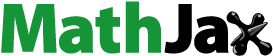
Abstract
The present study synthesised and characterised zinc oxide nanoparticles (ZnO NPs) using spinach tree, Cnidoscolus aconitifolius and investigated its potential use as nanofertilizer. The synthesised nanoparticles showed UV–Vis absorption peak at 378 nm which is a feature of ZnO NPs. FT-IR analysis further revealed the presence of O-H stretching, C = C bending, O-H bending and C-N stretching functional groups of the stabilising action of the plant extract on the surface of the nanoparticles. SEM images displayed the shape of NPs to be spherical whereas TEM images showed their distribution sizes to be 100 nm. Synthesised ZnO NPs were used as a nano fertilizer on Sorghum bicolour plant. An increase in the shoot leaf length with an average length of 16.13 ± 0.19 cm as compared to the control group of 15.13 ± 0.07 cm was observed. The rate of photosynthesis also showed a significant increase with total chlorophyll content of 0.2806 ± 0.006 mg/mL as compared with control of 0.2476 ± 0.002 mg/mL. The activity of antioxidative enzymes was measured with an increase in the specific activity of SOD in the plant when ZnO NPs were used over NPK whereas, the specific activities of CAT were similar in all cases.
GRAPHICAL ABSTRACT
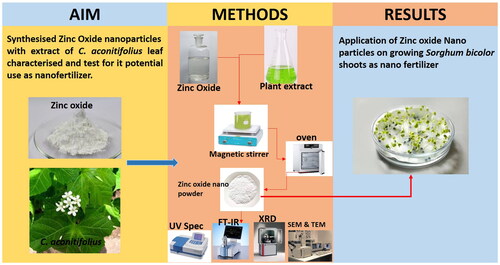
Introduction
Nanotechnology is an accelerating technology that has transformed many scientific scopes and being a ground-breaking technology plays an important role in distinctive fields of life science such as medicine, pharmaceuticals, agricultural science etc [Citation1,Citation2]. Nanotechnology has a wide range of agricultural uses [Citation3] such as nanofertilizers [Citation4], nanopesticides [Citation5], and nano-enabled environmental restoration [Citation6]. A number of studies have shown that nanoparticles can improve seed germination [Citation7], plant development, increase yield [Citation8], and resistance against metal oxide toxicities [Citation9]. In order to increase food crops at levels of sustainability and productivity and to contribute to agriculture security, nanotechnology has been presented as a key instrument and strategy for implementing new products in agriculture at the nanoscale scale [Citation7].
Researchers are investigating the use of nanoparticles (NPs) in various types of plants, including monocotyledonous and dicotyledonous species, both in regulated and natural environments. The characteristics of NPs, such as their shape, concentration, size, composition, and surface properties, can affect their impact on plant growth during the germination and seedling phases [Citation10]. Several studies have examined the biosynthesis of ZnO NPs using different natural plant extracts, and the results suggest that the properties of the synthesised samples depend on the source of the plant extract used []. The green synthesis method relies on bioactive chemicals present in the plant extract to synthesise NPs. These substances can reduce metal ions to metal nanoparticles through various oxidation reactions. Functional groups in phytochemical substances such as polyphenols can chelate metal ions and form coordinated complexes, which are then thermally broken down to produce the desired NPs. Phytochemicals can also act as stabilising agents to prevent the formation of agglomerated NPs [Citation11]. Research conducted on the effects of Zinc oxide nanoparticles (ZnO NPs) on Arachis hypogaea plants showed an increase in seed germination, rapid shoot growth, heightened seedling vigour index, fast root growth, and an accelerated blooming stage, leading to a significant increase in pod size and yield [Citation12]. In another investigation, the use of ZnO NPs resulted in an increase in germination rate and protein content, indicating improved reproductive efficiency in Solanum [Citation13]. Multiple studies have demonstrated the positive impact of ZnO NPs on food crop yield [Citation14–20]. Bionanofertilizers have been designed to enhance the growth and productivity of cultivated plants by providing essential nutrients through the use of nanomaterials or nanocomplexes [Citation16,Citation21].
