ABSTRACT
During the light curing process, the high transmittance of fused quartz leads to overcuring of the paste, thereby affecting its forming precision. To address this issue, aniline black dye was incorporated into the SiO2f/SiO2 ceramic paste to enhance its ultraviolet light absorption. The impact of modifying agent on paste viscosity and the influence of sintering temperature on ceramic microstructure and properties were investigated. The results demonstrated that by adding the black dye, the dimensional deviation rate of SiO2f/SiO2 composite ceramic decreased to less than 8.0%. The optimal sintering temperature was determined as 900°C, resulting in a maximum flexural strength of 21.9 MPa at room temperature and 25.5 MPa at 600°C respectively. However, with further increase in temperature, flexural strength declined due to cristobalite formation. Additionally, dielectric properties were examined and it was found that the main failure mechanisms of the fibers in the SiO2f/SiO2 composite ceramic were fiber fracture and fiber pull-out.
1. Introduction
The radome serves as a protective structure for antenna systems, shielding them from external environmental factors. It exhibits excellent electromagnetic wave penetration characteristics in terms of electrical properties and demonstrates outstanding mechanical properties against adverse external conditions. Quartz fiber-reinforced fused quartz ceramics are widely utilized as high temperature resistant radome materials due to their exceptional dielectric properties, remarkable thermal shock stability, minimal thermal expansion coefficient, and resistance to chemical erosion. These materials are extensively employed in aerospace radome/window products [Citation1]. However, traditional molding methods such as dry pressing molding and gel-casting molding fail to meet the industry’s requirements for shaping complex-structured fused quartz products. Consequently, novel molding processes have emerged with additive manufacturing (AM) technology leading the way. AM technology offers advantages including high efficiency in shaping complex ceramic structures, high forming precision and economy of raw materials [Citation2,Citation3]. As a result of these benefits, ceramic AM technology has gained significant traction across various fields including aerospace, military applications, and biotechnology.
The commonly used ceramic AM techniques include fused deposition modeling (FDM) [Citation4,Citation5], vat photopolymerization such as stereolithography (SLA) and digital light processing (DLP) [Citation6–8], and selective laser sintering (SLS) [Citation9–11]. SLA technology is a widely adopted AM method for ceramics due to its high precision, high strength and excellent surface quality. The ceramic light-curing slurry is primarily composed of ceramic particles, photosensitive resin, and a variety of additives. Complex-structured ceramic products are typically obtained through a series of processes including SLA, debinding, sintering and other post-processing steps. Currently, some studies have been conducted on SLA technology for various ceramics, including alumina [Citation12,Citation13], beta-tricalcium phosphate [Citation14], zirconia [Citation15], hydroxyapatite [Citation16], h-BN-SiO2 fused quartz [Citation17], tetragonal zirconia polycrystals [Citation7], carbon oxide silicon [Citation17] and polysilazane [Citation18]. These studies have investigated various aspects such as preparation methods, rheological properties, forming processes, and mechanical properties. However, there is limited research on fiber-reinforced ceramics using SLA technology despite its potential to enhance the strength of ceramic products significantly. This is primarily attributed to the incorporation of fibers, which often results in increased agglomeration and viscosity of the slurry, thereby posing a significant challenge for the successful implementation of SLA technology in fiber-reinforced ceramic production. Therefore, it is essential to explore the application of fiber-reinforced fused quartz ceramic products based on SLA technology. It is worth noting that in the process of light curing, the high light transmittance of fused quartz leads to overcuring of the paste, thus affecting its forming precision. The aforementioned issue necessitates prompt attention and resolution.
In this study, the light absorption rate of the SiO2f/SiO2 composite paste was enhanced and the overcuring phenomenon caused by light transmission was eliminated through the utilization of organic black dye. A SiO2f/SiO2 composite paste with high solid content and suitable viscosity was prepared, enabling the production of ceramic green bodies with high forming precision using SLA technology. Specifically, an investigation into fiber orientation distribution within the matrix after SLA was conducted. Furthermore, a study on the flexural strength, phase composition, and fiber fracture mechanism of SiO2f/SiO2 composite ceramics at different sintering temperatures was carried out.
