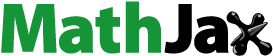
Abstract
This study proposes a new active direct evaporative cooling system that uses a bundle of cotton fibers as an evaporating surface and activated carbon as a moisture adsorbent. A two-stage evaporative cooling experimental setup consisting of an activated carbon adsorbent in between the cooling pad was designed, fabricated, and tested at Bahir Dar, Ethiopia. The fiber bundle (pad 1) was wetted with water and ambient air was forced through it using a fan then the air that exiting from pad 1 was forced through a bulk of activated carbon (moisture adsorbent), and then the air from the adsorbent passed through the second wetted fiber bundle (pad 2). The energy source for the fan and pump was the solar photovoltaic energy system. The average temperature drop of the cooler without the moisture adsorber was 9.33°C and with the inclusion of the moisture, the adsorber gave an additional temperature drop of 1.5°C to 4.95°C. During the hot dry season, the average relative humidity of the ambient air, pad 1 outlet, adsorbent outlet, and pad 2 outlets were 28.26%, 76.12%, 55%, and 88.2% respectively. The cooler with moisture adsorbent had a cooling capacity of 3653 W, an effectiveness of 94.25%, and a COP of 52.2. The developed solar evaporative cooling system can provide energy-efficient, sustainable, affordable cold air for different applications. The cooler could be used by small-scale farmers, wholesalers, and retailers for the cooling and storage of horticultural products to reduce the postharvest loss in fresh produces.
PUBLIC INTEREST STATEMENT
These work is focused on designing, developing and testing of solar-powered evaporative cooling systems. The developed evaporative cooler is environmentally friendly, has green technology, and is affordable for small-scale farmers. It uses water as a refrigerant, solar energy as the energy source, and uses local materials. It was developed with new materials, using locally grown cotton as a raw material which increases the air-cooling performance of the cooler. The result revealed that the cooler has an improved cooling performance. The cooler could be used by small-scale and cooperative farmers, wholesalers, and retailers for the cooling and storage of horticultural products such as tomatoes to reduce the postharvest loss.
1. Introduction
Evaporative cooling is an air conditioning system that works with water as a working fluid (Fikri et al., Citation2020). Such type of cooling is an ancient technology that has been used since pre-scientific eras to cool buildings about 2500 B.C. in Egypt. Over the years, evaporative cooling systems have evolved gradually from their primitive forms to advanced systems (Alamdari et al., Citation2020). It can be implemented by spraying water into the air or by using a humid surface called an evaporative cooling pad. The wet pad enables the mass and heat transfer between water and air (Tejero-González & Franco-Salas, Citation2021). An Evaporative cooling system is a good alternative to a vapor compression air conditioning system. Unlike vapor compression air conditioning, an evaporative cooling system can be driven by low-grade energy sources like solar, not releasing gases that deplete the ozone layer and acting as a climate-smart technology [Dhamneya et al., Citation2018]. Evaporative cooling systems can be classified as indirect, direct, and combined with direct-indirect evaporative cooling (Dhamneya et al., Citation2018). The direct evaporative cooler (DEC) is an old, efficient, and widely utilized technology. It is best suited to a hot and arid climate (Kashyap et al., Citation2021). The potential for direct evaporative cooling is measured by the wet-bulb temperature in comparison to the inlet air dry-bulb temperature (Lal Basediya et al., Citation2013). The working principle of the direct evaporative cooling process is based on the cooling of water in process of its evaporation and consequently a rejection of latent heat. To carry out such types of cooling an immediate contact of air and water is needed through wet media (Fouda & Melikyan, Citation2011). The sensible heat of the air is converted into latent heat in an adiabatic process as the water evaporates in the air (Velasco-Gómez et al., Citation2020). The degree of cooling increases with the evaporation rate (Zakari et al., Citation2016).
The performance of an evaporative air conditioning process is highly dependent on the type of evaporative cooling pad materials used. The pads can be classified according to their material and configuration in packages, rigid media, and fiber pads (Tejero-González & Franco-Salas, Citation2021). Different researchers have worked on different cooling media/pad materials to develop an effective evaporative cooling system (Dhamneya et al., Citation2018). A wide-ranging study has been done on the use of alternative materials for DEC media. Pads made of locally available vegetable fibers are the main subject of most research. The most widely used pad materials with varying cooling efficiency are PVC, sponge, fabric, cellulose pad, metal pad, coir fiber, palm fiber, jute, and luffa (Fouda & Melikyan, Citation2011; Lal Basediya et al., Citation2013; Velasco-Gómez et al., Citation2020).