Micronutrients such as zinc (Zn), copper (Cu), and iron (Fe) are widely used to promote healthy plant growth and development. They play critical roles in crop plant metabolism and biophysical characteristics, acting as cofactors to enhance the function of different metabolic enzymes. Nanofertilizers have been developed to provide micronutrients that offer ample nutrients for crops without harming the environment, thereby positively influencing crop growth and yields []. Zinc is an essential micronutrient that regulates enzyme activity in the metabolic pathways of plants [Citation22–24]. ZnO nanoparticles are soluble in the soil and have unique properties that make them useful in some agriculture applications. For example, the use of ZnO NPs has shown significant improvements in plant biomass, root and shoot length, chlorophyll and protein content, and phosphatase enzyme activity in various plants, including Brassica napus, Cicer ariatium, Cluster bean, Cucumis sativas, Gossypium hirsutum, Raphanus sativus, and Vigna radiata [Citation21]. Zinc oxide NPs have been responsible for crop growth, biomass, grain yield and efficiency [Citation21,Citation25]. This induced growth and outcome could be caused by various nano-mediated soil and plant levels mechanisms. These mechanisms can cover genomic change, the alteration of plant uptake of mineral nutrients, reactive oxygen species (ROS) production and photosynthesis, gas exchange, as well as plant growth and biomass induced. ROS-generated radicals act as signalling molecules, activating the plant’s antioxidant defence mechanism by using both enzyme agents, such as superoxide dismutase (SOD), catalase (CAT), ascorbic peroxidase (APX), guacol peroxidase (GPX), dehydroascorbate reductase (DHAR) and glutathione reductase (GR) and non-enzymatic antioxidants such as ascorbate, glutathione, thione, phenolics, etc. [Citation26–28].
Photosynthesis, which is the basis for all plant metabolic functions, is considered to be one of the most vulnerable physiological processes to environmental pressures. Various literature reports have indicated that metal oxide nanoparticles affect the energy transfer efficiency of isolated reaction centres, leading to changes in plant photosynthetic efficiency, photochemical fluorescence, and quantum yield [Citation29–32]. Studies using ZnO NPs on tomato plants with varying exposure durations have shown an increase in chlorophyll content [Citation33]. Global agricultural systems are facing increasing strain due to rising food demand, climate change, and environmental concerns. One potential solution to improve the efficiency of agrochemicals (fertilisers and pesticides) is through the use of nanostructures, which can reduce runoff, increase foliar absorption and bioavailability, and have lower environmental consequences. However, there is a gap in knowledge regarding the synthesis and use of these nanoparticles as fertilisers that needs further exploration. This article reports on the green synthesis of ZnO NPs using Cnidoscolus aconitifolius and its potential as a bionanofertilizer as we have reported the green synthesis of ZnO NPs from this plant and its antioxidant activities [Citation34]. The level of toxicity exerted by the NPs on sorghum plants was evaluated by measuring the activity of antioxidant enzymes (catalase and superoxide dismutase). The plant’s ethnopharmacological potential, as reported in previous studies, prompted its use in this research [Citation62,Citation63].
Materials and methods
Preparation of leaf extracts
Fresh matured leaves of Chaya Cnidoscolus aconitifolius was rinsed thoroughly with distilled water to remove all dust particles. The leaves were first finely chopped and then 10 g were blended in 100 ml of distilled water to form a slurry. This was kept in a water bath at 60 °C for 10 min and then cooled to room temperature. The aqueous plant extract (PE) was obtained by filtering through Whatman filter paper No. 1 and the filtrate was stored at 4 °C until further use [Citation35]. The total phenolic content (TPC) of the leaf extract is expressed as microgram Tannic Acid Equivalent (mg TAE) per gram of aqueous leaf extract of C. aconitifolius. The total flavonoids content was determined using the aluminium chloride (AlCl3) method and was expressed as Quercetin Equivalents (mg QE/g) [Citation36].
Green synthesis of ZnO NPs
The ZnO NPs were biosynthesized using the method of Sabir et al. [Citation64] as modified by Mfon et al. [Citation22]. To 30 ml leaf extract was added 70 ml of 0.5 M Zn(CH3COO)2 and 30 ml of 0.2 M NaOH. The mixture was stirred for 2 h using a magnetic stirrer and the resulting precipitate was kept in a water bath at 50 °C for 9 h. The colloidal suspension was centrifuged at 3000 rpm for 5 min and the supernatant was discarded. The precipitate was washed twice with distilled water and then oven-dried for 7 h. The product obtained was characterised using UV–Visible spectrophotometer (Shimadzu 3600 spectrophotometer Japan); FT-IR spectrophotometer (Shimadzu IR-Tracer 100 Japan); X-ray Diffractometer (PXRD) (SMART Bruker D8 Advance X-145 Ray Diffractometer; Cu-Kα radiation (λ = 1.54 A˚) Miniflex 600, Rigaku Corporation, Japan); Transmission electron microscope (FEI–Tecnai G2 20 S-TWIN Germany) and Scanning electron microscope (FE-SEM, Carl-Zeiss model ultra-microscope 55,151 Germany). In order to validate the need to add both NaOH and the plant extract, the same procedure was followed except that in one case only NaOH was added to the solution of Zn(CH3COO)2 and in another solution of Zn(CH3COO)2 only the plant extract was added. The UV-Vis spectra of each sample was obtained.
