ABSTRACT
Evidence is accumulating that developmental exposure to Bisphenol A (BPA), an abundant endocrine disrupting chemical (EDC), affects neurodevelopment and later brain function, including cognitive performance, social and affective behaviors. However, an impact of BPA exposure on the stress system regulators has only recently come into focus. This mini-review aims to summarize and discuss the available findings from animal studies regarding BPA effects on the stress system and the hypothalamic-pituitary- adrenal (HPA) axis in particular. It appears that perinatal exposure of rodents to low doses of BPA, around the human tolerable daily intake, can modify the levels, expression and epigenetic patterning of key stress mediators at later-life stages in a sex-specific way. These observations provide evidence that developmental exposure to one of the most highly produced chemicals today may contribute to the regulation of the stress axis. Given the importance of the HPA axis in coordination of the organisms' global homeostasis and its implications in stress-related disorders, further studies are needed to delineate the underling mechanisms of these BPA actions.
Introduction
Increasing evidence shows that perinatal exposure to endocrine disrupting chemicals (EDC), such as Bisphenol A (BPA), is particularly harmful for brain function as it induces long-lasting alterations in sex hormone receptors function, neurotransmitter signaling and related behaviors. However, little is known about the effects of EDCs on the stress system, and the few initial studies refer to lead effects in childrenCitation1 or to effects of nonylphenol, phthalatesCitation2 and recently of BPACitation3 in aquatic organisms. With respect to BPA, initial indirect indications for its impact on the stress response system of mammals derived from studies reporting changes in the anxiety state of pubertal or adult rats and mice exposed perinatally to the agent (these studies are summarized below). Recent observations in children also associate urinary BPA concentrations of either the children or their pregnant mothers with increased anxiety and other behavioral aberrations in childhood (for a systematic review see Ejaredar et al.Citation4). However, direct indications for the effects of BPA exposure on the hypothalamic-pituitary-adrenal (HPA) axis of rats appeared only recently.Citation5-10 These observations denote that perinatal exposure to this abundant EDC impacts on several components of the HPA axis that could modify stress responsiveness. Contemporary data suggest that the exposure window is critical for the detected BPA effects on the stress system.Citation11,12 Due to the ample exposure of organisms to BPA and the importance of the HPA axis in the maintenance of organisms' homeostasis, their possible interactions are worth further attention.
The scope of this mini-review is to present and discuss the available experimental data on this theme. The focus was set to the impact of BPA exposure on the HPA axis regulators. Accordingly, the literature search was bounded to recent studies that, in addition to behavioral observations, had examined components of the limbic-HPA axis involved in the stress response. The numerous studies reporting alterations in anxiety levels following BPA exposure are beyond the scope of the present mini-review and are not fully presented herein. Reviewing the relevant literature provided 8 studies (). All studies were conducted in rats, however their protocols differ in terms of the daily dose (2 or 40 μg BPA/Kg bw), the administration route (oral vs. injected), exposure windows (perinatal/long-term vs. pubertal/short-term) and time of outcome evaluation (puberty vs. adulthood). Some of the above parameters may significantly influence the detected effects and will be considered in the presentation of the results.
Table 1. Effects of BPA in the stress axis of rats.
The HPA axis
The organism's response to any situation that threatens homeostasis is driven by the stress system that comprises the sympathomedullary and the hypothalamic-pituitary-adrenal (HPA) axis. The HPA axis is the neuroendocrine effector of the system that mediates both enhancement and termination of the stress response. Following stress, the corticotrophin releasing hormone (CRH) and arginine-vasopressin (AVP), released from paraventricular hypothalamic neurons, trigger the secretion of the adrenocorticotropic hormone (ACTH) from the anterior pituitary, which in turn stimulates the release of glucocorticoids from the adrenal cortex. Extra-hypothalamic brain areas, such as the hippocampus, amygdala and prefrontal cortex, are also mutually interacting with the HPA axis, both at basal and under stress conditions. Glucocorticoids, the axis' final effectors, play key roles in both the potentiation of the response and its termination and return to homeostasis. By binding to their receptors (GR), they exert negative feedback in axis' activation at the level of the pituitary, the hypothalamus and the hippocampus (). Unsuccessful adaptation to stress is linked to severe pathophysiology including depression, obesity and chronic inflammation. Notably, HPA axis components have been identified as targets for epigenetic modifications induced by early life adversities that can persist throughout life.Citation13
Figure 1. The hypothalamic-pituitary-adrenal axis (HPA) and BPA's effects under basal conditions. Hypothalamic and higher brain centers such as the hippocampus control HPA axis function. Corticotrophin releasing hormone (CRH) from the paraventricular hypothalamic area stimulates the release of adrenocorticotrophin hormone (ACTH) from the anterior pituitary, which in turn triggers the release of glucocorticoids (corticosterone in rodents) from the adrenal cortex. Following stress, the highly secreted glucocorticoids, via their receptors (GR), are responsible for the termination of the axis activation through a negative feedback loop exerted on the central axis components. GR activity is tightly regulated by the co-chaperon FKBP51 that inhibits GR hormone binding affinity and nuclear translocation via an ultra-short feedback loop within the HPA axis. The figure illustrates the reported effects of BPA on the HPA axis under basal conditions. Perinatal BPA exposure appears to affect most HPA axis regulators, in a sex-specific manner, including FKBP51 expression.