The evaporative cooling is affected by environmental ambient factors such as air temperature, relative humidity (Abdel-Fadeel & Attalla, Citation2014), air velocity, and pad thickness. In the case of extreme ambient air which is saturated with water vapor, no evaporation can take place and no cooling occurs [15]. Greater cooling occurs in very dry air because it can absorb a lot of moisture (Lal Basediya et al., Citation2013). The performance of DEC improves as air temperature increases and humidity decreases (Amer et al., Citation2015). Saturation efficiency and cooling capacity of direct evaporative cooling are improved by increasing the contact surface area between air and water (Abdel-Fadeel & Attalla, Citation2014; Fouda & Melikyan, Citation2011) and by lowering the humidity of the cooling air in case of humid air. The larger the wet surface area the higher the rate of evaporation, resulting in high saturation efficiency (Dhamneya et al., Citation2018). Evaporative coolers with better evaporative surface area and water-absorbing material can improve their performance of evaporative coolers (Xuan et al., Citation2012).
Porous materials are commonly employed as water vapor adsorbents, with a wide range of uses depending on pore size (Okada et al., Citation2010). Air can be dehumidified in an adsorption process by using adsorbent materials that improve evaporative cooling performance (Zheng et al., Citation2014). Therefore, increasing the adsorption capacity of the composite adsorbent can significantly raise the coefficient of performance (COP) value (Tso & Chao, Citation2012). The most common approach for increasing water vapor adsorption capacity is the impregnation of hygroscopic salt on the surface of activated carbon (Lal Basediya et al., Citation2013; Liao & Chiu, Citation2002; Ndukwu & Manuwa, Citation2014; Zakari et al., Citation2016). Evaporative cooling technology can be used in a wide range of public, commercial, industrial, and agricultural buildings, and for the storage of fruits and vegetables (Xuan et al., Citation2012).
Studies have reported a significant postharvest loss of fruits and vegetable products (as high as 50 %) in developing countries, such as Ethiopia (Kasso & Bekele, Citation2016; Sipho & Tilahun, Citation2020). One of the main causes of the commonly observed high postharvest loss in developing countries is the lack of sustainable and affordable postharvest handling systems. Pre-cooling and cold storage systems are critical postharvest handling systems that can minimize this huge postharvest loss of fresh produces (Makule & Dimoso, Citation2022).To overcome such challenges solar powered evaporative cooling system is affordable, energy-efficient, and sustainable for cooling and storage of horticultural products (Lal Basediya et al., Citation2013).
As can be deduced from the literature survey, research is in progress to develop new cooling pad materials for enhancing the cooling potential and saturation efficiency of Evaporative cooling systems. The most widely investigated cooling pad fiber materials for EC systems are Metal (Dmitriev et al., Citation2021), coconut coir (Alam et al., Citation2017; Maurya et al., Citation2014), eucalyptus fiber (Laknizi et al., Citation2021; Tejero-González & Franco-Salas, Citation2021), palash fiber (Maurya et al., Citation2014), jute fiber (Alam et al., Citation2017), luffa (Velasco-Gómez et al., Citation2020), wood, plastic, ceramics (Dmitriev et al., Citation2021), CELdek (Kumar et al., Citation2021), cellulosic pad (Tejero-González & Franco-Salas, Citation2021), aspen pad (Maurya et al., Citation2014). Despite their good wicking ability and water-holding capacity (Velasco-Gómez et al., Citation2020),], there is a dearth of information from the literature on the use of textile fibers for evaporative cooling.
The existing direct evaporative cooling system has low saturation efficiency. Therefore, the present work proposes a multi-stage evaporative cooling system with a new evaporative cooling bundle pad made of cotton fiber. The aim of this study focused on the design, development, and experimental testing of a low-cost solar-powered evaporative cooling system using a cotton fiber bundle as a cooling pad. The current fiber-based cooling pad is differing from the research already studied by previous researchers. The cooling efficiency of the evaporative cooling was enhanced by charcoal impregnated with calcium chloride as an air dehumidification system.
2. Materials and method
2.1. Fabrication of the solar evaporative cooler
The fabricated size of the cooler was 100 cm in length, 35 cm in width, and 35 cm in height. The size of the wet media was rectangular with a dimension of 35 cm*35 cm*15 cm in height, length, and depth respectively. The cooler had internal and external parts. The internal frames supported the bundle of cotton fiber (cooling media) and the adsorbent. The adsorbent was packed with mesh wire. The external frame was covered with a 0.9 mm thick metal sheet (Figure ). The fabricated coolers had two cooling pads and adsorbent bed sections. There was two water reservoirs section. The top storage was perforated with a diameter of 0.3 cm. Each spanned cotton fiber with a diameter of 0.3 cm and length of 15 cm was plugged into the perforated metal bucket and connected to the bottom reservoir. Due to the good wicking ability of cotton fiber, the water was flowing from top to bottom by a combination of capillary-gravitational force. In both cases, the thickness of the cooling pad was 15 cm.