Nanofertilizer test
Sorghum bicolour seeds were soaked in ZnO NPs and the NPK solutions (100 mgL−1) and also in distilled water as control for 4 h and placed in a dry oven for 1 day at 25 °C. The seeds were germinated in Petri dishes filled with moistened cotton wool treated with 100 mgL−1 of the solutions of the ZnO NPs and NPK. These treatments were repeated a week after seed germination. Petri dishes with cotton wool moistened with distilled water served as blank control. Leaf length was measured in intervals of two days after germination and the total chlorophyll was determined 10 days after germination. The effect of ZnO NPs on the antioxidative enzymes superoxide dismutase (SOD) and catalase (CAT) on the plant was also investigated [Citation26].
Leaf length measurement
Leaf length was measured in intervals of two days after germination with a metre rule. The length was recorded as a mean of three readings.
Determination of chlorophyll content
Total chlorophyll content was determined according to the method of Asimovic et al.[Citation37]. Fresh leaves of the Sorghum bicolour shoot were washed thoroughly with distilled water. 1.0 g of fresh plant leaf sample was weighed and homogenised in 10 ml distilled water in a blender. Chlorophyll was extracted by addition of 10 ml acetone/methanol (80/20% v/v) and filtered through Whatman filter paper No.1. The mixture was then centrifuged at 5000 rpm for 5 min. Spectrophotometric determinant – ion of chlorophyll content was carried out by absorption measurements at 665 nm and 649 nm respectively and calculated according to the following equation [Citation37]:
Where;
= Total chlorophyll (a + b) in mg/g,
Absorbance at 649 nm
Absorbance at 665 nm
volume of supernatant collected and
W = weight of leaves.
Determination of superoxide dismutase activity
The method of McCord and Fridovich [Citation38] and modified by Kumar et al. [Citation39] was applied for the determination of the effect of antioxidant enzymes on the plant. To 150 µL of 75 mM of Tris-HCl buffer (pH 8.2), 30 mM EDTA, 30 µL of the sample and 30 µL of 2.0 mM of pyrogallol are added. An increase in absorbance was recorded at 420 nm for 3 min by a UV-Vis spectrophotometer. One unit of enzyme activity is 50% inhibition of the rate of autoxidation of pyrogallol as determined by the change in absorbance/min at 420 nm. The specific activity was expressed as unit/mg protein. The activity was calculated according to the following expression [Citation39].
Where A0 = absorbance after 30 s and A3 = absorbance after 150 s
Determination of catalase activity
Catalase (CAT) activity was measured spectrophotometrically at room temperature by monitoring the decrease in absorbance at 240 nm resulting from the decomposition of H2O2. Catalase activity was measured according to the previous method [Citation40]. The reaction mixture contained 100 mM sodium phosphate buffer (pH 7.4), 20 mM H2O2 and 50 µL of the test sample in a total volume of 1.10 ml. The extinction coefficient of H2O2 is 46.3 M−1cm−1 at 240 nm [Citation41]. One unit of activity is equal to 1.0 M of H2O2 degraded per minute and is expressed as units per milligram of protein. The specific activity was estimated by the following expression [Citation41].
Where: change in absorbance per min (min−1)
Df = dilution factor,
Av = total assay volume (mL)
hydrogen peroxide extinction coefficient (43.6M−1cm−1)
Ev = enzyme volume (mL)
Pc = total protein (mg)
l = path length (1 cm)
Results and discussion
Evaluated total phenolic and flavonoids contents
The result of TPC value and TFC content is shown in the TPC value was 509.96 ± 0.44 mg TAE/g from the leaf extract of C. aconitifolius while the TFC value was 133.08 ± 0.83 mg QE/g. This showed the presence of both phenolic and flavonoids compounds in the aqueous extract responsible for the reduction of the metal, as capping agents for the synthesis and stabilisation of the NPs as well as responsible for the bioactivity of the NPs synthesised [Citation42].
