Abstract
Metastatic melanoma cancer stem cells are subpopulations linked to tumour development, immunoevasive behaviour, treatment resistance, and metastasis, all of which contribute to a poor prognosis. Photodynamic treatment (PDT) is an alternate strategy to cancer eradication that involves the generation of reactive oxygen species. As a carrier, nanoparticles enable efficient cellular uptake of photosensitizers, improving organelle accumulation and cancer cell targeted therapy. This study considered at the effect of PDT on CD133+ Melanoma Stem Cells utilising an Aluminium Phthalocyanine Gold Nanoparticle (AlPcS4Cl-AuNP) combination. A ligand exchange approach was used to conjugate AlPcS4Cl–PEG-AuNP-COOH and was characterised using UV-Vis, FTIR, DLS and Zeta Potential. Stem cells isolated from the A375 cell line irradiated with a laser at 673.2 nm with a fluency of 5 J/cm2 were evaluated. Furthermore, it was important to study if apoptosis was one of the mechanisms causing to cell death which was substantiated with Annexin V/PI, caspase 3 and p53 analysis. The nanoparticle conjugate mediated PDT promoted apoptotic cell death, showing increased expression of p53 and caspase-3. The study proposed a strategy aimed at extending the understanding of PDT in enhancing the therapy of melanoma, suggesting a probable improved cell death when AlPcS4Cl was conjugated to AuNPs.
Introduction
Metastatic melanoma has a poor prognosis, particularly in patients whose disease has advanced to visceral metastases, with statistics indicating a median survival rate of only a few months [Citation1]. Melanoma was the third most frequent disease diagnosed worldwide in Australia in 2020, according to the Global Cancer Observatory. As a result, South Africa ranks just 16th globally in terms of melanoma incidence rates, behind Australia and New Zealand. In South Africa, in 2020, there were 1777 new cases of melanoma of the skin reported, affecting people of all ages and genders with 480 deaths. South Africa was rated 22nd in the world in terms of mortality rate in 2020 [Citation2]. Men are more likely than women to be diagnosed with melanoma. Men have a 1 in 13 probability of developing melanoma by the age of 85, while women have a 1 in 21 probabilities. The anticipated number of new instances in Africa for both sexes and ages 0 to 85 years has increased from 6963 to 13650 from 2020 to 2040 [Citation2].
In human malignant melanoma, the presence and involvement of cancer stem cells (CSCs) in tumour origin and development, chemo-resistance, and treatment failure is becoming increasingly clear [Citation3]. Targeted therapies that tries to stifle a tumour’s survival pathways and processes for growth within a cancer cell has previously yielded significant benefits. Despite the fact that targeted therapy has shown to have short-term therapeutic benefits in a variety of solid tumour types, most molecularly chosen patients with advanced cancer stages are not cancer-free after treatment [Citation4,Citation5]. These consequences necessitate more study into better therapy interventions and alternative strategies for Metastatic Melanoma and cancer stem cell therapy. Melanoma treatments are presently stage dependent. In situ melanoma is treated with surgical resection, lymph node mapping, and amputation, proceeded by interferon therapy if a relapse is detected. In the latter stages, when tumours are unresectable or relapse, immunotherapy and chemotherapeutic drugs are utilised. Targeted therapy with signal transduction inhibitors and palliative care are also utilised to improve patients’ quality of life [Citation6,Citation7].
Chemotherapy has the drawbacks of damaging healthy cells and having serious adverse effects on patients, including anaemia, fatigue, secondary infections, constipation, nausea, and vomiting. Due to numerous resistance mechanisms, malignant melanomas only respond to these types of medications in about 20% of cases [Citation8]. In contrast to external radiation, which is applied across a considerably greater region and is therefore viewed as less exact, internal administration precisely administers radiation exclusively to the patient’s body’s affected area. Radiation therapy has unwanted side effects that can harm healthy surrounding tissues and include skin changes, exhaustion, and nausea [Citation9]. Unlike chemotherapy, biological treatments work by helping the immune system fight cancer rather than by directly destroying the disease’s rapidly growing cells. Due to immune-related side effects and resistance considerations, molecular-targeted treatment is not effective in all patients [Citation10].
Several of reports have identified stem cells as responsible for driving melanomas. In a study, human metastatic melanoma cells were shown to self-renew, preserve multipotency, advance as spheroid cells, and be supplemented for tumour-forming capacity in vitro and in vivo. Melanoma spheroid cells did not express CD133, but they did initiate tumour growth in vivo, and CD20+ cells proliferated to create larger spheres than CD20+ cells [Citation11]. In both primary and metastatic clinical human malignant melanomas, another investigation found that the drug efflux transporter ABCB5 was preferentially expressed on subpopulations of CD133+ cancer cells [Citation11].
Most solid tumour cases evaluated have a lower occurrence of CSCs in their microenvironments. According to xenotransplantation in immune-deficient gene-modified mice, the incidence and proliferation of single human Melanoma CSCs in Melanoma tumours can reach 27% [Citation12]. Some of the prime Malignant Melanoma antigenic stem cell markers have been recognised as CD20 [Citation13], CD133 [Citation14] ,ABCB5 [Citation11], CD271 [Citation15] ,and ALDH1A [Citation16]. Prominin-1, also known as CD133, is a pentaspan transmembrane protein found in plasma membrane protrusions, including microvilli play a crucial role in membrane receptor communication [Citation17]. Subsequent to implantation into immune-deficient mice, CD133 expressing CSCs demonstrated self-rejuvenation capability and the potential to produce a histologically comparable tumour [Citation18]. The expression of CD133 has been linked to chemo-resistance and improved metastatic capacity in a variety of human malignancies [Citation19], despite the mechanisms underlying this being unknown.
Photodynamic therapy (PDT) has proven to be both a promising and effective cancer treatment. PDT has been listed and recognised by the US Food and Drug Administration in clinical trials as one of the more recent cancer treatment methods [Citation20]. With its impressive results, PDT is being employed to treat cancer either alone or in combination with conventional treatment modalities like surgery and chemotherapy [Citation21,Citation22]. Systematic PDT strategies have been developed in some Western countries [Citation23–25]. The mechanisms of action of photodynamic treatment involve two sorts of reactions. The first happens when the light stimulates the PS, forcing an electron to shift to a higher energy level [Citation9]. Light stimulation of the photosensitizers (PS) activates an additional action mechanism. When the energy is transported to the molecular oxygen ground state, singlet oxygen species are stimulated, which disrupts cellular processes and causes tumour death [Citation26].
Normal cells evade and limit PDT effects through the photosensitiser’s (PS's) space- and time-selective uptake properties, while laser radiation is focused directly at the tumour [Citation27].The most important and initial effector molecules of PDT are external reactive oxygen species (ROS) produced by cold photochemical reactions. The presence of PDT-induced ROS, in addition to external ROS, can cause endoplasmic reticulum (ER) stress and intracellular metabolic changes [Citation28]. As a result, the primary target position in the cell for photochemical reactions is frequently close to the subcellular constituents where the PS is localised, explaining the heterogeneity of PS effects [Citation29].
