ABSTRACT
This research aimed to investigate the possible anti-amnesic effects of a combined therapy involving melatonin and H2S in a rat model displaying Depressive-Like Behaviors caused by Chronic Unpredictable Mild Stress (CUMS). Our study aims to assess the efficacy of this treatment in mitigating oxidative stress and neuroinflammation in the striatum and hippocampus. Furthermore, we seek to investigate its ability to restore BDNF levels within this specific rat model. The rats underwent a 4-week CUMS protocol and were administered sodium hydrosulfide (a H2S donor) at a dose of 5.6 μmol/100 g/day, as well as melatonin at a dose of 1 mg/100 g/day from the first day of the CUMS protocol. Following the CUMS exposure, the rats were assessed through various behavioral tests. Subsequently, the rats were euthanized after the behavioral tests, and blood samples were collected for corticosterone analysis. Oxidative stress (OS) markers, BDNF and TNF-α levels were analyzed in both the striatum and HP. Concurrently administering H2S and melatonin in a rat model of CUMS-induced depression demonstrates antidepressant-like effects. Furthermore, this combined treatment effectively prevents the development of learning and memory impairments associated with CUMS. Additionally, it reduces Malondialdehyde and nitric oxide levels, enhances glutathione peroxidase and superoxide dismutase activity in the striatum and HP, and mitigates CUMS-induced elevations in TNF-α levels in both brain regions. Significantly, long-term administration of this combination reverses the chronic stress-induced reduction of hippocampal BDNF levels. These findings suggest that the synergy between H2S and melatonin holds promise in alleviating cognitive impairments.
Introduction
Major depressive disorder (MDD) is a prevalent mood disorder with increasing numbers of patients globally each year, representing a significant public health challenge and an important economic burden [Citation1]. Although several studies investigate the molecular and circuits pathophysiology of depression, the precise pathogenic mechanisms are still unclear. Extensive research has identified a complex interplay of pathways underlying depression, including monoamines, stress response, neurotrophins, neurotransmission, mitochondrial dysfunction, genetics, inflammation, the opioid system, myelination, and the gut-brain axis [Citation2]. The monoamine theory posits deficiencies in serotonin, noradrenaline, and dopamine as underlying factors in MDD, as well as disturbances in other neurotransmitter systems such as GABA and glutamate [Citation2,Citation3]. Additionally, the neurotrophic hypothesis of depression highlights disrupted neurotrophic support, particularly involving BDNF, as a key mechanism in MDD-related synaptic alterations [Citation4]. This interconnection of molecular pathways underscores the multifaceted nature of MDD and emphasizes the need for a comprehensive understanding of its pathophysiology. The main symptoms of MDD include psychomotor retardation or agitation, diminished interest or pleasure, fatigue, and cognitive decline [Citation5]. This latter encompasses difficulties in concentration, decision-making, and everyday functioning [Citation6]. Notably, cognitive impairment in MDD predominantly affects learning and executive/working memory, which have been associated with reduced hippocampal dendritic spine density [Citation3,Citation1]. Multiple neurobiological mechanisms contribute to cognitive dysfunction in MDD, including neuroinflammatory pathways and domain-specific deficits in learning, memory, executive function, processing speed, and attention [Citation7]. These interconnected domains of depression, including cognitive impairment, anhedonia, and depressed mood, contribute to MDD-related functional disability, highlighting the significance of a comprehensive examination of cognitive decline in MDD. Both depression and cognitive dysfunctions involve oxidative stress (OS), neuroinflammation and BDNF-CREB signaling pathway [Citation8]. OS primarily results from the excessive buildup of ROS, leading to a variety of cellular abnormalities, including changes in lipid and protein function, DNA damage, and ultimately cell death [Citation9]. Stress conditions induce pro-inflammatory cytokines release through microglial activation and alter neuronal plasticity in the hippocampus (HP), increasing the risk of depression [Citation1,Citation10]. Chronic stress may induce depressive-like behaviors by dysregulation of the glutamate – GABA cycle in the HP and striatum [Citation11]. In addition, low levels of BDNF in the HP were associated with depression pathogenesis, while in the dorsal striatum, it indirectly affects learning and decision-making, altered in depression [Citation10]. The HP’s strong connection with the HPA axis renders it highly sensitive to stress and depression. Recent findings link MDD to both dysregulated inflammatory processes and oxidative stress. Sustained immune responses, as seen in infection or autoimmune disease, may contribute to depression; and mitochondrial dysfunction generates free radicals and oxidative stress, contributing to neuronal damage and inflammatory signaling in MDD [Citation2]. Additionally, the neurotrophic hypothesis of depression proposes reduced BDNF levels as a key factor in depression pathogenesis. Animal studies demonstrate stress-induced dysregulation of BDNF expression, particularly in the hippocampus, which is associated with depressive-like behaviors. Similarly, clinical studies show decreased BDNF expression in depressed individuals, while antidepressant treatment increases BDNF levels, suggesting a role for BDNF in neuronal plasticity and depression [Citation12].
Available antidepressant medications encompass various classes, including tricyclic antidepressants (TCAs), monoamine oxidase inhibitors (MAOIs), selective serotonin reuptake inhibitors (SSRIs), serotonin noradrenaline reuptake inhibitors (SNRIs), serotonin‐2 antagonists and reuptake inhibitors (SARIs), NE reuptake inhibitors (NDRIs), specific serotonergic antidepressants (NaSSAs), and multimodal antidepressants (MMAs) [Citation4]. However, despite the diverse options, and they are ineffective in a large subset of patients due to their delayed efficacy (up to two weeks) and side effects. Around 30% of patients diagnosed with MDD exhibit resistance to treatment [Citation10]. Consequently, there is a pressing need for more effective, faster-acting, and nonmonoaminergic-based antidepressant medications. In this study, we examine the potential therapeutic effects of melatonin (Mel) and hydrogen sulfide (H2S), in mitigating depressive-like behaviors, considering their well-established antioxidant properties and their interactive role in the brain, particularly in relation to the brain-derived neurotrophic factor (BDNF) signaling pathway [Citation13,Citation14]. Melatonin exhibits robust antioxidant, anti-inflammatory, and neuroprotective effects by easily crossing cell and nuclear membranes, where it exerts antioxidant actions against various aspects of oxidative stress (OS) [Citation15,Citation16]. On the other hand, H2S, a crucial signaling molecule, has demonstrated inhibitory effects on anxiety-like and depressive-like behaviors in preclinical studies, as well as stress-induced depressive-like behaviors [Citation17,Citation18]. These findings collectively suggest that targeting H2S signaling in the brain could be a promising therapeutic intervention for major depressive disorder (MDD), with melatonin potentially modulating this effect, likely through the BDNF pathway. Hence, our study seeks to examine how a combined treatment of melatonin and H2S may alleviate amnesia in a rat model exhibiting depressive-like behaviors induced by CUMS. We aim to assess the efficacy of this treatment in mitigating OS and neuroinflammation in the HP and striatum, as well as its potential to restore BDNF levels within this specific rat model. By elucidating the mechanisms underlying the therapeutic effects of melatonin and H2S, our study contributes to a deeper understanding of potential treatment strategies for MDD.
Material and methods
Animals
The present study involved adult male Wistar rats (weighing 280 to 300 g). These rats were accommodated in hygienic conditions within plexiglass cages, with four to five rats per cage. The environment was maintained at a temperature of 24°C, following a 12-hour light/dark cycle. The rats had unrestricted access to standard rodent food and water. All animal care and experimental procedures adhered to the guidelines outlined in the National Institutes of Health Guide for the Care and Use of Laboratory Animals. These procedures were also approved by the Animal Ethics Committee under the supervision of the nutrition team at the laboratory in Kenitra, Morocco (Nut-ITU/2022_15).
