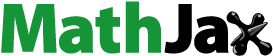
Abstract
In many real-world inventory deterioration concerns, the rate of deterioration of individual commodities is based on the deteriorating items during the storage period. Inventory models for degrading commodities have become more important in the food production sectors due to the unpredictability of product life spans. This deterioration can be overcome by involving a preservation factor to reduce the effects of decaying objects. This model is applicable for food production, in particular, dairy product (ice cream) suppliers to make inventory decisions in the inventory system under the effect of optimal values. In this paper, a preservative-based inventory model for deteriorating products with quality demand is discussed. The proposed model with cost functions yields the best operating plan and total sales. The policy combinations for dairy products are generally designed to reduce the deterioration rate, the expenditures, and the total cost. To illustrate the value of the proposed model numerical examples involving three flavours of ice creams have been studied. The model was tested with the real-time data obtained from the ice cream factory. Also, the proposed model’s numerical results are compared with the existing model. Further, the sensitivity analysis is performed to study the effect of changes in different parameters of the model. The complex algebraic equations are solved using MATLAB R2013a.
1. Introduction
Inventory management is an essential aspect of the trade. The objective of an inventory problem is to reduce the total cost of the inventory system by making the best decisions based on the mathematical methods that can be used to solve problems, such as optimum control analysis, dynamic programming, and network optimization. Generally, inventory decisions revolve around two questions: when should the inventory be renewed and how much order should be added to the inventory?
Inventory is being researched to see if it might help businesses save a lot of money. It is common practice in many firms to store inventory for future sale or use. To meet the demand, firms must have a supply of products on hand that can be sold quickly. The inventory theory objective is to identify the rules that management can use to reduce inventory costs and meet the demand from the market. To achieve an ideal total cost in an inventory model, most of the industrial organizations focus on inventory control and overcoming inventory challenges such as managing and maintaining inventory of degrading items.
In the year 1915, F.W. Harris (Harris, Citation1915) developed the standard Economic Order Quantity (EOQ) model known as Wilson’s square root formula. Several kinds of inventory models such as philip (philip, Citation1974), Mishra (Mishra, Citation1975), Rosenberg (Rosenberg, Citation1979), Park (Park, Citation1982), Mak (Mak, Citation1987), and Wee (Wee, Citation1989) have been proposed to obtain the reduction in overall cost based on the back orders model.
One of the most significant factors in inventory modeling management is deterioration. The term “deterioration“ refers to any damage, spoilage, dryness, vaporization, etc. The key issue with deterioration is preserving the product’s freshness till it reaches the customer’s end. Deterioration is one of essential parts of inventory (reduction of cost and maximise the profit) analysis. If the degrading objects are not removed from the system, it impacts the inventory system. Dave and Patel (Dave & Patel, Citation1981) developed another class of inventory model for the deterioration of items with time-proportional demand and no shortages. In the year 2006, Ouyang (Ouyang et al., Citation2006) introduced an inventory model for non-instantly deteriorating products. This model takes into account an acceptable delay in payments. Uthayakumar et al. (Uthayakumar & Geetha, Citation2009) proposed an inventory model in the direction of non-instantaneous deteriorating items in which the partial backlogging is considered. Hasan (Hasan et al., Citation2020) found an imperfect production method for deteriorating agricultural products.
The demand for a particular product varies according to the demand rate depending on stock availability, price of items, etc. Ghare and Scharader (Ghare & Schrader, Citation1963) developed the inventory model by taking into account the constant rate of demand, constant rate of depletion and without shortages. In recent years, Donaldson (Donaldson, Citation1977), Goyal (Goyal, Citation1986), Silver and Meal (Silver & Meal, Citation1969), Sana and Chaudhuri (Sana & Chaudhuri, Citation2008), and Khanra and Chaudhri (Khanra & Chaudhuri, Citation2003) have focused on different inventory models for aging goods in which the demand is usually presumed to be constant and is time-dependent or stock dependent. But both these factors work together for any product. Sindhuja et al. (Sindhuja et al., Citation2021) proposed a mathematical model based on quadratic demand and also considered time-dependent demand.