Photodynamic therapy with PSs such as Aluminium Phthalocyanines (AlPcSn) on melanomas suggests a positive outlook for the successful management of melanoma skin cancer, or even in conjunction with other therapeutic alternatives. Increased PS absorption and storage in tumours may improve circulatory half-life, cellular, or subcellular selecting. Conjugation of gold nanoparticles (AuNPs) to PS has been shown to increase the fluorescence by 150 times. This was justified in a study by Xu et al. in 2014, where AlPcS-AuNP conjugate increased fluorescence imaging of cancer cells [Citation30]. Gold nanoparticle features encompass tuneable optics and photothermal capabilities, which allow laser light to generate heat, enhancing directed cellular damage [Citation31]. Suitable gold nanoparticles for biological applications are those that are biocompatible and have low inherent toxicity [Citation32]. In a 2017 study by Mohammadi et al. they discovered that 5-aminolevulinic acid (5ALA) PS was double the efficiency than 5ALA when coupled with a gold nanoparticle. This effect could be used to improve melanoma cell death [Citation33].
Subcellular uptake and localisation of a PS into selected cells is one of the factors that determine the success of PDT. This is also especially important in establishing the system of photo-induced cell death and its’ effectiveness [Citation34]. Different cell death pathways are triggered by the PS's localisation in different cell organelles. PDT mechanisms generate ROS which generally causes cell death through apoptosis, autophagy, and or necrosis alone or in combination [Citation35]. At physiological amounts, ROS uphold cytokine balance and cell nutrition; conversely, in rare circumstances, minor fluctuations in ROS levels can have a dramatic influence on stem cell fate [Citation36], directly induce CSC heterogeneity in tumours or cause CSC differentiation [Citation24]. Furthermore, ROS molecules participate in a number of biological processes such as protein translation, gene expression and protein-protein or nucleic acid communications [Citation37]. PDT may increase systemic immunity or enhance tumour immunity by releasing ROS [Citation38]. Although little is known about how ROS play a role in stem cell immunity, researchers have recognised that understanding how ROS metabolism affects stem cell fate will help them better understand how to use PDT to prevent distant CSCs from spreading. PDT is typically used in skin cancer treatments, but due to its immunogenicity, it could also be used to eradicate systemic CSCs.
In melanoma cell lines, PDT enhances apoptosis by both intrinsic and extrinsic apoptotic mechanisms, according to studies. PDT may also induce necrosis and/or autophagy [Citation39]. There are three significant signalling routes at play. One method is the intrinsic pathway, which results in cellular death through DNA damage, p53 activation, and p53-mediated regulation of antiapoptotic proteins and kinases [Citation40]. By elevating ER stress levels and activating caspase cascade pathways, PSs have also been shown to cause intrinsic apoptosis. Lysosomes and mitochondria may also suffer harm as a result of PSs. PDT concentrations above sublethal dosages trigger the ER stress pathway, disrupting Ca2+ homeostasis and unfolding proteins, which cause apoptosis or autophagic cell death [Citation41,Citation42]. Another pathway involves the extrinsic pathway is triggered by oligomeric death ligands. The third pathway involves the release of granzyme B, a serine protease, into the target cell by cytotoxic T lymphocytes and natural killer cells, which causes apoptosis. These cellular death pathways depend on cysteine protease caspases to break down the cellular matrix at a common final stage where they converge. PDT-treated melanoma cells release caspase-3, caspase-8, and caspase-9 [Citation39].
Methods and materials
Stem Cell Isolation and Maintenance – MACS CD133 Microbead Kit. The European Collection of Authenticated Cell Cultures was used as the supplier for Human Malignant Melanoma cell line A375 (ECACC no: 88113005). Incubation conditions were at 85% humidity, 37 °C, and 5% CO2. Dulbecco’s Modified Eagle Medium (DMEM) complete medium was used for culturing; supplemented with 10% Foetal Bovine (FBS) Serum, 1% Amphotericin-B, and 1% Penicillin-Streptomycin. Using a Magnetic Activated Cell Sorting System (MACS), QuadroMACS Separator (Miltenyi Biotec, 130-090-976), and the cancer stem cell marker CD133 (MicroBead Kit, Miltenyi Biotec, 130-100-830), the CSCs were isolated from the rest of the cell population. The QuadroMACS Separator uses magnetism which separated cells using LS and MS columns positioned on the magnetic field. Isolated stem cells were subsequently characterised and analysed for toxicity post AlPcS4Cl-AuNP conjugate PDT treatment. The culture media for stem cells was supplemented with 5% FBS, 1% Penicillin-Streptomycin 1% Amphotericin-B, incubated at 85% humidity, 37 °C, 5% CO2.
Conjugation and characterisation of AlPcS4Cl and PEG-AuNP-COOH
Conjugation
Direct electrostatic contact between the Pc sulphate (S) and the NP surface results in self-assembly. Ligand exchange occurs on the NP surface as a result of direct coordination of the Pc S and/or N to the NP [Citation43]. For Pc-NP mixing, a single solvent, such as water, was utilised. Polymer chains on the PEGylated AuNPs were attached to the S groups on the AlPcS4Cl PS using a ligand exchange method, as shown in . A concentration of 35 µM, which was the standardised dosage for the A375 total cell population, was added to 50 ppm AuNPs (Sigma-Aldrich, 765465) in Eppendorf® LoBind microcentrifuge tubes (Sigma-Aldrich, Z666556). A Multifunction Vortex Mixer Set with a Microtube Platform Head (VM-10) (DAIHAN-brand®, N05042000008187) vortexed the mixture at room temperature overnight at high speed, avoiding subjection to light. Repetitive centrifugation for 1 h at 18,000 rpm (HeraeusTM FrescoTM 17 Microcentrifuge, Thermo ScientificTM, 75002402) was used to purify the mixture. After removing the supernatant, the product was resuspended and centrifuged again to extract all unbound PS.
Spectrophotometry (Ultraviolet-Visible)
The synthetic conjugate was suspended and subjected to spectroscopic examination between the wavelengths of 400 nm and 800 nm. For subsequent tests, a AuNP-PS conjugate batch was maintained at 4 °C and dissolved in ddH2O.
Fourier transform Infra-red (FTIR) spectroscopy study
The FTIR spectroscopy approach determined the success of conjugation using the PerkinElmer, Spectrum 100. Investigations examined the chemical bonds and functional groups of AlPcS4Cl PS and AuNPs. The hydraulic pressing technique was used for compressing the mixture into the potassium-bromide (KBr) disc. The data was processed with the Spectrum 10.4.2 software, for wave number frequencies ranging between 4000 and 500 cm−1
Zeta potential and Dynamic light scattering (DLS)
The Zetasizer Nano ZS was used to perform DLS and Laser Doppler Velocimetry (LDV) to measure the zeta potential (charge) and size of AlPcS4Cl, AuNPs, AlPcS4Cl-AuNP conjugate, using the Malvern Panalytical, ZEN 3600. The AlPcS4Cl, AuNPs samples were prepared at concentrations of 35 mM and 50 ppm, respectively.