Chronic mild unpredictable stress procedure
The procedure for inducing CUMS followed a previously established protocol as described by Xu et al. [Citation19]. During an initial acclimatization phase of five weeks, all rats were systematically trained to consume a 1% sucrose solution until reaching a consistent level of sucrose preference. The CUMS paradigm incorporated a series of distinct stressors, including water and food deprivation (24 hrs), 3-hour period of daytime darkness, exposure to damp sawdust spanning 12 hours, 45° tilted cage (24 hrs), paired housing (24 hrs), cold exposure (5 mins), overnight illumination (12 hrs), tail clamp (60 sec), and restraint (3 hrs). Throughout a 4-week period, rats assigned to the CUMS group were subjected to a daily administration of two randomly selected stressors.
Animal grouping and treatment
After a five-week acclimation period, all experimental rats were randomly assigned to distinct groups, including the control group not exposed to CUMS (Sham, n = 8), and four separate CUMS groups, each comprising eight rats. Within these CUMS groups, three underwent treatment involving sodium hydrosulfide a H2S donor (56 μmol/kg/d, i.p.), melatonin (10 mg/kg/day, i.p.), or a combination of both (see ). Subsequent to these treatments, a battery of behavioral tests was administered. Following the completion of behavioral assessments, the rats were euthanized via decapitation. The entire bilateral HP and striatum tissues were promptly dissected from the brain to assess the levels of TNF-alpha, OS markers, and BDNF. A detailed schedule outlining the administration of drugs and the CUMS procedure are elaborated upon in .
Table 1. Animal grouping and treatment.
Behavioral tests
Animal tests for depression-like behavior
The Sucrose preference test (SPT) was executed following a previously outlined protocol [Citation20]. Each cage was equipped with a pair of bottles, each containing a 1% (w/v) sucrose solution, and this setup was maintained for a 24-hour period. Subsequently, one of the bottles was substituted with tap water for the subsequent 24 hours. To prevent any potential bias, the positions of the bottles were alternated every 4 hours during the adaptation phase. On the designated test day, the rats were subjected to a one-hour deprivation of both food and water. The rats were presented with two bottles: one containing a 100 ml 1% (w/v) sucrose solution and the other containing 100 ml tap water. After 4 hours, the volumes of consumed water and sucrose solution were quantified, enabling the calculation of sucrose preference as a percentage using the following formula: Sucrose preference (%) = Sucrose consumption/(Sucrose consumption + water consumption) x 100.
The forced swimming test (FST) was utilized to evaluate depressive-like states in rats. In order to acquaint all rats with the procedure, they initially participated in a 15-minute swim training session. After a 24-hour interval, each rat was placed individually within a transparent Plexiglas cylinder, which contained tap water at a temperature of 25°C. The behaviors of the rats were monitored for a duration of 5 minutes. The results of the FST were measured by determining the immobility time (%), representing the duration the rats spent floating on the water’s surface.
Learning and memory tests
The evaluation of reference memory and spatial learning was performed using the Morris water maze test (MWM), as previously outlined [Citation21]. The apparatus utilized for this test comprised a circular tank (120 cm in diameter × 40 cm in depth) containing tap water at 22°C, maintained at a height of 30 cm. The MWM setup was segmented into four distinct quadrants, with each quadrant being designated by a visual cue attached to the outer wall of the tank above the water surface. An obscured platform (11 × 14 cm) was positioned within the center of one of the quadrants, situated 2 cm beneath the water’s surface. This platform remained in a consistent position throughout all trial sessions. The testing procedure was divided into two segments: the training session encompassed five successive trials, with each trial commencing from a different starting point. During each trial, the rat was placed facing the wall and allotted 60 seconds to navigate and locate the concealed platform. Rats that managed to reach the platform were permitted to remain there for 10 seconds, while those unable to locate it within 60 seconds were gently guided to it. In a manner akin to the training session, the test session was recorded, and the time taken to reach the concealed platform was calculated. This particular metric reflects the duration it takes for a rat to locate the hidden platform submerged within the opaque water.
The novel object recognition test (NORT) was implemented to assess both short- and long-term recognition memory in rats, following the protocol described by Bikri et al. [Citation21]. This examination occurred within an open box measuring 50 cm × 50 cm x 40 cm and extended across three consecutive days: Day 1 for habituation, Day 2 for training with identical objects, and Day 3 for a long-term recognition memory (LTM) assessment. For short-term memory (STM), rats encountered a new object alongside a familiar one just two hours after the initial 5-minute training session. In contrast, the LTM evaluation took place 24 hours later, involving a familiar object and a novel object. To evaluate the STM and LTM index, the calculation involves determining the percentage of time spent exploring novel object/total exploration time of both objects.
Plasma corticosterone assay
A day after conducting the behavioral tests, the rats were euthanized by decapitation. Subsequently, blood samples were drawn from the jugular vein and then subjected to centrifugation for 10 minutes at 4°C (4000 rpm). For each biochemical analysis, distinct aliquots of frozen samples were prepared from each rat and kept chilled on ice for immediate use. Plasma corticosterone concentration was quantified using a commercially available rat ELISA kit as per the manufacturer’s instructions. The absorbance was recorded at 450 nm utilizing a microplate reader, and the corticosterone levels were expressed in ng/ml.
Preparation of homogenates
After the rats were euthanized, the HP and striatum were dissected from both hemispheres while placed on dry ice. Subsequently, the collected tissue were then homogenized in a cold lysis buffer (consisting of RIPA lysate solution and 1 mM PMSF) with the utilization of a Dounce homogenizer. The resultant homogenates underwent centrifugation at 14,000 g for a duration of 15 minutes, after which they were preserved at −80°C, awaiting subsequent analysis [Citation22].
Assessment of oxidative stress markers
Determination of superoxide dismutase (SOD) activity
SOD activity was quantified in brain homogenates using the procedure detailed in the methodology described by Beauchamp and Fridovich [Citation23]. The reduction of NBT to its blue formazan derivative by superoxide radicals was quantified through absorbance readings at 560 nm. The assessment kit employed in this analysis employed a tetrazolium salt to detect superoxide radicals that arise from the activity of hypoxanthine and xanthine oxidase. The content of SOD was computed and expressed as IU per mg of protein.
Glutathione peroxidase
Glutathione peroxidase (GPx) activity was measured in all groups using an enzyme immunoassay. All procedures were performed according to the kit manufacturer’s instructions (ab102530; Abcam, USA). GPx activity was expressed as pmol/min/mg protein.
Lipid peroxidation assay
The assessment of lipid peroxide generation in brain homogenates involved measuring the concentration of intracellular thiobarbituric acid reactive substances (TBARS), following the methodology established by Draper and Hadley [Citation24]. The procedure involved mixing the samples with 1 mL of 10% trichloroacetic acid and an additional 1 mL of 0.67% thiobarbituric acid solution. This mixture was subsequently subjected to a boiling water bath at a temperature of 90°C for a duration of 15 minutes. Following this, a solution of butanol (in a ratio of 2:1 v/v) was incorporated into the mixture, followed by centrifugation at 8000 g for a period of 5 minutes. Ultimately, the content of TBARS was quantified through absorbance measurements at 535 nm. The resulting lipid peroxidation values were expressed in nanomoles per milligram of protein.
Nitrite/Nitrate assay
In the present study, we utilized the Griess reaction-based diazotization approach, a recognized indirect method for assessing NO generation, to quantify nitrite concentrations in tissue homogenates, following the established protocol by Chao et al. [Citation25]. Briefly, a 500 µl tissue sample was introduced into a tube and treated with equal volumes of Griess reagent, comprising 1% sulfanilamide (1 ml) and 0.1% N-1-naphthylethylenediamine dihydrochloride (1 ml), both dissolved in 2.5% orthophosphoric acid. After a 30-minute incubation at room temperature, absorbance was measured at 540 nm. Using linear regression analysis with a standard calibration curve for sodium nitrite, we calculated nitrite concentrations in these homogenates. Tissue nitrite levels were expressed as μmol per gram of protein.