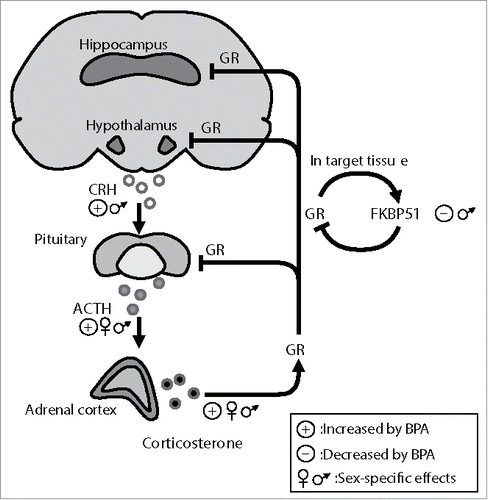
One of the HPA axis' regulators that is sensitive to environmental factors and epigenetic changes is FKBP51. This co-chaperone (the protein product of the Fkbp5 gene), is a negative regulator of GR translocation to the nucleus.Citation14,15 Interestingly, Fkbp5 is itself a GR target and glucocorticoids induce its expression.Citation16 Chronic exposure to glucocorticoids persistently changes Fkbp5 expression by altering DNA methylation of the gene in the mouse hippocampus and hypothalamus.Citation17,18 Similar epigenetic changes have been detected in patients with post-traumatic stress disorder and bipolar disorder,Citation19,20 highlighting the importance of this GR coregulator in stress related disorders.Citation21
BPA
BPA is one of the most highly produced chemicals today,Citation22 used in the production of polycarbonate plastics and epoxy- resins. Common BPA-containing products include water bottles, food and beverage cans, personal care products, thermal receipt paper, and medical equipment.Citation23 Consequently, humans and animals are exposed to BPA on a daily basis and biomonitoring studies estimate that over 90% of the population has measurable urinary BPA levels.Citation23 Early toxicokinetic studies suggested that BPA is rapidly metabolized (6 h in plasma after oral administration).Citation24,25 However, it seems that exposure route and type (acute vs. chronic), age, sex, and genetic background can affect BPA bioaccumulation and/or excretion from the organism. Epidemiological studies show higher exposure and urine BPA accumulation in children compared to adults.Citation23 Moreover, BPA readily passes the placenta and accumulates in the fetus due to its immature drug-metabolizing system.Citation26 It particularly accumulates in the fetal brain,Citation27,28 where its average concentration is higher in males than in females.Citation27
The tolerable daily intake, based on a classical dose-response relationship, is set to 50 μg/kg in USA and 4 μg/kg in Europe. However, BPA at biologically relevant levels has repeatedly shown non-monotonic effects in different cell and animal models and even in humans.Citation29 The reason for these non-monotonic, low-dose effects may reside in BPA's numerous mechanisms of action at the cellular level. The most studied mechanism is via the 2 estrogen receptors, ERα and ERβ. BPA acts as partial agonist for these receptors, albeit with 10,000-100,000 fold lower affinity than estradiol. Its potency differs for the 2 ERs and is cell type-dependent.Citation30 However, it is now clear that BPA affects other targets in the cell, thereby interfering with additional signaling pathways. For example, it can induce fast non-genomic responses via ERα or the transmembrane G-protein coupled receptor GPR30.Citation31-36 Further, both in vitroCitation37 and in vivoCitation38 studies have shown that BPA binds and activates estrogen related receptor gamma (ERRγ), an orphan receptor that interferes with ER signaling.Citation39 Additionally, BPA is a weak androgen receptor (AR) antagonist,Citation30 it interferes with thyroid hormone signalingCitation30 and activates GR.Citation40
BPA effects on the stress system of rodents
Initial observations
Indirect evidence for a potential BPA effect on the stress response derived from several studies showing BPA-induced changes in anxiety-related behavior of rats and mice. The effects vary depending on the dose, time of exposure and evaluation, and the animal's sex and species. A handful of these studies, mostly conducted in mice, report increased anxiety levels upon perinatal BPA exposureCitation41-44 or pubertal exposureCitation45 while others find no changesCitation46-50 or even anxiolytic effects.Citation51-53 Importantly, in the study applying several exposure doses, non-monotonic effects for this BPA action were detected.Citation53
Although anxiety-like behavior is often expressed during stress exposure and the anxiety status can modify stress coping, it cannot be directly, or solely, associated with the function of the neuroendocrine stress axis, since anxiety is often the outcome of changes in other circuits and neurotransmitters. Indeed BPA-induced affective and neurotransmitter changes have been reported in previous animal studies. For example, in perinatally exposed mice the altered anxiety state has been related to changes in dopaminergicCitation43 and glutamatergicCitation42,52 systems.
Therefore, we and others initiated studies directly addressing potential BPA effects on the key regulators of the stress response.
Effects on the HPA axis effectors
Direct evidence for possible BPA effects on the HPA axis of rats upon perinatal exposure were first reported in 2010Citation5 and further elaborated in the following years.Citation6-10 Based on the available literature (), the BPA-induced alterations in HPA axis of exposed animals differ depending on the exposure window, dose, sex and age at evaluation. All studies using perinatal BPA administrationCitation6-10 report significant alterations in HPA axis' components at later life stages (i.e. puberty or adulthood) that appear dose-dependent and sex-specific. In contrast, short-term BPA exposure in adolescence revealed no significant alterations in any HPA axis component at either puberty or adulthood.Citation11,12 All studies used BPA doses (2 and 40 μg /kg /d) within the range of the current tolerable daily intake for humans (4-50 μg /kg /d).
Effects on adrenals and stress hormone levels
Perinatal exposure to 40 μg BPA /kg /d increased adrenal weight of female BPA-offspring at mid-puberty.Citation6 Additionally, BPA-offspring of both sexes exhibited a hyperplastic zona fasciculata (the glucocorticoid synthesising zone), while male BPA-offspring had a diminished zona reticularis, where the non-gonadal androgens are synthesized. Although there are no further studies for BPA effects on adrenal glands, reports from other EDCs support an impact of xenoestrogens in adrenal physiology. Early life exposure to genisteinCitation54 reduces adrenal steroidogenesis and expands zona fasciculata and reticularis of pubertal male offspring, while nonylphenol exposure leads to hyperadrenalism in both sexes.Citation55
Perinatal BPA exposure (40 μg BPA /kg /d) increased basal plasma corticosterone levels in female, but not male, offspring tested at puberty.Citation6 By using the same protocol and BPA dose, Zhou et al.Citation10 also detected increased corticosterone and ACTH concentrations in BPA-exposed pubertal females at circadian peak and nadir. These findings were further supported by behavioral data of the same studies showing increased anxiety of the adolescent female BPA-offspring in different anxiety tests.