Melanoma CSC AlPcS4Cl-AuNP irradiation
The stem cell population was categorised into ten groups, seeded at 5 × 105 cells/ml and exposed to low intensity laser irradiation. Using a power output of 673.2 nm and fluency of 5 J/cm2, the total time of laser irradiation for each cell culture dish was calculated to be 10.40 s. The groups were as follows: CSCs only, CSCs + laser, CSCs + AuNPs, CSCs + 35 µm AlPcS4Cl, A375 Cells + 35 µM AlPcS4Cl-AuNP, CSCs + 35 µM AlPcS4Cl-AuNP, CSCs + AuNPs + laser, CSCs + 35 µM AlPcS4Cl + laser, A375 Cells + 35 µM AlPcS4Cl-AuNP + laser, CSCs + 35 µM AlPcS4Cl-AuNP + laser.
Annexin V FITC/PI flow cytometry
After being exposed to radiation for 24 h, the FITC Annexin V Apoptosis Detection Kit evaluated cell death. (BD PharmingenTM, 556547). The test identifies the necrotic or apoptotic modes of cell death process. In order to count the fluorescently tagged cells, the test used the Annexin V-FITC (fluoresces green) and the PI (fluoresces red) reagent dyes that were fluorescently labelled and then read on the C6 a flow cytometer. includes the parameters for emission and excitation.
Table 1. Excitation and emission parameters for fluorescence microscopy and flowcytometry.
Apoptotic proteins immunofluorescence qualitative analysis
The cells were cultured on coverslips and fixed with 4% paraformaldehyde before being blocked with BSA. The cells are rinsed with ice-cold PBS after each procedure. Cells mounted on glass slides were first directly labelled using primary fluorescent, FITC, Goat anti-Mouse Ab, counter stained with DAPI, and then observed using the Carl Zeiss Axio Observer Z1 with Zen software, according to the manufacturer’s methodology. (Live imaging microscope). includes parameters for excitation and emission.
Apoptotic proteins flow cytometry Quantitative Analysis
The expression of apoptotic proteins was examined 24 h post-PDT treatment. Cells were first labelled with primary mouse anti-human caspase 3 or p53 antibodies, and then counterstained with FITC goat anti-mouse (NovusBio, NB720-F-1mg) secondary fluorescent antibodies. Following the incubation period, the fluorescent probe on the attached antibody was detected using a C6 flow cytometer after the labelled cells were re-suspended in 300 ml of PBs. (BD Biosciences, BD ACCURI C6 PLUS). Caspase 3 or p53 expression was checked as a result; untreated cells served as a negative control.
Statistical analysis
Experiment sets were performed six times (n = 6) for each biochemical assay, and the results were averaged. For normal distributed data, one-way analysis of variances (ANOVA) was employed, while Dunnett’s test was used for non-normal distributed data. The difference between the control and experimental groups was considered statistically significant if the calculated values fell within the 95% confidence range (p < 0.05*, p < 0.01**, or p < 0.001***). Using Sigma Plot version 14.0, the graphs show the mean and standard deviation of biochemical tests
Results and discussion
Spectrophotometry (Ultraviolet-Visible)
The UV–vis of single molecules exhibited the AuNPs absorption spectrum of 520 nm, while AlPcS4Cl was 675 nm. The AlPcS4Cl-AuNP conjugation however, had an absorption spectrum of 525 nm, as seen in , which represents the product of conjugation of 50 ppm AuNPs to 35 µl AlPcS4Cl. When the wavelength of the AuNPs was compared to the wavelength of the PS conjugated AuNPs, an absorbance shift from 520 nm to 525 nm was found. AuNPs with diameters range of 2 to 100 nm contain a surface plasmon resonance (SPR) absorption band about 520 nm, as indicated by studies [Citation44]. A red shift can validate the conjugation of PS and AuNPs [Citation45,Citation46].
Figure 2. Ultraviolet–Visible spectroscopy (UV‐Vis) of AuNPs, AlPcS4Cl and AlPcS4Cl‐AuNP dispersed in ddH2O, read at the spectral region between 400 and 800 nm. The absorption peaks are at 675 nm for AlPcS4Cl and 520 nm for PEG‐AuNP‐COOH. Post conjugation, ALPcS4Cl‐PEG‐AuNP‐COOH indicates absorption peaks at 525 nm and 675 nm.
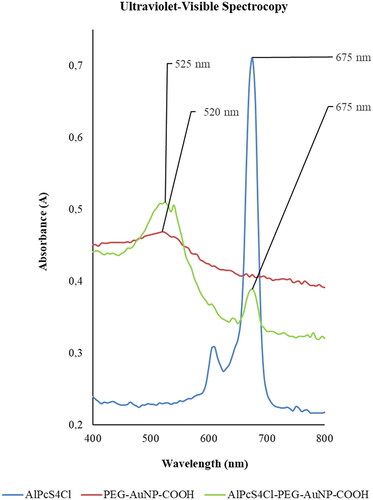
The wavelength absorption peak of phthalocyanines is located between 650 and 750 nm [Citation47]. The PS excitation wavelength in this investigation was 673.2 nm. When AuNP-PEG-AlPcS4Cl-Ab, irradiated at 673.2 nm, was used to treat lung CSC, Crous et al.'s (2019) study found significant PDT results [Citation48]. Phthalocyanine-conjugated AuNPs have the ability to boost the quantum of singlet oxygen generation, modulate photophysical characteristics, and transport drugs more effectively [Citation49,Citation50].
Gold nanoparticles absorb in the visible spectrum and their properties can be assessed using a variety of instruments and methods. The UV-Vis spectroscopy shows optical features, demonstrating that the collective electron oscillation in the AuNPs conduction band is in resonance with a particular wavelength of incident light. The information collected from this can be used to calculate the concentration and average size of the AuNPs. The functional groups can be identified using FTIR data, while the particle size and structural shape of AuNPs can be determined using Transmission Electron Microscopy (TEM) [Citation51]. Resonance Rayleigh Scattering (RSS) is another method that can be used for characterisation. This elastic scattering process occurs whenever the frequency of the incident light is close to an absorption band [Citation52].In PDT, one of the principal ways that light into tissue is attenuated is by scattering, limiting the penetration depth. Due to the substantial wavelength dependence of scattering intensity, longer excitation wavelengths are preferred to counteract this [Citation53].
Melanin absorbs light across the whole visible spectral range employed in PDT (400–750 nm) [Citation53].To avoid decreased PDT efficacy, photobleaching or depigmentation techniques are used [Citation54]. Amelanotic A375 cells were used for this study.
Fourier Transform Infra-red (FTIR) spectroscopy study
The qualitative molecular features were studied by means of Fourier transform infra-red spectroscopy (FTIR). Peptide bonds as well as ligand and absorption bond forms could be identified. depicts the outcomes. The conjugate was suspended in distilled deionised water (ddH20) and O-H stretching could be recognised at 3286.9 cm−1. The O-H bending was observed at 1363.1 – 1540 cm−1 due to carboxylic acid. C=C stretching was observed at 1540 cm−1. The polyether structure in PEG resulted in the C-O stretching, observed at 1038.8 cm−1. Due to the PS's structural elements, including its benzene rings and the PEG that was already present on the NP, the AlPcS4Cl-AuNP-PEG-COOH conjugate demonstrated C=C stretching at 1540 cm−1. The alkane methyl group’s translation into the C-H bending at 1471 cm−1 comes from the PS structure. The O-H bending from carboxylic acid was seen at 1430 cm−1, S=O stretching at 130 8.8 cm−1. The PS with sulphate functionalization was seen at 107.2 cm−1.