Assessment of TNF-alpha
The quantification of TNF-α levels was conducted utilizing a commercially available rat TNF-α ELISA kit (KRC3011; Invitrogen), following the guidelines provided by the manufacturer. The resulting TNF-α measurements within tissue homogenates were reported as picograms of the cytokine per gram of protein.
BDNF levels evaluation
A rat BDNF ELISA kit (ab213899; Abcam, USA) was employed to accurately quantify rat BDNF concentrations within brain homogenates. The quantified BDNF levels were then represented as picograms per milligram of protein.
Protein assay
The total protein concentration present in the samples was determined using the Bradford protein assay, in accordance with the guidelines provided by the manufacturer (E530 Biotechnology). This method involved utilizing bovine serum albumin (with a concentration range of 0–15 mg/ml) as a standard reference [Citation26].
Statistical analysis
Statistical analysis of all data was performed using the SPSS software (version 26). The findings were presented as the mean ± standard deviation (SD. To elucidate significant differences between the groups, both one-way and two-way analysis of variance (ANOVA) were implemented, followed by Tukey’s multiple comparison test. A significance level of P˂0.05 was utilized as the threshold for indicating statistical significance.
Results
Combined effect of H2S and melatonin on corticosterone levels in the rat CUMS model
illustrates the effect of H2S and melatonin on blood corticosterone levels. The results demonstrate that corticosterone concentrations were markedly higher in the CUMS, CUMS-Mel, and CUMS-H2S groups compared to the Sham group (p = .000; p = .000; p = .000, respectively). Additionally, the CUMS-Mel and CUMS-H2S groups exhibited a significant reduction in corticosterone concentrations (p = .002; p = .027, respectively) when contrasted with the CUMS control group. Furthermore, the combined treatment of H2S and melatonin displayed a noteworthy effect in restoring corticosterone levels to a normal state compared to the H2S (p = .000) or melatonin (p = .021) treatments.
Figure 2. Effects of H2S (5.6 μmol/100 g) and Melatonin (1 mg/100 g) on corticosterone blood levels of rats exposed to CUMS. Mean ± SD (n = 8). +++P < 0.001 vs Sham group; @P < 0.05, @@P < 0.01, @@@P < 0.001 vs CUMS group; $ p < 0.05, $$ p < 0.01, $$$P < 0.001 vs CUMS-H2S-Mel group; ANOVA/Post hoc (Tukey test).
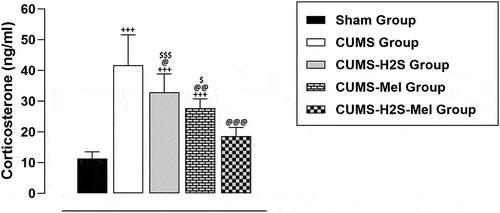
Antidepressant-like combined effects of H2S and melatonin in the rat CUMS model
The untreated CUMS group exhibited prolonged immobility time in the FST () and reduced sucrose preference in the SPT () in comparison to the Sham group (p = .000; p = .000, respectively). When compared to the CUMS control group, the administration of H2S to the CUMS+H2S group or melatonin to the CUMS+Mel group significantly decreased the immobility time (p = 0.000; p = 0.000, respectively) and substantially increased sucrose preference (p = 0.003; X%, p = 0.000, respectively). The combined treatment of H2S and melatonin displayed a remarkable effect in elevating the percentage of sucrose preference in comparison to the H2S treatment (p = 0.003). However, no significant differences were observed in immobility time (%) or sucrose preference (%) between the group that received the combined treatment and the group treated with melatonin (p = 0.385; p = 0.621, respectively).
Figure 3. Effects of H2S (5.6 μmol/100 g) and Melatonin (1 mg/100 g) on depressive-like behaviors of rats exposed to CUMS: (a) Percentage of sucrose preference in SPT; (b) Percentage of immobility time in the FST. Mean ± SD (n = 8). Mean ± SD (n = 8). +P < 0.05, ++P < 0.01, +++P < 0.001 vs Sham group; @@P < 0.01, @@@P < 0.001 vs CUMS group; $$$P < 0.001 vs CUMS-H2S-Mel group; ANOVA/Post hoc (Tukey test).
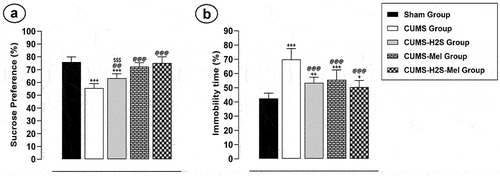
Effects of H2S and melatonin on CUMS-Induced learning and memory impairment in rats
The analysis of recognition index percentages during the STM recognition phases revealed no statistically significant differences (p = .245) among the various experimental groups (). Conversely, it is noteworthy that the untreated CUMS group exhibited a substantial decrease in the LTM index (), when compared to the Sham group (p = .001). Furthermore, the repeated administration of melatonin and H2S over a 4-week period did not yield statistically significant improvements in the LTM index (p = .3126; p = .051, respectively), as compared to the CUMS control group. However, it is important to note that the combined treatment with H2S and melatonin demonstrated a remarkable effect in ameliorating long-term recognition memory impairment when compared to the CUMS control group (p = 0.006). Nevertheless, no statistically significant differences were observed in the LTM index when comparing the group receiving the combined treatment to the groups treated with melatonin or H2S alone (p = .416; p = .917, respectively).
Figure 4. Effects of H2S (5.6 μmol/100 g) and Melatonin (1 mg/100 g) on CUMS-Induced Learning and Memory Impairment: (a) STM in the nor test; (b) LTM in the nor test; (c) Escape latency (sec) in the MWM test; (d) Platform quadrant time (%) in the MWM test. +++P < 0.001 vs Sham group; @P < 0.05, @@P < 0.01, @@@P < 0.001 vs CUMS group; $P < 0.05, $$$P < 0.001 vs CUMS-H2S-Mel group; ANOVA/Post hoc (Tukey test).
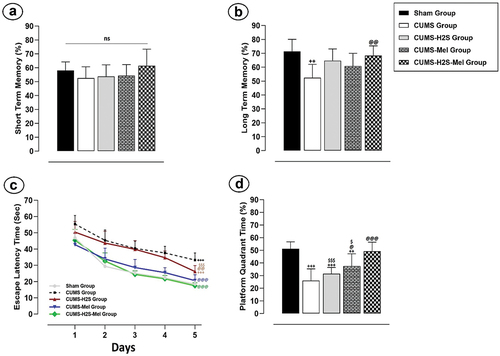
Additionally, this study employed the MWM test () to assess spatial memory capability. The trained rats in all groups demonstrated a notable decrease in ELT across all five days of learning trials. On the initial day of training, no statistically significant variations in ELT were observed among the experimental groups (p = .541). At the end of the training period, a highly significant distinction in ELT emerged, highlighting a substantial difference between the CUMS control group and the Sham group (p = .000). Furthermore, the results illuminated that the administration of H2S and Melatonin over a 4-week duration significantly reduced ELT in both the CUMS-H2S and CUMS-Mel groups when compared to the CUMS control group (p = .001, p = .000, respectively). Additionally, statistically significant differences in ELT were evident when contrasting the rats receiving the combined treatment with those treated with H2S alone (p = .000).
