021/ja076473o
- Hu Z-L, Aizawa M, Wang Z-M, Yoshizawa N, Hatori H. Synthesis and characteristics of graphene oxide-derived carbon nanosheet-Pd nanosized particle composites. Langmuir. 2010;26(9):6681–6688. doi: 10.1021/la9040166
- Matsuo Y, Higashika S, Kimura K, Miyamoto Y, Fukutsuka T, Sugie Y. Synthesis of polyaniline-intercalated layered materials via exchange reaction. J Mater Chem. 2002;12:1592–1596. doi: 10.1039/b107436a
- Wang Z-M, Hoshinoo K, Shishibori K, Kanoh H, Ooi K. Surfactant-mediated synthesis of a novel nanoporous carbon-silica composite. Chem Mater. 2003;15:2926–2935. doi: 10.1021/cm020965c
- Matsuo Y, Fukunaga T, Fukutsuka T, Sugie Y. Silylation of graphite oxide. Carbon. 2004;42:2113–2130. doi: 10.1016/j.carbon.2004.03.024
- Pei S, Cheng H-M. The reduction of graphene oxide. Carbon. 2012;50:3210–3228. doi: 10.1016/j.carbon.2011.11.010
- Guardia L, Villar-Rodil S, Paredes JI, Rozada R, Martínez-Alonso A, Tascón JMD. UV light exposure of aqueous graphene oxide suspensions to promote their direct reduction, formation of graphene–metal nanoparticle hybrids and dye degradation. Carbon. 2012;50:1014–1024. doi: 10.1016/j.carbon.2011.10.005
- Park S, Ruoff RS. Chemical methods for the production of graphenes. Nat Nanotechnol. 2009;4:217–224. doi: 10.1038/nnano.2009.58
- Stankovich S, Dikin D, Piner RD, Kohlhaas KA, Kleinhammes A, Jia Y, Wu Y, Nguyen SBT, Ruoff RS. Synthesis of graphene-based nanosheets via chemical reduction of exfoliated graphite oxide. Carbon. 2007;45:1558–1565. doi: 10.1016/j.carbon.2007.02.034
- Georgakilas V, Bourlinos AB, Zboril R, Steriotis TA, Dallas P, Stubos AK, Trapalis C. Organic functionalisation of graphenes. Chem Commun. 2010;46:1766–1768. doi: 10.1039/b922081j
- Stankovich S, Piner RD, Nguyen ST, Ruoff RS. Synthesis and exfoliation of isocyanate-treated graphene oxide nanoplatelets. Carbon. 2006;44:3342–3347. doi: 10.1016/j.carbon.2006.06.004
- Xu C, Wu X, Zhu J, Wang X. Synthesis of amphiphilic graphite oxide. Carbon 2008;46(2):386–389. doi: 10.1016/j.carbon.2007.11.045
- Brodie BC. On the atomic weight of graphite. Philos Trans R Soc Lond. 1859;149:249–259. doi: 10.1098/rstl.1859.0013
- Bourlinos AB, Gournis D, Petridis D, Szabó T, Szeri A, Dékány I. Graphite oxide: chemical reduction to graphite and surface modification with primary aliphatic amines and amino acids. Langmuir. 2003;19:6050–6055. doi: 10.1021/la026525h
- You S, Luzan SM, Szabo T, Talyzin AV. Effect of synthesis method on solvation and exfoliation of graphite oxide. Carbon. 2013;52:171–180. doi: 10.1016/j.carbon.2012.09.018
- Barroso-Bujans F, Cerveny S, Verdejo R, del Val JJ, Alberdy JM, Alegría A, Colmenero J. Permanent adsorption of organic solvents in graphite oxide and its effect on the thermal exfoliation. Carbon. 2010;48:1079–1087. doi: 10.1016/j.carbon.2009.11.029
- Szabó T, Berkesi O, Forgó P, Josepovits K, Sanakis Y, Petridis D, Dékány I. Evolution of surface functional groups in a series of progressively oxidized graphite oxides. Chem Mater. 2006;18:2740–2749. doi: 10.1021/cm060258+
- Herrera-Alonso M, Abdala AA, McAllister MJ, Aksay IA, Prud'homme RK. Intercalation and stitching of graphite oxide with diaminoalkanes. Langmuir. 2007;23:10644–10649. doi: 10.1021/la0633839
- Matsuo Y, Miyabe T, Fukutsuka T, Sugie Y. Preparation and characterization of alkylamine-intercalated graphite oxides. Carbon. 2007;45:1005–1012. doi: 10.1016/j.carbon.2006.12.023
- Matsuo Y, Niwa T, Sugie Y. Preparation and characterization of cationic surfactant intercalated graphite oxide. Carbon. 1999;37:897–901. doi: 10.1016/S0008-6223(98)00226-7
- Dékány I, Krüger-Grasser R, Weiss A. Selective liquid sorption properties of hydrophobized graphite oxide nanostructures. Colloid Polym Sci. 1998;276:570–576. doi: 10.1007/s003960050283
- Singh V, Joung D, Zhai L, Das S, Khondaker SI, Seal S. Graphene based materials: past, present and future. Progress Mater Sci. 2011;56:1178–1271. doi: 10.1016/j.pmatsci.2011.03.003
- Dongil AB, Bachiller-Baeza B, Guerrero-Ruiz A, Rodríguez-Ramos I, Martínez-Alonso A, Tascón JMD. Surface chemical modifications induced on high surface area graphite and carbon nanofibers using different oxidation and functionalization treatments. J Col Inter Sci. 2011;355:179–189. doi: 10.1016/j.jcis.2010.11.066
- Niyogi S, Bekyarova E, Itkis ME, McWilliams JL, Hamon MA, Haddon RC. Solution properties of graphite and graphene J Am Chem Soc. 2006;128:7720–7721. doi: 10.1021/ja060680r
- Wang S, Chia P-J, Chua L-L, Zhao L-H, Png R-Q, Sivaramakrishnan S, Zhou M, Goh RG-S, Friend RH, Wee AT-S, Ho PK-H. Band-like transport in surface-functionalized highly solution-processable graphene nanosheets. Adv Mater. 2008;20:3440–3446. doi: 10.1002/adma.200800279