Zeta potential and Dynamic light scattering (DLS)
lists indirect measurements made by Dynamic Light Scatter (DLS) and Laser Doppler Velocimetry (LDV) of the conjugates’ hydrodynamic size and zeta potential. By knowing the surface state of the nanoparticles, the zeta potential can be utilised to forecast their long-term stability. A method for identifying and measuring the Brownian motion of macromolecules in solution brought on by solvent molecule bombardment is dynamic light scattering (or D). It is also known as quasi-elastic light scattering or photon correlation spectroscopy. Macromolecule motion is influenced by the size, temperature, and viscosity of the solvents [Citation55].
Table 2. DLS, Zeta potential and PDL Characterisation.
Table 3. Mean and standard deviation significance breakdown of Annexin V/PI outcomes.
The final AlPcS4Cl-AuNP PS conjugate measured at a Z-average diameter of 50.59 ± 34.92 nm. The conjugate gave out a charge of −17.0 ± 2.02 with a PDI of 0.015 when the zeta potential was measured. Due to the fact that nanostructures are defined as exhibiting a size range from 1 to 100 nm, the conjugate’s size can still be regarded as an active nanodrug-carrying structure [Citation56]. Stated PDI purity values were lower than 0.3, representative monodispersity and possibly the absence of aggregation. Typically, these samples comprised of single-sized, monodispersed particles [Citation57]. The zeta potential value of indicated that it had a slightly negative charge and was moderately stable. This implies that it should be passively assumed and localised more selectively within tumorous cells [Citation56,Citation58].
Annexin V FITC/PI flow cytometry
The presence of a CD133+ population in cutaneous tissues promotes the progression of melanoma. Additionally, by having a cytotoxic effect, CD133 monoclonal antibody can decrease the growth of FEMX-I melanoma cells and stop the spread of the disease. It was also proven that CD133 downregulation by shRNA can lessen the visibility of melanoma metastases in the lung and spinal cord. Furthermore, andrographolide can decrease the production of notch1-dependent CD133 in melanoma cells and lessen the activation of the MAPK signalling pathway, which can inhibit tumour growth, angiogenesis, and metastasis [Citation39].
Classifying and describing the consequences of ROS on PDT in various cell structures is a necessary step in the precise regulation and eradication of cancer cells and associated CSCs. Using a flow cytometry tool called an Annexin V FITC apoptosis detection kit, it was possible to distinguish between necrotic and apoptotic cells (). is a graphic representation of the analysed scatter-plots from the different control and experimental sets. Cell death by apoptosis has been connected to Annexin V, which binds to phosphatidylserine and transfers it to the plasma membrane [Citation59].
Figure 4. Annexin V/PI Cell death Analysis in Isolated A375 CSCs following PDT: the cells that received no treatment served as the control. The illustration clearly shows triggering of early apoptosis in A375 and CSCs. When compared to non-irradiated groups, this cell death mechanism enhanced in PS-AuNP conjugate groups irradiated with a laser at 673.2 nm with a fluency of 5 J/cm2 (laser irradiation at 10.40 s, calculated using a power output of 75mW semiconductor diode laser).
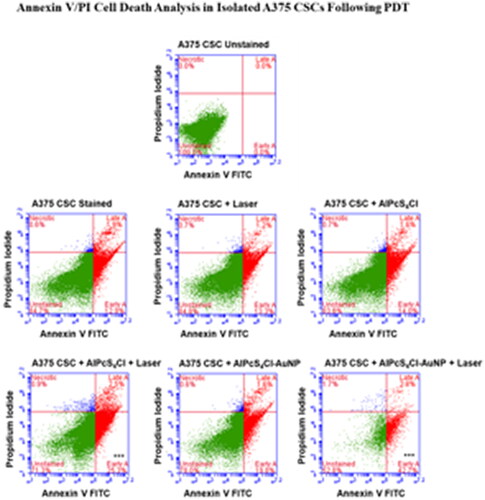
In order to distinguish necrotic cells, Propidium iodide (PI) was used. This is a nucleic acid intercalator that enables DNA by infiltrating porous cell membranes [Citation60]. Due to intact plasma membranes, the PI stain does not stain living cells or cells that have undergone early apoptosis. Using control cells that had not been altered or dyed, the cell population was created. While the results from the quadrant (early and late apoptosis, necrosis) were used to evaluate the level of cell death induced by the PS alone and their conjugation in the presence of light, untreated stained cells were utilised as the control to compare the results of viable cells. In comparison to the untreated control, all PDT control and treatment groups displayed considerable cell death through particular pathways. The method of cell death, however, varied between groups, which may have been caused by the activation of the necrotic or apoptotic pathway.
Irradiated cells, AlPcS4Cl cells, and AlPcS4Cl-AuNP cells had no substantial cell death, with viabilities of 84.8%, 83.8%, and 78.0%, respectively. Early apoptosis was significantly increased in cells treated with PDT utilising AlPcS4Cl and AlPcS4Cl-AuNP (***p < 0.001). The quantity of early apoptosis caused an increased from 25.3% to 42.7% when the cell death mechanism of PDT irradiation with just the AlPcS4Cl was reviewed against irradiation with the nanoconjugate PS respectively. This demonstrated that the chosen cell treatment mechanism of apoptosis was successfully attained while treating Melanoma CSCs. In a study by Crous et al. it was found that PDT-induced apoptosis was the favoured cell death method. The study also revealed that photoactivated AlPcS4Cl-AuNP-PEG-COOH and AlPcS4Cl-AuNP-PEG-CD133 were more efficient at eradicating lung CSCs and inducing apoptosis. A larger percentage of early apoptosis was witnessed when the photoactivated conjugates were compared to PDT alone (with sole AlPcS4Cl usage) [Citation57].
Apoptotic protein Immunofluorescence Qualitative Analysis
The occurrence of apoptotic proteins p53 and caspase 3 was detected qualitatively using immunofluorescence. Previous research found that PDT promoted apoptosis and necrosis; conversely, after different PS and cell types were employed, apoptosis was found to be the more common form of cell death [Citation61]. The caspase-dependent pathway was responsible for the increased percentages of early and late apoptotic cell populations in response to PDT utilising AlPcS4Cl and AlPcS4Cl-AuNP treatments. Immunofluorescence experiments assessed the expression of Caspase 3 and p53 apoptotic proteins for additional investigations for cell death mechanisms that result in morphological abnormalities, decrease in cellular ATP levels, suppression of cell growth, and release of LDH in earlier research by our group [Citation62]. A study also proved AuNP photothermal characteristics, which convert laser light into heat and promote cell destruction; nonetheless, the cellular responses of the populations investigated showed that PS-AuNP conjugation significantly improves PDT outcomes [Citation62]. Results from other studies, in contrast, showed that AlPcS4 conjugated with gold nanorods has significant anticancer effects. The gold nanorods acted as drug carriers for delivering AlPcS4 and also enhanced singlet oxygen generation and photothermal effects that directly caused cell death [Citation63]. Furthermore, increased ROS generation was demonstrated in a 2023 study. The therapeutic efficacy of the Pcs on the MCF-7 cells was improved in vitro when the Pcs were conjugated to silver and gold nanoparticles, and AuNP shown superior activity in comparison to those attached to silver nanoparticles [Citation64].