I n the same test, we measured the ability of reference memory by tracking the duration spent in the virtual platform quadrant (VPQ) (). During the probe trial session, the CUMS, CUMS-Mel, and CUMS-H2S groups all displayed significantly reduced time spent in the VPQ compared to the Sham group (p = .000; p = .008; p = .000, respectively). Notably, melatonin treatment had a substantial impact on the time spent VPQ when compared to the CUMS control group (p = .033). Furthermore, the combined administration of H2S and melatonin exhibited a significant effect in increasing the time spent in the VPQ, surpassing the effects of melatonin alone (p = .028) or H2S alone (p = .000) treatments.
Synergistic impact of H2S and melatonin on oxidative stress in the striatum and hippocampus of rats subjected to CUMS
In both the striatum and HP, there was a significant elevation in NO () and MDA () levels observed in the CUMS control group when compared to the Sham group (p = .000). The administration of H2S treatment effectively lowered the levels of NO and MDA in the striatum (p = .015; p = .001, respectively) and HP (p = .007; p = .000, respectively) within the CUMS+H2S group as compared to the CUMS control group. Similarly, the administration of Melatonin for 4 weeks resulted in a notable reduction in NO and MDA levels in the striatum (p = .001; p = .048, respectively) and HP (p = .001; p = .002, respectively) in the CUMS+Mel group when compared to the CUMS control group.
Figure 5. Effects of H2S (5.6 μmol/100 g) and Melatonin (1 mg/100 g) on Oxidative Stress Markers of rats exposed to CUMS. (a) NO concentration; (b) MDA level; (c) Catalase activity; (d) SOD activity. Mean ± SD (n = 7). +P < 0.05, ++P < 0.01, +++P < 0.001 vs Sham group; @P < 0.05, @@P < 0.01, @@@P < 0.001 vs CUMS group; $ p < 0.05, $$ p < 0.01, $$$P < 0.001 vs CUMS-H2S-Mel group; ANOVA/Post hoc (Tukey test).
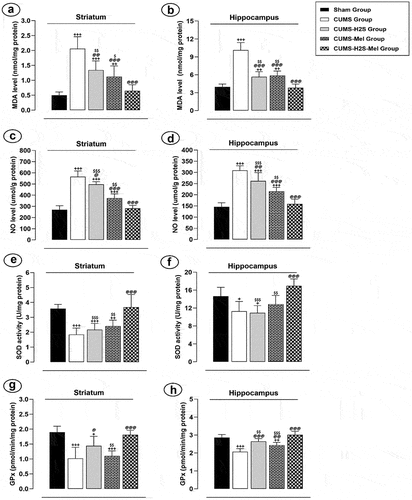
Furthermore, the activity of SOD () and GPx () exhibited significant decreases in both the striatum (p = .000; p = .000, respectively) and HP (p = .040; p = .000, respectively) within the CUMS control group when compared to the Sham group (p = .000). Moreover, following a 4-week administration of H2S, there was a significant increase in GPx activity observed in both the striatum (p = .022) and HP (p = .003). However, Melatonin treatment effectively enhanced the activity of this antioxidant enzyme only in the HP when compared to the CUMS control group”.
In addition, the combined administration of H2S and melatonin resulted in a significant reduction in MDA and NO levels in both the striatum and HP, while also significantly enhancing the activities of SOD and GPx in these brain regions. This effect was observed when compared to either melatonin alone or H2S alone treatments.
Combined effect of H2S and melatonin on TNF-alpha in the striatum and hippocampus of rats exposed to CUMS
The TNF-α level displayed a substantial increase in both the striatum () and HP () of the CUMS group (p = .000; p = .000, respectively), CUMS-Mel group (p = .000; p = .000, respectively), and CUMS-H2S group (p = .000; p = .000, respectively) when compared to the Sham group. The Melatonin treatment effectively reduced the TNF-α level in the striatum (p = .031) and HP (p = .000) of the CUMS-Mel group in comparison to the CUMS control group. Nevertheless, the administration of H2S treatment led to a reduction solely in the TNF-α level within the HP (p = .038) of the CUMS-H2S group in contrast to the CUMS control group. Furthermore, the combined administration of H2S and melatonin demonstrated a significant effect in restoring the TNF-α levels in the striatum and HP to normal, as compared to either melatonin alone (p = .003; p = .000, respectively) or H2S alone (p = .000; p = .000, respectively) treatments.
Figure 6. Effects of H2S (5.6 μmol/100 g) and Melatonin (1 mg/100 g) on TNF-α level of rats exposed to CUMS: (a) striatum (b) hippocampus. Mean ± SD (n = 7). +++P < 0.001 vs Sham group; @P < 0.05, @@@P < 0.001 vs CUMS group; $$ p < 0.01, $$$P < 0.001 vs CUMS-H2S-Mel group; ANOVA/Post hoc (Tukey test).
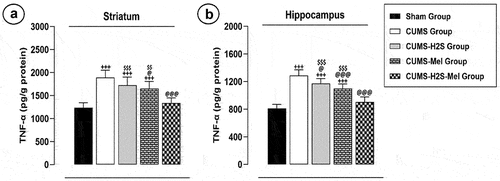
Combined effect of H2S and melatonin on BDNF levels in the striatum and hippocampus of CUMS-exposed rats
The exposure to CUMS for a duration of 4 weeks resulted in a significant reduction in hippocampal BDNF levels (). The BDNF level displayed a substantial decrease in the HP () of the CUMS group (p = 0.005), CUMS-Mel group (p = 0.006), and CUMS-H2S group (p = 0.02) when compared to the Sham group. However, the findings indicated no significant variance in hippocampal BDNF levels between the untreated CUMS group and the CUMS groups treated with melatonin or H2S. Nonetheless, the combined administration of H2S and melatonin notably increased hippocampal BDNF levels compared to H2S treatment (p = .012).
Figure 7. Effects of H2S (5.6 μmol/kg) and Melatonin (1 mg/100 g) on BDNF levels of rats exposed to CUMS: (a) striatum (b) hippocampus. Mean ± SD (n = 7). +P < 0.05, ++P < 0.01 vs Sham group; @P < 0.05 vs CUMS group; $ p < 0.05 vs CUMS-H2S-Mel group; ANOVA/Post hoc (Tukey test).
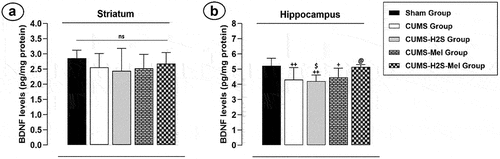
In contrast, the results of this study demonstrated a notable absence of statistically significant variation (p = .143) in BDNF levels within the striatum when comparing all groups (). This suggests that the CUMS exposition did not result in notable alterations in BDNF expression within this brain region across the experimental conditions. These results indicates a consistency in BDNF levels among the groups examined, implying that the interventions, including melatonin and H2S, did not lead to significant changes in BDNF concentrations within the striatum.
Discussion
In this study, we found that the concurrent administration of H2S and melatonin exhibited antidepressant-like effects in a CUMS-induced rat model of depression. Furthermore, this combination treatment notably prevented the development of learning and memory impairments induced by CUMS. The administration of H2S and melatonin effectively reduced oxidative stress markers while enhancing the antioxidant enzymes activity in the HP and striatum of the rat model. Additionally, this treatment effectively mitigated the CUMS-induced elevation of TNF-alpha in both the HP and striatum. Moreover, long-term administration of this combination markedly countered the chronic stress-induced reduction of Hippocampal BDNF. These findings suggest that the combination of H2S and melatonin may offer advantages in alleviating cognitive impairments. The anti-amnesic mechanisms likely involve restoring oxidative stress, reducing neuro-inflammation, and upregulating BDNF expression.