2.2. Experimental setup and Instrumentation
The experimental setup used in this study was as shown in (Figure ). A study was carried out in April and September 2021 at the Faculty of Chemical and Food Engineering, Bahir Dar Institute of Technology, Bahir Dar University, Bahir Dar, Ethiopia (lat.11° 34’ 27.1524” N, long.37° 21’ 40.8708” E). We preferred April and September to test the performance of the proposed evaporative cooling system because April is the hottest and driest month and September is the most humid month. During the study, the daily temperatures and relative humidity in April were (Temp; 21.5–33.5°C and RH; 24%—35%) and in September (Temp; 21.5–25°C and RH; 24%—64%). The two cooling pads, moisture adsorbent, air fan, water circuit, and solar panel are the primary components of the experimental test setup. The test rig also includes measuring instruments for air temperatures, relative humidity, and velocity. The axial fan was located at the entrance of the cooler and each stage was equipped with a HOBO data logger for temperature and relative humidity (RH) measurement. There are two loops in the test rig: an air loop and a water loop. In the air loop, the atmospheric air was forced to the cooler pads and the adsorbent by a fan (40 Watt Ventilator Exhaust Fan, 220 Volt Ac, size (350 mm×350 mm)). On the other hand, in the water loop; the water was circulated through evaporative cooling pads using a pump (50 Watt Leo Group Pump (Zhejiang) Co., Ltd). The adsorbent was used to dehumidify the humid air. A solar panel (Monocrystalline solar panel, maximum power 2*100 W, temperature range (−40°C—90°C), dimension 1062*631*36 mm) was used as a source of energy for the fan and pump. Different instruments were used during the experimental testing of the developed evaporative cooler as shown in (Figure ). Temperature and humidity data loggers were used to measure the air temperature and relative humidity at each stage of the cooler respectively. The Testo 405i velocity hot-wire anemometer was used to measure air velocity. The instruments with their accuracy are listed in Table .
Table 1. Characteristics of Instruments
2.3. Uncertainty Analysis
The errors and uncertainties of the experimental results were determined. The uncertainties of temperature and air velocity cause uncertainty of cooling capacity (Q) and the uncertainty of relative humidity (wet-bulb temperature) and dry-bulb temperature causes uncertainty of saturation efficiency (SE). The uncertainty of the calculated parameter was determined by EquationEq. (2)(2)
(2) : according to (Doğramacı & Aydın, Citation2020; John, R. Taylor Citation2015).
Where; represents the overall uncertainty of the calculated parameter such as cooling capacity and saturation efficiency and (T, RH, and V) are the set of parameters that are directly measured (temperature, relative humidity, and velocity) during experiments. The overall uncertainties of the measurements of velocity, relative humidity, and temperature as well as the uncertainty of the calculated cooling capacity and saturation efficiency are shown in Table .
Table 2. Error analysis
2.4. Composite adsorbent preparation
Eucalyptus wood charcoal was treated with hygroscopic salt (calcium chloride) to improve the dehumidification performance of air. To incorporate CaCl2 in the charcoal the following procedure was carried out; the charcoal was crushed and washed with distilled water. The washed charcoal was dried in an oven for 24 h at 105°C. Calcium chloride was dissolved in distilled water to obtain a solution of calcium chloride. The oven-dried charcoal was soaked in a 15%wt. calcium chloride solution at ambient temperature for 4 h according to (Solomon et al., Citation2013). Finally, calcium chloride-impregnated charcoal was dried in the oven.
2.5. Water holding capacity determination
The water adsorption capacity of cotton fiber was determined according to the following methods. The fiber sample was oven-dried at 105°C for 6 h. The dry weight of the samples was 0.839, 0.654, and 0.7887 g. The dried weighted fiber sample was soaked in water for 5 minutes at room temperature. After that, the sample was soaked out and hung for 3 minutes to remove excess water according to (Cruz et al., Citation2017). Finally, the weight of wet fiber was weighed by using digital mass balance. The percentage of water take-up was calculated by (EquationEq.3)(3)
(3) according to (Das et al., Citation2007).
2.6. Performance analysis of air cooler
The performance of the developed evaporative cooler was evaluated in terms of the following parameters:
2.6.1. Saturation efficiency of the cooler
The saturation efficiency of the evaporative cooler was calculated by using (; Okada et al., Citation2010; Tso & Chao, Citation2012; Xuan et al., Citation2012; Zheng et al., Citation2014).
2.6.2. Cooling capacity of the cooler
The cooling capacity (Q)of the evaporative cooler was determined by using (; Budaev et al., Citation2019; Yu et al., Citation2019; Zheng et al., Citation2014).
The specific heat capacity of air was calculated using (Liao et al., Citation2008).
The mass flow rate of air can be defined as (Laknizi et al., Citation2019):
The density of air can define as (; Delele et al., Citation2013).