Perinatal BPA exposure also led to sex-specific changes in the stress-induced corticosterone response of the offspring at puberty. Upon exposure to a 15-min swimming stress, female BPA-offspring exhibited lower stress-induced corticosterone levels than control females at 30 and 60 min post-stress, whereas male BPA-offspring showed higher stress-induced corticosterone levels at 30 and 120 min post-stress compared to BPA-treated females.Citation6 In addition, Zhou et al.Citation10 detected an inefficient response to the dexamethasone suppression test in pubertal female BPA-offspring, denoting an impairment of negative feedback inhibition in these animals. These data suggest that perinatal BPA exposure impacts corticosterone responses primarily in female offspring at puberty. These alterations imply HPA axis hyperactivity that in humans is related to the appearance of affective disorders with early onset in adolescence.Citation56
Interestingly, differential impact of perinatal BPA exposure on corticosterone levels has been reported when rats were exposed to 2 μg BPA/Kg /d, instead of 40μg, and evaluated in adulthood, instead of puberty.Citation7,8 BPA-exposed males, but not females, had increased corticosterone, ACTH and CRH levels at basal conditions. These changes were followed by increased anxiety and depression-like behavior in male BPA-offspring and reduced anxiety in female offspring. BPA-treated males had also higher corticosterone and ACTH levels immediately after stress. However, according to corticosterone stress kinetics,Citation57 hormonal determinations so close to stress onset (5 min) are not informative for the corticosterone stress response. Further studies are required, preferably by using the same group of animals in several sampling points following stress, to clarify the stress-induced hormonal responses.
The aforementioned contrasting observations may rely on the existence of non-monotonic BPA actions previously reported for this agent.Citation29 Differential dose- and sex-specific behavioral effects of BPA have been detected among mice exposed in utero to 2, 20 and 200 μg of BPA/Kg/d.Citation58 Another explanation could reside in the differences of HPA axis physiology between puberty and adulthood, since puberty is a period of ongoing maturation of the axis.Citation59 Furthermore, short pubertal exposure of rats to 40 μg BPA/Kg /d had no effects on anxiety and corticosterone levels during stress at either puberty or adulthoodCitation11,12 denoting that the perinatal period is probably the most susceptible for BPA effects on corticosterone levels, in agreement with observations in other systems.Citation27,28
Effects on stress hormone receptors
Upon perinatal BPA exposure (40 μg BPA/kg/d), glucocorticoid receptor levels were reduced in the hypothalamiCitation6 and hippocampiCitation5,10 homogenates from pubertal female rats. The decreases in GR levels may represent a tissue response to the increased corticosterone levels observed in these animals under basal conditions. The detected GR down-regulation, reportedly a stress-induced mechanism to protect the brain from excess of glucocorticoids, implies that these animals were in a stress-like state. Following stress, no further alterations were found in GR levels of female BPA-treated offspring, compared to basal conditions, whereas the receptor levels were down-regulated in the hypothalami of control females,Citation6 suggesting possible disturbances in the negative feedback mediated by this receptor at the level of the hypothalamus. No effects of BPA were detected in the basal mRNA levels of pituitary proopiomelanocortin (POMC) and CRH receptor 1 (CRHR1) in adolescent rats of either sex, upon perinatal exposure to the dose of 40 μg /kg /d. However, following stress, CRHR1 and POMC levels were increased in male BPA-offspring, implying that perinatal BPA exposure heightens stress response at the level of the pituitary in a sex-specific manner.Citation6
Similarly to the hormonal levels, different findings are reported for the adult rats upon perinatal exposure to 2μg (instead of 40μg) BPA /kg /d, that are however compatible with the hormonal and behavioral measures of the same animals. Decreased hippocampal GR and MR mRNA were detected in the adult male BPA-offspring, under basal conditions, whereas GR levels in the PVN and GR and MR in the hippocampus of female BPA-offspring were increased.Citation7 Non-monotonic BPA actionsCitation29 and differences between adolescent and adult brain physiologyCitation59 could possibly account for these differences among studies, but further studies are required to delineate these findings.
HPA and gonadal hormone signaling
It is well accepted that HPA axis function can be modulated by gonadal hormones. Estrogens are enhancing, whereas androgens are inhibitory to the activation of the axis.Citation60 ERα and ERβ in the brain are implicated in affective behaviorsCitation61 whereby ERβ mediates anxiolytic actions.Citation62 BPA can interfere with gonadal receptor signaling due to its agonistic properties on ERα and ERβ and antagonistic properties on AR.Citation30
Developmental BPA exposure reduces male steroidogenesis.Citation63 Low doses of perinatal BPA affect rodent estrogen receptor levels in a sexually dimorphic manner. Khurana and co-workersCitation64 observed that neonatal BPA exposure increases the expression of ERα in the female hypothalamus and the male pituitary. Another study reported sex- and BPA dose-dependent changes in ERα, ERβ and ERRγ mRNA levels in the hippocampus, hypothalamus, and prefrontal cortex of juvenile mice.Citation58 Chen et al.Citation7 showed reduced circulating testosterone levels and decreased ERβ mRNA in adult male rats perinatally exposed to BPA, as well as increased ERα in the hypothalamus of both sexes. In the last study, the reduced testosterone levels along with the increased ERα and decreased ERβ levels, could confer to the enhanced HPA responsiveness and increased anxiety detected in these male BPA-offspring.