The CUMS model has been widely employed as an animal model to study depression, and previous research has revealed that most of the depressive effects induced by CUMS can be effectively reversed by antidepressant agents [Citation27]. In our current investigation, we observed an extended time of immobility during the FST and a significant reduction in sucrose preference in the SPT among the rats exposed to CUMS. These observations serve as indicators of a depressive-like state. Comparing these findings to the CUMS control group, we administered either H2S or melatonin to the CUMS-exposed groups, resulting in a noteworthy decrease in immobility time and a substantial increase in sucrose preference. H2S appears to exert its influence by enhancing the availability and function of neurotransmitters like serotonin [Citation28] or mitigating the effects of oxidative stress [Citation29], which are essential for mood regulation. The synergy between melatonin and H2S arises from their distinct yet complementary actions, addressing multiple facets of depressive behavior concurrently. Notably, when H2S and melatonin were co-administered, they exhibited a remarkable effect, significantly increasing the percentage of sucrose preference compared to the group treated with H2S alone. In line with our current results, a study conducted by Stefanovic et al. [Citation30] also reported melatonin’s potential to induce antidepressant-like effects in the FST, characterized by a reduction in immobility time. Moreover, in models involving CUMS, melatonin exhibited antidepressant-like properties, particularly at higher concentrations (1 mg/100 g). These effects were observed as melatonin effectively counteracted CUMS-induced depressive-like behaviors, such as reduced immobility duration in the FST and diminished sucrose intake [Citation31,Citation32]. Melatonin exerts its antidepressant efficacy through a range of intricate mechanisms [Citation33]. These include its pivotal role in modulating the HPA axis, effectively managing the balance of stress hormones. Additionally, melatonin displays its prowess in suppressing neuroinflammation and mitigating oxidative stress, both prominent factors in depressive conditions. It also engages in the upregulation of neurotrophic factors, such as BDNF, thereby fostering an environment conducive to neuronal growth and enhancing neuroplasticity. In addition to these actions, melatonin contributes to the elevation of neurotransmitter levels, notably serotonin.
Chronic stress is a well-established trigger of the adrenal cortex in animals, leading to the generation of corticosterone through a complex hormonal process [Citation34]. Corticosterone levels serve as a widely accepted biomarker of stress. An expanding body of research has demonstrated a clear link between elevated corticosterone levels and depressive-like behaviors, with consistent evidence that antidepressant-like interventions can decrease corticosterone concentrations [Citation20,Citation35]. The subsequent increase in corticosterone levels and the disruption of HPA axis due to CUMS exposure have gained increasing recognition in recent years [Citation36].
In the present study, we observed a significant increase in corticosterone levels in rats subjected to the CUMS model compared to those not exposed to CUMS. Chronic administration of either melatonin or H2S effectively reversed the elevation of corticosterone levels. These findings align with previous investigations [Citation37,Citation38], which similarly reported elevated corticosterone levels in response to stressors. Additionally, [Citation39] highlighted the potential of melatonin to reduce glucocorticoid levels by inhibiting adrenocorticotropin release. Indeed, our study marks the first exploration of H2S’s impact on blood corticosterone levels within the CUMS rat model. It is intriguing to consider that H2S has previously demonstrated the ability to influence the HPA axis, particularly by modulating the hypothalamic release of corticotropin-releasing factor [Citation40]. This intriguing mechanism may underlie the observed attenuation of corticosterone release induced by CUMS in our study. Furthermore, the present study results revealed that the combined treatment of H2S and melatonin displayed a noteworthy effect in restoring corticosterone levels to a normal state compared to the H2S or melatonin treatments. We propose that the combined treatment of H2S and melatonin is likely to have a substantial impact on normalizing corticosterone levels in rats exposed to CUMS. This expected outcome may arise from the complementary actions of these compounds: H2S, with its recognized anti-inflammatory and antioxidant properties, may mitigate oxidative stress induced by chronic stressors, while melatonin’s ability to alleviate depressive symptoms could further attenuate the stress response. When administered together, these two substances are expected to synergistically enhance their therapeutic effects on corticosterone levels compared to individual H2S or melatonin treatments.
An imbalance between the generation of free radicals and the functioning of antioxidants leads to elevated levels of oxidative stress [Citation41]. Numerous studies have increasingly highlighted the brain’s vulnerability to oxidative harm, underscoring the significant role that oxidative stress plays in depression induced by CUMS [Citation35,Citation42]. Long-term exposure to chronic stress can exert a substantial influence on the functioning of the HPA axis, leading to heightened production of ROS [Citation43]. Furthermore, the available evidence points to a significant connection between depressive disorders and neuroinflammation [Citation44]. In response to chronic stress, the body’s ultimate reaction involves the immune system, which initiates the release of inflammatory cytokines. These mediators have the ability to impact neural activity within the brain [Citation45]. In this context, numerous studies have demonstrated the critical involvement of neuroinflammation and oxidative stress in the development and complications associated with depression [Citation46]. This notion is corroborated by the elevated levels of MDA and reduced levels of SOD and ascorbic acid observed in individuals diagnosed with depression [Citation47]. Moreover, individuals experiencing depression also display heightened concentrations of proinflammatory cytokines like Interleukin-6 and TNF-α [Citation48]. In our recent investigation, we observed clear signs of oxidative stress in the striatum and HP of the CUMS group when compared to the Sham group. These signs were evident through a decrease in the activity of antioxidant enzymes such as SOD and GPx. Additionally, there were significant increases in both MDA and NO levels. Moreover, the TNF-α level displayed a substantial increase in both the striatum and HP of the CUMS group. In line with our results, a previous study utilizing an animal model of CUMS reported a decrease in catalase and SOD activity in the striatum and HP, along with elevated levels of NO and MDA in the HP and striatum [Citation20,Citation49]. In our study, it became evident that the administration of Melatonin and H2S had a significant mitigating effect on oxidative stress in the HP and striatum of the CUMS rat model. This was accomplished by restoring catalase levels and suppressing the NO and MDA levels induced by CUMS. Additionally, this treatment resulted in a reduction of neuroinflammation, as evidenced by the restoration of TNF-alpha levels in these brain regions. Melatonin is recognized for its significant antioxidant properties, operating at multiple levels within the body. Patients suffering from depression often exhibit reduced melatonin levels [Citation50]. Research has indicated that melatonin treatment effectively mitigates neuronal apoptosis, maintains energy balance and curbs neuroinflammation [Citation15]. Moreover, when administered orally, melatonin has been shown to diminish nuclear factor-κB, NO, and proinflammatory cytokines in the rat brain [Citation51]. On the other hand, H2S has demonstrated therapeutic promise in mitigating the detrimental impacts of hippocampal oxidative stress induced by CUMS [Citation52]. Its antioxidant functions are linked to the regulation of enzyme activities, including glutathione and SOD. Additionally, H2S exhibits anti-inflammatory actions through diverse signaling pathways. It possesses the capacity to inhibit crucial pro-inflammatory transcription factors and intracellular signaling molecules, such as phosphodiesterases and NF-κB. Furthermore, it suppresses the generations of inflammatory cytokines and prevents the adhesion of endothelial cells and leukocytes [Citation53]. In this research, the concurrent application of H2S and melatonin yielded noteworthy reductions in the levels of MDA, NO, and TNF-alpha within both the striatum and HP. Additionally, it significantly boosted the activities of SOD and GPx in these specific brain regions. This observed effect stands in contrast to the outcomes achieved with either melatonin or H2S administered individually. We suggest that, melatonin and H2S when used in combination complement each other’s antioxidant and anti-inflammatory effects in the CUMS-induced rat model of depression. They target different aspects of the oxidative stress and inflammation pathways, resulting in a synergistic effect. This means that the combination treatment is more effective at reducing oxidative stress markers and inflammatory cytokines while simultaneously enhancing the activities of antioxidant enzymes compared to using melatonin or H2S alone. In essence, their combined action creates a more robust and comprehensive defense against oxidative stress and inflammation in the brain, making the combination treatment more significant than either compound alone.