2.6.3. Coefficient of performance
The coefficient of performance of the developed evaporative cooler was defined as the ratio of cooling capacity to the electric power consumption of the air fan and calculated by (; Kasso & Bekele, Citation2016; Makule & Dimoso, Citation2022; Yu et al., Citation2019) and presented as:
3. Result and discussion
3.1. Water take-up capacity of cotton fiber
In this study, the average capacity for water absorption of the cotton fiber was found 3.126 g/g. Cotton fiber has better water take-up capacity compared to other existing cooling pads such as Jute (2.1 g/g), charcoal (0.5 g/g), wood shaving (2.5 g/g; Manuwa & Odey, Citation2012), Jute (1.76 g/g) and Hessian (2.54 g/g; Olosunde et al., Citation2009), cellulose pad, metal pad, PVC pad, aspen, rice straw, and vermiculites. This is owing to its superior capillary diffusion and wicking capabilities (Ndukwu & Manuwa, Citation2014). Cotton satisfies one of the most important features of a cooling pad which is the ability to hold water. Cotton fiber can absorb water three times its dry weight, as depicted in the result. Therefore textile materials such as cotton fibers are a good cooling pad materials for direct evaporative cooling. This is because these materials have a good wicking ability; this enhances the heat and mass transfer resulting in a good cooling capacity.
3.2. Supply air temperature of a cooler
The supply (outlet) temperature of the air decreased as the air passed through the pad/bundle of the cotton fibers as depicted in (Figure ). The temperature reduction was due to the sensible heat transferred from the air to the water-saturated pad for evaporation of water. During the experimental testing (in April 2021), the daily average inlet air temperature was 31.23°C. The average outlet temperature of pad 1 was 21.92°C, and the outlet temperature of the air that passed through the adsorbent and pad 2 was in the range of 17°C to 20.42°C (an average drop of 12.19°C; Figure ). The temperature drop across pad 1 was 9.33°C and pad 2 was in the range of 4.95°C to 1.5°C. This cold air can be used to cool tomato produce. The supply air temperature was colder when the air passed through the moisture adsorbent before it passed through Pad 2. The adsorbent can reduce the latent load (relative humidity) of air that exits from pad 1. The lowest supply air temperature of pad 2 at the beginning of cooling was due to the presence of a higher number of active adsorbent sites. With time the adsorbent becomes saturated with water vapor and after 150 min and there was no dehumidification (Figure ). Then the temperature drop decreased over time.
Figure 4. The relative humidity of the Multi-stage Evaporative cooler: (a) in April and (c) in September.

The average daily temperature in April (Figure ) and September (Figure ) was 31.23°C and 25.53°C, respectively.
The lower outlet air temperature of the cooler was obtained at the initial cooling before the saturation of adsorbent with moisture; it was 17°C in April and 18.7°C in September as depicted (Figure ). Compared with September, in April the cooler leads to the lower outlet temperature this is because of the hot and dry season provides favorable for direct evaporative cooling. The cooler better performed in a hot and arid climate.
The results from the current study were compared with other similar previous studies. The highest temperature drop for the CELdek 7090 cooling pad was 9.5°C with an inlet temperature of 37°C, air velocity of 1 m/s, and with cooling pad thickness of 100 mm (Laknizi et al., Citation2019). With a cellulose-based cooling pad, the average temperature drop was 5.62°C at 1 m/s air velocity (Daǧtekin et al., Citation2011). Khater obtained an outlet air temperature of 25.73°C and 28.2°C under 1.5 m/s and 6 m/s air velocity, respectively, from an ambient temperature of 30°C (Khater, Citation2014). The temperature reduction of other existing cooling materials are 6.38°C (CELdek), 3.38°C (Shading net), and 5.98°C (volcanic tuff) with a velocity of 1.6 m/s and thickness of 15 cm (Gunhan et al., Citation2007), 11.3°C (eucalyptus fibers; Abohorlu Doğramacı et al., Citation2019). Compared with previous research work as discussed above, the cooling effect in the current study was higher; this was due to the characteristics of higher water-holding capacity of the cotton yarn, the increase in total evaporation surface area as the sum of the individual yarn area, and adsorption of the water vapor from the air by adsorbent reduce the saturation effect (latent load).
3.3. Air humidification
The pads and adsorbent humidified and dehumidified the flowing air, respectively. As depicted in (Figure ), both pad 1 and pad 2 increased the humidity of air; this was due to the evaporation of water from the surface of water-saturated pads to the flowing air. On the contrary, the adsorbent caused dehumidification of the humid air which exited from pad 1.