Table 1. Total phenolic content and flavonoids in aqueous extract of C. aconitifolius. The results are shown for n = 3 determinations as the average ± standard error of mean (SEM).
Characterisation of Zinc oxide nanoparticles
UV-VIS absorption Spectroscopy of the ZnO NPs
The absorption spectrum showed a characteristic peak at 350 nm of the green synthesised ZnO NPs (). The energy gap was calculated as 3.3 eV which is consistent with previous research [Citation65]. UV-VIS absorption spectroscopy is a widely used technique to examine the optical properties of nanosized particles [Citation66]. Surface Plasmon Resonance (SPR) is responsible for the unique optical properties of ZnO NPs by factors such as particle size, particle shape, their distance from each other (concentration) and refractive index of the changes in the surrounding environment [Citation43]. The surface plasmon resonance (SPR) of ZnO NPS is between 310–380 nm [Citation44]. The UV-Vis spectra, revealed that the plant extract contains phytochemicals that are UV-Vis active with peaks around 275–278 nm and another around 316 nm. On the formation of ZnO NP (red line), the peak at 316 disappears and there is a gentle sloping peak of 371 nm, while the PE peak at shifted to lower wavelength (269 nm).
Fourier transform Infra-Red (FT-IR) Spectroscopy of ZnO NPs
The FT-IR spectra of the plant extract and the ZnO NP are shown in . The IR spectrum of the ZnO NP revealed peaks at 3358, 2926 and 2855 cm−1 corresponding to functional groups of O–H, C–H(sp3) (stretching vibration). Other related peaks present were 1021 cm−1 (C–O stretching alcohols), 932 cm−1 (O–H bending), 676 − 670 cm−1 (=C–H bending). The spectra also revealed bands at 1579 cm−1 (1˚ amine), 1334 cm−1 (stretching aromatic amines), 1077 cm−1 (C–N stretching aliphatic amines), which indicates the presence of primary amines denotes the availability of polyamines [Citation45]. Previous literature strongly supports the presence of IR bands in ZnO NPs and other metal oxides [Citation35,Citation46,Citation47].
X-ray diffraction (XRD) analysis of ZnO NPs
XRD profile of the green synthesised ZnO NPs, showed several Bragg peaks corresponding to the orientation of ZnO NPs with diffraction peaks at (2θ) 31.21°, 36.34°, 39.70° and 47.01° of (100), (101), (110) and (112) planes respectively (). These planes of hexagonal zinc oxide can be indexed to the wurtzite ZnO with high crystallinity (Joint Committee on Powder Diffraction Standard no. 36–1451). A specific line broadening of diffraction peaks indicates that the synthesised materials are in the nanometre range and the manifestation of the peak at 2θ = 36.34° is the confirmation of ZnO NPs [Citation48].
TEM and SEM imaging of the ZnO NPs
The TEM micrographs of ZnO NPs are shown in , where the size distribution of these ZnO NPs size was 200 nm. Image J (version 1.53t 24 August 2022) was used to measure the size of these nanoparticles from the TEM micrographs. SEM micrograph showed that zinc oxide nanoparticles synthesised using C. aconitifolius were spherical. The micrographs were showing zinc oxide nanoparticles synthesised using C. aconitifolius in the scale bar of 0.1 µm and a distribution size of 100 nm ().
Leaf length
ZnO nanoparticles easily dissolve in soil and are uptaken by plants and are employed in a wide range of applications in agriculture, due to their unique properties. In this study, the use of ZnO NPs as nanofertilizers was investigated using the Sorghum bicolour plant as a model. Results obtained from the test of ZnO NP as nanofertilizer indicate a significant increase in leaf length caused by the nanoparticles as against the control group (). However, the NPK treated group showed the highest leaf length, this indicates that macronutrients such as phosphorus (P), nitrogen (N), potassium (K), are required in large quantities in agricultural farm activities. Be as it may, ZnO NPs were able to demonstrate their role as needed in the growth and development of the plant as results shown were significantly different from the control group. Similar studies reported that tomato plants sprayed with foliar ZnO NPs promoted the highest plant height, root length, biomass increased chlorophyll content [Citation17]. Also, ZnO NPs sprayed by foliar on pearl millet show the shoot and root growth, chlorophyll (photosynthetic pigment) content, and total soluble leaf protein, which significantly increased [Citation49].