Many individuals experiencing depression also grapple with cognitive deficits [Citation54,Citation55]. The underlying mechanisms behind cognitive impairment during depressive episodes are a subject of extensive speculation [Citation56]. These symptoms may be linked to elevated levels of oxidative stress and inflammation, aligning with the emerging theory that suggests depression could be regarded as an inflammatory disorder. The results of this experiment revealed that CUMS had a significant negative impact on various cognitive functions in rats. More specifically, it led to notable impairments in long-term recognition memory as well as spatial and reference memory. Consistent with our findings, an experimental study demonstrated substantial impairment in the cognitive capabilities of mice exposed to CUMS, affecting various aspects of memory, including short-term spatial memory, long-term spatial memory, recognition memory, and reference memory [Citation57]. It has been revealed that prolonged stress, which activates the HPA axis, triggers an increased synthesis of glucocorticoids. This elevated secretion of glucocorticoids can result in harmful effects on neural circuits located in regions heavily implicated in the processes of cognitive functions [Citation58]. In the current study, the results unveiled a heightened effectiveness of melatonin and H2S in guarding against the harmful consequences of CUMS-induced spatial memory impairment in rats. Additionally, the findings demonstrated a noteworthy disparity between melatonin and H2S in their ability to shield rats from the detrimental effects of CUMS-induced reference memory impairment. Furthermore, the simultaneous administration of H2S and melatonin displayed a substantial impact on preserving long-term recognition memory, spatial memory, and reference memory, surpassing the effects observed with either melatonin or H2S treatment alone. In this context, H2S has been found to facilitate long-term potentiation induction and enhance synaptic transmission [Citation59]. Dysregulation of synaptic plasticity is widely recognized as a contributing factor to the pathophysiology of depression [Citation60]. Moreover, research has established a connection between chronic stress-induced depression and the degradation of H2S production in the HP [Citation61]. Previous comprehensive studies have consistently highlighted H2S’s neuroprotective properties, including its role in preventing neuronal damage and enhancing cognitive function [Citation62]. On the other hand, melatonin has also shown promise in improving spatial learning and memory impairments. These favorable outcomes have been strongly linked to a reduction in neuroinflammation and neuroapoptosis, as reported by Fang et al. [Citation63]. Additionally, another study by Dera et al. [Citation64] suggests that melatonin may have a potential protective effect against hippocampal damage and deficits in memory function.
Further research has shown that concurrent administration of melatonin exerts an anti-amnesic effect and restores the reduced expression of Myelin Basic Protein by increasing BDNF expression in the hippocampal formation of mice [Citation13]. BDNF, a pivotal protein involved in the plastic changes linked with learning and memory, has been suggested as a fundamental neurobiological foundation for the process of memory consolidation [Citation65]. Moreover, chronic stress, a factor linked to an increased risk of cognitive impairment [Citation66], has been found to be correlated with reduced BDNF expression in the HP [Citation35]. In the present study, the exposure to CUMS for a duration of 4 weeks resulted in a significant reduction in hippocampal BDNF levels. However, the findings revealed that melatonin or H2S alone did not significantly affect BDNF levels in the HP in the context of CUMS. However, when melatonin and H2S were administered together, there was a notable increase in BDNF levels. This suggests that there may be an interaction or synergistic effect between melatonin and H2S in promoting BDNF production in the HP under conditions of CUMS. Melatonin is known to upregulate BDNF expression via its receptors and downstream signaling pathways, promoting neuronal survival and growth [Citation67]. Similarly, H2S has been reported to enhance BDNF synthesis and release, contributing to its neuroprotective properties. We propose that the combination of melatonin and H2S may serve as a significant neuroprotective agent against the cognitive impairments induced by depression resulting from CUMS. This neuroprotective potential is likely mediated through their ability to regulate BDNF. Moreover, melatonin supplementation has been shown to offer protective effects on various brain regions by safeguarding brain cells from damage, boosting BDNF expression, and potentially enhancing learning and memory functions [Citation68]. Consistent with the findings of our study, a prior research study demonstrated that H2S administration improved spatial memory deficits by increasing BDNF expression levels and promoting neurogenesis in the HP [Citation14].
Conclusion
These study findings suggest the potential applicability of this combination therapy in clinical practice for managing depressive symptoms and associated cognitive declines. Given that depressive disorders often co-occur with cognitive deficits, our study’s results highlight the importance of considering treatment strategies that address both mood-related symptoms and cognitive impairments simultaneously. The ability of the combination treatment to prevent the development of cognitive functions impairments induced by CUMS further underscores its potential clinical value. By targeting multiple facets of depression, including mood regulation, neuroinflammation, oxidative stress, and neurotrophic support, the combination of H2S and melatonin presents a comprehensive approach that may lead to improved patient outcomes and quality of life. Future clinical studies are warranted to validate these preclinical findings and to assess the safety and efficacy of this combination therapy in human subjects [Citation69].
Author contributions
BS and AY, contributed to design of the investigation. BS, EM, and BD conducted the experiments. BS and AY carried out the statistical analysis. BS, FN, EM, BD and AY prepared the manuscript. Each author contributed to the manuscript and has approved the final version for submission.
Disclosure statement
No potential conflict of interest was reported by the author(s).
Data availability statement
The raw data supporting the conclusions of this article will be made available by the authors, without undue reservation.
Additional information
Funding
References
- Worthen RJ, Garzon Zighelboim SS, Torres Jaramillo CS, et al. Anti-inflammatory IL-10 administration rescues depression-associated learning and memory deficits in mice. J Neuroinflammation. 2020;17(1):246.
- Fries GR, Saldana VA, Finnstein J, et al. Molecular pathways of major depressive disorder converge on the synapse. Mol Psychiatry. 2023;28(1):284–297.
- Tartt AN, B MM, Hen R, et al. Dysregulation of adult hippocampal neuroplasticity in major depression: pathogenesis and therapeutic implications. Mol Psychiatry. 2022;27:2689–2699. doi: 10.1038/s41380-022-01520-y
- Tian H, Hu Z, Xu J, et al. The molecular pathophysiology of depression and the new therapeutics. MedComm. 2022 21;3(3):e156. doi: 10.1002/mco2.156
- Park SC, Kim D. The centrality of depression and anxiety symptoms in major depressive disorder determined using a network analysis. J Affective Disorders. 2020;271:19–26. doi: 10.1016/j.jad.2020.03.078
- Kriesche D, Woll CFJ, Tschentscher N, et al. Neurocognitive deficits in depression: a systematic review of cognitive impairment in the acute and remitted state. Eur Arch Psychiatry Clin Neurosci. 2023;273(5):1105–1128.
- Pan Z, Park C, Brietzke E, et al. Cognitive impairment in major depressive disorder. CNS Spectr. 2019;24(1):22–29.
- Zhao YT, Zhang L, Yin H, et al. Hydroxytyrosol alleviates oxidative stress and neuroinflammation and enhances hippocampal neurotrophic signaling to improve stress-induced depressive behaviors in mice. Food Funct. 2021;12(12):5478–5487.