Few research works which focuses on both demand and deterioration. Wu et al. (Wu et al., Citation2000) proposed an inventory model in which inventory is decreased by demand and deterioration. An inventory model based on the deterioration and with random demand was developed by Bragila et al. (Braglia et al., Citation2019).
Perishable products have high and varying deterioration rates when passing through their logistics system. Few recent technical advancements includes preservation technology, supply chain technology and warehouse technology that have resulted in the development of new technologies that can be utilized to effectively measure and regulate the rate of deterioration. In practice, the deterioration rate of products can be controlled and reduced through various efforts such as operational changes and specialized equipment acquisition. By the efforts of investing in reducing the deterioration rate, the supplier can reduce the economic losses, improve the service level to the retailer, and increase business competitiveness.
Preservation technology is an essential component in minimizing the deteriorating effect. It may be used to measure and control the pace of deterioration effectively. In practice, the deterioration rate of products can be controlled and reduced through various efforts such as operational changes and specialized equipment acquisition. The supplier can reduce economic losses, improve the service level to the retailer, and increase business competitiveness by investing in preservation technology and lowering the deterioration rate.
In this context, several businesses and organizations are required to use preservation technology in their inventory management systems. Hsu et al. (Hsu et al., Citation2010) first introduced a model using preservation technology. An optimal replenishment policy for deteriorating items with effective investment in preservation technology was developed by Dye and Hsieh (Dye & Hsieh, Citation2012). Hsieh and Dye (Hsieh & Dye, Citation2013) derived a production model under time-dependent demand with a controllable deterioration effect. A deteriorating inventory model with preservation technology under stock dependent demand was developed by Zhang et al. (Zhang & Tang, Citation2014). Yang et al. (Yang et al., Citation2015) proposed a preservation inventory model under trade credit with a deterioration effect. A preservation inventory model under price-dependent demand with shortage was proposed by Mishra et al. (Mishra et al., Citation2017). Subhash Chandra Das et al. (Das et al., Citation2020) proposed a mathematical model based on an application of preservation technology which is followed by selling price dependent demand. Khanna et al. proposed a model based on time-varying holding cost under the investment of preservation technology. Carbon control policies by developing an optimal inventory model dealing with deteriorating items with preservation technology are developed by Khanna et al. Sustainable production strategies for deteriorating and imperfect quality items with an investment in preservation technology are presented by Priyamvada et al. Das et al. proposed an inventory model based on preservation technology for a non-instantaneous deteriorated inventory model considering multi-period trade credit policy and variable demand dependent on selling price. Mishra et al. (Mishra et al., Citation2017) proposed a model based on the preservation technology, this paper again revisited by Priyamvada et al.
2. Research gap and contributions
In this paper, an attempt has been made to develop an inventory model with a controllable deterioration rate by allowing preservation technology parameter as the most important decision variable in conjunction with the inventory system. The quality control deterioration rate by choosing the cost containment with preservation effort. The main objective of this model is to find the optimal time cycle length and preservation technology investment strategies while minimizing the total cost per unit time.
In Table , few research papers based on preservation technology are identified and compared with the proposed model.
Table 1. Comparisons of this model with previous works based on demand, deterioration, shortage
The report focuses on what sets the study apart from the literature:
Introducing the holding cost with controllable preservation and constant holding cost. Demand is related to the quality of the perishable commodity, which is determined by its rate of deterioration. The effect of deterioration on items, as well as preservation technology measures to control the rate of deterioration.
By applying the model, the supplier benefits at each point, decreasing the order quantity to decrease the production loss due to deterioration. Furthermore, the practitioner must choose not only the traditional order quantity decision, but also the quality control deterioration rate by choosing the following factors.