2. Experimental procedure and characterization
2.1. Raw materials
The raw materials include fused quartz (SiO2) (d50 = 2.5 μm and d50 = 200 nm, Shanghai Chaowei Nano Technology Co., Ltd.), chopped quartz fiber (SiO2f, diameter = 8 ~ 10 μm, length = 3 mm, Henan Shenjiu New Material Co., Ltd.), stearic acid (SA, Sinopharm Chemical Reagent Co., Ltd.), 3-glycidoxypropyltrimethoxysilane (KH560) and Benzoin dimethyl ether (Akadin Reagent Co., Ltd.), diaryl iodonium salt (Nanjing Jiazhong Chemical Technology Co., Ltd.), diglycidyl 1,2-cyclohexanedicarboxylate (TTA21, Jiangsu Titel New Materials Technology Co., Ltd.), dipentaerythritol hexaacrylate (DPHA), 1,6-diacetoxyhexane (HDDA), and aniline black dye (Shanghai Boer Chemical Reagent Co., Ltd.).
2.2. Preparation of SiO2f/SiO2 composite paste
2.2.1. Pre-treatment of SiO2 and SiO2f
400 g of SiO2 powder with d50 = 2.5 μm and 100 g of SiO2 powder with d50 = 200 nm with no modifying agent served as control group (S1). The initial components were listed in . The mixtures from the four groups were added separately to 1 L of alcohol for ball milling and homogenization. The ratio of grinding balls to material was 2 to 1. The ball milling speed was 250 r/min and the ball milling time was 15 hours. The mixture was dried in a drying oven at 85°C and then passed 100 mesh sieves. The pre-treated mixed SiO2 ceramic powder was obtained finally.
Table 1. Nomenclature used to differentiate the slurry and raw materials composition.
An appropriate amount of SiO2f was placed in boiling water for 2 h and then moved in a Muffle furnace at 380°C for 2 h. The heat-treated SiO2f were gently kneaded until they achieved complete dispersion.
2.2.2. Preparation of hybrid photosensitive resin
TTA21, HDDA, DPHA, diaryl iodonium salt and benzoin dimethyl in a ratio of 57.5:31.3:10.4:0.6:0.2 were mixed in a drum ball mill at a speed of 260 r/min for 6 h. The ratio of grinding media to material was 2:1.
2.2.3. Preparation of SiO2f/SiO2 composite paste
The preparation steps of the SiO2f/SiO2 composite paste were as follows. 56 g of pre-treated mixed SiO2 ceramic powder, 18.5 g of hybrid photosensitive resin and 0.1 g of aniline black dye were manually stirred with a glass stick. The mixture was placed in a homogenizing mixer (ZYMC-700VS, Shenzhen Zye Science&Technology Co., Ltd., China) at the speed of 2500 r/min for 40 s to obtain the SiO2 paste. And then, 1.68 g of single dispersed SiO2f was added into the SiO2 paste at the speed of 2500 r/min for 60 s. Finally, the SiO2f/SiO2 composite paste with 60.9vol% solid content was obtained. shows the preparation process of the SiO2f/SiO2 composite paste.
2.3. Ceramic stereolithography, debinding and sintering
A self-designed SLA600 ceramic printing equipment with a laser wavelength of 355 nm was used in this experiment. The laser power was 145 mW. The scanning speed was 2500 mm/s. The scanning spacing was 0.06 μm and the layer thickness was 0.025 mm. shows the samples at different stages and the debinding curve. This debinding process for ceramic samples is based on our previous experience. In order to get the appropriate sintering temperature, the SiO2f/SiO2 composite ceramics were sintered at 800°C, 900°C, and 1000°C, respectively. The rate of temperature increase was 0.5°C/min and the holding time at maximum temperature was 2 hours. The radome picture can not be disclosed due to confidentiality agreements.