The existing evidence suggest that BPA effects on the stress system and related behaviors could be partly exerted through changes induced in the gonadal hormone signaling, however further studies are required to clarify the underlying mechanisms.
Epigenetic alterations
Brain maturation during puberty is under the influence of gonadal hormones, and epigenetic modifications are involved in this sex-specific process. Epigenetic mechanisms, apart from regulating the programmed brain development, are also responsive to environmental inputs that contribute to the final brain shaping.Citation65 Epigenetic mechanisms include DNA methylation, post-translational modification at histone tails, and regulation by non-coding RNAs. DNA methylation is considered the most stable epigenetic mark with a clear mechanism of heritability brought about by DNA methyltransferase 1 (DNMT1) that establishes the methylation pattern of the mother strand at the daughter strand during replication.Citation66 In various experimental systems, BPA was shown to induce gene-specific DNA methylation changes, among others in the brain.Citation58,67 To investigate possible epigenetic modifications in the HPA axis induced by perinatal BPA, we examined DNA methylation changes in the promoter of the GR gene and in intronic areas of its negative regulator Fkbp5. We found increased DNA methylation of Fkbp5 intron 5 and reduced protein levels in the hippocampus of exposed male rats. Similar effects were obtained in the male hippocampal cell line HT22 when exposed to BPA during differentiation. The mechanisms underlying epigenetic effects of BPA are still unknown. Several studies report that BPA changes expression of the DNMTs.Citation58,68,69 This alone, however, would lead to global rather than to gene-specific effects on DNA methylation. In our study, we demonstrated an involvement of ERβ in the epigenetic effect of BPA.Citation9 In another study, we have found that ERβ is involved in regulating local DNA methylation by recruiting thymine DNA glycosylase (TDG), an enzyme involved in DNA demethylation, to specific genomic regions.Citation70 Thus a possible mechanism for how BPA exerts its effects on DNA methylation could involve the disruption of the interaction between ERβ and TDG, and thus the recruitment of this enzyme to specific genomic regions including intron 5 in Fkbp5 ().
Figure 2. Suggested model for the role of ERβ in the inhibitory effect of BPA on Fkbp5, based on observations in differentiating HT22 cells. In the absence of BPA treatment, ERβ prevents DNA-methylation of CpGs at the glucocorticoid response element (GRE) of the Fkbp5 gene. This might be mediated by ERβ's recruitment of thymine DNA-glycosylase (TDG) that is involved in active DNA demethylation. As a result, a transcriptional complex is formed that can cooperate with upstream promoter regions to mediate Fkbp5 expression by activated GRs.Citation19 However, when the cells are exposed to BPA during differentiation, BPA might disrupt ERβ binding, resulting in no TDG recruitment, DNA hypermethylation and lower Fkbp5 expression. This, translated to the HPA axis, could result in enhanced GR activity.
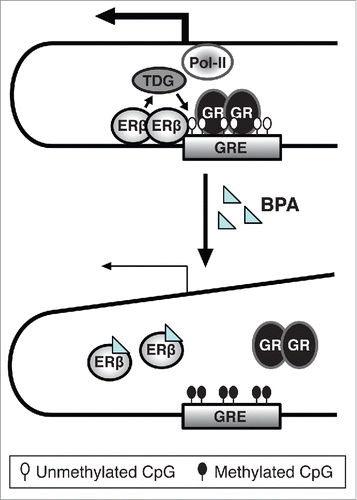
Conclusions
The evidence summarized here implicates BPA in the regulation of an organism's stress system in addition to its known implication in the reproductive and metabolic tracts. The available literature indicates that perinatal BPA exposure of rats significantly impacts most HPA axis' regulators of the exposed offspring at 2 later life-stages (adolescence and adulthood), whereas adolescent exposure does not appear to impact corticosterone or anxiety levels. The perinatal BPA-induced alterations are associated with changes on related behaviors but appear dose- age- area- and sex-specific (, ). The impact of BPA on the stress axis could be influenced by effects on the gonadal steroid hormone signaling and may additionally involve epigenetic changes induced by this endocrine disruptor. However, more studies are required to delineate the molecular events leading to these HPA axis alterations and to shed more light on possible non-monotonic effects. Most importantly, these studies conducted in rodents should be linked to epidemiological findings in order to better understand if developmental BPA exposure could be linked to stress-related disorders in humans.
Abbreviations
ACTH | = | adrenocorticotropic hormone |
AR | = | Androgen receptor |
AVP | = | arginine-vasopressin |
BPA | = | Bisphenol A |
Bw | = | body weight |
CRH | = | corticotrophin releasing hormone |
CRHR1 | = | CRH receptor 1 |
DNMT | = | DNA methyltransferase |
EDCs | = | endocrine disruptive chemicals |
Erα | = | Estrogen receptor α |
ERβ | = | Estrogen receptor β |
ERs | = | Estrogen receptors |
ERRγ | = | estrogen related receptor gamma |
Fkbp5 | = | FK506 binding protein 5 |
FKBP51 | = | FK506 binding protein 51 |
GR | = | glucocorticoid receptor |
GRE | = | Glucocorticoid responsive element |
HPA | = | Hypothalamic Pituitary Adrenal |
MR | = | mineralocorticoid receptor |
POMC | = | proopiomelanocortin |
TDG | = | thymine DNA glycosylase |
Disclosure of potential conflicts of interest
No potential conflicts of interest were disclosed.
Funding
JR receives funding from the Swedish Research Council FORMAS, JR and EK receive funding from the European Union's Horizon 2020 research and innovation program under grant agreement No 634880.