1) The depreciating worth of the goods
2) Cost containment with preservation effort
3) Quality-sensitive demand.
The rest of the paper is organized as follows. The research gap and contributions are discussed in Section 2. The description, notations, and assumptions are discussed in Section 3. In Section 4, a brief description of the existing model is explained. In Section 5, the proposed mathematical model is explained. The solution approach of the proposed model is explained in detail in Section 6. Numerical examples are illustrated in Section 7. The sensitivity analysis, which considers the impact of changes in a parameter on the inventory system’s decision variables, is discussed in Section 8. The conclusion and the scope for future work are discussed in Section 9.
3. Description, notations, and assumptions
In this section, the model’s description is explained and the components are elaborated under notations, and the assumptions are mentioned below as well.
3.1. Problem description
The policy of a dairy company, in particular, an ice cream factory, should be set so that the average overall cost is kept as low as possible. The perishable nature of materials presents a significant challenge, putting constraints on planning systems. The absence of freshness, especially noticeable in ice cream maintained in a stable environment, is one of the most considerable factors determining the pace of degradation. Additionally, ice cream can absorb volatile flavors from the storage environment. The cost of using modern preservation technologies, on the other hand, has been estimated because of the suggested model, the supplier benefits by determining an optimal time cycle duration strategy.
3.2. Notations
In this inventory management problem, the following notations are utilised.
• —represents the fixed cost of ordering.
• —is the deterioration rate.
• —is the preservation indicator.
• - is the constant holding cost.
• - is the actual controlled holding cost when it comes to preservation.
• -is the maximum preservation holding cost per unit and per time unit.
• - is the overall cost.
• The demand is related to the quality of the perishable commodity, which is influenced by its rate of deterioration.
• is fixed demand.
• -demand-on-quality variable.
• is the deterioration cost value per unit.
• is the shortage cost per unit.
3.3. Assumptions
The mathematical model proposed in this paper is based on the following assumptions.
• A single item is considered in this inventory system.
• A deterministic demand pattern is considered.
• The demand is dependent on the product quality. The quality of the perishable commodity, which in turn depends on its rate of deterioration, determines demand.
• Ordering cost is unchangeable. Assume that an order containing a specific type of preserved product has a higher ordering cost. In general, the initial freshness of each and every product ordered determines the retailer’s maximum effective preservation effort. The preservation benefits include the expense of adopting advanced preservation technologies that have been pre-allocated.
• The holding cost with preservation effort is directly related to the expected deterioration rate by allocating required personnel costs, equipment, and other operational expenditures.
• The rate of deterioration is quite low when a corporation makes every effort to preserve a product, such as . In this case, the highest level of product quality is maintained. Because preservation necessitates the use of storage devices, preservation equipment, higher power efficiency, and human labour, the holding cost is linked to the quality indicator.
• Shortages are allowed.
• Deteriorated items should not be replaced during the cycle period.
• Lead time is assumed to be zero.
4. Existing model
Subhash Chandra Das et al. (Das et al., Citation2020) proposed a mathematical model based on an application of preservation technology and selling price-dependent demand. Considering the instantaneous inventory level at any time
is determined by the following differential equations:
From the above equations, it is observed that is the inventory amount at any time “t” which is defined by the differential equations based on demand and deterioration.
The deterioration is considered by three-parameter Weibull distribution deterioration rate, i.e.,
5. Proposed mathematical model
In this section, a mathematical model is developed to minimize the total inventory cost under the condition of preservation technology with quality-dependent demand on time. The proposed model can be defined by the inventory level decreases due to demand and degradation of the goods over the period , according to the notations and assumptions indicated above. When the inventory level goes to zero at the time
the shortages begin and the inventory level continues throughout the period from
to
.