Total chlorophyll content
The result obtained from this study suggests that the application of ZnO NPs increased the plant photosynthetic pigment. There was no significant difference between the total chlorophyll content obtained from the inorganic fertiliser NPK and the synthesised ZnO NPs () also reported ZnO NPs treatments under different durations of exposure showed an increase in the chlorophyll content level in tomato plants [Citation33]. This study is in agreement with other studies that ZnO NPs are found to increase photosynthesis by an increase in chlorophyll content [Citation50]. It has also been established that a decrease in chlorophyll content of leaves leads to a gradual inactivation of photosynthesis which is detrimental to plants thereby leading to a reduction in the energy produced in the form of adenosine triphosphate (ATP) thereby a reduction in the plant cell activities which includes growth [Citation37]. Hence, chlorophyll could be used as an index for plant growth, as an increase in the amount of chlorophyll will ultimately yield an increase in the photosynthetic rate and thereby increasing plant growth.
Superoxide dismutase and catalase activities
It is presumed that metal and metal oxide nanoparticles exposure could cause oxidative stress when exposed to crop plants by generating reactive oxygen species (ROS). In this study, increased activity of SOD was observed () which means there was an increase in the oxidative stress which in turn increases the oxidative enzyme expression. However, studies suggested that the application of NPs at low concentrations was found effective in alleviating various abiotic stresses and enhancing plant growth and development [Citation51,Citation52]. In the case of catalase (), there was no significant difference between the two groups treated with NPs and inorganic fertiliser. However, both treated groups showed increase activities of the enzyme as compared with the control group. Other studies have shown NPs to cause oxidative stress by an increase in the activity of antioxidant enzymes that exposure to CuO and ZnO NPs caused oxidative stress in wheat, which was evidenced by increased lipid peroxidation level and oxidised glutathione rate in roots [Citation21,Citation53,Citation54]. As described above, generated ROS radicals act as signalling molecules, thereby activating the plant antioxidant defence mechanism of plants employing both enzymatic agents, such as superoxide dismutase (SOD) and catalase (CAT) and nonenzymatic antioxidants, such as ascorbate, glutathione, phenolics, etc [Citation28].
Conclusions
Green synthesis of nanoparticles using plants has been regarded as a cheap, eco-friendly and effective method for the delivery of drugs. ZnO NPs are a representative soluble nanoparticles that can be readily used as fertiliser due to its ability to be easily biotransform. These results indicate that the could be use as alternative fertilisers. These studies mainly focussed on the increase in leaf length, increase rate of photosynthesis and the impact of oxidative stress on antioxidants enzymes, also the toxicity studies could be carried out to ascertain the threshold concentration to be use for different plant species. Subsequent studies could be carried out to assess the plant growth, dynamic transformation processes and how it will impact particle fate in the tissues. These findings will enable us to understand the mechanisms of ZnO NPs absorption and distribution in plant leaves and will provide appreciated information to guide the sustainable use of ZnO NPs and potentially NPs in agriculture.
Author contributions
R.S.D, R.C.G and F.K.A designed the experiments. R.S.D performed the experiments, R.C.G, R.S.D and U.O.S analysed the data with supervision by F.K.A and R.S.D wrote the manuscript.
Acknowledgements
The authors are grateful for the laboratory facilities provided by Department of Biochemistry and Toxicology Institute of Occupational Health, Johannesburg.
Disclosure statement
No potential conflict of interest was reported by the author(s).
Data availability statement
The data that support the findings of this study are available from the corresponding author, R.S.D, upon reasonable request.
Additional information
Funding
References
- Dave PN, Chaturvedi S. 2021. The economic contributions of nanotechnology to green and sustainable growth. In Handbook of Functionalized Nanomaterials (pp. 365–380). Elsevier, Amsterdam.
- Fakhari S, Jamzad M, Kabiri Fard H. Green synthesis of zinc oxide nanoparticles: a comparison. Green Chem Lett Rev. 2019;12(1):19–24.
- Kah M, Tufenkji N, White JC. Nano-enabled strategies to enhance crop nutrition and protection. Nat Nanotechnol. 2019;14(6):532–540.
- Okey‐Onyesolu CF, Hassanisaadi M, Bilal M, et al. Nanomaterials as nanofertilizers and nanopesticides: an overview. ChemistrySelect. 2021;6(33):8645–8663.
- Liu Q, Li F, Lu H, et al. Enhanced dispersion stability and heavy metal ion adsorption capability of oxidized starch nanoparticles. Food Chem. 2018;242:256–263.