References
- Gump BB, Stewart P, Reihman J, Lonky E, Darvill T, Parsons PJ, Granger DA. Low-level prenatal and postnatal blood lead exposure and adrenocortical responses to acute stress in children. Environ health Perspect 2008; 116:249-55; PMID:18288326; http://dx.doi.org/10.1289/ehp.10391
- Palermo FA, Cocci P, Nabissi M, Polzonetti-Magni A, Mosconi G. Cortisol response to waterborne 4-nonylphenol exposure leads to increased brain POMC and HSP70 mRNA expressions and reduced total antioxidant capacity in juvenile sole (Solea solea). Comp Biochem Physiol Toxicol Pharmacol 2012; 156:135-9; PMID:22918179; http://dx.doi.org/10.1016/j.cbpc.2012.08.002
- Birceanu O, Mai T, Vijayan MM. Maternal transfer of bisphenol A impacts the ontogeny of cortisol stress response in rainbow trout. Aquat Toxicol 2015; 168:11-8; PMID:26398930; http://dx.doi.org/10.1016/j.aquatox.2015.09.002
- Ejaredar M, Lee Y, Roberts DJ, Sauve R, Dewey D. Bisphenol A exposure and children's behavior: A systematic review. J Expo Sci Environ Epidemiol 2016; PMID:26956939; http://dx.doi.org/10.1038/jes.2016.8
- Poimenova A, Markaki E, Rahiotis C, Kitraki E. Corticosterone-regulated actions in the rat brain are affected by perinatal exposure to low dose of bisphenol A. Neuroscience 2010; 167:741-9; PMID:20219646; http://dx.doi.org/10.1016/j.neuroscience.2010.02.051
- Panagiotidou E, Zerva S, Mitsiou DJ, Alexis MN, Kitraki E. Perinatal exposure to low-dose bisphenol A affects the neuroendocrine stress response in rats. J Endocrinol 2014; 220:207-18; PMID:24323913; http://dx.doi.org/10.1530/JOE-13-0416
- Chen F, Zhou L, Bai Y, Zhou R, Chen L. Sex differences in the adult HPA axis and affective behaviors are altered by perinatal exposure to a low dose of bisphenol A. Brain Res 2014; 1571:12-24; PMID:24857958; http://dx.doi.org/10.1016/j.brainres.2014.05.010
- Chen F, Zhou L, Bai Y, Zhou R, Chen L. Hypothalamic-pituitary-adrenal axis hyperactivity accounts for anxiety- and depression-like behaviors in rats perinatally exposed to bisphenol A. J Biomed Res 2015; 29:250-8; PMID:26060449
- Kitraki E, Nalvarte I, Alavian-Ghavanini A, Ruegg J. Developmental exposure to bisphenol A alters expression and DNA methylation of Fkbp5, an important regulator of the stress response. Mol Cell Endocrinol 2015; 417:191-9; PMID:26427651; http://dx.doi.org/10.1016/j.mce.2015.09.028
- Zhou R, Chen F, Feng X, Zhou L, Li Y, Chen L. Perinatal exposure to low-dose of bisphenol A causes anxiety-like alteration in adrenal axis regulation and behaviors of rat offspring: a potential role for metabotropic glutamate 2/3 receptors. J Psychiatr Res 2015; 64:121-9; PMID:25812946; http://dx.doi.org/10.1016/j.jpsychires.2015.02.018
- Bowman RE, Luine V, Khandaker H, Villafane JJ, Frankfurt M. Adolescent bisphenol-A exposure decreases dendritic spine density: role of sex and age. Synapse 2014; 68:498-507; PMID:24975924; http://dx.doi.org/10.1002/syn.21758
- Bowman RE, Luine V, Diaz Weinstein S, Khandaker H, DeWolf S, Frankfurt M. Bisphenol-A exposure during adolescence leads to enduring alterations in cognition and dendritic spine density in adult male and female rats. Horm Behav 2015; 69:89-97; PMID:25554518; http://dx.doi.org/10.1016/j.yhbeh.2014.12.007
- McEwen BS, Gray JD, Nasca C. 60 YEARS OF NEUROENDOCRINOLOGY: Redefining neuroendocrinology: stress, sex and cognitive and emotional regulation. J Endocrinol 2015; 226:T67-83; PMID:25934706; http://dx.doi.org/10.1530/JOE-15-0121
- Touma C, Gassen NC, Herrmann L, Cheung-Flynn J, Bull DR, Ionescu IA, Heinzmann JM, Knapman A, Siebertz A, Depping AM, et al. FK506 binding protein 5 shapes stress responsiveness: modulation of neuroendocrine reactivity and coping behavior. Biol Psychiatry 2011; 70:928-36; PMID:21907973; http://dx.doi.org/10.1016/j.biopsych.2011.07.023
- Wochnik GM, Ruegg J, Abel GA, Schmidt U, Holsboer F, Rein T. FK506-binding proteins 51 and 52 differentially regulate dynein interaction and nuclear translocation of the glucocorticoid receptor in mammalian cells. J Biol Chem 2005; 280:4609-16; PMID:15591061; http://dx.doi.org/10.1074/jbc.M407498200
- Vermeer H, Hendriks-Stegeman BI, van der Burg B, van Buul-Offers SC, Jansen M. Glucocorticoid-induced increase in lymphocytic FKBP51 messenger ribonucleic acid expression: a potential marker for glucocorticoid sensitivity, potency, and bioavailability. J Clin Endocrinol Metab 2003; 88:277-84; PMID:12519866; http://dx.doi.org/10.1210/jc.2002-020354