Considering the effect of the quality demand and the condition of preservation technology, under various conditions of deterioration rate, the order quantity varies. Each order cycle results in a decrease in the inventory level due to both demand and product deterioration. The inventory level at any point of time ,
can be expressed by the following differential equation:
=
The boundary conditions are and
. Solving the Equationequation (3)
(3)
(3) the inventory level can be expressed as
In Equationequation 3(3)
(3) by applying the boundary condition
,
is obtained as below
To apply in the preceding equation, use
as a condition, hence
is represented by
The solution of Equationequation (4)(4)
(4) is yielded below by applying the condition
is
Initial order quantity is
Ordering cost ””—makes up the overall relevant cost of each cycle.
Inventory holding cost in the system during the time intervals and the perishable inventory holding cost is listed below.
• The constant holding cost is comprises both physical storage and opportunity costs.
• - is the cost of freshness preservation, which is incurred to keep the storage state under control.
The number of units deteriorated is . Hence the deterioration cost is
During a cycle, the loss caused by a shortage, the stockout cost between the time intervals is given by
Given the limitations of preservation technology, the preservation technology investment cost is calculated as follows:
The total cost per cycle is
To resolve the approximation exponential function, Equationequation (13)(13)
(13) is obtained.
The total relevant cost can be reduced based on the following necessary conditions.
The optimal values of and
are obtained by simultaneously solving
6. Solution approach
Total cost analysis based on deduction of optimal cost in Equationequation (14)(14)
(14) is analyzed below.
6.1. Total cost analysis based on deduction of optimal cost
According to the optimality necessary condition, derive Equationequation (14)(14)
(14) with respect to
and
The optimal values of and
are obtained by solving simultaneously for
Since
Since
6.2. Solution procedure
In this section an approach to evaluate the inventory strategy that minimizes the overall cost per unit time has been described. In an inventory mathematical model, the essential things the ice cream supplier should consider are arranging downtime and ordering up time.
• The life span of the item is assumed as constant deterioration followed by a preservation factor, i.e., .
• Differential equations are used to calculate inventory’s real-time status. The initial condition and boundary condition are used to determine the inventory level. The inventory level at any point of time , Equationequation (5)
(5)
(5) represents
. The maximum stock level obtained from the initial condition is
=
. The initial order quantity is determined before the cost estimation.
• The supplier policies for various estimated costs are taken into account.
• From Equationequations (9)(9)
(9) , (Equation10
(10)
(10) ), (Equation11
(11)
(11) ) and (Equation12
(12)
(12) ) the estimated costs such as ordering, holding, deterioration, and shortage costs are obtained.
• From Equationequation (9)(9)
(9) , the actual controllable holding cost with preservation is represented by
. The holding cost with preservation effort directly results in the expected deterioration rate by allocating required labour cost, equipment, and other operational expenditures.
• For each unit and per time unit, is the maximum holding cost with preservation. The rate of deterioration is relatively low when a company makes every effort to preserve a product, such as
. In this case, the highest level of product quality is maintained. Because preservation necessitates the use of storage devices, preservation equipment, higher power consumption, and human labor, the holding cost is linked to the quality indicator.
• In this model, the total cost is composed per cycle denoted as which is defined as
, where
,
,
,
are considered as ordering cost, holding cost, shortage cost, deterioration cost, and preservation cost.
• To obtain the perishable inventory’s optimal policies, the model considers the function of time with the total cost per unit time. Differentiating Equationequation (13)(13)
(13) with respect to
and
, the optimal values are obtained. Taking the differentiation for Equationequation (13)
(13)
(13) with respect to
and
, the optimal values of
and
are obtained.
• Thus the cost variation differs for each flavour of the ice cream which is considered in the case study.
6.3. Solution algorithm
• Step 1: Set the parameters ,
,
,
,
,
,
,
.
• Step 2: The following costs compose total cost: the ordering cost, carrying cost, stockout cost, deterioration cost. The total cost per cycle is defined as
,which is expressed in Equationequation (14)
(14)
(14) .