- Sharma S, Singh S, Ganguli AK, et al. Anti-drift nano-stickers made of graphene oxide for targeted pesticide delivery and crop pest control. Carbon. 2017;115:781–790.
- Fincheira P, Tortella G, Duran N, et al. Current applications of nanotechnology to develop plant growth inducer agents as an innovation strategy. Crit Rev Biotechnol. 2020;40(1):15–30.
- Dimkpa CO, White JC, Elmer WH, et al. Nanoparticle and ionic Zn promote nutrient loading of sorghum grain under low NPK fertilization. J Agric Food Chem. 2017;65(39):8552–8559.
- Chaudhary RG, Bhusari GS, Tiple AD, et al. Metal/metal oxide nanoparticles: toxicity, applications, and future prospects. Curr Pharm Des. 2019;25(37):4013–4029.
- Avellan A, Yun J, Morais BP, et al. Critical review: role of inorganic nanoparticle properties on their foliar uptake and in planta translocation. Environ Sci Technol. 2021;55(20):13417–13431.
- Abdullah JAA, Eddine LS, Abderrhmane B, et al. Green synthesis and characterization of iron oxide nanoparticles by pheonix dactylifera leaf extract and evaluation of their antioxidant activity. Sustainable Chem Pharm. 2020;17:100280.
- Fuku X, Diallo A, Maaza M. Nanoscaled electrocatalytic optically modulated ZnO nanoparticles through green process of Punica granatum L. and their antibacterial activities. Int J Electrochem. 2016;2016:1–10.
- Naseer M, Aslam U, Khalid B, et al. Green route to synthesize zinc oxide nanoparticles using leaf extracts of Cassia fistula and Melia azadarach and their antibacterial potential. Sci Rep. 2020;10(1):1–10.
- Sackey J, Nwanya A, Bashir AKH, et al. Electrochemical properties of Euphorbia pulcherrima mediated copper oxide nanoparticles. Mater Chem Phys. 2020;244:122714.
- Aldeen TS, Mohamed HEA, Maaza M. ZnO nanoparticles prepared via a green synthesis approach: physical properties, photocatalytic and antibacterial activity. J Phys Chem Solids. 2022;160:110313.
- Soren S, Kumar S, Mishra S, et al. Evaluation of antibacterial and antioxidant potential of the zinc oxide nanoparticles synthesized by aqueous and polyol method. Microb Pathog. 2018;119:145–151.
- Singh A, Singh NB, Hussain I, et al. Green synthesis of nano zinc oxide and evaluation of its impact on germination and metabolic activity of Solanum lycopersicum. J Biotechnol. 2016;233:84–94.
- Adrees M, Khan ZS, Hafeez M, et al. Foliar exposure of zinc oxide nanoparticles improved the growth of wheat (Triticum aestivum L.) and decreased cadmium concentration in grains under simultaneous cd and water deficient stress. Ecotoxicol Environ Saf. 2021;208:111627.
- El-Mahdy MT, Elazab DS. Impact of zinc oxide nanoparticles on pomegranate growth under in vitro conditions. Russ J Plant Physiol. 2020;67(1):162–167.
- Liu R, Lal R. Potentials of engineered nanoparticles as fertilizers for increasing agronomic productions. Sci Total Environ. 2015;514:131–139.
- Raliya R, Nair R, Chavalmane S, et al. Mechanistic evaluation of translocation and physiological impact of titanium dioxide and zinc oxide nanoparticles on the tomato (Solanum lycopersicum L.) plant. Metallomics. 2015;7(12):1584–1594.
- Šebesta M, Kolenčík M, Sunil BR, et al. Field application of ZnO and TiO2 nanoparticles on agricultural plants. Agronomy. 2021;11(11):2281.
- Watson JL, Fang T, Dimkpa CO, et al. The phytotoxicity of ZnO nanoparticles on wheat varies with soil properties. Biometals. 2015;28(1):101–112.
- Zafar H, Ali A, Ali JS, et al. Effect of ZnO nanoparticles on brassica nigra seedlings and stem explants: growth dynamics and antioxidative response. Front Plant Sci. 2016;7:535–539.
- Priyanka N, Geetha N, Ghorbanpour M, Venkatachalam, P. 2019. Role of engineered zinc and copper oxide nanoparticles in promoting plant growth and yield: present status and future prospects. Advances in Phytonanotechnology, 183–201.