2.4. Characterization technique
The stacking density was determined with an apparent density meter (BT-200, Dandong Bai Te Instrument Co., Ltd., China). The particle size distribution was recorded utilizing a laser particle sizer (HELOS-RODOS, Sympatec in Germany). The contact angle was observed by using a contact angle meter (JC2000DM, Shanghai Zhongchen Digital Technology Equipment Co., Ltd.). The viscosity of the SiO2f/SiO2 composite paste was obtained utilizing a viscometer (MCR 302, Anton Paar in Austria) with a shear rate of 75/s. The shear rate was the same as the movement speed of the scraper in the SLA600. The number and distribution of fibers were studied by a super-depth-of-field microscope (VHX-950F, Keyence in Japan). X-ray diffraction (XRD) analysis of the samples was carried out under a Bruker D8 X-ray diffractometer with Cu Kα radiation to identify the crystalline products. The microstructure was studied with a scanning electron microscopy (SEM, JSM-7900F, Japan electron optics laboratory). The density and relative density were obtained by using the Archimedes principle. The flexural strength at room temperature was determined utilizing a universal tester (SANS2CMT5105, Shenzhen Xinsansi Co., Ltd., China), following the Chinese GB/T 4741–1999 standard. The flexural strength at 600°C was determined utilizing a universal tester (HSST-6003QP, Baowu Steel Group, China), following the Chinese GB/T 14,390–1993 standard. The cross-head-speed was 0.5 mm/min and the load cells were from Anhui Zhimin Electric Technology Co., LTD.
3. Results and discussion
3.1. Morphology and particle size analysis of raw materials
illustrates the morphology and particle size analysis of the pre-treatment materials. ) demonstrates that the mixed SiO2 ceramic powder is uniform and exhibits excellent sphericity. The surface of the fused quartz fiber is clean (refer to ). This facilitates the uniform mixing of ceramic powder and fibers. ) displays the stacking density of mixed SiO2 ceramic powders with different mass ratios (SiO2 ceramic powders with d50 = 2.5 μm and d50 = 200 nm). It can be seen that the stacking density of SiO2 ceramic powder (d50 = 2.5 μm) with a single particle size is higher than that of SiO2 ceramic powder with d50 = 200 nm. As the ratio of fine particles to coarse particles increases, the stacking density initially rises and then declines. This is primarily due to the effective filling of gaps among coarse particles by fine particles. However, when the gaps are filled, excessive aggregation of fine particle clusters leads to a decrease in stacking density. A high stacking density corresponds to a high solid content of the ceramic paste, while also producing a denser sintered body. The particle size distribution of the mixed powder under this ratio is shown in ). Therefore, the optimal ratio of coarse powder to fine powder is 8:2.
3.2. The influence of powder pre-treatment on contact angle and viscosity
) shows the contact angles between the four types of powders in and the hybrid photosensitive resin. The results indicate that the contact angle between untreated powder and the hybrid photosensitive resin is the highest, with a value of 43°. Other contact angles are smaller than that of S1. The contact angle of sample S4 is the smallest, reaching 34°. This indicates that the combination of SA and KH560 has the most optimal effect on surface modification of the mixed powder. The viscosity of ceramic pastes prepared from four types of powders in is shown in ). Similarly, the viscosity of paste S1 is measured at 2010.9 Pa·s, whereas the viscosities of pastes S2, S3, and S4 are all comparatively lower than that of S1. Notably, paste S4 exhibits the lowest viscosity among all pastes with a value as low as 54.8 Pa·s. This is because the surface of SiO2 particles modified by SA and KH560 contains more polar groups, which enhances the chemical compatibility between SiO2 particles and the hybrid photosensitive resin. This effect increases the lipophilicity of SiO2 particles and reduces the repulsion between SiO2 particles and the hybrid photosensitive resin. As a result, it lowers the contact angle and viscosity of ceramic slurry [Citation19]. The modification mechanism is illustrated in ). The modification method of S4 is thus deemed as the optimal approach.