• Step 3: Taking the first and the second order partial differentiation for Equationequation (13)(13)
(13) with respect to
and
, the equations
and
are obtained.
• Step 4: Simultaneously solving the equations and
, the optimal (minimum) values
and
are obtained.
• Step 5: From Equationequations (15)(15)
(15) and (Equation16
(16)
(16) ) the conditions of first order to have a minimum value should be satisfied.
• Step 6: The Equationequation (17)(17)
(17) , i.e.
, satisfies the efficient inventory policy.
• Step 7: Set the particular value .
• Step 8: Set the parameters ,
,
,
,
,
,
,
along with the values of
and
in Equationequation (13)
(13)
(13) .
• Step 9: Evaluate the total cost .
• Step 10: Construct the optimal total cost .
• Step 11: Evaluate the optimal total cost and corresponding optimal value of
,
,
.
7. Numerical example
In this section, numerical values based on the cost variation in the real-time data from Dairy Fresh Ice Cream-Chennai 600020, Tamil Nadu, India, are used to represent the numerical outcomes in Examples 1, 2, and 3. The proposed model is tested with existing model (Subhash Chandra Das et al. (Das et al., Citation2020)) in Example 4.
7.1. Example 1
Considering the parametric values of the inventory model of deteriorating items in proper units based on the real-time data from ice cream company, the cost variation for vanilla ice cream such as =12,000,
=2000,
=2000,
=0.001,
=0.001,
=0.002,
=1500,
=1200,
=150. The values of
=0.75,
=0.81,
= 2000,
= 12,460.
Note: The constant holding cost () for vanilla flavored ice cream is calculated only based on the amount spent on milk, skimmed milk powder, and sugar. The controlled holding cost (
) is calculated by assigning labour, equipment, and operational costs.
7.2. Example 2
Considering the parametric values of the inventory model of deteriorating items in proper units based on the real-time data from the ice cream company, the cost variation for strawberry ice cream such as =12,800,
=2500,
=3600,
=0.001,
=0.001,
=0.002,
=1500,
=1200,
=150. The values of
= 0.78,
=0.82,
= 2750,
= 12,985.
Note: The constant holding cost () for strawberry flavor ice cream is calculated based on the amount spent on milk, skimmed milk powder, sugar, artificial colour, and strawberry fruit. The controlled holding cost (
) is calculated by assigning labour, equipment, and operational costs.
7.3. Example 3
Considering the parametric values of the inventory model of deteriorating items in proper units based on the real-time data from ice cream company, the cost variation for butterscotch ice cream such as =13,800,
=2800,
=3700,
=0.001,
=0.001,
=0.002,
=1500,
=1200,
=150. The values of
= 0.80,
=0.87,
= 3750,
= 13,995.
Note: The constant holding cost () of the ice cream is calculated using the quantities spent on milk, skim milk powder, sugar, caramel balls, and nuts. The controlled holding cost (
) is calculated by assigning labor, equipment, and operational costs.
Variations in the time of positive inventory, the time cycle, the initial ordering amount, and the total cost are discussed in Figure for all three flavours. This indicates that the total cost value and initial order quantity required values are minimized in all circumstances.
7.4. Example 4
The parametric values of the inventory model of deteriorating items in proper units from Subhash Chandra Das et al. (Das et al., Citation2020) are
=150,
=6,
=3,
=0.21,
=0.001,
=0.3,
=16,
=25,
=15,
=0.3 and the total cost obtained from the existing model and the proposed model is given in Table and represented graphically in Figure .
Figure 2. Comparison of Subhash Chandra Das et al. (Das et al., Citation2020) and proposed model.

Table 2. Comparison of the results
From Table , the comparative results of ,
,
,
on applying Das et al. and the proposed model are discussed. It is observed that the initial stock requirement
is higher in the proposed model when compared to the existing model. Also, the optimal time cycle values
,
are less in the proposed model when compared to the existing model, it is also observed that there is a greater reduction in the total cost
in the proposed model.