- Tirichen H, Yaigoub H, Xu W, et al. Mitochondrial reactive oxygen species and their contribution in chronic kidney disease progression through oxidative stress. Front physiol. 2021;12:398. doi: 10.3389/fphys.2021.627837
- Miyanishi H, Nitta A. A role of BDNF in the depression pathogenesis and a potential target as antidepressant: the modulator of stress sensitivity “Shati/Nat8l-BDNF system” in the Dorsal Striatum. Pharmaceuticals. 2021;14(9):889. doi: 10.3390/ph14090889
- Shaohua X, Yiyun L, Juncai P, et al. Chronic stress in a rat model of depression disturbs the Glutamine–Glutamate–GABA cycle in the Striatum, Hippocampus, and Cerebellum. Neuropsychiatr Dis Treat. 2021;16:557–570. doi: 10.2147/NDT.S245282
- Lee BH, Kim YK. The roles of BDNF in the pathophysiology of major depression and in antidepressant treatment. Psychiatry Investigation. 2010 Dec;7(4):231–235. 2010 Nov 23. Epub: 10.4306/pi.2010.7.4.231
- Chen BH, Park JH, Lee TK, et al. Melatonin attenuates scopolamine-induced cognitive impairment via protecting against demyelination through BDNF-TrkB signaling in the mouse dentate gyrus. Chem Biol Interact. 2018;285(285):8–13.
- Mohseni F, Bagheri F, Rafaiee R, et al. Hydrogen sulfide improves spatial memory impairment via increases of BDNF expression and hippocampal neurogenesis following early postnatal alcohol exposure. Physiol Behav. 2020;215:112784. doi: 10.1016/j.physbeh.2019.112784
- Rehman SU, Ikram M, Ullah N, et al. Neurological enhancement effects of melatonin against brain injury-induced oxidative stress, neuroinflammation, and neurodegeneration via AMPK/CREB signaling. Cells. 2019;8(7):760. doi: 10.3390/cells8070760
- Shokri-Mashhadi N, Darand M, Rouhani MH, et al. Effects of melatonin supplementation on BDNF concentrations and depression: A systematic review and meta-analysis of randomized controlled trials. Behav Brain Res. 2023;436:114083. doi: 10.1016/j.bbr.2022.114083
- Chen W-L, Xie B, Zhang C, et al. Antidepressant-like and anxiolytic-like effects of hydrogen sulfide in behavioral models of depression and anxiety. Behav Pharmacol. 2013;24(7):590–597.
- Liu SY, Li D, Zeng H-Y, et al. Hydrogen sulfide inhibits chronic unpredictable mild stress-induced depressive-like behavior by upregulation of Sirt-1: involvement in suppression of hippocampal endoplasmic reticulum stress. Int J Neuropsychopharmacol. 2017;20(11):867–876.
- Xu S, Liu Y, Pu J, et al. Chronic stress in a rat model of depression disturbs the Glutamine-Glutamate-GABA cycle in the striatum, hippocampus, and cerebellum. Neuropsychiatr Dis Treat. 2020;16:557–570. doi: 10.2147/NDT.S245282
- Bikri S, Talhaoui A, Fath N, et al. Insulin supplemented with phenolic fraction concentrates displays anxiolytic and antidepressant-like properties with reductions of oxidative brain damage in chronically stressed diabetic rats. J Herbmed Pharmacol. 2022a;11(4):562–574.
- Bikri S, Fath N, El Aboubi M, et al. Phenolic fraction concentrates supplementation ameliorates learning and memory impairments in chronically stressed streptozotocin-diabetic rats by reducing brain tumor necrosis factor-α. J Herbmed Pharmacol. 2022b;11(4):592–603.
- Benmhammed H, El Hayek S, Nassiri A, et al. Effects of lipopolysaccharide administration and maternal deprivation on anxiety and depressive symptoms in male and female Wistar rats: Neurobehavioral and biochemical assessments. Behav Brain Res. 2019;362:46–55. doi: 10.1016/j.bbr.2019.01.005
- Beauchamp C, Fridovich I. Superoxide dismutase: improved assays and an assay applicable to acrylamide gels. Anal Biochem. 1971;44(1):276–287. doi: 10.1016/0003-2697(71)90370-8
- Draper HH, Hadley M. Malondialdehyde determination as index of lipid peroxidation. Methods Enzymol. 1990;186:421–431. doi: 10.1016/0076-6879(90)86135-i
- Chao CC, Hu S, Molitor TW, et al. Activated microglia mediate neuronal cell injury via a nitric oxide mechanism. J Immunol. 1992;149(8):2736–2741.
- Bradford MM. A rapid and sensitive method for the quantitation of microgram quantities of protein utilizing the principle of protein-dye binding. Anal Biochem. 1976;72(1–2):248–254. doi: 10.1016/0003-2697(76)90527-3
- Youssef B, Ramadan KS, ElShebiney S, et al. Antidepressant-like effects of aqueous extracts of miswak (Salvadora persica) and date palm (Phoenix dactylifera) on depression-like behaviors using CUMS model in male rats. J Food Biochem. 2022;46(8):e14164.
- Wang M, Zhu J, Pan Y, et al. Hydrogen sulfide functions as a neuromodulator to regulate striatal neurotransmission in a mouse model of P arkinson’s disease. J Neurosci Res. 2015;93(3):487–494.
- Tang ZJ, Zou W, Yuan J, et al. Antidepressant-like and anxiolytic-like effects of hydrogen sulfide in streptozotocin-induced diabetic rats through inhibition of hippocampal oxidative stress. Behav Pharmacol. 2015;26(5):427–435. doi: 10.1097/FBP.0000000000000143
- Stefanovic B, Spasojevic N, Jovanovic P, et al. Melatonin mediated antidepressant-like effect in the hippocampus of chronic stress-induced depression rats: Regulating vesicular monoamine transporter 2 and monoamine oxidase a levels. Eur Neuropsychopharmacol. 2016;26(10):1629–1637. doi: 10.1016/j.euroneuro.2016.07.005
- Detanico BC, Piato AL, Freitas JJ, et al. Antidepressant-like effects of melatonin in the mouse chronic mild stress model. Eur J Pharmacol. 2009;607(1–3):121–125.
- Vega-Rivera NM, Ortiz-López L, Granados-Juárez A, et al. Melatonin reverses the depression-associated behaviour and regulates microglia, fractalkine expression and neurogenesis in adult mice exposed to chronic mild stress. Neuroscience. 2020;440:316–336. doi: 10.1016/j.neuroscience.2020.05.014
- Wang YQ, Jiang YJ, Zou MS, et al. Antidepressant actions of melatonin and melatonin receptor agonist: Focus on pathophysiology and treatment. Behav Brain Res. 2022;420:113724. doi: 10.1016/j.bbr.2021.113724
- Stephens MA, Wand G. Stress and the HPA axis: role of glucocorticoids in alcohol dependence. Alcohol Res. 2012;34(4):468–483.
- Liao D, Lv C, Cao L, et al. Curcumin attenuates chronic unpredictable mild stress-induced depressive-like behaviors via restoring changes in oxidative stress and the activation of Nrf2 signaling pathway in rats. Oxidative medicine and cellular longevity 9268083. Oxid Med Cell Longevity. 2020;2020:1–11. doi: 10.1155/2020/9268083
- Quan W, Liu F, Zhang Y, et al. Antidepressant-like effects of magnesium lithospermate B in a rat model of chronic unpredictable stress. Pharm Biol. 2015;53(8):1168–1175. doi: 10.3109/13880209.2014.967783
- Wang S, Xu X, Ju X, et al. Melatonin ameliorated CUMS-induced depression-like behavior via restoring endoplasmic reticulum stress in rat hippocampus. Neuroreport. 2021;32(1):8–15. doi: 10.1097/WNR.0000000000001554
- Gomaa AM, Galal HM, Abou-Elgait AT. Neuroprotective effects of melatonin administration against chronic immobilization stress in rats. Int J Physiol Physiol Pharmacol. 2017 Apr 15;9(2):16–27.