3.3. Increasing the forming precision of the SLA process
illustrates the process of laser passing through the ceramic paste and the dimensional deviation rate (DDR) of green bodies prepared using SLA technology. The high transparency of fused quartz under ultraviolet light, as illustrated in ), leads to the occurrence of overcuring due to light transmission. The light absorption rate of the ceramic paste was enhanced by incorporating black dye in this study, which can effectively eliminate the phenomenon of overcuring. DDR is the ratio of the actual forming size to the standard model size (x × y× z = 40.0 mm × 4.2 mm × 3.2 mm). The x-axis is parallel to the direction of scraper movement, while the y-axis is perpendicular to the x-axis in a horizontal direction. The z-axis is parallel to the vertical direction. The forming size of the SiO2f/SiO2 composite paste without black dye was found to exceed the model size, as illustrated in ). Conversely, the forming size of the SiO2f/SiO2 composite paste with black dye exhibited a smaller dimension compared to the model size. It is crucial to note that the DDR of SiO2f/SiO2 composite paste without the addition of black dye is significantly higher than that of SiO2f/SiO2 composite slurry with black dye. During the SLA process, the hybrid photosensitive resin in the paste absorbed laser energy and initiated curing, accompanied by volume shrinkage. The penetrability of SiO2f/SiO2 composite paste without black dye is high and has a low light absorption rate, resulting in the phenomenon of overcuring. Conversely, the incorporation of black dye into SiO2f/SiO2 composite paste enhances laser energy absorption and reduces the DDR, thereby facilitating precise control over the forming process. It is worth noting that the worst forming precision is observed in the y-axis direction, possibly due to fiber orientation distribution.
3.4. The orientation distribution of fibers
The orientation distribution of fibers in the green body of the SiO2f/SiO2 composite is depicted in . To enhance clarity, yellow-line segments are utilized in ) to demarcate the observed fiber distribution captured by the super-depth-of-field microscope. The statistical analysis of the angle between the fibers and the direction of scraper movement is presented in ). Image Pro-Plus software was employed for angle measurement and statistical evaluation of fiber orientation distribution. Notably, a significant proportion (32%) of fibers exhibit an angle ranging from 0° to 3°, while over 70% have an angle within the range of 0° to 9°. Consequently, it can be inferred that there exists consistency between fiber orientation distribution and scraper movement direction, thereby inducing mechanical anisotropy. In practical applications, it is imperative to take into account the impact of mechanical anisotropy on load-bearing structures.
3.5. The properties of sintered SiO2f/SiO2 composite ceramic
The phase composition of the SiO2f/SiO2 composite ceramic at different sintering temperatures is illustrated in . At the sintering temperature of 800°C, a distinct amorphous diffraction peak is observed in the XRD pattern of the sample, indicating the absence of crystallization transformation in the fused quartz. Upon reaching a temperature of 900 degrees, an evident crystallization peak emerges above the amorphous diffraction peak, indicating the incipient crystallization of a small quantity of fused quartz. However, a clear emergence of crystalline peaks is observed in the XRD pattern when the sintering temperature reaches 1000°C, indicating the conversion of fused quartz to cristobalite. Nevertheless, it should be noted that the formation of cristobalite in fused quartz may potentially compromise its thermal shock resistance and stability [Citation20], thus necessitating caution to avoid such transformation.
Figure 7. The phase composition of the SiO2f/SiO2 composite ceramic at different sintering temperatures.