Immunofluorescence microscopy at 200x magnification show that A375 CSCs express the apoptotic proteins Caspase 3 and p53, respectively. Cells were indirectly labelled using FITC post PDT treatment and counterstained with DAPI (blue). Labelled apoptotic proteins were shown to fluoresce green, while the nucleus fluoresced blue. Untreated CSC was designated as a negative control, displaying no expression. When comparing AlPcS4Cl-AuNP PDT groups to AlPcS4Cl PDT groups, less cells can be seen in the photos. These photos show inhibited cell development and decreased proliferation. There were fewer cells after PDT, and detached, dead cells were lost during washing and staining methods. Caspases 3/7 cleave a number of vital cellular proteins during the execution stage of intrinsic and extrinsic apoptosis pathways [Citation65]. The tumour suppressor protein p53 is critical in cancer control, and PDT has been demonstrated to boost its expression, which contributes to cellular apoptosis [Citation66]. Chan et al. researched the aftermath of PDT on Human Umbilical Vein Endothelial Cells (HUVEC) and found that Ca2+ influx and NO generation boosted p53 expression. MMP is lost, and caspases, PAK2, and JNK are activated [Citation66].
Figure 5. 200x magnification Immunofluorescence microscopy of A375 CSCs expressing caspase 3 apoptotic proteins indirectly labelled with FITC (green) and counterstained with DAPI. (blue). untreated CSCs designated as a negative control, displaying no expression. Caspase 3 expression, on the other hand, is abundantly visible in the PS-AuNP conjugate + laser treated A375 and CSC groups.
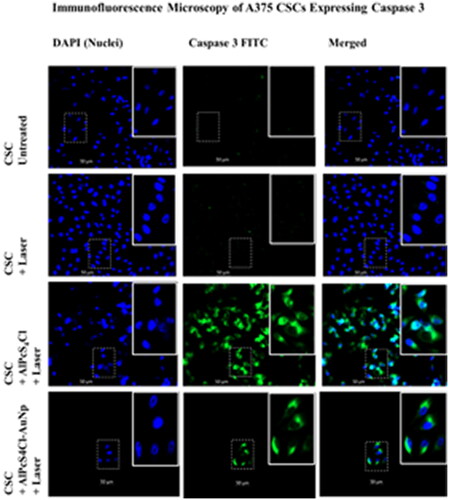
Figure 6. 200x magnification Immunofluorescence microscopy of A375 CSCs expressing p53 apoptotic proteins indirectly labelled with FITC (green) and counterstained with DAPI (blue). untreated CSCs designated as a negative control, displaying no expression. PS-AuNP conjugate + laser treated A375 and CSC groups show p53 expression.
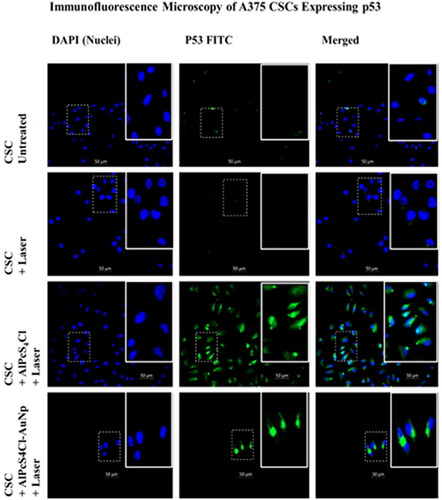
Apoptotic protein flow cytometry quantitative analysis
In order to determine the percentages of apoptotic proteins caspase 3 and p53 post PDT, flow cytometry was utilised as a quantitative technique in comparison to qualitative results in and . Cysteine-aspartic proteases known as caspases are crucial in the signalling of apoptosis [Citation67]. Results from previous studies revealed that localisation of the PS used are mainly in the mitochondria and lysosomes of the cells [Citation62]. The important "effector" caspases 3 and 7 are known for causing cell death when activated [Citation68]. Apoptosis is made possible via intrinsic mitochondrial or extrinsic death receptor pathways in many different cell types [Citation69]. If the apoptosis-activating function of the p53 protein is disrupted, tumour growth and chemoresistance may result. Through transcription-dependent and independent processes that work collectively to maintain the cell death pathway, p53 promotes apoptosis [Citation70].
The occurrence of the apoptotic proteins p53 and caspase 3 was quantified by means of flow cytometry. illustrates the expression of FITC-conjugated p53 and caspase 3. This deduces that apoptosis was the mode of cell death during PDT. The highest levels of p53 and caspase apoptotic proteins were found in cell groups that had undergone conjugate and laser irradiation treatment as compared to untreated cell groups, according to Figure 7's flow cytometry analysis of the PDT-treated A375 subpopulation. A platform for detecting caspase dependent apoptosis is provided by flow cytometry measurement of caspase 3 and p53 in A375 CSC. Caspase 3 activity was detected in AlPcS4Cl-treated A375 CSCs at a rate of 38.2% and in AlPcS4Cl-AuNP treated A375 CSCs at a rate of 40.2%. The p53 results showed that AlPcS4Cl-treated A375 CSCs had a percentage of 29.2 and AlPcS4Cl-AuNP treated A375 CSCs had a percentage of 34.4 ().
Figure 7. Post AlPcS4Cl and AlPcS4Cl-AuNP PDT, flow cytometry scatterplot analysis of p53 and caspase 3 apoptotic proteins on A375 total cell population and A375 CSC subpopulation. In AlPcS4Cl-AuNP PDT groups, both apoptotic proteins were found to be overexpressed.
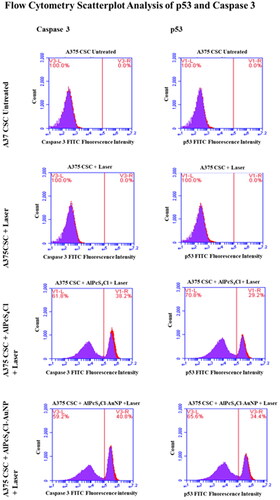
The only values for caspase 3 and p53 in CSCs exposed to laser irradiation were 5.6% and 4.9%, respectively. The fact that there weren’t many apoptotic cells among the viable cell population was probably to blame for this. Effector caspases, such as caspases 3 and 7, cleave numerous crucial cellular proteins in the course of the execution stage of extrinsic and intrinsic apoptotic pathways [Citation65].
Conclusion
Melanoma comprises of a high number of cells resistant to systemic treatment, as evidenced by the fact that medication has a limited effect in patients with advanced tumours. This proposes the presence of CSCs, which exhibit an important role in cancer recurrence and metastasis [Citation32,Citation71] The CSC theory depicts that tumours can arise from a single CSC, this is why all of them must be eradicated during therapy. Higher drug doses, individually or in conjunction with other anti-cancer drugs, may eliminate cancer cells but leave CSCs behind to renew or metastasise to distant organs and initiate the growth of other tumours[Citation72,Citation73].