- Torres-Farfan C, Richter HG, Rojas-Garcia P, et al. mt1 Melatonin receptor in the primate adrenal gland: inhibition of adrenocorticotropin-stimulated cortisol production by melatonin. J Clin Endocrinol Metab. 2003;88:450–458.
- Dello Russo C, Tringali G, Ragazzoni E, et al. Evidence that hydrogen sulphide can modulate hypothalamo-pituitary-adrenal axis function: in vitro and in vivo studies in the rat. J Neuroendocrinol. 2000 Mar;12(3):225–233. doi: 10.1046/j.1365-2826.2000.00441.x
- Chaudhary P, Janmeda P, Docea AO, et al. Oxidative stress, free radicals and antioxidants: potential crosstalk in the pathophysiology of human diseases. Front Chem. 2023;11:1158198. doi: 10.3389/fchem.2023.1158198
- Cobley JN, Fiorello ML, Bailey DM. 13 reasons why the brain is susceptible to oxidative stress. Redox Biol. 2018;15:490–503. doi: 10.1016/j.redox.2018.01.008
- Juszczyk G, Mikulska J, Kasperek K, et al. Chronic stress and oxidative stress as common factors of the pathogenesis of depression and Alzheimer’s disease: the role of antioxidants in prevention and treatment. Antioxidants (Basel). 2021;10(9):1439.
- Hassamal S. Chronic stress, neuroinflammation, and depression: an overview of pathophysiological mechanisms and emerging anti-inflammatories. Front Psychiatry. 2023;14:1130989. doi: 10.3389/fpsyt.2023.1130989
- Harrison NA, Cercignani M, Voon V, et al. Effects of inflammation on hippocampus and substantia nigra responses to novelty in healthy human participants. Neuropsychopharmacology. 2015;40(4):831–838.
- Bakunina N, Pariante CM, Zunszain PA. Immune mechanisms linked to depression via oxidative stress and neuroprogression. Immunology. 2015;144(3):365–373. doi: 10.1111/imm.12443
- Bajpai A, Verma AK, Srivastava M, et al. Oxidative stress and major depression. J Clin Diagn Res: JCDR. 2014;8(12):CC04–CC7.
- Liu Y, Ho RC-M, Mak A. Interleukin (IL)-6, tumour necrosis factor alpha (TNF-α) and soluble interleukin-2 receptors (sIL-2R) are elevated in patients with major depressive disorder: a meta-analysis and meta-regression. J Affective Disorders. 2012;139(3):230–239. doi: 10.1016/j.jad.2011.08.003
- Lucca G, Comim CM, Valvassori SS, et al. Effects of chronic mild stress on the oxidative parameters in the rat brain. Neurochem Int. 2009 May-Jun;54(5–6):358–362. doi: 10.1016/j.neuint.2009.01.001
- Ogłodek EA, Just MJ, Szromek AR, et al. Melatonin and neurotrophins NT-3, BDNF, NGF in patients with varying levels of depression severity. Pharmacol Rep. 2016;68(5):945–951.
- Rosales-Corral S, Tan DX, Reiter RJ, et al. Orally administered melatonin reduces oxidative stress and proinflammatory cytokines induced by amyloid-beta peptide in rat brain: a comparative, in vivo study versus vitamin C and E. J Pineal Res. 2003;35(2):80–84. doi: 10.1034/j.1600-079x.2003.00057.x
- Hu M, Zou W, Wang CY, et al. Hydrogen sulfide protects against chronic unpredictable mild stress-induced oxidative stress in hippocampus by upregulation of BDNF-TrkB pathway. Oxid Med Cell Longev. 2016;2016:2153745. doi: 10.1155/2016/2153745
- Sunzini F, De Stefano S, Chimenti MS, et al. Hydrogen sulfide as potential regulatory gasotransmitter in arthritic diseases. Int J Mol Sci. 2020;21(4):1180.
- Ali M, Fahmy M, Haggag W, et al. Evaluation of cognitive impairment in patients with major depressive disorder in remission. Middle East Curr Psychiatry. 2021;28(1). doi: 10.1186/s43045-021-00149-x
- Liu J, Chen Y, Xie X, et al. The percentage of cognitive impairment in patients with major depressive disorder over the course of the depression: A longitudinal study. J Affect Disord. 2023;329(329):511–518.
- Dobielska M, Bartosik NK, Zyzik KA, et al. Mechanisms of cognitive impairment in depression. May probiotics help? Front Psychiatry. 2022;13(13):904426.
- Alqurashi GK, Hindi EA, Zayed MA, et al. The impact of chronic unpredictable mild stress-induced depression on spatial, recognition and reference memory tasks in mice: behavioral and histological study. Behav Sci (Basel). 2022;12(6):166.
- Ulrich-Lai YM, Figueiredo HF, Ostrander MM, et al. Chronic stress induces adrenal hyperplasia and hypertrophy in a subregion-specific manner. Am J Physiol Endocrinol Metab. 2006;291(5):E965–E973. doi: 10.1152/ajpendo.00070.2006
- Abe K, Kimura H. The possible role of hydrogen sulfide as an endogenous neuromodulator. J Neurosci. 1996;16(3):1066–1071. doi: 10.1523/JNEUROSCI.16-03-01066.1996
- Duman RS, Aghajanian GK. Synaptic dysfunction in depression: potential therapeutic targets. Science. 2012;338(6103):68–72. doi: 10.1126/science.1222939
- Tan H, Zou W, Jiang J, et al. Disturbance of hippocampal H2S generation contributes to CUMS-induced depression-like behavior: involvement in endoplasmic reticulum stress of hippocampus. Acta Biochim Biophys Sin (Shanghai). 2015;47(4):285–291. doi: 10.1093/abbs/gmv009
- Wei HJ, Li X, Tang XQ. Therapeutic benefits of H₂S in Alzheimer’s disease. J Clin Neurosci. 2014;21(10):1665–1669. doi: 10.1016/j.jocn.2014.01.006
- Fang X, Han Q, Li S, et al. Melatonin attenuates spatial learning and memory dysfunction in developing rats by suppressing isoflurane-induced endoplasmic reticulum stress via the SIRT1/Mfn2/PERK signaling pathway. Heliyon. 2022;8(9):e10326. doi: 10.1016/j.heliyon.2022.e10326
- Dera HA, Alassiri M, Kahtani RA, et al. Melatonin attenuates cerebral hypoperfusion-induced hippocampal damage and memory deficits in rats by suppressing TRPM7 channels. Saudi J Biol Sci. 2022;29(4):2958–2968.
- Gonzalez MC, Radiske A, Cammarota M. On the involvement of BDNF signaling in memory reconsolidation. Front Cell Neurosci. 2019;13(13):383. doi: 10.3389/fncel.2019.00383
- Ávila-Villanueva M, Gómez-Ramírez J, Maestú F, et al. The role of chronic stress as a trigger for the Alzheimer disease continuum. Front Aging Neurosci. 2020;12:561504. doi: 10.3389/fnagi.2020.561504
- Miranda-Riestra A, Estrada-Reyes R, Torres-Sanchez ED, et al. Melatonin: a neurotrophic factor? Molecules (Basel, Switzerland). 2022;27(22):7742. doi: 10.3390/molecules27227742
- Labban S, Alshehri FS, Kurdi M, et al. Melatonin improves short-term spatial memory in a mouse model of Alzheimer’s disease. Degener Neurol Neuromuscul Dis. 2021;11:15–27. doi: 10.2147/DNND.S291172
- Shu-Yun L, Dan L, Hai-Ying Z, et al. Hydrogen sulfide inhibits chronic unpredictable mild stress-induced depressive-like behavior by upregulation of Sirt-1: involvement in suppression of hippocampal endoplasmic reticulum stress. Int J Neuropsychopharmacol. 2017;20(11):867–876.