- Lee RS, Tamashiro KL, Yang X, Purcell RH, Harvey A, Willour VL, Huo Y, Rongione M, Wand GS, Potash JB. Chronic corticosterone exposure increases expression and decreases deoxyribonucleic acid methylation of Fkbp5 in mice. Endocrinology 2010; 151:4332-43; PMID:20668026; http://dx.doi.org/10.1210/en.2010-0225
- Yang X, Ewald ER, Huo Y, Tamashiro KL, Salvatori R, Sawa A, Wand GS, Lee RS. Glucocorticoid-induced loss of DNA methylation in non-neuronal cells and potential involvement of DNMT1 in epigenetic regulation of Fkbp5. Biochem Biophys Res Commun 2012; 420:570-5; PMID:22445894; http://dx.doi.org/10.1016/j.bbrc.2012.03.035
- Klengel T, Mehta D, Anacker C, Rex-Haffner M, Pruessner JC, Pariante CM, Pace TW, Mercer KB, Mayberg HS, Bradley B. Allele-specific FKBP5 DNA demethylation mediates gene-childhood trauma interactions. Nat Neurosci 2013; 16:33-41; PMID:23201972; http://dx.doi.org/10.1038/nn.3275
- Fries GR, Vasconcelos-Moreno MP, Gubert C, dos Santos BT, Sartori J, Eisele B, Ferrari P, Fijtman A, Ruegg J, Gassen NC, et al. Hypothalamic-pituitary-adrenal axis dysfunction and illness progression in bipolar disorder. Int J Neuropsychopharmacol 2014; 18; PMID:25522387
- Schmidt MV, Paez-Pereda M, Holsboer F, Hausch F. The prospect of FKBP51 as a drug target. ChemMedChem 2012; 7:1351-9; PMID:22581765; http://dx.doi.org/10.1002/cmdc.201200137
- Vandenberg LN, Hauser R, Marcus M, Olea N, Welshons WV. Human exposure to bisphenol A (BPA). Reproduct Toxicol 2007; 24:139-77; PMID:17825522; http://dx.doi.org/10.1016/j.reprotox.2007.07.010
- Calafat AM, Ye X, Wong LY, Reidy JA, Needham LL. Exposure of the U.S. population to bisphenol A and 4-tertiary-octylphenol: 2003–2004. Environ health Perspect 2008; 116:39-44; PMID:18197297; http://dx.doi.org/10.1289/ehp.10753
- Pottenger LH, Domoradzki JY, Markham DA, Hansen SC, Cagen SZ, Waechter JM, Jr. The relative bioavailability and metabolism of bisphenol A in rats is dependent upon the route of administration. Toxicol Sci 2000; 54:3-18; PMID:10746927; http://dx.doi.org/10.1093/toxsci/54.1.3
- Volkel W, Colnot T, Csanady GA, Filser JG, Dekant W. Metabolism and kinetics of bisphenol a in humans at low doses following oral administration. Chem Res Toxicol 2002; 15:1281-7; PMID:12387626; http://dx.doi.org/10.1021/tx025548t
- Nishikawa M, Iwano H, Yanagisawa R, Koike N, Inoue H, Yokota H. Placental transfer of conjugated bisphenol A and subsequent reactivation in the rat fetus. Environ health Perspect 2010; 118:1196-203; PMID:20382578; http://dx.doi.org/10.1289/ehp.0901575
- Mita L, Baldi A, Diano N, Viggiano E, Portaccio M, Nicolucci C, Grumiro L, Menale C, Mita DG, Spugnini EP, et al. Differential accumulation of BPA in some tissues of offspring of Balb-C mice exposed to different BPA doses. Environ Toxicol Pharmacol 2012; 33:9-15; PMID:22047638; http://dx.doi.org/10.1016/j.etap.2011.09.008
- Doerge DR, Twaddle NC, Vanlandingham M, Brown RP, Fisher JW. Distribution of bisphenol A into tissues of adult, neonatal, and fetal Sprague-Dawley rats. Toxicol Appl Pharmacol 2011; 255:261-70; PMID:21820460; http://dx.doi.org/10.1016/j.taap.2011.07.009
- Vandenberg LN, Colborn T, Hayes TB, Heindel JJ, Jacobs DR, Jr., Lee DH, Shioda T, Soto AM, vom Saal FS, Welshons WV, et al. Hormones and endocrine-disrupting chemicals: low-dose effects and nonmonotonic dose responses. Endocr Rev 2012; 33:378-455; PMID:22419778; http://dx.doi.org/10.1210/er.2011-1050
- Delfosse V, Grimaldi M, le Maire A, Bourguet W, Balaguer P. Nuclear receptor profiling of bisphenol-A and its halogenated analogues. Vitam Horm 2014; 94:229-51; PMID:24388193; http://dx.doi.org/10.1016/B978-0-12-800095-3.00009-2
- Ge LC, Chen ZJ, Liu HY, Zhang KS, Liu H, Huang HB, Zhang G, Wong CK, Giesy JP, Du J, et al. Involvement of activating ERK1/2 through G protein coupled receptor 30 and estrogen receptor alpha/beta in low doses of bisphenol A promoting growth of Sertoli TM4 cells. Toxicol Lett 2014; 226:81-9; PMID:24495410; http://dx.doi.org/10.1016/j.toxlet.2014.01.035
- Kang NH, Hwang KA, Lee HR, Choi DW, Choi KC. Resveratrol regulates the cell viability promoted by 17beta-estradiol or bisphenol A via down-regulation of the cross-talk between estrogen receptor alpha and insulin growth factor-1 receptor in BG-1 ovarian cancer cells. Food Chem Toxicol 2013; 59:373-9; PMID:23810794; http://dx.doi.org/10.1016/j.fct.2013.06.029
- Kochukov MY, Jeng YJ, Watson CS. Alkylphenol xenoestrogens with varying carbon chain lengths differentially and potently activate signaling and functional responses in GH3/B6/F10 somatomammotropes. Environ health Perspect 2009; 117:723-30; PMID:19479013; http://dx.doi.org/10.1289/ehp.0800182