The average relative humidity of ambient air was 28.26% and 58.14%, pad 1 outlet 76.12% and 90.1%, adsorbent outlet 55% and 73%, and pad 2 outlet 88.2% and 92.3% in April (Figure ) and September (Figure ) respectively. The relative humidity drop across the adsorbent was 20.4% and 18.5% at the initial of the air cooling in April and September, respectively. In both, the dehumidification capacity of the adsorbent decreased with time. The increase of water vapor concentration in adsorbents decreased the driving force for mass transfer which decreased the adsorption process and the adsorbent’s active space becomes saturated with time. The adsorbent saturation time in April was longer than in September. This is because the latent load of pad 1 outlet air was lower in April than in September. The water vapor saturation efficiency of the cooler in the current study is reduced by the presence of an adsorbent in between the two cooling pads. Therefore the presence of water vapor adsorbent can increase the cooling performance of the cooler by reducing the latent load of the air exiting from pad 1.
3.4. Cooling capacity of the cooler
The cooling effect of a direct evaporative cooling pad is determined by the type of pad material and wetted surface area (Wijaksana et al., Citation2018). In the current study the cooling capacity of the developed evaporative cooling was calculated by (EquationEq.5)(5)
(5) . In April, its value was 3.653 kW under a frontal airflow rate of 0.245 m3/s. Compared with other cooling pad materials; cotton fiber bundles produced a relatively strong cooling capacity. The previous Studies revealed that the average cooling capacity of cellulose pad (4.54 kW), khus-grass (1.69 kW), and wood wool (2.84 kW) with a thickness of 10.16 cm, and an airflow rate of 0.35 m3/s (Khobragade & Dr, Citation2016), eucalyptus fibers (0.41 kW; Abohorlu Doğramacı et al., Citation2019). The cylindrical fiber that was used in this study had a relatively large wetted surface area which leads to an increase in the evaporation rate. The cooling capacity of the developed evaporative cooler agrees with other previous work.
3.5. Saturation efficiency analysis
The saturation efficiency of an evaporative cooler is directly related to the actual temperature drop. The saturation efficiency rises with an increase in temperature drop. The wet-bulb temperature was read from an air psychometric chart by using measured dry bulb temperature and relative humidity. In April, the developed multi-stage evaporative air conditioning system gave an average saturation efficiency of 94.25%. When compared to other previous studies; the average saturation efficiency with cellulose-based cooling pads was 86.45% at 1 m/s air velocity (Daǧtekin et al., Citation2011). At an airflow rate of 0.25 m3/s and a pad thickness of 10.16 cm, the saturation efficiency of a cellulose cooling pad was 92.8% (Khobragade et al., Citation2016). The efficiency of a new pad consisting of coarse and fine fabric PVC sponge mesh for 15 cm thickness was 86.3% and 85.5%, respectively (Liao & Chiu, Citation2002). At 10 m/s air velocity and 15 cm thickness, the fine pumice stones pad had a saturation efficiency of 93.1% (Gunhan et al., Citation2007). Coir material pads gave saturation efficiencies of 89% for a frontal air velocity of 2 m/s and 15 cm pad thickness (Liao et al., Citation2008), saturation efficiency of other existing pad materials are wood wool (87.5%), eucalyptus fiber (70%), stainless steel wire mesh (50%), coconut coir fiber (64.7%; Doğramacı & Aydın, Citation2020). The saturation efficiency of other related fibers is Jute (62.1%), luffa 55.1%, date palm fiber 38.9%, rice straw 66.6% (Korese & Hensel, Citation2016); eucalyptus fibers 71% (Abohorlu Doğramacı et al., Citation2019), jute fiber pads 78%, and sackcloth 69% (Alam et al., Citation2017). The results of the current study revealed that the cotton fiber bundle has a better performance compared with other existing evaporative cooling materials. Therefore, it is a good alternative for cooling purposes.
3.6. Coefficient of performance
The coefficient of performance of the developed multi-stage cooler in April was 52.2. The coefficient of performance of the current cooler was compared with other previous works as stated in Table . As the coefficient of performance tells about the ratio of the amount of cooling per the input energy for the water pump and air fan, the cooler has a good COP.
Table 3. Comparison of the COP of present work with related work
4. Conclusion
Evaporative cooling is an ancient technology that has been used since pre-scientific eras to cool buildings since about 2500 B.C. in Egypt. Over the years, evaporative cooling systems have evolved gradually from their primitive forms to advanced systems. The performance of an evaporative air conditioning system is highly dependent on the type of evaporative cooling pad materials used. In the present work, a multi-stage solar-powered evaporative cooler was developed by using a novel bundle of cotton fiber as a pad material and vapor adsorbent material. The working fluid (water) flows from the top to the bottom reservoir by a combined effect of capillary diffusion and gravitational force. In the current study, the fundamental performance of the cooler (temperature reduction, relative humidity increment, saturation efficiency, cooling capacity, and coefficient of performance) was tested experimentally. Compared with other existing cooling pad material cotton fiber has a good wicking ability and water-holding capacity which leads to increased mass and heat transfer. The presence of water vapor adsorbent between the two pads increases the cooling performance of the evaporative cooler. This is because the adsorbent reduces the latent load of the humid air. As revealed from the study the evaporative coolers’ cooling capacity can be increased by employing more cooling pad stages and water vapor adsorbent material until the air becomes saturated with water vapor. Since the developed solar evaporative cooling system has a good air cooling performance, therefore, it could be used by small-scale farmers, wholesalers, and retailers for the cooling and storage of horticultural products such as tomatoes to reduce postharvest loss.