8. Sensitivity analysis
An illustration of the proposed model is studied by varying the parameters such as ,
,
,
,
,
,
,
and
in the above examples (Figures ). This leads to a change in the near optimal values of
,
,
and
.
The findings are determined by changing one parameter at a time while maintaining the original values of the other parameters. Table shows the outcomes of these analyses based on numerical example 1.
Table 3. Sensitivity analysis of ,
,
,
,
,
,
,
based on the cost variation for vannila ice cream
Based on the cost variation obtained from numerical example 2, Table illustrates the sensitivity analysis of ,
,
,
,
,
,
,
.
Table 4. Sensitivity analysis of ,
,
,
,
,
,
,
based on the cost variation for strawberry ice cream
Based on the cost variation obtained from numerical example 3, Table illustrates the sensitivity analysis of ,
,
,
,
,
,
,
.
Table 5. Sensitivity analysis of ,
,
,
,
,
,
,
and
based on the cost variation for butterscotch ice cream
Based on the parametric values obtained from the existing model illustrated in numerical example 4, Table depicts the sensitivity analysis of ,
,
,
,
,
,
,
.
Table 6. Sensitivity analysis of ,
,
,
,
,
,
,
based on the parametric values obtained from the existing model
8.1. Sensitivity analysis with graphical representation
The graphical representations corresponding to Table are summarized as follows.
8.2. Observation and the managerial implications
Based on numerical results the following management phenomena are obtained from examples 1, 2,3,4 in Section 7.
Based on the influence of the optimal values in the inventory system, the supplier can raise the order size (or) the time cycle duration to reduce costs. The proposed EOQ model will allow the supplier to increase the efficiency of maintaining the initial stock order, assure fresh stock availability in the inventory system and reduce the percentage of lost stock. The proposed model can be widely used in analyzing situations that arise in the areas such as those dealing with food processing units, particularly dairy products (ice cream). As a result, a significant contribution to the company is to supply ice cream to its distributors in determining the optimal time to increase profit.
• The time of positive inventory and the time cycle have a positive correlation with the ordering cost().
and
shows a positive correlation with parameter
.
• The time of positive inventory and the time cycle have a negative correlation with the controllable holding cost () and maximum holding cost (
).
shows a negative correlation with (
) and (
).
exhibits a negative correlation with (
) and (
).
• The time of positive inventory and time cycle have a negative correlation with .
shows a negative correlation while
exhibits a positive correlation with
.
• The time of positive inventory and time cycle have a negative correlation with .
and
shows a negative correlation with
.
• The time of positive inventory and time cycle have a positive correlation with purchase cost .
shows a negative correlation as well as
exhibits a negative correlation with
.
• The time of positive inventory have a negative correlation with and the time cycle has a negative correlation with
.
and
shows a positive correlation with
.
• The preservation parameter has a negative correlation with the time of positive inventory and time cycle.
and
shows a negative correlation with
.
9. Conclusion
A mathematical model for deteriorating items based on preservation technology with time-dependent quality demand under shortages has been developed in this paper. The rate of deterioration impacts dairy food products, which increases with time. The cost functions applied in the proposed model benefit the supplier in deciding the optimum operating plan and total sales. Hence, the preservation technology with time-dependent quality demand can be widely used in analyzing situations that arise in the food processing, particularly dairy products (ice cream). After evaluating the model, this proposed model helps the supplier of the company to reduce total costs in terms of the deterioration rate governed by the deteriorating parameter () by reducing order size (or) cycle time.
However, in many realistic circumstances emerging in the food processing industry’s, the commodity’s life cycle is unpredictable and depends on time. It is also noted that the commodity’s life cycle has a finite upper bound and that the rate of decay is proportional to time. When the total cost value of the three varieties of ice cream flavours is examined, the amount of deterioration cost and maximum holding cost are related to product freshness preservation are significant considerations for dairy products (ice cream).