- Tanabe N, Kimoto T, Kawato S. Rapid Ca(2+) signaling induced by Bisphenol A in cultured rat hippocampal neurons. Neuro Endocrinol Lett 2006; 27:97-104; PMID:16648781
- Li X, Zhang S, Safe S. Activation of kinase pathways in MCF-7 cells by 17beta-estradiol and structurally diverse estrogenic compounds. J Steroid Biochem Mol Biol 2006; 98:122-32; PMID:16413991; http://dx.doi.org/10.1016/j.jsbmb.2005.08.018
- Noguchi S, Nakatsuka M, Asagiri K, Habara T, Takata M, Konishi H, Kudo T. Bisphenol A stimulates NO synthesis through a non-genomic estrogen receptor-mediated mechanism in mouse endothelial cells. Toxicol Lett 2002; 135:95-101; PMID:12243868; http://dx.doi.org/10.1016/S0378-4274(02)00252-7
- Takayanagi S, Tokunaga T, Liu X, Okada H, Matsushima A, Shimohigashi Y. Endocrine disruptor bisphenol A strongly binds to human estrogen-related receptor gamma (ERRgamma) with high constitutive activity. Toxicol Lett 2006; 167:95-105; PMID:17049190; http://dx.doi.org/10.1016/j.toxlet.2006.08.012
- Tohme M, Prud'homme SM, Boulahtouf A, Samarut E, Brunet F, Bernard L, Bourguet W, Gibert Y, Balaguer P, Laudet V. Estrogen-related receptor gamma is an in vivo receptor of bisphenol A. FASEB J 2014; 28:3124-33; PMID:24744145; http://dx.doi.org/10.1096/fj.13-240465
- Giguere V. To ERR in the estrogen pathway. Trends Endocrinol Metab 2002; 13:220-5; PMID:12185669; http://dx.doi.org/10.1016/S1043-2760(02)00592-1
- Sargis RM, Johnson DN, Choudhury RA, Brady MJ. Environmental endocrine disruptors promote adipogenesis in the 3T3-L1 cell line through glucocorticoid receptor activation. Obesity 2010; 18:1283-8; PMID:19927138; http://dx.doi.org/10.1038/oby.2009.419
- Patisaul HB, Bateman HL. Neonatal exposure to endocrine active compounds or an ERbeta agonist increases adult anxiety and aggression in gonadally intact male rats. Horm Behav 2008; 53:580-8; PMID:18308321; http://dx.doi.org/10.1016/j.yhbeh.2008.01.008
- Xu X, Hong X, Xie L, Li T, Yang Y, Zhang Q, Zhang G, Liu X. Gestational and lactational exposure to bisphenol-A affects anxiety- and depression-like behaviors in mice. Horm Behav 2012; 62:480-90; PMID:23240141; http://dx.doi.org/10.1016/j.yhbeh.2012.08.005
- Matsuda S, Matsuzawa D, Ishii D, Tomizawa H, Sutoh C, Nakazawa K, Amano K, Sajiki J, Shimizu E. Effects of perinatal exposure to low dose of bisphenol A on anxiety like behavior and dopamine metabolites in brain. Prog Neuro-psychopharmacol Biol Psychiatry 2012; 39:273-9; PMID:22760093; http://dx.doi.org/10.1016/j.pnpbp.2012.06.016
- Gioiosa L, Parmigiani S, Vom Saal FS, Palanza P. The effects of bisphenol A on emotional behavior depend upon the timing of exposure, age and gender in mice. Horm Behav 2013; 63:598-605; PMID:23470777; http://dx.doi.org/10.1016/j.yhbeh.2013.02.016
- Diaz Weinstein S, Villafane JJ, Juliano N, Bowman RE. Adolescent exposure to Bisphenol-A increases anxiety and sucrose preference but impairs spatial memory in rats independent of sex. Brain Res 2013; 1529:56-65; PMID:23872220; http://dx.doi.org/10.1016/j.brainres.2013.07.018
- Negishi T, Kawasaki K, Suzaki S, Maeda H, Ishii Y, Kyuwa S, Kuroda Y, Yoshikawa Y. Behavioral alterations in response to fear-provoking stimuli and tranylcypromine induced by perinatal exposure to bisphenol A and nonylphenol in male rats. Environ health Perspect 2004; 112:1159-64; PMID:15289160; http://dx.doi.org/10.1289/ehp.6961
- Fujimoto T, Kubo K, Aou S. Prenatal exposure to bisphenol A impairs sexual differentiation of exploratory behavior and increases depression-like behavior in rats. Brain Res 2006; 1068:49-55; PMID:16380096; http://dx.doi.org/10.1016/j.brainres.2005.11.028
- Fujimoto T, Kubo K, Nishikawa Y, Aou S. Postnatal exposure to low-dose bisphenol A influences various emotional conditions. J Toxicol Sci 2013; 38:539-46; PMID:23824010; http://dx.doi.org/10.2131/jts.38.539
- Ryan BC, Vandenbergh JG. Developmental exposure to environmental estrogens alters anxiety and spatial memory in female mice. Horm Behav 2006; 50:85-93; PMID:16540110; http://dx.doi.org/10.1016/j.yhbeh.2006.01.007
- Miyagawa K, Narita M, Narita M, Akama H, Suzuki T. Memory impairment associated with a dysfunction of the hippocampal cholinergic system induced by prenatal and neonatal exposures to bisphenol-A. Neurosci Lett 2007; 418:236-41; PMID:17467901; http://dx.doi.org/10.1016/j.neulet.2007.01.088
- Farabollini F, Porrini S, Dessi-Fulgherit F. Perinatal exposure to the estrogenic pollutant bisphenol A affects behavior in male and female rats. Pharmacol Biochem Beha 1999; 64:687-94; PMID:10593191; http://dx.doi.org/10.1016/S0091-3057(99)00136-7