Nomenclature
Cp specific heat capacity of air, kJ/kg k
T dry bulb temperature of the air, °C
RH relative humidity of air, %
Q cooling capacity, W
A cross-sectional area of the cooling pad, m2
V frontal air velocity, m/s
Tidinlet dry bulb temperature, °C
Todoutlet dry bulb temperature, °C
Twbwet-bulb temperature, °C
Abbreviations
Sw percentage of water absorbed, g/g
mwfiber wet mass, g
mdfiber dry mass, g
SEsaturation efficiency, %
mamass flow rate of air, kg],
COP coefficient of performance
Wf power consumption of air fan, W
Wppower consumption of water pump, W
Greek symbols
the density of air, kg/m3
Author Contribution Statement
Tadelle N. Mekonen: Conceived and designs the experimental set-up; performed the experimental testing; Analyzed and interpreted the result; Wrote draft the paper.
Mulugeta A. Delele: Conceived and designs the experimental set-up; Supervised the research; Revised the draft paper.
Sisay W. Molla: performed the experimental testing; Revised the draft paper.
Disclosure statement
No potential conflict of interest was reported by the author(s).
Additional information
Funding
Notes on contributors

Tadelle N. Mekonen
Tadelle N. Mekonen, Email: [email protected]
Mulugeta A. Delele
Mulugeta A. Delele, [email protected]
References
- Abdel-Fadeel, W. A., & Attalla, M. (2014). Experimental study on the palm fiber using as a direct evaporative cooler pad. Int. J. Mech. Mechatronics Eng, 14(4), 14–14.
- Abohorlu Doğramacı, P., Riffat, S., Gan, G., & Aydın, D. (2019). Experimental study of the potential of eucalyptus fibers for evaporative cooling. Renew. Energy, 131, 250–260. https://doi.org/10.1016/j.renene.2018.07.005
- Alamdari, P., Saedodin, S., & Rejvani, M. (2020). Do non-metallic material and radiation shields affect the operation of direct evaporative cooling systems? Int. J. Refrig, 114, 98–105. https://doi.org/10.1016/j.ijrefrig.2020.02.038
- Alam, M. F., Sazidy, A. S., Kabir, A., Mridha, G., Litu, N. A., & Rahman, M. A. (2017). An experimental study on the design, performance, and suitability of evaporative cooling system using different indigenous materials. AIP Conf. Proc, 1851. https://doi.org/10.1063/1.4984704
- Amer, O., Boukhanouf, R., & Ibrahim, H. G. (2015). A Review of Evaporative Cooling Technologies. Int. J. Environ. Sci. Dev, 6(2), 111–117. https://doi.org/10.7763/ijesd.2015.v6.571
- Budaev, Z. B., Livanova, A. V., Meshcheryakov, E. P., Isupova, L. A., & Magaev, O. V. (2019). Influence of the composition of the adsorbent ‘hygroscopic salt/aluminum oxide’ on its physicochemical properties. IOP Conf. Ser. Mater. Sci. Eng, 597(1), 3–10. https://doi.org/10.1088/1757-899X/597/1/012025
- Cruz, J., Leitão, A., Silveira, D., Pichandi, S., Pinto, M., & Fangueiro, R. (2017). Study of moisture absorption characteristics of cotton terry towel fabrics. Procedia Eng, 200, 389–398. https://doi.org/10.1016/j.proeng.2017.07.055
- Daǧtekin, M., Karaca, C., Yildiz, Y. I., Başçetinçelik, A., & Paydak, Ö. (2011). The effects of air velocity on the performance of pad evaporative cooling systems. African J. Agric. Res, 6(7), 1813–1822. https://doi.org/10.5897/AJAR10.1110
- Das, B., Das, A., Kothari, V. K., Fanguiero, R., & de Araújo, M. (2007). Moisture transmission through textiles: Part I: Processes involved in moisture transmission and the factors at play. Autex Res. J, 7(2), 100–110.