The company reduces its order quantity to inventory loss due to deterioration at each stage. This indicates that the total cost value and beginning order quantity required values are minimised in all circumstances. The proposed model is determined using the quality demand based on the controllable holding cost () and maximum holding cost (
). The significance of the above two parameters enables the supplier to increase the efficiency of maintaining the initial stock order, assure fresh stock availability in the inventory system, and reduce the percentage of lost stock. Thus, the proposed model helps the distributors recover from equipment failure, ice crystal formation, and power factor.
Future research can be extended to trade credit, where advance payment is considered. The proposed inventory model can be supported with a production-inventory model.
Disclosure statement
No potential conflict of interest was reported by the author(s).
Additional information
Funding
Notes on contributors

S. Sindhuja
S.Sindhuja is a Research Scholar in the Department of Mathematics and Actuarial Sciences, B.S.Abdur Rahman Crescent Institute of Science & Technology, India. She obtained her M.Sc from Meenakshi College for Women, Chennai, India. Her research area includes Mathematical Modeling and Inventory Control.
P. Arathi
P.Arathi is an Assistant Professor in the Department of Mathematics in B.S.Abdur Rahman Crescent Institute of Science and Technology, India. She has been serving in the department as a faculty since July 2012. She completed her doctorate degree in the year 2013. Her research area includes Numerical Linear Algebra, Graph theory and Inventory.
References
- Braglia, M., Castellano, D., Marrazzini, L., & Song, D. (2019). A continuous review, (Q, r) inventory model for a deteriorating item with random demand and positive lead time. Computers & Operations Research, 109, 102–25. https://doi.org/10.1016/j.cor.2019.04.019
- Das, S. C., Zidan, A. M., Manna, A. K., Shaikh, A. A., & Bhunia, A. K. (2020). An application of preservation technology in inventory control system with price dependent demand and partial backlogging. Alexandria Engineering Journal, 59(3), 1359–1369. https://doi.org/10.1016/j.aej.2020.03.006
- Dave, U., & Patel, L. K. (1981). (T, Si) policy inventory model for deteriorating items with time proportional demand. Journal of the Operational Research Society, 32(2), 137–142. https://doi.org/10.1057/jors.1981.27
- Donaldson, W. A. (1977). Inventory replenishment policy for a linear trend in demand: An analytical solution. Operations Research Quarterly, 28(3), 663–670. https://doi.org/10.1057/jors.1977.142
- Dye, C. Y., & Hsieh, T. P. (2012). An optimal replenishment policy for deteriorating items with effective investment in preservation technology. European Journal of Operational Research, 218(1), 106–112. https://doi.org/10.1016/j.ejor.2011.10.016
- Ghare, P. N., & Schrader, G. F. (1963). A model for exponentially decaying inventories. The Journal of Industrial Engineering, 15(5), 238–243.
- Goyal, S. K. (1986). On improving replenishment policies for linear trend in demand. Engineering Costs and Production Economics, 10(1), 73–76. https://doi.org/10.1016/0167-188X(86)90022-4
- Harris, F. W. (1915). Operations and cost, factory management. Production and Operation Management, 5(1), 1–6.