- Tian YH, Baek JH, Lee SY, Jang CG. Prenatal and postnatal exposure to bisphenol a induces anxiolytic behaviors and cognitive deficits in mice. Synapse 2010; 64:432-9; PMID:20169576; http://dx.doi.org/10.1002/syn.20746
- Jones BA, Watson NV. Perinatal BPA exposure demasculinizes males in measures of affect but has no effect on water maze learning in adulthood. Horm Behav 2012; 61:605-10; PMID:22370244; http://dx.doi.org/10.1016/j.yhbeh.2012.02.011
- Ohno S, Nakajima Y, Inoue K, Nakazawa H, Nakajin S. Genistein administration decreases serum corticosterone and testosterone levels in rats. Life Sci 2003; 74:733-42; PMID:14654166; http://dx.doi.org/10.1016/j.lfs.2003.04.006
- Chang LL, Wun WS, Wang PS. In utero and neonate exposure to nonylphenol develops hyperadrenalism and metabolic syndrome later in life. I. First generation rats (F(1)). Toxicology 2012; 301:40-9; PMID:22765982; http://dx.doi.org/10.1016/j.tox.2012.06.017
- Boyle MP, Brewer JA, Funatsu M, Wozniak DF, Tsien JZ, Izumi Y, Muglia LJ. Acquired deficit of forebrain glucocorticoid receptor produces depression-like changes in adrenal axis regulation and behavior. Proc Natl Acad Sci U S A 2005; 102:473-8; PMID:15623560; http://dx.doi.org/10.1073/pnas.0406458102
- Vallee M, Mayo W, Dellu F, Le Moal M, Simon H, Maccari S. Prenatal stress induces high anxiety and postnatal handling induces low anxiety in adult offspring: correlation with stress-induced corticosterone secretion. J Neurosci 1997; 17:2626-36; PMID:9065522
- Kundakovic M, Gudsnuk K, Franks B, Madrid J, Miller RL, Perera FP, Champagne FA. Sex-specific epigenetic disruption and behavioral changes following low-dose in utero bisphenol A exposure. Proc Natl Acad Sci U S A 2013; 110:9956-61; PMID:23716699; http://dx.doi.org/10.1073/pnas.1214056110
- Eiland L, Romeo RD. Stress and the developing adolescent brain. Neuroscience 2013; 249:162-71; PMID:23123920; http://dx.doi.org/10.1016/j.neuroscience.2012.10.048
- Viau V. Functional cross-talk between the hypothalamic-pituitary-gonadal and -adrenal axes. J Neuroendocrinol 2002; 14:506-13; PMID:12047726; http://dx.doi.org/10.1046/j.1365-2826.2002.00798.x
- Tetel MJ, Pfaff DW. Contributions of estrogen receptor-alpha and estrogen receptor-ss to the regulation of behavior. Biochim Biophys Acta 2010; 1800:1084-9; PMID:20097268; http://dx.doi.org/10.1016/j.bbagen.2010.01.008
- Lund TD, Rovis T, Chung WC, Handa RJ. Novel actions of estrogen receptor-beta on anxiety-related behaviors. Endocrinology 2005; 146:797-807; PMID:15514081; http://dx.doi.org/10.1210/en.2004-1158
- Akingbemi BT, Sottas CM, Koulova AI, Klinefelter GR, Hardy MP. Inhibition of testicular steroidogenesis by the xenoestrogen bisphenol A is associated with reduced pituitary luteinizing hormone secretion and decreased steroidogenic enzyme gene expression in rat Leydig cells. Endocrinology 2004; 145:592-603; PMID:14605012; http://dx.doi.org/10.1210/en.2003-1174
- Khurana S, Ranmal S, Ben-Jonathan N. Exposure of newborn male and female rats to environmental estrogens: delayed and sustained hyperprolactinemia and alterations in estrogen receptor expression. Endocrinology 2000; 141:4512-7; PMID:11108262
- Morrison KE, Rodgers AB, Morgan CP, Bale TL. Epigenetic mechanisms in pubertal brain maturation. Neuroscience 2014; 264:17-24; PMID:24239720; http://dx.doi.org/10.1016/j.neuroscience.2013.11.014
- Bird A. DNA methylation patterns and epigenetic memory. Genes Dev 2002; 16:6-21; PMID:11782440; http://dx.doi.org/10.1101/gad.947102
- Kundakovic M, Gudsnuk K, Herbstman JB, Tang D, Perera FP, Champagne FA. DNA methylation of BDNF as a biomarker of early-life adversity. Proc Natl Acad Sci U S A 2014; PMID:25385582
- Wolstenholme JT, Edwards M, Shetty SR, Gatewood JD, Taylor JA, Rissman EF, Connelly JJ. Gestational exposure to bisphenol a produces transgenerational changes in behaviors and gene expression. Endocrinology 2012; 153:3828-38; PMID:22707478; http://dx.doi.org/10.1210/en.2012-1195
- Doshi T, D'Souza C, Dighe V, Vanage G. Effect of neonatal exposure on male rats to bisphenol A on the expression of DNA methylation machinery in the postimplantation embryo. J Biochem Mol Toxicol 2012; 26:337-43; PMID:22730197; http://dx.doi.org/10.1002/jbt.21425
- Liu Y, Duong W, Krawczyk C, Bretschneider N, Borbely G, Varshney M, Zinser C, Schar P, Ruegg J. Oestrogen receptor beta regulates epigenetic patterns at specific genomic loci through interaction with thymine DNA glycosylase. Epigenetics Chromatin 2016; 9:7; PMID:26889208; http://dx.doi.org/10.1186/s13072-016-0055-7