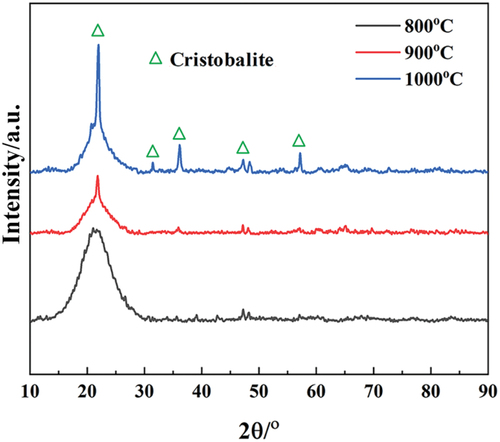
The effect of sintering temperature on the bulk density, open porosity, sintering shrinkage, and mechanical properties of SiO2f/SiO2 composite ceramics is illustrated in . As depicted in , an increase in the sintering temperature leads to a gradual enhancement in the bulk density of SiO2f/SiO2 composite ceramics, accompanied by a corresponding decrease in open porosity. Notably, higher density correlates with increased shrinkage (refer to ). The shrinkage ratio sequentially increases along the X-axis, Y-axis, and Z-axis directions due to the orientation distribution characteristics of fibers and layer-by-layer accumulation during the light-curing process [21]. The flexural strength of the SiO2f/SiO2 composite ceramics exhibits an initial increase followed by a decrease at room temperature and 600°C with increasing sintering temperature, reaching its maximum value at 900°C (refer to ). When the sintering temperature reaches 1000°C, cristobalite formation from fused quartz leads to a reduction in flexural strength [Citation20]. The flexural strength of the SiO2f/SiO2 composite ceramic attains its maximum value at a sintering temperature of 900°C.
Figure 8. The effect of sintering temperature on the bulk density, open porosity, sintering shrinkage, and mechanical properties of the SiO2f/SiO2 composite ceramics: (a) bulk density and open porosity, (b) sintering shrinkage, and (c) flexural strength.
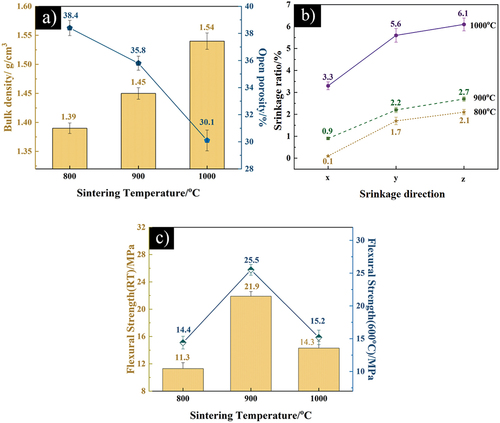
shows the effect of the sintering temperature on the dielectric properties of the SiO2f/SiO2 composite ceramic. As depicted in , both the dielectric constant (ε) and the dielectric loss tangent (tanδ) of the SiO2f/SiO2 composite ceramic gradually decrease with increasing frequency. According to the Debye dispersion equation [Citation21], it can be attributed to certain polar groups failing to respond adequately to changes in electric field at higher testing frequencies, resulting in a reduction in orientation polarization within the SiO2f/SiO2 composite ceramic structure. Furthermore, it is observed that as sintering temperature increases, both ε and tanδ of the SiO2f/SiO2 composite ceramic exhibit a gradual increase. This behavior can be explained by an increase in density and a decrease in porosity within the material, leading to enhanced ε and tanδ values. At a sintering temperature of 900°C and a frequency of 1 MHz, ε and tanδ values for the SiO2f/SiO2 composite ceramic are determined as 3.8 and 0.035 respectively, satisfying application requirements.
Figure 9. The effect of the sintering temperature on the dielectric properties of the SiO2f/SiO2 composite ceramics: (a) dielectric constant and (b) dielectric loss tangent.
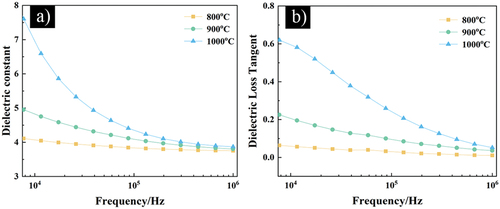
Overall, these findings highlight how variations in sintering temperature impact dielectric properties while emphasizing their significance for potential applications.