The outcomes of the present study revealed the effects of gold nanoparticle-mediated photodynamic therapy on melanoma and its CSCs. Enhancing PSs with AuNPs clearly is beneficial to PDT. Biocompatible AuNPs with low intrinsic toxicity are excellent for biological usages. Because of their high surface area-to-volume ratio, AuNPs can be modified with a wide range of ligands and supplemented to PSs [Citation74]. Characterisation assays corroborated the successful conjugation of the AlPcS4Cl PS and AuNPs. The final PS drug conjugate’s DLS results revealed that the PDT nanoconjugate PS drug carrier was nanosized. The zeta potential showed monodispersity with a moderate size distribution, making the conjugate moderately stable. Compared to free AlPcS4Cl and their controls, treatment with the nanoconjugate induced a significant rise in cytotoxicity and apoptosis. A reduction in CSC proliferation and viability, denoted enhanced subcellular localisation of the conjugate. Once flow cytometry results were evaluated for modes of cell death, apoptosis was favoured as the prime mechanism of cell death which could be confirmed by Annexin V/PI results and the expression of caspase 3 and p53 apoptotic proteins. The study has revealed that PDT activates caspase-3 and p53 which leads to apoptotic pathways cleavage/activation and DNA fragmentation.
The study established the effect of AlPcS4Cl and AlPcS4Cl-AuNP on A375 CD133+ cells in PDT. Developing future approaches that could possibly then be useful to in vivo investigations or clinical trials treatments. Additionally, combining conventional therapy with PDT that is specifically focused towards CSCs can eliminate CSCs with little harm relative to non-cancerous cells by successfully inducing cell death in CSCs. Investigating these methodologies might result in brand-new treatment strategies that result in total tumour cell death and better cancer therapy management, ultimately enhancing prognosis and quality of life.
Author contributions
Bridgette Mkhobongo and Rahul Chandran contributed toward the conceptualization and design of the work. Bridgette Mkhobongo did all the investigation and result interpretation. Bridgette Mkhobongo and Rahul Chandran were involved in writing the original draft. Rahul Chandran and Heidi Abrahamse supervised the project and had substantial contributions to the review & editing of the article. Heidi Abrahamse was accountable for funding acquisition. All authors have given approval to the final version of the manuscript. All authors agree to be accountable for all aspects of the work
Acknowledgment
We acknowledge that the contents of this manuscript are a part of the Master dissertation by Ms Mkhobongo (First author), supervised by Dr Rahul Chandran and Prof Heidi Abrahamse submitted at the University of Johannesburg.
Disclosure statement
No potential conflict of interest was reported by the author(s).
Data availability statement
The authors confirm that the data supporting the results are available in this article. Additional data are available from the corresponding author, upon reasonable request.
Additional information
Funding
References
- Balch CM, Soong SJ, Gershenwald JE, et al. Prognostic factors analysis of 17,600 melanoma patients: validation of the American joint committee on cancer melanoma staging system. J Clin Oncol. 2001;19(16):3622–3634. doi: 10.1200/JCO.2001.19.16.3622.
- Ferlay J, Ervik M, Lam F, et al. Global cancer observatory: cancerToday, Lyon: International Agency for Research on Cancer; 2021, https://gco.iarc.fr/today.
- Schatton T, Frank MH. Cancer stem cells and human malignant melanoma. Pigment Cell Melanoma Res. 2008;21(1):39–55. doi: 10.1111/j.1755-148X.2007.00427.x.
- Gore ME, Larkin JMG. Challenges and opportunities for converting renal cell carcinoma into a chronic disease with targeted therapies. Br J Cancer. 2011;104(3):399–406. doi: 10.1038/sj.bjc.6606084.
- Diaz LA, Jr, Williams RT, Wu J, et al. The molecular evolution of acquired resistance to targeted EGFR blockade in colorectal cancers. Nature. 2012;486(7404):537–540. doi: 10.1038/nature11219.
- Dummer R, Hauschild A, Lindenblatt N, et al. Cutaneous melanoma: ESMO clinical practice guidelines for diagnosis, treatment and follow-up. Ann Oncol. 2015;26(Suppl (5):v126–v132. doi: 10.1093/annonc/mdv297.
- Kozovska Z, Gabrisova V, Kucerova L. Malignant melanoma: diagnosis, treatment and cancer stem cells. Neoplasma. 2016;63(4):510–517. PMID: 27268913. doi: 10.4149/neo_2016_403.
- Tang J-Q, Hou X-Y, Yang C-S, et al. Recent developments in nanomedicine for melanoma treatment. Int J Cancer. 2017;141(4):646–653. doi: 10.1002/ijc.30708.
- Naidoo C, Kruger CA, Abrahamse H. Photodynamic therapy for metastatic melanoma treatment, a review. Technol Cancer Res Treat. 2018;17:1533033818791795. doi: 10.1177/1533033818791795.
- Michot JM, Bigenwald C, Champiat S, et al. Immune-related adverse events with immune checkpoint blockade: a comprehensive review. Eur J Cancer. 2016;54:139–148. doi: 10.1016/j.ejca.2015.11.016.
- Meacham CE, Morrison SJ. Tumour heterogeneity and cancer cell plasticity. Nature. 2013;501(7467):328–337. doi: 10.1038/nature12624.
- Quintana E, Shackleton M, Sabel MS, et al. Efficient tumour formation by single human melanoma cells. Nature. 2008;456(7222):593–598. doi: 10.1038/nature07567.
- Takahashi K, Yamanaka S. Induction of pluripotent stem cells from mouse embryonic and adult fibroblast cultures by defined factors. Cell. 2006;126(4):663–676. doi: 10.1016/j.cell.2006.07.024.
- Barroca V, Lassalle B, Coureuil M, et al. Mouse differentiating spermatogonia can generate germinal stem cells in vivo. Nat Cell Biol. 2009;11(2):190–196. doi: 10.1038/ncb1826.
- Filipp FV, Li C, Boiko AD. CD271 is a molecular switch with divergent roles in melanoma and melanocyte development. Sci Rep. 2019;9(1):7696. doi: 10.1038/s41598-019-42773-y.
- Lengauer C, Kinzler KW, Vogelstein B. Genetic instabilities in human cancers. Nature. 1998;396(6712):643–649. doi: 10.1038/25292.
- Karbanová J, Lorico A, Bornhäuser M, et al. Prominin-1/CD133: lipid raft association, detergent resistance, and immunodetection. Stem Cells Transl Med. 2018;7(2):155–160. doi: 10.1002/sctm.17-0223.
- Glumac PM, LeBeau AM. The role of CD133 in cancer: a concise review. Clin Transl Med. 2018;7(1):e18. doi: 10.1186/s40169-018-01981.
- Toledo-Guzmán ME, Bigoni-Ordóñez GD, Hernández MI, et al. Cancer stem cell impact on clinical oncology. World J Stem Cells. 2018;10(12):183–195. doi: 10.4252/wjsc.v10.i12.183.
- Kessel D. Photodynamic therapy: a brief history. JCM. 2019;8(10):1581. doi: 10.3390/jcm8101581.