- Delele, M. A., Ngcobo, M. E. K., Getahun, S. T., Chen, L., Mellmann, J., & Opara, U. L. (2013). Studying airflow and heat transfer characteristics of a horticultural produce packaging system using a 3-D CFD model. Part I: Model development and validation. Postharvest Biol. Technol, 86, 536–545. https://doi.org/10.1016/j.postharvbio.2013.08.014
- Dhamneya, A. K., Rajput, S. P. S., & Singh, A. (2018). Thermodynamic performance analysis of a direct evaporative cooling system for increased heat and mass transfer area. Ain Shams Eng. J, 9(4), 2951–2960. https://doi.org/10.1016/j.asej.2017.09.008
- Dmitriev, A. V., Madyshev, I. N., Kharkov, V. V., Dmitrieva, O. S., & Zinurov, V. E. (2021). Experimental investigation of fill pack impact on the thermal-hydraulic performance of evaporative cooling tower. Therm. Sci. Eng. Prog, 22, 100835. https://doi.org/10.1016/j.tsep.2020.100835
- Doğramacı, P. A., & Aydın, D. (2020). Comparative experimental investigation of novel organic materials for direct evaporative cooling applications in hot-dry climate. J. Build. Eng, 30, 101240. https://doi.org/10.1016/j.jobe.2020.101240
- Fikri, B., Sofia, E., & Putra, N. (2020). Experimental analysis of a multistage direct-indirect evaporative cooler using a straight heat pipe. Appl. Therm. Eng, 171, 115133. https://doi.org/10.1016/j.applthermaleng.2020.115133
- Fouda, A., & Melikyan, Z. (2011). A simplified model for analysis of heat and mass transfer in a direct evaporative cooler. Appl. Therm. Eng, 31(5), 932–936. https://doi.org/10.1016/j.applthermaleng.2010.11.016
- Gunhan, T., Demir, V., & Yagcioglu, A. K. (2007). Evaluation of the Suitability of Some Local Materials as Cooling Pads. Biosyst. Eng, 96(3), 369–377. https://doi.org/10.1016/j.biosystemseng.2006.12.001
- John, R. Taylor. (2015). An Introduction to Error Analysis_ The Study of Uncertainties in Physical Measurements.
- Kashyap, S., Sarkar, J., & Kumar, A. (2021). Performance assessment of dual-mode evaporative cooler for futuristic climatic scenarios considering climate change effect. J. Build. Eng, 42, 103043. https://doi.org/10.1016/j.jobe.2021.103043
- Kasso, M., & Bekele, A. (2016). Post-harvest loss and quality deterioration of horticultural crops in Dire Dawa Region, Ethiopia. Journal of the Saudi Society of Agricultural Sciences. https://doi.org/10.1016/j.jssas.2016.01.005
- Khater, E.-S. G. (2014). Performance of Direct Evaporative Cooling System under Egyptian Conditions. J. Climatol. Weather Forecast, 2(2). https://doi.org/10.4172/2332-2594.1000119
- Khobragade, N. N., & Dr, S. C. (2016). Experimental Performance of Different Evaporative Cooling Pad Material of Direct Evaporative Cooler in Hot and Dry Region. Int. J. Innov. Technol. Res, 4(3), 2920–2923.
- Korese, J. K., & Hensel, O. (2016). Experimental evaluation of bulk charcoal pad configuration on evaporative cooling effectiveness. Agric. Eng. Int. CIGR J, 18(4), 11–21.
- Kumar, S., Salins, S. S., Reddy, S. V. K., & Nair, P. S. (2021). Comparative performance analysis of a static & dynamic evaporative cooling pads for varied climatic conditions. Energy, 233, 121136. https://doi.org/10.1016/j.energy.2021.121136
- Laknizi, A., Ben Abdellah, A., Faqir, M., Essadiqi, E., & Dhimdi, S. (2021). Performance characterization of a direct evaporative cooling pad based on pottery material. Int. J. Sustain. Eng, 14(1), 46–56. https://doi.org/10.1080/19397038.2019.1677800
- Laknizi, A., Mahdaoui, M., Ben Abdellah, A., Anoune, K., Bakhouya, M., & Ezbakhe, H. (2019). Performance analysis and optimal parameters of a direct evaporative pad cooling system under the climate conditions of Morocco. Case Stud. Therm. Eng, 13, 100362. https://doi.org/10.1016/j.csite.2018.11.013
- Lal Basediya, A., Samuel, D. V. K., & Beera, V. (2013). Evaporative cooling system for storage of fruits and vegetables - A review. J. Food Sci. Technol, 50(3), 429–442. https://doi.org/10.1007/s13197-011-0311-6
- Liao, C. M., & Chiu, K. H. (2002). Wind tunnel modeling the system performance of alternative evaporative cooling pads in Taiwan region. Build. Environ, 37(2), 177–187. https://doi.org/10.1016/S0360-1323(00)00098-6
- Liao, C. M., Singh, S., & Sen, T. (2008). Journal of Environmental Science and Health, Part A: Toxic/Hazardous Substances and Environmental Engineering Characterizing the performance of alternative evaporative cooling pad media in thermal environmental control applications. Journal of Environmental Science and Health, 2015, 1391–1417. https://doi.org/10.1080/10934529809376795
- Makule, E., & Dimoso, N. (2022). Precooling and Cold Storage Methods for Fruits and Vegetables in Sub-Saharan Africa — A Review. Horticulturae, 8(9), 1–15. https://doi.org/10.3390/horticulturae8090776
- Manuwa, S. I., & Odey, S. O. (2012). Evaluation of pads and geometrical shapes for constructing evaporative cooling system. Mod. Appl. Sci, 6(6), 45–53. https://doi.org/10.5539/mas.v6n6p45
- Maurya, R., Shrivastava, N., & Shrivastava, V. (2014). Performance evaluation of alternative evaporative cooling media. Int. J. Sci. Eng. Res, 5(9), 676–684.