The fracture morphology of the SiO2f/SiO2 composite ceramic at different sintering temperatures is shown in . At a sintering temperature of 800°C (refer to ), the fracture surface of the SiO2f/SiO2 composite ceramic exhibits a fluffy appearance with no formation of sintering necks between particles. In this case, the flexural strength of the sample is low. As the sintering temperature increases to 900°C (refer to ) and 1000°C (refer to ), the fracture surface becomes denser. Notably, microcracks are observed on the fracture surface at a sintering temperature of 1000°C in ). This can be attributed to the crystalline transition of fused quartz within the SiO2f/SiO2 composite ceramic during sintering at this specific temperature, resulting in significant volume changes and subsequent microcrack formation [Citation20]. These microcracks significantly reduce the flexural strength of the SiO2f/SiO2 composite ceramic.
Figure 10. The fracture morphology of the SiO2f/SiO2 composite ceramic at different sintering temperatures: (a) 800°C, (b) 900°C, (c) 1000°C, (d)~(f) fiber morphology at 900°C.
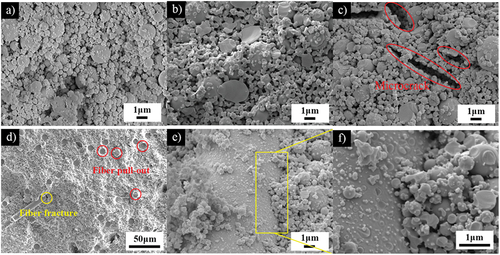
In , it can be seen that the fibers are uniformly distributed in the matrix and the fracture energy is consumed by fiber pulling out. In , effective bonding between fibers and SiO2 ceramic powders is achieved through successful sintering, leading to improved binding forces between fibers and matrix materials. These findings highlight how variations in sintering temperatures influence both morphological characteristics and mechanical properties of SiO2f/SiO2 composite ceramics.
4. Conclusions
In this study, we conducted a systematic investigation on the vat photopolymerization process of fused quartz ceramics. To address the issue of overcuring in highly transparent fused quartz paste, black dye was introduced into SiO2f/SiO2 composite ceramic paste. By enhancing the light absorption rate of the ceramic paste, the phenomenon of overcuring has been eliminated. Furthermore, the influence of powder modification on paste viscosity and sintering temperature on the microstructure and properties of SiO2f/SiO2 ceramics was investigated. The results demonstrated that the addition of black dye reduced the dimensional deviation rate of SiO2f/SiO2 green bodies to below 8.0%. The optimal sintering temperature was determined to be 900°C, resulting in a maximum flexural strength at room temperature of 21.9 MPa and at 600°Cof 25.5 MPa. The values of ε and tanδ at a frequency of 1 MHz were determined to be 3.8 and 0.035, respectively. However, as temperature increased further due to cristobalite formation, flexural strength decreased accordingly. The main failure mechanisms of the fibers in the SiO2f/SiO2 composite ceramic were fiber fracture and fiber pull-out.
Disclosure statement
No potential conflict of interest was reported by the author(s).