- Wang X, Ramamurthy G, Shirke AA, et al. Photodynamic therapy is an effective adjuvant therapy for image-guided surgery in prostate cancer. Cancer Res. 2020;80(2):156–162. doi: 10.1158/0008-5472.CAN-19-0201.
- Lan M, Zhao S, Liu W, et al. Photosensitizers for photodynamic therapy. Adv Healthc Mater. 2019;8(13):e1900132. doi: 10.1002/adhm.201900132.
- Ozog DM, Rkein AM, Fabi SG, et al. Photodynamic therapy: a clinical consensus guide. Dermatol Surg. 2016;42(7):804–827. doi: 10.1097/DSS.0000000000000800.
- Bichakjian CK, Olencki T, Aasi SZ, et al. Basal cell skin cancer, version 1.2016, NCCN clinical practice guidelines in oncology. J Natl Compr Canc Netw. 2016;14(5):574–597. doi: 10.6004/jnccn.2016.0065.
- Gilaberte Y, Aguilar M, Almagro M, et al. Spanish-Portuguese consensus statement on the use of daylight photodynamic therapy with methyl aminolevulinate in the treatment of actinic keratosis. Actas Dermo-Sifiliográficas (English Edition). 2015;106(8):623–631. doi: 10.1016/j.adengl.2015.07.019.
- Ravanat J, Douki T, Cadet J. Direct and indirect effects of UV radiation on DNA and its components. J Photochem Photobiol B. 2001;63(1-3):88–102. doi: 10.1016/s1011-1344(01)00206-8.
- Huis In 't Veld RV, Ritsma L, Kleinovink JW, et al. Photodynamic cancer therapy enhances accumulation of nanoparticles in tumor-associated myeloid cells. J Control Release. 2020;320:19–31. doi: 10.1016/j.jconrel.2019.12.052.
- Kong F, Zou H, Liu X, et al. MiR-7112-3p targets PERK to regulate the endoplasmic reticulum stress pathway and apoptosis induced by photodynamic therapy in colorectal cancer CX-1 cells. Photodiagnosis Photodyn Ther. 2020;29:101663. doi: 10.1016/j.pdpdt.2020.101663.
- Ethirajan M, Chen Y, Joshi P, et al. The role of porphyrin chemistry in tumor imaging and photodynamic therapy. Chem Soc Rev. 2011;40(1):340–362. doi: 10.1039/b915149b.
- Xu Y-K, Hwang S, Kim S, et al. Two orders of magnitude fluorescence enhancement of aluminum phthalocyanines by gold nanocubes: a remarkable improvement for cancer cell imaging and detection. ACS Appl Mater Interfaces. 2014;6(8):5619–5628. doi: 10.1021/am500106c.
- Hong EJ, Choi DG, Shim MS. Targeted and effective photodynamic therapy for cancer using functionalized nanomaterials. Acta Pharm Sin B. 2016;6(4):297–307. doi: 10.1016/j.apsb.2016.01.007.
- Calavia PG, Bruce G, Pérez-García L, et al. Photosensitiser-gold nanoparticle conjugates for photodynamic therapy of cancer. Photochem Photobiol Sci. 2018;17(11):1534–1552. doi: 10.1039/C8PP00271A.
- Mohammadi Z, Sazgarnia A, Rajabi O, et al. Comparative study of x-ray treatment and photodynamic therapy by using 5-aminolevulinic acid conjugated gold nanoparticles in a melanoma cell line. Artif Cells Nanomed Biotechnol. 2017;45(3):467–473. doi: 10.3109/21691401.2016.1167697.
- Oliveira CS, Turchiello R, Kowaltowski AJ, et al. Major determinants of photoinduced cell death: subcellular localization versus photosensitization efficiency. Free Radic Biol Med. 2011;51(4):824–833. doi: 10.1016/j.freeradbiomed.2011.05.023.
- Mfouo-Tynga I, El-Hussein A, Abdel-Harith M, et al. Photodynamic ability of silver nanoparticles in inducing cytotoxic effects in breast and lung cancer cell lines. IJN. 2014;9(1):3771–3780. doi: 10.2147/IJN.S63371
- Lee BWL, Ghode P, Ong DST. Redox regulation of cell state and fate. Redox Biol. 2019;25:101056. doi: 10.1016/j.redox.2018.11.014.
- Holmström KM, Finkel T. Cellular mechanisms and physiological consequences of redox-dependent signalling. Nat Rev Mol Cell Biol. 2014;15(6):411–421. doi: 10.1038/nrm3801.
- Li Z, Wang C, Deng H, et al. Robust photodynamic therapy using 5-ALA-Incorporated nanocomplexes cures metastatic melanoma through priming of CD4 + CD8+ double positive T cells. Adv Sci (Weinh). 2019;6(5):1802057. doi: 10.1002/advs.201802057.
- Yin Q, Shi X, Lan S, et al. Effect of melanoma stem cells on melanoma metastasis. Oncol Lett. 2021;22(1):566. doi: 10.3892/ol.2021.12827.
- Naumann SC, Roos WP, Jöst E, et al. Temozolomide- and fotemustine-Induced apoptosis in human malignant melanoma cells: response related to MGMT, MMR, DSBs, and P53. Br J Cancer. 2009;100(2):322–333. doi: 10.1038/sj.bjc.6604856.
- Beck D, Niessner H, Smalley KSM, et al. Vemurafenib potently induces endoplasmic reticulum stress-mediated apoptosis in BRAFV600E melanoma cells. Sci Signal. 2013;6(260):ra7. doi: 10.1126/scisignal.2003057.
- Mohammadalipour Z, Rahmati M, Khataee A, et al. Differential effects of N-TiO2 nanoparticle and its photo-activated form on autophagy and necroptosis in human melanoma A375 cells. J Cell Physiol. 2020;28:1–14. doi: 10.1002/jcp.29479.
- Majeed SA, Sekhosana KE, Tuhl A. Progress on phthalocyanine-conjugated Ag and Au nanoparticles: synthesis, characterization, and photo-physicochemical properties. Arab J Chem. 2020;13(12):8848–8887. doi: 10.1016/j.arabjc.2020.10.014.
- Chen X, Ye Q, Ma D, et al. Gold Nanoparticles-Pyrrolidinonyl metal phthalocyanine nanoconjugates: synthesis and photophysical properties. J Lumin. 2018;195:348–355. doi: 10.1016/j.jlumin.2017.11.047.
- Decker R, Oldenburg S. Covalent Bioconjugation of Antibodies to Carboxyl Terminated Nanoparticles PDF Protein Purification Nanoparticle https://www.scribd.com/document/511702433/Decker-R-Oldenburg-S-Covalent-Bioconjugation-of-Antibodies-to-Carboxyl-Terminated-Nanoparticles. (accessed 2021 -10 -25).
- Curry D, Cameron A, MacDonald B, et al. Adsorption of doxorubicin on citrate-capped gold nanoparticles: insights into engineering potent chemotherapeutic delivery systems. Nanoscale. 2015;7(46):19611–19619. doi: 10.1039/C5NR05826K.
- Ranyuk E, Cauchon N, Klarskov K, et al. Phthalocyanine–peptide conjugates: receptor-targeting bifunctional agents for imaging and photodynamic therapy. J Med Chem. 2013;56(4):1520–1534. doi: 10.1021/jm301311c.