- Nada, S. A., Elattar, H. F., Mahoud, M. A., & Fouda, A. (2020). Performance enhancement and heat and mass transfer characteristics of direct evaporative building free cooling using corrugated cellulose papers. Energy, 211, 118678. https://doi.org/10.1016/j.energy.2020.118678
- Ndukwu, M. C., & Manuwa, S. I. (2014). Review of research and application of evaporative cooling in preservation of fresh agricultural produce. International Journal of Agricultural and Biological Engineering, 7, 85–102. https://doi.org/10.3965/j.ijabe.20140705.010
- Okada, K., Nakanome, M., Kameshima, Y., Isobe, T., & Nakajima, A. (2010). Water vapor adsorption of CaCl2-impregnated activated carbon. Mater. Res. Bull, 45(11), 1549–1553. https://doi.org/10.1016/j.materresbull.2010.07.027
- Olosunde, W. A., Igbeka, J. C., & Olurin, T. O. (2009). Performance evaluation of absorbent materials in the evaporative cooling system for the storage of fruits and vegetables. Int. J. Food Eng, 5(3). https://doi.org/10.2202/1556-3758.1376
- Sipho, S., & Tilahun, S. W. (2020). Potential causes of postharvest losses, low-cost cooling technology for fresh produce farmers in Sub-Saharan Africa. African J. Agric. Res, 16(5), 553–566. https://doi.org/10.5897/ajar2020.14714
- Solomon, Ioan., Ribeiro, A. M., Santos, Jo˜ao C., Loureiro, Jos´e M., Rodrigues, Al´ırio E., Sandu, Ion., & M˘amalig, Ioan. (2013). Adsorption equilibrium of water vapor on activated carbon and alumina and carbon and alumina impregnated with hygroscopic salt. Turkish J. Chem, 37(3), 358–365. https://doi.org/10.3906/Kim-1206-40
- Tejero-González, A., & Franco-Salas, A. (2021). Optimal operation of evaporative cooling pads: A review. Renew. Sustain. Energy Rev, 151, 111632. https://doi.org/10.1016/j.rser.2021.111632
- Tso, C. Y., & Chao, C. Y. H. (2012). Activated carbon, silica-gel and calcium chloride composite adsorbents for energy efficient solar adsorption cooling and dehumidification systems. Int. J. Refrig, 35(6), 1626–1638. https://doi.org/10.1016/j.ijrefrig.2012.05.007
- Velasco-Gómez, E., Tejero-González, A., Jorge-Rico, J., & Rey-Martínez, F. J. (2020). Experimental investigation of the potential of a new fabric-based evaporative cooling pad. Sustain, 12(17). https://doi.org/10.3390/su12177070
- Wijaksana, H., Winaya, I. N. S., Sucipta, M., Ghurri, A., & Suarnadwipa, N. (2018). The investigation on cooling capacity and CELdek material pad classification of evaporative cooling pad system using different pad material with water temperature and water discharge variations. AIP Conf. Proc, 1983, 1–7. https://doi.org/10.1063/1.5046215
- Xuan, Y. M., Xiao, F., Niu, X. F., Huang, X., & Wang, S. W. (2012). Research and application of evaporative cooling in China: A review (I) - Research. Renew. Sustain. Energy Rev, 16(5), 3535–3546. https://doi.org/10.1016/j.rser.2012.01.052
- Yu, Q. Y., Zhao, H., Sun, S., Zhao, H., Li, G., & Li, M. (2019). Characterization of MgCl2/AC composite adsorbent and its water vapor adsorption for solar drying system application. Renew. Energy, 138, 1087–1095. https://doi.org/10.1016/j.renene.2019.02.024
- Zakari, M. D., Abubakar, Y. S., Muhammad, Y. B., Shanono, N. J., Nasidi, N. M., Abubakar, M. S., Muhammad, A. I., Lawan, I., & Ahmad, R. K. (2016). Design and construction of an evaporative cooling system for the storage of fresh tomato. ARPN J. Eng. Appl. Sci, 11(4), 2340–2348.
- Zheng, X., Ge, T. S., & Wang, R. Z. (2014). Recent progress on desiccant materials for solid desiccant cooling systems. Energy, 74(1), 280–294. https://doi.org/10.1016/j.energy.2014.07.027