- Kalra T, Tomar PC, Arora K. Micronutrient encapsulation using nanotechnology: nanofertilizers. Plant Arch. 2020;20:1748–1753.
- Nongbet A, Mishra AK, Mohanta YK, et al. Nanofertilizers: a smart and sustainable attribute to modern agriculture. Plants. 2022;11(19):2587.
- Wang SL, Nguyen AD. Effects of Zn/B nanofertilizer on biophysical characteristics and growth of coffee seedlings in a greenhouse. Res Chem Intermed. 2018;44(8):4889–4901.
- Mfon RE, Odiaka NI, Sarua A. Interactive effect of colloidal solution of zinc oxide nanoparticles biosynthesized using Ocimum gratissimum and Vernonia amygdalina leaf extracts on the growth of Amaranthus cruentus seeds. African Journal of Biotechnology. 2017;16(26):1481–1489.
- Saboor A, Ali MA, Hussain S, et al. Zinc nutrition and arbuscular mycorrhizal symbiosis effects on maize (Zea mays L.) growth and productivity. Saudi J Biol Sci. 2021;28(11):6339–6351.
- Umair Hassan M, Aamer M, Umer Chattha M, et al. The critical role of zinc in plants facing the drought stress. Agriculture. 2020;10(9):396.
- Sharma P, Urfan M, Anand R, et al. Green synthesis of zinc oxide nanoparticles using Eucalyptus lanceolata leaf litter: characterization, antimicrobial and agricultural efficacy in maize. Physiol Mol Biol Plants. 2022;28(2):363–381.
- Rai-Kalal P, Jajoo A. Priming with zinc oxide nanoparticles improve germination and photosynthetic performance in wheat. Plant Physiol Biochem. 2021;160:341–351.
- Tondey M, Kalia A, Singh A, et al. Seed priming and coating by nano-scale zinc oxide particles improved vegetative growth, yield and quality of fodder maize (Zea mays). Agronomy. 2021;11(4):729.
- Tripathi DK, Singh S, Singh VP, et al. Silicon nanoparticles more efficiently alleviate arsenate toxicity than silicon in maize cultiver and hybrid differing in arsenate tolerance. Front Environ Sci. 2016;4:46–56.
- Giglou MT, Giglou RH, Esmaeilpour B, et al. A new method in mitigation of drought stress by chitosan-coated iron oxide nanoparticles and growth stimulant in peppermint. Ind Crops Prod. 2022;187:115286.
- Mony C, Kaur P, Rookes JE, et al. Nanomaterials for enhancing photosynthesis: interaction with plant photosystems and scope of nanobionics in agriculture. Environ Sci Nano. 2022;9(10):3659–3683.
- Rico CM, Barrios AC, Tan W, et al. Physiological and biochemical response of soil-grow barley (Hordeum vulgare L.) to cerium oxide nanoparticles. Environ Sci Pollut Res Int. 2015;22(14):10551–10558.
- Sun H, Qu G, Li S, et al. Iron nanoparticles induced the growth and physio-chemical changes in Kobresia capillifolia seedlings. Plant Physiol Biochem. 2023;194:15–28.
- Faizan M, Faraz A, Yusuf M, et al. Zinc oxide nanoparticle-mediated changes in photosynthetic efficiency and antioxidant system of tomato plants. Photosynt. 2018;56(2):678–686.
- Dangana RS, George RC, Agboola FK. The biosynthesis of zinc oxide nanoparticles using aqueous leaf extracts of Cnidoscolus aconitifolius and their biological activities. Green Chem Lett Rev. 2023;16(1):2169591.
- Reddy SB, Mandal BK. Facile green synthesis of zinc oxide nanoparticles by Eucalyptus globulus and their photocatalytic and antioxidant activity. Adv Powder Technol. 2017;28(3):785–797.
- Liao WC, Lai YC, Yuan MC, et al. Antioxidative activity of water extract of sweet potato leaves in Taiwan. Food Chem. 2011;127(3):1224–1228.
- Asimovic Z, Sarajevo FS, Cengic L, et al. Spectrophotometric determination of total chlorophyll content in fresh vegetables. Godina LXI Broj. 2016;66:104.
- McCord JM, Fridovich I. Superoxide dismutase: an enzymic function for erythrocuprein (hemocuprien). J Biol Chem. 1969;244(22):6049–6055.