- Crous A, Dhilip Kumar SS, Abrahamse H. Effect of dose responses of hydrophilic aluminium (III) phthalocyanine chloride tetrasulphonate based photosensitizer on lung cancer cells. J Photochem Photobiol B. 2019;194:96–106. doi: 10.1016/j.jphotobiol.2019.03.018.
- Goodman CM, McCusker CD, Yilmaz T, et al. Toxicity of gold nanoparticles functionalized with cationic and anionic side chains. Bioconjug Chem. 2004;15(4):897–900. doi: 10.1021/bc049951i.
- Murphy CJ, Gole AM, Stone JW, et al. Gold nanoparticles in biology: beyond toxicity to cellular imaging. Acc Chem Res. 2008;41(12):1721–1730. doi: 10.1021/ar800035u.
- Katas H, Moden NZ, Lim CS, et al. Biosynthesis and potential applications of silver and gold nanoparticles and their chitosan-based nanocomposites in nanomedicine. J Nanotechnol. 2018;2018:e4290705–13. doi: 10.1155/2018/4290705.
- Vasquez G, Hernández Y, Coello Y. Portable Low-Cost instrumentation for monitoring rayleigh scattering from chemical sensors based on metallic nanoparticles. Sci Rep. 2018;8(1):14903. doi: 10.1038/s41598-018-33271-8.
- Algorri JF, Ochoa M, Roldán-Varona P, et al. Light technology for efficient and effective photodynamic therapy: a critical review. Cancers. 2021;13(14):3484. doi: 10.3390/cancers13143484.
- Li X-Y, Tan L, Dong L-W, et al. Susceptibility and resistance mechanisms during photodynamic therapy of melanoma. Front Oncol. 2020;10:597. doi: 10.3389/fonc.2020.00597.
- Stetefeld J, McKenna SA, Patel TR. Dynamic light scattering: a practical guide and applications in biomedical sciences. Biophys Rev. 2016;8(4):409–427. doi: 10.1007/s12551-016-0218-6.
- Yu X, Trase I, Ren M, et al. Design of nanoparticle-based carriers for targeted drug delivery. J Nanomater. 2016;2016:e1087250–15. doi: 10.1155/2016/1087250.
- Danaei M, Dehghankhold M, Ataei S, et al. Impact of particle size and polydispersity index on the clinical applications of lipidic nanocarrier systems. Pharmaceutics. 2018;10(2):57. E57 doi: 10.3390/pharmaceutics10020057.
- Wu B, Zhao N. A targeted nanoprobe based on carbon nanotubes-natural biopolymer chitosan composites. Nanomaterials. 2016;6(11):216. doi: 10.3390/nano6110216.
- Demchenko A. Beyond annexin V: fluorescence response of cellular membranes to apoptosis. Cytotechnology. 2013;65(2):157–172. doi: 10.1007/s10616-012-9481-y.
- Rieger AM, Nelson KL, Konowalchuk JD, et al. Modified annexin V/propidium iodide apoptosis assay for accurate assessment of cell death. JoVE. 2011;(50):2597. doi: 10.3791/2597.
- Baldea I, Olteanu DE, Bolfa P, et al. Efficiency of photodynamic therapy on WM35 melanoma with synthetic porphyrins: role of chemical structure, intracellular targeting and antioxidant defense. J Photochem Photobiol B. 2015;151:142–152. doi: 10.1016/j.jphotobiol.2015.07.019.
- Mkhobongo B, Chandran R, Abrahamse H. In vitro photodynamic treatment modality for A375 melanoma cell line using a sulphonated aluminum phthalocyanine Chloride-Photosensitizer-Gold nanoparticle conjugate. Pharmaceutics. 2022;14(11):2474. doi: 10.3390/pharmaceutics14112474.
- Xin J, Wang S, Wang B, et al. AlPcS4-PDT for gastric cancer therapy using gold nanorod, cationic liposome, and pluronic® F127 nanomicellar drug carriers. Int J Nanomedicine. 2018;13:2017–2036. doi: 10.2147/IJN.S154054.
- Nene LC, Nyokong T. Enhancement of the in vitro anticancer photo-sonodynamic combination therapy activity of cationic thiazole-phthalocyanines using gold and silver nanoparticles. Journal of Photochemistry and Photobiology A: chemistry. 2023;435:114339. doi: 10.1016/j.jphotochem.2022.114339.
- Porter AG, Jänicke RU. Emerging roles of caspase-3 in apoptosis. Cell Death Differ. 1999;6(2):99–104. doi: 10.1038/sj.cdd.4400476.
- Chan W-H. Photodynamic treatment induces an apoptotic pathway involving calcium, nitric oxide, P53, P21-Activated kinase 2, and c-Jun N-Terminal kinase and inactivates survival signal in human umbilical vein endothelial cells. Int J Mol Sci. 2011;12(2):1041–1059. doi: 10.3390/ijms12021041.
- Earnshaw WC, Martins LM, Kaufmann SH. Mammalian caspases: structure, activation, substrates, and functions during apoptosis. Annu Rev Biochem. 1999;68(1):383–424. doi: 10.1146/annurev.biochem.68.1.383.
- Slee EA, Adrain C, Martin SJ. Executioner caspase- 3, -6, and -7 perform distinct, non-redundant roles during the demolition phase of apoptosis. J Biol Chem. 2001;276(10):7320–7326. doi: 10.1074/jbc.M008363200.
- Kroemer G, Galluzzi L, Brenner C. Mitochondrial membrane permeabilization in cell death. Physiol Rev. 2007;87(1):99–163. doi: 10.1152/physrev.00013.2006.
- Fridman JS, Lowe SW. Control of apoptosis by P53. Oncogene. 2003;22(56):9030–9040. doi: 10.1038/sj.onc.1207116.
- Simbulan-Rosenthal, C. M.; Dougherty, R.; Vakili, S.; Ferraro, A. M.; Kuo, L.-W.; Alobaidi, R.; Aljehane, L.; Gaur, A.; Sykora, P.; Glasgow, E.; Agarwal, S.; Rosenthal, D. S. CRISPR-Cas9 knockdown and induced expression of CD133 reveal essential roles in melanoma invasion and metastasis. Cancers 2019, 11 (10):1490. doi: 10.3390/cancers11101490.
- Simbulan-Rosenthal CM, Gaur A, Zhou H, et al. CD133 is associated with increased melanoma cell survival after multikinase inhibition. J Oncol. 2019;2019:e6486173. doi: 10.1155/2019/6486173.
- El-Khattouti A, Sheehan NT, Monico J, et al. CD133+ melanoma subpopulation acquired resistance to caffeic acid phenethyl ester-induced apoptosis is attributed to the elevated expression of ABCB5: significance for melanoma treatment. Cancer Letters. 2015;357(1):83–104. doi: 10.1016/j.canlet.2014.10.043.
- El-Khattouti A, Selimovic D, Haïkel Y, et al. Identification and analysis of CD133+ melanoma stem-like cells conferring resistance to taxol: an insight into the mechanisms of their resistance and response. Cancer Lett. 2014;343(1):123–133. doi: 10.1016/j.canlet.2013.09.024.