- Hasan, R., Mashud, A. H. M., Daryanto, Y., & Wee, H. M. (2020). A non- instantaneous inventory model of agricultural products considering deteriorating impacts and pricing policies. Kybernetes. http://dx.doi.org/10.1108/K-05-2020-0288
- Hsieh, T. P., & Dye, C. Y. (2013). A production inventory model incorporating the effect of preservation technology investment when demand is fluctuating with time. Journal of Computational and Applied Mathematics, 239, 25–36. https://doi.org/10.1016/j.cam.2012.09.016
- Hsu, P. H., Wee, H. M., & Teng, H. M. (2010). Preservation technology investment for deteriorating inventory. International Journal of Production Economics, 124(2), 388–394. https://doi.org/10.1016/j.ijpe.2009.11.034
- Khanra, S., & Chaudhuri, K. S. (2003). A note on an order level inventory model for a deteriorating item with time dependent quadratic demand. Computer and Operations Research, 30(12), 1901–1916. https://doi.org/10.1016/S0305-0548(02)00113-2
- Mak, K. L. (1987). Determining optimal production-inventory control policies for an inventory system with partial backlogging. Computers & Operations Research, 14(4), 299–304. https://doi.org/10.1016/0305-0548(87)90067-0
- Mishra, R. B. (1975). Optimum production lot size model for a system with deteriorating inventory. International Journal of Production Research, 13(5), 495–505. https://doi.org/10.1080/00207547508943019
- Mishra, U., Cardenas-Barron, L. E., Tiwari, S., Shaikh, A. A., & Trevino- Garza, G. (2017). An inventory model under price and stock dependent demand for controllable deterioration rate with shortages and preservation technology investment. Annals of Operations Research, 254(1–2), 165–190. https://doi.org/10.1007/s10479-017-2419-1
- Ouyang, L. Y., Wu, K. S., & Yang, C. T. (2006). A study on an inventory model for non-instantaneous deteriorating items with permissible delay in payments. Computers & Industrial Engineering, 51(4), 637–651. https://doi.org/10.1016/j.cie.2006.07.012
- Park, K. S. (1982). Inventory model with partial backorders. International Journal of Systems Science, 13(12), 1313–1317. https://doi.org/10.1080/00207728208926430
- philip, G. C. (1974). A generalized EOQ model for items with Weibull distribution deterioration, AIIE Transaction. AIIE Transaction, 6(2), 159–162. https://doi.org/10.1080/05695557408974948
- Rosenberg, D. (1979). A new analysis of a lot-size model with partial backlogging. Naval Research Logistics Quarterly, 26(2), 349–353. https://doi.org/10.1002/nav.3800260214
- Sana, S., & Chaudhuri, K. S. (2008). A deterministic EOQ model with delays in payments and price-discount offer. European Journal of Operational Research, 184(2), 509–533. https://doi.org/10.1016/j.ejor.2006.11.023
- Silver, E. A., & Meal, H. C. (1969). A simple modification of the EOQ for the case of varying demand rate. Production and Inventory Control, 10(4), 52–65.
- Sindhuja, S., Arathi, P., & Mohan, V. (2021). A quadratic demand eoq model for deteriorating items with time dependent shortage. International Journal of Mathematics in Operational Research, 20(1), 41–59. https://doi.org/10.1504/IJMOR.2021.117636
- Uthayakumar, R., & Geetha, K. V. (2009). A replenishment policy for non- instantaneous deteriorating inventory system with partial backlogging. Tamsui Oxford Journal of Mathematical Sciences, 25(3), 313–322.
- Wee, H. M. (1989). Optimal inventory policy with partial backordering optimal control applications and methods. Optimal Control Applications & Methods, 10(2), 181–187. https://doi.org/10.1002/oca.4660100208
- Wu, J., Lin, C. H., Tran, B., & Lee, W. C. (2000). An EOQ inventory model with time varying demand and weibull deterioration with shortages. International Journal of Systems Science, 31(10), 663–683. https://doi.org/10.1080/00207720050030716
- Yang, C. T., Dye, C. Y., & Ding, J. F. (2015). Optimal dynamic trade credit and preservation technology allocation for a deteriorating inventory model. Computers & Industrial Engineering, 87, 356–369. https://doi.org/10.1016/j.cie.2015.05.027
- Zhang, Z. B., & Tang, W. (2014). Optimal pricing policy for deteriorating items with preservation technology investment. Journal of Industrial & Management Optimization, 10(4), 1261–1277. https://doi.org/10.3934/jimo.2014.10.1261