- Kumar A, Dutt S, Bagler G, et al. Engineering a thermo-stable superoxide dismutase functional at sub-zero to > 50 C, which also tolerates autoclaving. Sci Rep. 2012;2(1):1–8.
- Aebi H. B. Isolation, purification, characterization and assay of antioxygenic enzymes. (13) Catalase in vitro. Methods Enzymol. 1984;105:121–126.
- Aebi H. 1970. Methoden der enzymatischen analyse. Vol 1. (Bermeyer, H. U. ed.) Verlag Chemie, Weinheim, p. 637.
- Bandeira M, Giovanela M, Roesch-Ely M, et al. Green synthesis of zinc oxide nanoparticles: a review of the synthesis methodology and mechanism of formation. Sustainable Chem Pharm. 2020;15(2):100223.
- Salem SS, Fouda A. Green synthesis of metallic nanoparticles and their prospective biotechnological applications: an overview. Biol Trace Elem Res. 2021;199(1):344–370.
- Agarwal H, Shanmugam V. A review on anti-inflammatory activity of green synthesized zinc oxide nanoparticle: mechanism-based approach. Bioorg Chem. 2020;94:103423.
- Zare E, Pourseyedi S, Khatami M, et al. Simple biosynthesis of zinc oxide nanoparticles using nature’s source and it’s in vitro bio-activity. J Mol Struct. 2017;1146:96–103.
- Ahmed B, Ishwarya R, Alsalhi MS, et al. Green fabrication, characterization and antibacterial potential of zinc oxide nanoparticles using Aloe socotrina leaf extract: a novel drug delivery approach. J Drug Delivery Sci Technol. 2020;55(9):101465.
- Muthuvel A, Jothibas M, Manoharan C. Effect of chemically synthesis compared to biosynthesized ZnO-NPs using Solanum nigrum leaf extract and their photocatalytic, antibacterial and in vitro antioxidant activity. J Environ Chem Eng. 2020;8(2):103705.
- Raoufi D. Synthesis and microstructural properties of ZnO nanoparticles prepared by precipitation method. Renew Energy. 2013;50:932–937.
- Tarafdar JC, Raliya R, Mahawar H, et al. Development of zinc nanofertilizer to enhance crop production in pearl millet (Pennisetum americanum). Agric Res. 2014;3(3):257–262.
- Singh A, Singh NÁ, Afzal S, et al. Zinc oxide nanoparticles: a review of their biological synthesis, antimicrobial activity, uptake, translocation and biotransformation in plants. J Mater Sci. 2018;53(1):185–201.
- Soliman AS, El-Feky SA, Darwish E. Alleviation of salt stress on moringa peregrina using foliar application of nanofertilizers. Journal of Horticulture and Forestry. 2015;7(2):36–47.
- Venkatachalam P, Jayaraj M, Manikandan R, et al. Zinc oxide nanoparticles (ZnO NPs) alleviate heavy metal-induced toxicity in Leucaena leucocephala seedlings: a physiochemical analysis. Plant Physiol Biochem. 2017;110:59–69.
- Zhu J, Shen S, Zeng X, et al. Environmental science. Environment Science Nano. 2020;1(2):1–43.
- Dimkpa CO, McLean JE, Britt DW, et al. Nano-CuO and interaction with nano-ZnO or soil bacterium provide evidence for the interference of nanoparticles in metal nutrition of plants. Ecotoxicology. 2015;24(1):119–129.
- Iwalewa EO, Adewunmi CO, Omisore NOA, et al. Pro-and antioxidant effects and cytoprotective potentials of nine edible vegetables in Southwest Nigeria. J Med Food. 2005;8(4):539–544.
- Oyagbemi AA, Odetola AA, Azeez OI. Ameliorative effects of Cnidoscolus aconitifolius on anaemia and osmotic fragility induced by protein energy malnutrition.Afr J Biotechnol. 2008;7(11):1721–1726.
- Sabir S, Arshad M, Chaudhari SK. Zinc oxide nanoparticles for revolutionizing agriculture: synthesis and applications. Scientific World J. 2014;2014:1–8.
- Pranjali P, Meher MK, Raj R, et al. Physicochemical and antibacterial properties of PEGylated zinc oxide nanoparticles dispersed in peritoneal dialysis fluid. ACS Omega. 2019;4(21):19255–19264.
- Manjunatha R, Usharani K, Dhananjay N. Synthesis and characterization of ZnO nanoparticles: a review. J Pharmacognosy Phytochem. 2019;8(3):1095–1101.