References
- Zhang XY, Li N, Lan T, et al. In-situ reaction synthesis of porous Si2N2O-Si3N4 multiphase ceramics with low dielectric constant via silica poly-hollow microspheres. Ceram Int. 2017;43(5):4235–4240. doi: 10.1016/j.ceramint.2016.12.058
- Chun S, Kim T, Ye B, et al. Penetrated surface characteristics of cement - mixed sand in powder bed 3D printing. J Asian Ceram Soc. 2022;10(2):306–313. doi: 10.1080/21870764.2021.2024734
- Mamatha S, Biswas P, Ramavath P, et al. Effect of parameters on 3D printing of alumina ceramics and evaluation of properties of sintered parts. J Asian Ceram Soc. 2021;9(3):858–864. doi: 10.1080/21870764.2021.1920159
- Man Y, Ding G, Xu L, et al. A review on porous ceramics with hierarchical pore structure by 3D printing-based combined route. J Asian Ceram Soc. 2021;9(4):1377–1389. doi: 10.1080/21870764.2021.1981571
- Guo W, Liu C, Bu W, et al. 3D printing of polylactic acid/boron nitride bone scaffolds: mechanical properties, biomineralization ability and cell responses. Ceram Int. 2023;49(15):25886–25898. doi: 10.1016/j.ceramint.2023.05.137
- Yang P, Sun Z, Huang S, et al. J Asian Ceram Soc. 2022;10(1):69–82. doi: 10.1080/21870764.2021.2009096
- Li Y, Wang S, Wang M, et al. J Asian Ceram Soc. 2021;9(2):727–732. doi: 10.1080/21870764.2021.1913912
- Yang P, Sun Z, Huang S, et al. J Asian Ceram Soc. 2022;10(1):69–82. doi: 10.1080/21870764.2021.2009096
- Ismail N, Saat N, Zaid M. Effect of soda lime silica glass doping on ZnO varistor ceramics: dry milling method. J Asian Ceram Soc. 2020;8(3):909–914. doi: 10.1080/21870764.2020.1793472
- Lu D, Cheng S, Zhang L, et al. Study on growth factors of SiC whisker in situ in SiCW/SiC composites based on selective laser sintering technology. Ceram Int. 2023;49(7):10673–10681. doi: 10.1016/j.ceramint.2022.11.257
- Ratsimba A, Zerrouki A, Tessier-Doyen N, et al. Densification behaviour and three-dimensional printing of Y2O3 ceramic powder by selective laser sintering. Ceram Int. 2021;47(6):7465–7474. doi: 10.1016/j.ceramint.2020.11.087
- Li W, Liu W, Qi F, et al. Determination of micro-mechanical properties of additive manufactured alumina ceramics by nanoindentation and scratching. Ceram Int. 2019;45(8):10612–10618. doi: 10.1016/j.ceramint.2019.02.128
- Lia Y, Hu Y, Cong W, et al. Additive manufacturing of alumina using laser engineered net shaping: effects of deposition variables. Ceram Int. 2017;43(10):7768–7775. doi: 10.1016/j.ceramint.2017.03.085
- Bae CJ, Halloran JW. Integrally cored ceramic mold fabricated by ceramic stereolithography. Int J Appl Ceram Technol. 2011;8(6):1255–1262. doi: 10.1111/j.1744-7402.2010.02568.x
- Sua CY, Wang JC, Chen DS, et al. Additive manufacturing of dental prosthesis using pristine and recycled zirconia solvent-based slurry stereolithography. Ceram Int. 2020;46(18):28701–28709. doi: 10.1016/j.ceramint.2020.08.030
- Chen QH, Zou B, Lai QG, et al. A study on biosafety of HAP ceramic prepared by SLA-3D printing technology directly. J Mech Behav Biomed Mater. 2019;98:327–335. doi: 10.1016/j.jmbbm.2019.06.031
- Tian Z, Yang Y, Wang Y, et al. Fabrication and properties of a high porosity h-BN–SiO2 ceramics fabricated by stereolithography-based 3D printing. Mater Lett. 2019;236:144–147. doi: 10.1016/j.matlet.2018.10.058
- Wang M, Xie C, He R, et al. Polymer-derived silicon nitride ceramics by digital light processing based additive manufacturing. J Am Ceram Soc. 2019;102(9):5117–5126. doi: 10.1111/jace.16389
- Song S, Gao Z, Xu H, et al. Rapid preparation and performance of degradable ceramic scaffolds based on stereolithography. J Asian Ceram Soc. 2022;10(1):58–68. doi: 10.1080/21870764.2021.2008102
- Tao X, Zhang LY, Ma XH, et al. Preparation of a flexible high emissivity coating on quartz fiber fabric for thermal protection. Ceram Int. 2017;43(16):14292–14300. doi: 10.1016/j.ceramint.2017.07.181
- Kindt JTK, Schmuttenmaer CA. Far-infrared dielectric properties of polar liquids probed by femtosecond terahertz pulse spectroscopy. J Phys Chem. 1996;100(24):10373–10379. doi: 10.1021/jp960141g